“After having studied aphids during half a century I have met riddles difficult to solve and questions difficult to answer. These questions are concerning the following fields: The choice of host plants, host alternation, variation in sizes of populations, morphology, geographical distribution, palaeontology and evolution” Ole E. Heie (Reference Heie2009)
The peculiar features of the geographical distribution of aphids in the Late Eocene formed the riddle that drew the senior author to this problem. One of the considerable differences in the composition between the Baltic and Rovno amber faunas of the same age is the fact that aphids are underrepresented in the Rovno fauna. They make up 4–6.5 % of all insects (excluding Entognatha) in the representative Baltic amber collections from Sambia (Sontag Reference Sontag2003; Perkovsky et al Reference Perkovsky, Rasnitsyn, Vlaskin and Taraschuk2007) and only 2.8 % in the representative Rovno amber collection of the Schmalhausen Institute, National Academy of Sciences of Ukraine; the source area of Late Eocene Baltic amber was Russo–Scandia (northern Subparatethys Sedimentation Province). The source area of Late Eocene Rovno amber is the Volyn region on the south coast of Subparatethys (Ivanov et al. Reference Ivanov, Melnitsky and Perkovsky2016, fig.1), so the climate of the Rovno amber forest (Ukraine) was warmer than that of the Baltic amber (Perkovsky et al. Reference Perkovsky, Zosimovich, Vlaskin and Penney2010).
These differences are especially notable in the representation of Germaraphis Heie, Reference Heie1967, which is a dominant genus of late Eocene amber aphids. These aphids make up 5.7 % of all the insects (excluding Entognatha) in the representative collection of Baltic amber from Sambia that is stored in Brighton, and 2.4 % of all the insects in the small section of a representative collection of Rovno amber (see Perkovsky et al. Reference Perkovsky, Rasnitsyn, Vlaskin and Rasnitsyn2012). The modern counterparts of the longirostred ant-attended aphids of the genus Germaraphis (Perkovsky Reference Perkovsky2011; LaPolla et al. Reference LaPolla, Dlussky and Perrichot2013) are aphids of the genus Stomaphis Walker, Reference Walker1870, which are obligate ant symbionts (Heie & Wegierek Reference Heie and Wegierek2009).
1. Aphids: geographical distribution and the evolution of their life cycle – prevailing hypotheses
The patterns of geographical and geological distribution are among the main riddles of aphids. Scientists have been puzzled by the fact that the world distribution of aphids does not seem to follow a typical pattern (there are numerous species in temperate zones of the northern hemisphere, but only a few species in the southern tropics). It was Heie (Reference Heie1994) who discussed a previously formulated hypothesis about the adaptation of aphid life cycles to seasons of temperate zones (Bodenheimer & Swirski Reference Bodenheimer and Swirski1957). He also commented on the hypothesis focusing on difficulties aphids encounter in the tropics (Dixon Reference Dixon1985, Reference Dixon, Minks and Harrewijn1987; Dixon et al. Reference Dixon, Kindlmann, Lepš and Holman1987), indicating that the low species richness in tropics is due to high plant diversity, making the chance of successful migration between conspecific hosts very small. Challenging the latter, Heie stated that aphids in tropics are monophages. He was equally skeptical of Dixon's other proposals, such as the method of comparing areas rich and poor in fauna (Heie Reference Heie1994). Moreover, Heie pointed out that most aphids occuring in tropical and southern temperate countries are introduced, cosmopolitan and, most often, polyphagous species and they cope quite well with the climate. He also showed that the tendency to avoid the tropics is not equally strong in different aphid families (Fig. 1).
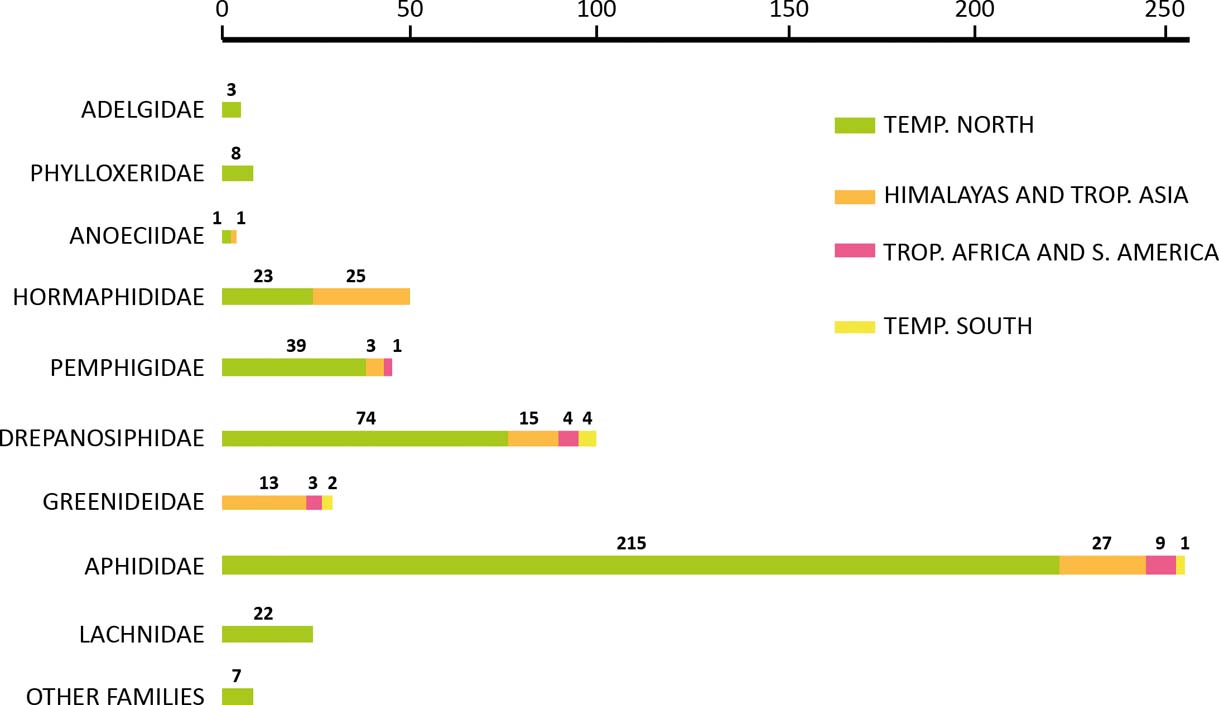
Figure 1 The recent distribution of aphids: geographical origins of 500 aphid genera. (After Heie Reference Heie1994).
It should be noted that all other Hemiptera Sternorrhyncha (coccids, white flies and psyllids) do remarkably well in the tropics, although their sizes, mobility and feeding habits are comparable to those of aphids (Evans Reference Evans2008; García Morales et al. Reference García Morales, Denno, Miller, Miller, Ben-Dov and Hardy2016; Ouvrard Reference Ouvrard2016).
According to Heie (Reference Heie1994), aphids became cyclically parthenogenetic while adapting to the warm climate of the Mesozoic. This idea seems quite plausible, because during most of the long history of aphids' existence (more than 250 million years), the temperatures were considerably higher than those which have prevailed for the last 25 million years. Originally, life cycles were adapted to dry periods (Heie Reference Heie1994). However, this does not deny the fact that aphids, except during the earliest stages of their history, prefer cooler climates (Kania & Wegierek Reference Kania and Wegierek2008; Martin et al. Reference Martin, Skidmore and Stilwell2016).
The geological and climatic changes of the second half of the Cenozoic resulted in the differentiation of climatic zones on the Earth. This, in turn, influenced the development of northern hemisphere vegetation. Herbs and open grassy areas became available and aphids adapted their life cycle (and some morphs; Heie Reference Heie1994) to maximise the use of those plant nutrients (Dixon Reference Dixon1985). It may also help to explain the fact that the Aphididae family, the aphid group richest in number of species, did not spread into the tropics. According to Heie (Reference Heie1994), Drepanosiphidae, Hormaphididae and Greenideidae had already adapted to a warmer climate in the Tertiary, and the south temperate aphid fauna is more similar to that of the Tertiary than to that of the northern temperate zones; great evolutionary events take place in northern hemisphere (but see below). The southern hemisphere aphid fauna became quite distinct because of the tropical barrier (Heie Reference Heie1994). We disagree with Heie (Reference Heie1994) that Drepanosiphidae, Hormaphididae and Greenideidae “had already adapted to a warmer climate in the Tertiary”. The majority of drepanosiphid subfamilies (all including myrmecophiles, see below) were never found in the tropics or temperate regions of the Southern Hemisphere.
Another hypothesis (e.g., Blackman & Eastop Reference Blackman and Eastop2006) stressed that the stimuli that induce the sexual phase (a long scotophase and decreasing temperature; Kawada Reference Kawada, Minks and Harrewijn1987) are lacking in the tropics, and the very lack of stimuli makes aphids lose the sexual phase of the life cycle, further resulting in their loss of the evolutionary potential dependent on the gene recombination. However, the occurrence of sexuales is a complicated phenomenon not triggered exclusively by a photoperiod. For example, Mindarus Koch, Reference Koch1857 in Poland produces sexuales in June when the day is longest (Heie Reference Heie1980, p. 85: “…. sexuales are born in early summer or in mid summer…”). The aphids living under bark in complete darkness (e.g., Stomaphis) also produce sexuales in autumn (Depa et al. Reference Depa, Kanturski, Junkiert and Wieczorek2015). A similar situation was observed in the genus Pterochloroides Mordvilko, Reference Mordvilko1914 and the species P. persicae (Cholodkovsky, Reference Cholodkovsky1899) in which, at the same latitudes, sexuales occurred in the mountains; whereas anholocyclic forms prevail in wet lowlands. (“The place of collection [of sexuals] – Isfahan (Esfahan) is located at 1590 m above sea level, in the foothills of the Zagros mountain range. Isfahan has an arid climate with hot summer and cold winter. This type of climate is favourable for the occurrence of sexual forms of aphids. Although P. persicae species, a serious pest of orchards, is reported from numerous locations, records of sexuales are extremely rare.” Wieczorek et al. Reference Wieczorek, Kanturski and Junkiert2013, p. 98).
Another example of the complicated control of aphid sexuality represents the fact that anholocyclic species transferred from warm climates do not restore sexual forms, and the attempts to produce them under controlled experimental conditions are mostly unsuccessful (Durak & Durak Reference Durak and Durak2015: in Cinara tujafilina (del Guercio, Reference del Guercio1909), breeding males were obtained only in the 126th generation in the climatic chamber). To cope with environmental uncertainty, aphids have evolved different types of polyphenism (Tagu et al. Reference Tagu, Sabater-Muñoz and Simon2005), so finding alternative stimuli responsible for inducing the sexual phase is not impossible. We remember that the primary aphid adaptation has been the one towards using the dry vs. wet season alteration. Nevertheless, most aphids lose their sexual forms in the tropics, a fact deserving explanation (see discussion below). In a temperate climate, when days become shorter in the autumn, most aphid clones switch to the production of sexual forms, because only eggs and not nymphs can survive low temperatures (Simon et al. Reference Simon, Rispe and Sunnucks2002). In contrast, the warmer, equable climate of the Cretaceous through to the Eocene should permit nymphal hibernation, which suggests different stimuli were used to induce the sexual phase at that time as compared to the present. This point of view confirms the fact that aphid males are unexpectedly frequent in Burmese amber (Poinar & Brown Reference Poinar and Brown2005).
All three mentioned hypotheses concentrated on external factors in trying to account for the distribution of aphids. In this paper, it also seems important to indicate the internal limitations which are due to the strong dependence of aphids on their symbionts. This has influenced the geographical distribution of aphids, at least since the mid-Cretaceous, as was suggested in our earlier paper (Perkovsky Reference Perkovsky2012).
2. Internal factors influencing aphid distribution
2.1. Importance of functional integration with symbionts and biggest extinction
Aphids and symbiont genotypes have evolved to form a functionally integrated whole, so that in many contexts both genomes need to be considered (Hales et al. Reference Hales, Tomiuk, Wörhmann and Sunnucks1997). At low latitudes, aphids usually switch to obligate parthenogenesis, and if their initial genetic diversity is low, they soon lose their evolutionary potential. It may prove to be one of the factors determining the world distribution of the group. Some aphid families (Hormaphididae and Greenideidae) managed to cross the climatic barrier, thanks to individual adaptations discussed below.
Fossil remains of aphids are known from at least the Middle Triassic (but see Szwedo et al. Reference Szwedo, Lapeyrie and Nel2015); however, they have been mostly described on the basis of isolated wings. The only exception is the family Dracaphididae (Naibioidea), but its assignment to aphids is disputable (Heie & Wegierek Reference Heie and Wegierek2011). Slightly more numerous aphids are known from the Jurassic, including the oldest undoubted aphid body fossils; but overall, the fossil record is rather scarce and a significant abundance of aphid fossils begins from the Early Cretaceous.
We believe that at least some of the answers are linked to yet another riddle of aphids, which as yet has attracted no attention: their mass extinction in the Late Cretaceous. Most Cretaceous families became extinct at the end of the epoch. The richest Cenozoic fauna is known from Baltic amber (Late Eocene) and, with the exception of Elektraphididae, represents exclusively Recent families, which originated at the beginning of the Cenozoic (with two families dating from the end of the Cretaceous; for stratigraphic distribution of families with number of species included, see Heie & Wegierek Reference Heie and Wegierek2011, p. 71).
2.2. Susceptibility of primary obligate aphid symbionts to high temperature
Currently, the distribution of aphids (Heie Reference Heie1994; Fig. 1) is largely limited by the low thermal tolerance of their primary obligate symbionts – the bacteria Buchnera aphidicola (Fig. 2 a, b) (phylum Proteobacteria, class Gammaproteobacteria, family Enterobacteriaceae) (Perkovsky Reference Perkovsky2012). The genome of B. aphidicola is one seventh of that of its close relative, Escherichia coli, and these endosymbionts are not able to live outside their host (Charles & Ishikawa Reference Charles and Ishikawa1999). It is believed that associations between insects and their primary endosymbionts are the result of a single ancient infection (Buchner Reference Buchner1965). Within Recent aphids, only representatives of the superfamily Phylloxeroidea do not contain endosymbiotic microorganisms; Adelgoidea may harbour gamma- and/or beta-proteobacterial endosymbionts, but not Buchnera (Michalik et al. Reference Michalik, Szklarzewicz, Jankowska and Wieczorek2014). The gamma-proteobacterium Buchnera aphidicola is present in all existing aphids of the superfamily Aphidoidea [classification after Heie & Wegierek Nieto (Nafría & Favret Reference Nieto Nafría and Favret2011)], except for some representatives of the family Hormaphididae.
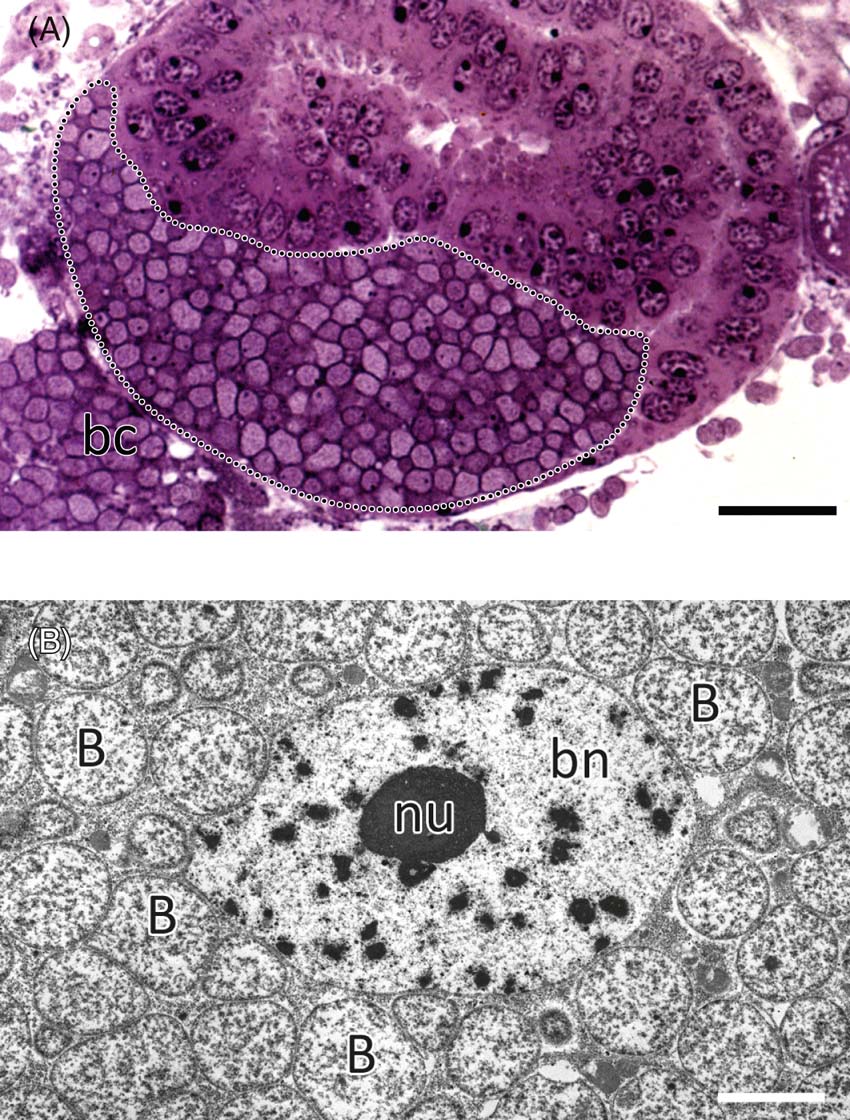
Figure 2 Pemphigus spyrothecae, posterior pole of the young embryo (cross-section), containing numerous cells of bacterium Buchnera aphidicola (encircled with dotted line). bc=bacteriocyte. Methylene blue. Scale bar=20 µm. (B) Pemphigus spyrothecae, fragment of the bacteriocyte with bacterium Buchnera aphidicola (B). bn=bacteriocyte nucleus, nu=nucleolus. TEM. Scale bar=4 µm.
Mutualism between aphids and B. aphidicola has reached such an extent that both partners have become completely interdependent and neither can reproduce in the absence of the other; the young adult pea aphid Acyrthosiphon pisum (Harris, Reference Harris1766), maintained at 21°C, had 1.2×107 B. aphidicola cells (Simonet et al. Reference Simonet, Duport, Gaget, Weiss-Gayet, Colella, Febvay, Charles, Viñuelas, Heddi and Calevro2016). These intracellular symbionts synthesise most of the irreplaceable amino acids (Douglas Reference Douglas2006), as well as riboflavin and other compounds that are absent in the phloem sap (Chen et al. Reference Chen, Lai and Kuo2009). During their intracellular existence [it has been firmly proven that symbiotic Buchnera was already present in the common ancestors of all Aphidoidea (Moran et al. Reference Moran, Munson, Baumann and Ishikawa1993)] for over 200 million years (Unterman et al. Reference Unterman, Baumann and McLean1989; Moran & Baumann Reference Moran and Baumann1994; Martinez-Torres et al. Reference Martinez-Torres, Buades, Latorre and Moya2001; Moran et al. Reference Moran, McCutcheon and Nakabachi2008), Buchnera aphidicola underwent genetic degradation, which is the almost inevitable fate of all obligate intracellular symbionts. The genome of Buchnera became simpler and lost its plasticity as a result of the loss of mobile genetic elements and repetitions (Perkovsky Reference Perkovsky2012). Genetic recombinations are strongly limited in Buchnera populations, which are isolated in the host bacteriocytes and pass through a bottleneck with each new host generation (Charles & Ishikawa Reference Charles and Ishikawa1999). The ability to repair DNA is also limited in Buchnera (the part of the genome that provided much of this ability was lost during the shift to symbiosis); the genotype has accumulated too many harmful mutations (a classical example of Muller's ratchet; in some lachnids it has degraded to only 362 protein-coding genes, which is very close to the minimum number even for symbionts that have lost most of their metabolic functions and its repair machinery is more strongly reduced than in other strains (Pérez-Brocal et al. Reference Pérez-Brocal, Gil, Ramos, Lamelas, Postigo, Michelena, Silva, Moya and Latorre2006).
It is a firmly established fact (Rasnitsyn & Quicke Reference Rasnitsyn and Quicke2002; Kania & Wegierek Reference Kania and Wegierek2008; Heie & Wegierek Reference Heie and Wegierek2011; Perkovsky Reference Perkovsky2012) that as early as the Cretaceous (in contrast to the Late Jurassic), aphids were abundant in areas with a warm temperate climate (such as the Early Cretaceous Baissa Lagerstätte (125–129 Mya; nearly 50 species) and Cretaceous Siberian (85–107 Mya) and Canadian (78–79 Mya) ambers) and very rare south of 40°N palaeolatitude. They are absent in Charentese amber (99–100 Mya); very rare in Early Cretaceous Lebanese (130 Mya; three species, four specimens), Spanish (105 Mya; one specimen) and Late Cretaceous New Jersey (92 Mya; two specimens) ambers; and uniform and rather scant in Burmese amber (98–99 Mya, just above the Early–Late Cretaceous boundary) (Fig. 3). And yet we are not aware of any attempts to explain this fact before Perkovsky (Reference Perkovsky2012). Interestingly, the two Cretaceous aphids from the Southern Hemisphere were also recorded from two sites that supposedly had a temperate-seasonal climate (Rayner et al. Reference Rayner, Bamford, Brothers, Dippenaar-Schoeman, Mckay, Oberprieler and Waters1997; Drinnan & Chambers Reference Drinnan and Chambers1986): in the Orapa Lagerstätte (90–94 Mya) in Botswana and Koonwarra (115–118 Mya); and well inside the Antarctic Circle of the day (Rich et al. Reference Rich, Rich, Wagstaff, McEwen-Mason, Douthitt and Gregory1989) in Victoria, Australia (Martin et al. Reference Martin, Skidmore and Stilwell2016).

Figure 3 Geographic distribution of the studied Cretaceous assemblages (from Rasnitsyn et al. Reference Rasnitsyn, Bashkuev, Kopylov, Lukashevich, Ponomarenko, Popov, Rasnitsyn, Ryzhkova, Sidorchuk, Sukatsheva and Vorontsov2016, with changes). Map modified from Blakey Reference Blakey2011. 1=Levantine amber belt; 2=Russia, Eastern Siberia, Baissa; 3=Spain Escucha Formation; 4=Spain Utrillas Group; 5=Russia, Taimyr Peninsula, Begichevo Formation; 6=France, Charentese amber; 7=Myanmar, Hukawng Valley; 8=Russia, Taimyr Peninsula, Dolgan Formation; 9=United States, Raritan Formation ‘New Jersey amber’; 10=Russia, Taimyr Peninsula, Kheta Formation; 11=Russia, Far East, Obeshchayushchiy; 12=Canada, Alberta and Manitoba, Foremost Formation.
A major impact of heat on cellular function stems from the degradation of protein tertiary structures. Most cellular organisms have the capacity to respond to heat stress by directing transcription and/or translation to the production of chaperones (Feder & Hoffman Reference Feder and Hofmann1999). They function by ‘forcibly' giving other proteins the right configuration. Buchnera has four transcriptional promoters, which affect the expression of the five heat-shock genes (Wilcox et al. Reference Wilcox, Dunbar, Wolfinger and Moran2003) that are responsible for chaperone synthesis. Some genes, which are usually activated in other organisms under extreme heat, are constitutively highly expressed in Buchnera, a feature that has been interpreted as an adaptation that was made in order to maintain the function of proteins that had been destabilised by mutational accumulation (Wilcox et al. Reference Wilcox, Dunbar, Wolfinger and Moran2003). Buchnera is doomed to the continuous accumulation of its mutation load because it cannot perform homological recombination, and because of the loss of many of the genes that are important for DNA reparation and replication (Funk et al. Reference Funk, Wernegreen and Moran2001; van Ham et al. Reference van Ham, Kamerbeek, Palacios, Rausell, Abascal, Bastolla, Fernández, Jiménez, Postigo, Silva, Tamames, Viguera, Latorre, Valencia, Morán and Moya2003). Moreover, it also lacks the chaperones that smooth out the negative effects of mutations somewhat by folding the partly incorrect proteins in the correct way. It is believed that the chaperones of intracellular bacteria are subject to a relatively strong pressure of selection (van Ham et al. Reference van Ham, Kamerbeek, Palacios, Rausell, Abascal, Bastolla, Fernández, Jiménez, Postigo, Silva, Tamames, Viguera, Latorre, Valencia, Morán and Moya2003). The density of Buchnera in the host decreases sharply if the temperature rises from as little as 25°–30°C, even in aphids that were collected on the subtropical island of Taiwan (Chen et al. Reference Chen, Lai and Kuo2009). This decrease in Buchnera density is accompanied by the fecundity of the host dropping to a small fraction of its former values.
When Dunbar et al. (Reference Dunbar, Wilson, Ferguson and Moran2007) measured the activity of Buchnera genes in three laboratory lines of A. pisum (Aphididae) at a normal temperature and when overheated, it turned out that in one of the three lines the heat shock in Buchnera does not activate the expression of ibpA, which encodes a small heat-shock protein that is not only present in bacteria but in almost all living organisms. The ibpA heat-shock protein is detrimental in cool conditions, perhaps due to its binding with other Buchnera proteins (Burke et al. Reference Burke, Fiehn and Moran2010). The line that had this anomaly was produced only five years ago from a ‘normal' line in which heat shock activates ibpA. It was revealed that the anomalous response to overheating was caused by a mutation (most probably single) that appeared less than five years ago. In these bacteria, adenine is absent in the chromosome at the site to which the promoter σ32, which activates the gene ibpA (and other heat-shock genes), attaches when the animal is under stress (Dunbar et al. Reference Dunbar, Wilson, Ferguson and Moran2007).
Investigations with other laboratory lines of the pea aphid revealed another case of the independent appearance of the same mutation in one of them and indicated that the mutation appeared in two lines in 2001 and 2005 (Dunbar et al. Reference Dunbar, Wilson, Ferguson and Moran2007). It turned out that the mutation is rather common (present in 13–21 % of individuals) in Acyrthosiphon pisum from the northern US states of New York and Wisconsin, which have rather cold summers with daytime temperatures sometimes never reaching 35°? for several years; but which were absent in Arizona and Utah, where daytime temperatures in the summer normally reach 35°C.
Experiments have confirmed that the gene ibpA is not activated by overheating in all of the mutants, although it is activated by overheating in all of the lines that lack this mutation. The other heat-shock genes were activated in exactly the same way in both the mutant and normal Buchnera. It has also been proven experimentally that a mutation arising in the bacterial symbiont affects the viability and reproductive potential of the symbiotic superorganism. Aphids that had both mutant and normal Buchnera were grown under different temperature conditions – some at a stable temperature of 15°C or 20°C and some heated for three hours to 35.5°C two days after hatching. It turned out that after overheating, the aphids that had mutant symbionts became almost infertile, while the aphids with normal symbionts bred quite well (only 17 % were infertile). However, at a constant temperature of 15°C or 20°C, the aphids that had mutant symbionts had clear advantages. They began breeding earlier and produced more offspring on average.
Additional experiments have shown why brief overheating is so fatal to aphids with mutant Buchnera. When the temperature rises to 35.5°C or higher, many bacterial symbionts die rapidly and one aphid ends up having only about 1000 bacteria (about 0.001 of the norm) and these insects weigh only 40 % as much as the control group (‘normal' aphids weigh 80 % as much as the control group after overheating; Dunbar et al. Reference Dunbar, Wilson, Ferguson and Moran2007). Thus, the mutant Buchnera gives advantages to aphids at constant and rather low temperatures, while ‘normal' Buchnera can be beneficial only when overheating is possible (chaperones make up 10 % of all of their protein).
The direction of selection in natural populations of aphids may probably change depending on the climate, and even on the season; sometimes aphids with normal symbionts are favoured by selection and sometimes aphids with mutant symbionts are favoured. As a result, both genetic variants are preserved in natural populations and none of them can completely replace the other. However, this polymorphism is especially effective if overheating happens rather seldom. Recently, it was found (Moran & Yun Reference Moran and Yun2015) that Buchnera in most A. pisum matrilines evolve to the heat-sensitive genotype after extended laboratory culture at 15–20°C, reflecting a high mutation rate at this allele and selection favouring this genotype under cool conditions. The replacement of Buchnera not producing ibpA by a heat-tolerant mutant (injection after heat treatment was used) greatly increased the heat tolerance of the A. pisum aphid, demonstrating a major effect of the symbiont genotype on host fitness (Moran & Yun Reference Moran and Yun2015).
2.3. The dependence of aphid's sexual generation on Buchnera susceptibility to overheating
Approximately 30–40 % of the aphid species that have been described include both cyclical-parthenogenic and asexual clones (Fig. 4) (Moran Reference Moran1992; Dixon Reference Dixon1998; Simon et al. Reference Simon, Stoeckel and Tagu2010). The strictly asexual species (or clones) tend to be distributed in low-latitude regions. Strictly asexual generations have only been described in approximately 3 % of all aphid species (Simon et al. Reference Simon, Rispe and Sunnucks2002). Dramatically varying population sizes make parthenogenesis much more beneficial. The same species (Macrosiphum rosae (Linnaeus, Reference Linnaeus1758) have cyclic parthenogenesis in Norway, cyclic in some populations and obligate in other populations in England and exclusively obligate in the Canary Islands (Wőhrmann & Tomiuk Reference Wőhrmann and Tomiuk1988); similar differences are also known for many other species (Hales et al. Reference Hales, Tomiuk, Wörhmann and Sunnucks1997; Dedryver et al. Reference Dedryver, Hulle, Le Gallic, Caillaud and Simon2001).

Figure 4 Life cycles of the aphid.
The genetic diversity of M. rosae also sharply decreases from north to south (Wőhrmann & Tomiuk Reference Wőhrmann and Tomiuk1988), and has a negative correlation with the average temperature of July. The cold resistance of overwintering eggs is considered to be very important, but live parthenogenic individuals can resist temperatures above −8°C or −10°C (Simon et al. Reference Simon, Stoeckel and Tagu2010).
In species that had been introduced into the tropics, only obligate parthenogenesis is found. Penetrating into the tropics, aphids simply lose the sexual phase of their life cycle, as well as their evolutionary potential to the extent of its dependence on gene recombination. Those that omit sexual reproduction from their life cycle become encumbered by the negative effects of exclusively asexual reproduction; e.g., mutation load and sluggish adaptation (Smith & Maynard-Smith Reference Smith and Maynard-Smith1978; Otto Reference Otto2009; McDonald et al. Reference McDonald, Rice and Desai2016). It should be noted that in nonaphidid aphids (sensu Heie & Wegierek Reference Heie and Wegierek2009), sexual reproduction is very closely linked to the primary host plant (Hardy et al. Reference Hardy, Peterson and Dohlen2015). Hardy et al. (Reference Hardy, Peterson and Dohlen2015) revealed a very strong correlation between heteroecy and holocycly (i.e. sexual reproduction) in nonaphidid aphids, which is indicative of the presence of formidable developmental constraints that oppose adaptation to changing host-plant communities.
We suggest that the loss of the full life cycle of aphids in the tropics is due to Buchnera's low tolerance to overheating, and by the peculiar life cycle and sex determination of aphids. The fact that 10–30 parthenogenic generations of aphids are followed by only one sexual generation means that the prerequisites are high for switching to obligate parthenogenesis when the probability of the overheating of the sexual generation is also high; whilst the decreased viability of males additionally decreases their chances of surviving overheating. Male aphids have a single X chromosome and all of the mutations associated with this chromosome manifest themselves. As a result, the number of malformations is huge (Depa et al. Reference Depa, Kanturski, Junkiert and Wieczorek2015) and the general viability of males is decreased. This is the likely explanation of the two-fold mortality rate of male embryos from the recessive mutations that are lethal at any temperature. Let us also remember that even in parthenogenic females without mutations of ibpA in Buchnera, 17 % of the individuals became infertile after three hours of overheating; in males the proportion should be much higher. Warm temperate regions demonstrate an instable equilibrium of holocyclic and anholocyclic clones of comparable abundancy. The sexual generation develops here in rather cool environments with a low risk of overheating, in contrast to the parthenogenetic ones which experienced some 40 % loss, even in northern Germany (Ma et al. Reference Ma, Hau and Poehling2004).
Further south, the loss becomes inevitable in sexual generations, as well resulting in the overwhelming prevalence of obligate parthenogenetic populations. In our opinion, the loss of sexual generation due to overheating accelerates genetical drift and reduces the already low genetic diversity in populations. The occasional complete death of sexual generations due to genetic problems in the symbiotic bacteria reduces genetic recombination dramatically beyond the level at which loss of sexual generations cannot be compensated by the two-fold fecundity preference of the thelytoky (female-producing parthenogenesis).
There are also aphids with sexual forms occurring concurrently with parthenogenetic females (e.g., many species in the family Greenideidae; Tomiuk et al. Reference Tomiuk, Hales, Wőrhmann and Morris1991); we discuss this phenomenon below.
2.4. Modifications of aphids in tropics to save a sexual generation
In our opinion, the cyclic parthenogenesis can be preserved in the tropics when the males are freed from their dependence on Buchnera, when sexual forms occurr concurrently with parthenogenetic females and/or when the sex-determination system changes.
Let us discuss the families Hormaphididae and Greenideidae, which have, respectively, half or all of their genera distributed in the tropics. Hormaphidid aphids are gall-forming species that often possess soldier nymphs. Males and the egg-laying females that mate with them are wingless and dwarfish in Hormaphididae and aphagous in many species. The best known, most successful and best studied hormaphidid aphids are cerataphidines. Their colonies include up to a million individuals that cause galls in their primary hosts. The diversity and relative abundance of cerataphidines in the tropics are determined by their sociality, which made it possible for them to evolve aphagous males (Kurosu et al. Reference Kurosu, Buranapanichpan and Aoki2006) which, in turn, makes them independent from Buchnera. The life cycle of gall-forming cerataphidines makes the search for mates easier; hence, adult males of the species that have Buchnera can be short-lived and not dependent on additional feeding.
In those species of Cerataphis Lichtenstein, Reference Lichtenstein1882 that have preserved Buchnera, the males and egg-laying females are aphagous, and so their survival is not affected by the death of Buchnera from overheating. This made it possible for some cerataphidines to replace Buchnera with yeast-like symbionts (Pyrenomycetes; see Fukatsu et al. Reference Fukatsu, Aoki, Kurosu and Ishikawa1994). Three genera of Cerataphidinae are an example, with one of these genera still preserving two species of Buchnera. The nymphs of egg-laying females that have the fungus are not aphagous and have a long rostrum, while in the males or females without the fungus, the rostrum is always short or absent. The importance of male aphagy is confirmed by the fact that it also evolved independently in the second family of gall-forming social aphids, Eriosomatidae. Moreover, in social eriosomatids not only males, but also soldiers, are aphagous, which could increase the evolutionary potential of the taxon.
Thus, the high mortality of Buchnera from overheating leads to the replacement of the bacterial symbiont with a fungal symbiont, the emergence of aphagous males or the increased role of secondary symbionts of the genus Serratia (Chen et al. Reference Chen, Montllor and Purcell2000; Montllor et al. Reference Montllor, Maxmen and Purcell2002; Russell & Moran Reference Russell and Moran2006), which are also transmitted transovarially (Michalik et al. Reference Michalik, Szklarzewicz, Jankowska and Wieczorek2014).
There is no reason to infer the obligate association between cerataphidine males and Buchnera lost as early as the Miocene, since all cerataphidines, in contrast to the aphagous eriosomatid males, still have fully-fledged bacteriocytes (Braendle et al. Reference Braendle, Miura, Bickel, Shingleton, Kambhampati and Stern2003). The late breakup of the association between Cerataphidinae and Buchnera (it can be assumed that their sexual generation was not yet aphagous in the Miocene) can explain the extinction of American cerataphidines. Cerataphidines are widespread in the Old World in all areas in which their primary host plant, Styrax, is present. Although Styrax species are still abundant in the New World, cerataphidines became extinct after the Middle Miocene.
To preserve sexual reproduction, greenideids have not only switched to the concurrent existence of sexual and parthenogenic generations that decrease inbreeding (Tomiuk et al. Reference Tomiuk, Hales, Wőrhmann and Morris1991), which is important because of greenideid poor flight capacity. They also changed the XX–X0 sex-determination system that is characteristic of all other aphids. The genetic sex-determination system of some greenideids, which is unique amongst Paraneoptera (Gavrilov-Zimin et al. Reference Gavrilov-Zimin, Stekolshchikov and Gautam2015), was discovered by Hales (Reference Hales1989). She found multiple X chromosomes fused with autosomes (X1+A, X1, X2+A, X2) in Schoutedenia Rübsaamen, Reference Rübsaamen1905 (Fig. 5), so as all four X chromosomes appear to be of different length (Hales et al. Reference Hales, Tomiuk, Wörhmann and Sunnucks1997). The study of male meiosis has shown that X1 or X2, apparently at random, gave up its attached autosome which was then lost in the inviable (not X-bearing) spermatocyte (Hales et al. Reference Hales, Tomiuk, Wörhmann and Sunnucks1997). This special system of sex determination should kill half of the gametes which, hence, should be counterbalanced with another, yet unknown, adaptation.
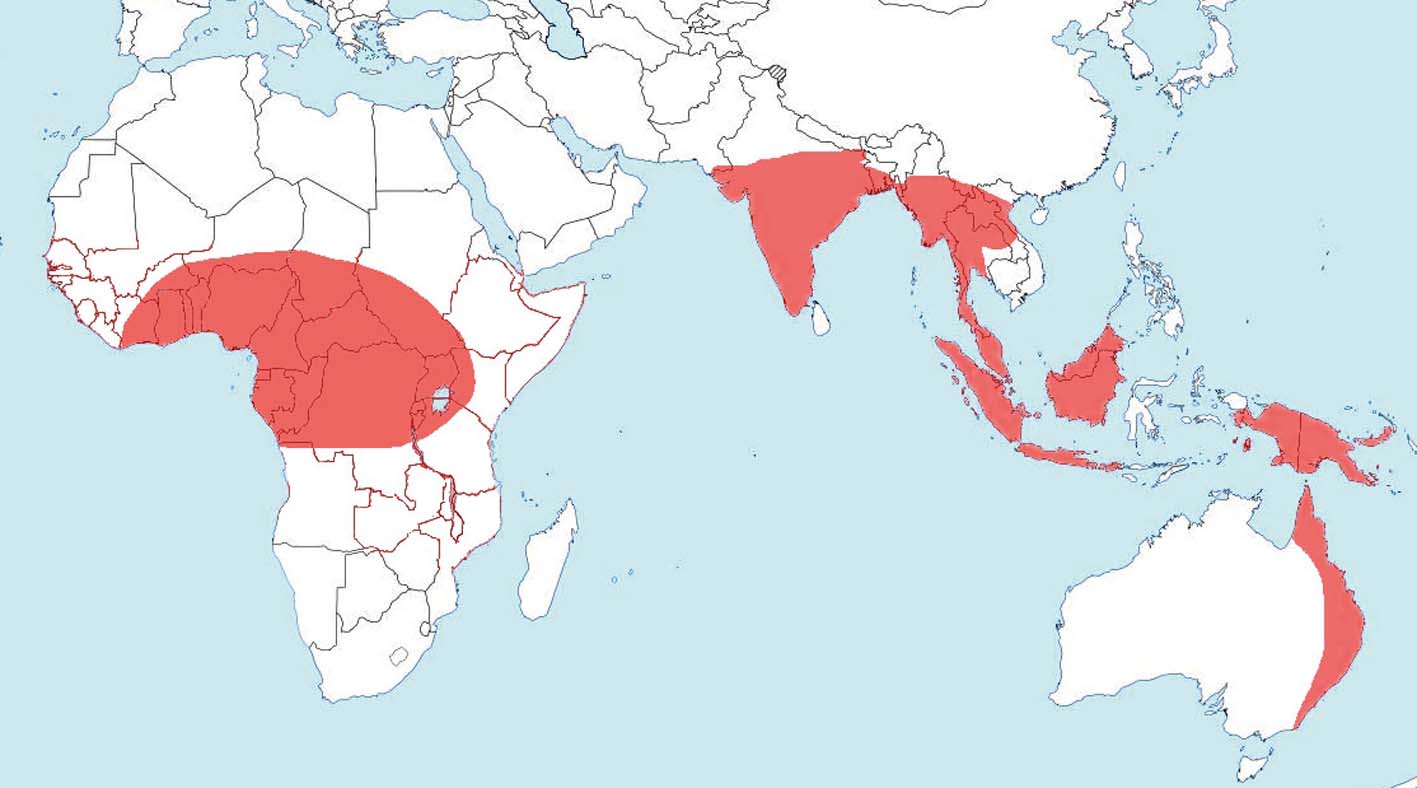
Figure 5 The world distribution of Schoutedenia ralumensis Rübsaamen, Reference Rübsaamen1905 (data from Blackman & Eastop Reference Blackman and Eastop2006).
The X chromosomes of aphids consist mostly of heterochromatin, and their composition, apart from rDNA (located in X chromosomes in all aphids), is not discussed. Thus, males have half as much rDNA as females (a single copy rather than two copies). This may be the explanation for the two-fold mortality rate of male embryos from mutations that are lethal at any temperature and for the very low growth rate of males (Dixon et al Reference Dixon, Holman, Thieme, Nieto Nafría and Dixon1998). In Schoutedenia, rDNA is transferred to paired autosomes; i.e., the amount of rDNA is doubled and any lethal recessive mutations of rDNA have no effect on most males. Thus, at least the main advantage that has been acquired by Schoutedenia is fairly obvious. The shift of rDNA into autosomes is probably of principal importance; male lachnids of the genus Stomaphis also have two X chromosomes, but 32 % of adult males show various morphological aberrations, many of them very striking (Depa et al. Reference Depa, Kanturski, Junkiert and Wieczorek2015, figs 11–13), which have not yet been recorded in Schoutedenia.
Because of above adaptations, greenideids have advantages at high temperatures, but in cooler climates they are poor competitors. The family has appeared in the fossil record since the Early Miocene (Fig. 6) and do so in abundance (greenideines reached 18 % of all of the aphids recorded in Rubielos de Mora, Aragon), thus apparently reflecting the increasing seasonality of the climate beginning with the Oligocene. During the Middle Miocene Climatic Optimum, the number of recorded greenideines reached 25 % of all of the aphids recorded in Stewart Valley, Nevada; five of the six aphids recorded from the pre-optimum Radoboj, Croatia, also belong to Greenideinae; extant greenideids (all subfamilies) account for 18 % of aphids found only on Java; north of the Equator, they are more poorly represented (at most 13 % in India and 5 % in Sri Lanka). The family is absent in the fauna of North America and Western Eurasia after the Middle Miocene (Fig. 6). Greenideids are easily distinguishable and, therefore, the absence of records of this family from pre-Miocene sites, including Fushun and other Early and Middle Eocene localities that had high temperatures in July, actually supplies evidence of the relatively young age of this taxon.

Figure 6 The recent (orange) and past (red) world distribution of Greenideinae.
As indicated above, an alternative explanation of the scarcity of aphids in the tropics is the huge diversity of plants, which makes it difficult to locate a particular host plant species (Dixon Reference Dixon1998). Dixon (Reference Dixon1998) supported this claim by noting the abundance of greenideids in a plantation near Kolkata at 30°C and above. The fact that the aphids in the plantation had switched to obligate parthenogenesis (Agarwala & Dixon Reference Agarwala and Dixon1986) apparently did not bother him. We suppose that the sexual reproduction retained in the Himalaya secures the evolutionary potential of northern Indian populations.
Middle Miocene greenideines are known also in Stavropol, Russia (Fig. 6). However, the family make up only 3 % of all aphids there, although the fossil site also occurred not far from the Miocene optimum at that time. The genus Stomaphis was dominant in Stavropol (Wegierek & Mamontova Reference Wegierek and Mamontova1993). Most representatives of this genus probably survive overheating rather well (many of them live under bark). They include dwarf arostrate non-feeding males (many of them living on underground parts of the trunk base or under bark; Depa et al. Reference Depa, Mróz and Szawaryn2012) and also obligatory myrmecophiles, which was probably the main reason of their success. Lasius ants, the permanent symbionts of Stomaphis, also increased their abundance in Stavropol in parallel to that of Stomaphis (Dlussky Reference Dlussky1981; Wegierek & Mamontova Reference Wegierek and Mamontova1993). Greenideidae have never evolved strong association with ants.
The riddle of aphid biogeography has one more aspect, a poverty of the southern temperate realm in aphids. To try and decipher it, we need to consider the event of the Late Cretaceous aphid extinction (Fig. 7), which has never been discussed by aphidologists. The extinction at the Cretaceous–Palaeogene boundary is traditionally explained by floristic changes; moreover, Late Cretaceous aphids were considered to be similar to Early Cretaceous ones (Kononova Reference Kononova1976). Recently, Early Cretaceous aphids have been studied (Heie & Wegierek Reference Heie and Wegierek2011b) much better than Late Cretaceous ones, and the results are very different. Eighty-four Early Cretaceous aphid species are now known from 414 specimens, whilst 44 Late Cretaceous species are known from only 61 specimens. Since thelaxids, which are not known from the Late Cretaceous (Fig. 7), exist to this day, it appears that none of the Early Cretaceous superfamilies, and only 58 % of families (seven out of 13), became extinct at the time of the Cretaceous Terrestrial Revolution. Three strictly Early Cretaceous families are known from only seven specimens, and if the Early and Late Cretaceous faunas were studied at similar levels, the proportion of families that are known to have become extinct in the Early Cretaceous would be even smaller.
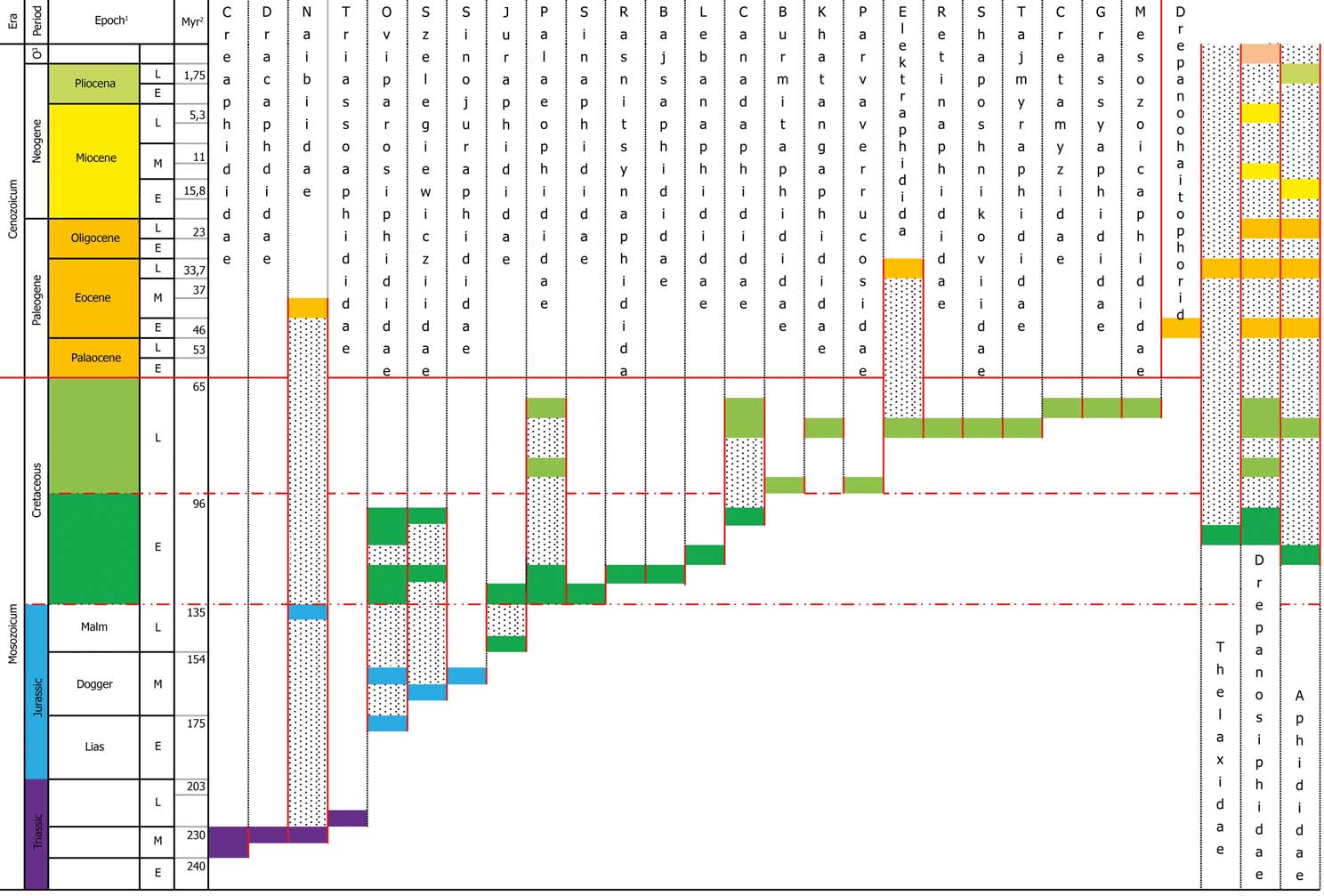
Figure 7 The distribution of aphid families in geological time.
The Cretaceous Terrestrial Revolution is characterised by the rise of the angiosperms (Dilcher Reference Dilcher2000; Davies et al. Reference Davies, Barraclough, Chase, Soltis, Soltis and Savolainen2004; Magallon & Castillo Reference Magallon and Castillo2009; Wang et al. Reference Wang, Moore, Soltis, Bell, Brockington, Alexandre, Davis, Latvis, Manchester and Soltis2009; Coiffard et al. Reference Coiffard, Gomez, Daviero-Gomez and Dilcher2012). It had huge ecological consequences, including the extinction of the closest counterparts of the angiosperms Bennettitales and Czekanowskiales, as well as most Ginkgophyta and most Gnetophyta (Ponomarenko Reference Ponomarenko1998). This means changes can be expected in the family composition of Late Cretaceous aphids as compared to Early Cretaceous ones. However, the much deeper aphid extinction at the Cretaceous/Paleogene (K/Pg) boundary cannot be explained by the floristic changes. Half of the 30 plant morphotypes represented by more than 30 specimens were preserved on the best studied sites of North America (western North Dakota and western South Dakota), at the transition from the Cretaceous to the Palaeogene (Johnson Reference Johnson2002). The floristic associations of the Russian Far East and high latitudes of Asia and North America also provide no evidence of any considerable reorganisation of the terrestrial flora at the Cretaceous–Palaeogene boundary (Herman et al. Reference Herman, Akhmetiev, Kodrul, Moiseeva and Yakovleva2009).
In contrast to the modest floristic turnover at the K/Pg boundary, the aphid faunal transformation was drastic there. In the Kheta Formation of Taimyr (Santonian), 78 % of the aphid families are extinct (Perkovsky & Wegierek Reference Perkovsky and Wegierek2015). For comparison, all of the Kheta Formation arthropod assemblages include 16 % extinct families, and non-aphids include only 11 % extinct families; 20 % of all arthropod families are unknown from the Early Cretaceous (Burmese and Charentese amber dated near the boundary of the Early and Late Cretaceous are calculated as Early Cretaceous; Rasnitsyn et al. Reference Rasnitsyn, Bashkuev, Kopylov, Lukashevich, Ponomarenko, Popov, Rasnitsyn, Ryzhkova, Sidorchuk, Sukatsheva and Vorontsov2016). In Canadian amber (Campanian), the proportion of extinct aphid families is 83 % and in all of the aphid fauna of the Late Cretaceous, this proportion is 86 %.
To sum up, 79 % of Late Cretaceous aphid families became extinct at the Cretaceous–Palaeogene boundary. Almost all tajmyraphidoids (five families) and palaeoaphidoids (two families), as well as three of the five families of aphidoids known from the Late Cretaceous, also became extinct. Aphids of the first two superfamilies lacked the siphunculi that had a signalling function and without these siphunculi, it proved impossible for aphids to survive the increased pressure of predators. This was particularly fatal because almost all Late Cretaceous aphids had a long or very long rostrum. It is well known that even among members of the same genus, a longer rostrum increases both the time required to escape a predator and the rate of the destruction of one colony by predators by a considerable factor (Shingleton et al. Reference Shingleton, Stern and Foster2005). This particularly concerns the longirostred aphids (Depa et al. Reference Depa, Kanturski, Junkiert and Wieczorek2015). In the Late Cretaceous, only three (Cretamyzus Heie, 1992 (Cretamyzidae), Calgariaphis Heie, 1992 in Heie & Pike Reference Heie and Pike1992 (Mesozoicaphididae) and larvae II type) of the 12 morphotypes of wingless aphids had the proboscis not longer than the body (Kononova Reference Kononova1977; Heie & Pike Reference Heie and Pike1992); and even in winged aphids, such as Nordaphis Kononova, Reference Kononova1977 (Drepanosiphidae, Lizeriinae) and Palaeoforda Kononova, Reference Kononova1977 (Aphidomorpha incertae sedis) the proboscis is longer than the body, and it almost reaches the apex of the abdomen in some representatives of other families.
At the same time, elongation of the rostrum was a pre-adaptation to symbiosis with ants (Shingleton et al. Reference Shingleton, Stern and Foster2005; Perkovsky Reference Perkovsky2012). It is noteworthy that the only recent genus of aphids that has a rostrum longer than the body (Stomaphis) depends entirely on ants. Although the trophobiotic association of Late Eocene longirostred aphids with formicines and dolichoderines have also been proven (Perkovsky Reference Perkovsky2010), in the Late Cretaceous there were simply no ants that had colonies large enough to ‘keep' aphids (Dlussky & Rasnitsyn Reference Dlussky and Rasnitsyn2007; LaPolla et al Reference LaPolla, Dlussky and Perrichot2013): even now, aphid milkers from small colonies (hundreds of specimens) never protect their symbionts (Novgorodova Reference Novgorodova2015), and homopteran-tending ants are more likely to be dominant, with large colonies of 104–105 workers (Oliver et al. Reference Oliver, Leather and Cook2008).
3. The Cretaceous–Paleogene (K–Pg) extinction event and the development of a symbiotic relationship between aphids and ants
It is well known that aphids are one of the main sources of hydrocarbon food for ants, particularly for Formicinae and Dolichoderinae (Stadler & Dixon Reference Stadler and Dixon2005; Oliver et al. Reference Oliver, Leather and Cook2008). At the same time, aphids form an important resource for a wide array of predators and parasites (Skaljac Reference Skaljac and Vilcinskas2016). In reward, the ants protect the tended aphids from competitors including aphidophages (Stadler & Dixon Reference Stadler and Dixon2005), acting both as a symbiont and a predator (Rosengren & Sundström 1991; Sakata, Reference Sakata1995; Offenberg Reference Offenberg2001). Among almost 5000 aphid species (Blackman & Eastop Reference Blackman and Eastop2006), some 60 % are visited by ants (Stadler Reference Stadler, Dettner, Bauer and Vőlkl1997). Unless tended by ants, aphid colonies fail quickly, due to predation (Watanabe et al. Reference Watanabe, Murakami, Yoshimura and Hasegawa2016).
Worth mentioning is that prior to the large ant colonies having evolved with their efficient ant–aphid mutualism, the aphids remain unprotected before the growing ant predation. The origin of the aphid trophobiosis with large colonies of Formicinae and Dolichoderinae resulted in the steep decline of aphids left beyond that ant–aphid symbiotic network. By at least the basal Eocene, ant proportions in the entomofauna increased sharply (Fig. 8), up to 6.9 % in the Early Eocene of the Green River and 13.1 % in the Middle Eocene of Messel, as compared with 0.0001–1.5 % in the Late Cretaceous (Dlussky & Rasnitsyn Reference Dlussky and Rasnitsyn2007). In the Cretaceous, all of the representatives of extant subfamilies occupied positions that were subordinate to Armaniinae and Sphecomyrminae. Moreover, the scape is very short in armaniines and in most sphecomyrmines, which has even raised some doubts about their sociality (Dlussky Reference Dlussky1983; Dlussky & Rasnitsyn Reference Dlussky and Rasnitsyn2007); whilst the long scapus of some Cretaceous fossils suggests group recruitment and socially advanced behaviour amongst stem-group ants (Perrichot et al. Reference Perrichot, Wang and Engel2016). The fauna did not include any evident dominants (Dlussky & Rasnitsyn Reference Dlussky and Rasnitsyn2007).
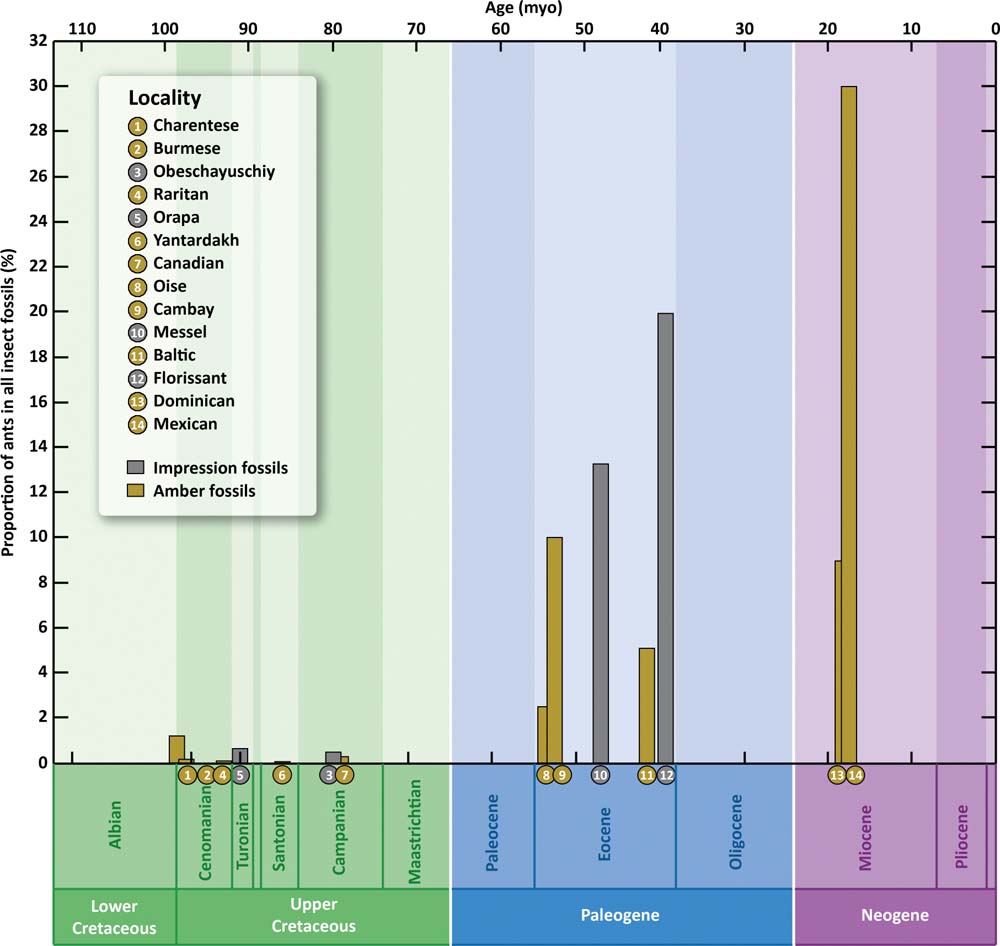
Figure 8 Ant abundance in different Lagerstättes (from all insects). (Redrawn from LaPolla et al. Reference LaPolla, Dlussky and Perrichot2013, fig. 1, with many changes)
In contrast, in the Eocene, the dolichoderines and formicines were absolute dominants in all fossil myrmecofaunas (except for the Messel, Eckfeld and Mo-clay assemblages, dominated, for taphonomic reasons only, by giant ants; Rust & Andersen Reference Rust and Andersen1999; Archibald et al. Reference Archibald, Johnson, Mathewes and Greenwood2011). The evolutionary success of dolichoderines and formicines is considered to be directly linked to their symbiosis with homopterans (Wilson & Hőlldobler Reference Wilson and Hőlldobler2005; Dlussky & Rasnitsyn Reference Dlussky and Rasnitsyn2007). Two evident dominants of the myrmecofauna emerged as early as the Early Eocene of the Green River – the dolichoderine or formicine Eoformica pinguis (Scudder, Reference Scudder1877) (40 % of the ants) and dolichoderine Dolichoderus kohlsi Dlussky & Rasnitsyn, Reference Dlussky and Rasnitsyn2003 (25 % of the ants) (Dlussky & Rasnitsyn Reference Dlussky and Rasnitsyn2003; LaPolla & Greenwalt Reference LaPolla and Greenwalt2015). This means that highly social species with a large family size had appeared (Dlussky & Rasnitsyn Reference Dlussky and Rasnitsyn2007). The dominants of Eocene myrmecofauna belonged to extant aphidophilous ant subfamilies (with the above taphonomically-caused exceptions), while the dominants of aphidofauna were myrmecophilous. Unfortunately, the Paleocene ants are practically unknown: the Sakhalinian amber is currently dated as Middle Eocene (Kodrul Reference Kodrul1999; Baranov et al. Reference Baranov, Andersen and Perkovsky2015), and its impoverished ant fauna (Dlussky & Rasnitsyn Reference Dlussky and Rasnitsyn2007; La Polla et al. 2013) is probably due to its origin amongst wetland.
Thus the extinction of Late Cretaceous aphid families should be associated with the emergence of new dominants among ants from among the representatives of the subfamilies Formicinae and Dolichoderinae, which were symbionts of homopterans and developed a characteristic dolichoderine–formicine proventriculus that allows the crop to store large amounts of liquid (Eisner & Wilson Reference Eisner and Wilson1952); it regulates the amount of this liquid which passes into the midgut and in this way separates the individual supply of food in the midgut from the communal supply in the crop.
The predation of these ants and of other aphidophages that appeared by the basal Cenozoic, such as syrphids (not known from the Cretaceous as was believed earlier; Perkovsky & Makarkin Reference Perkovsky and Makarkin2015; Popov Reference Popov2015), proved to be such a serious problem to aphids that the only survivors were those that either became poorly accessible to ants in some way (e.g., by having found shelters in galls) or could transform ant predation into symbiosis.
Adaptation to symbiosis with ants occurred in the extratropical regions of the Northern Hemisphere and because of the climatic limitations discussed above, this adaptation failed to cross the tropical belt and reach the south temperate realm, which otherwise could have served as another heaven for the ant–aphid mutualistic system. The Cenozoic dominant groups of aphids, especially Aphididae (Heie Reference Heie1994), failed to cross into the tropics and colonise the temperate regions of the Southern Hemisphere, where only five relict genera with a short rostrum and well-developed siphunculi remain. These genera are also shown to be ancient due to the divisible variations in the number of chromosomes within one genus (in other aphids the number of chromosomes is usually stable within one genus, especially if the genus is small). The degree of the depletion of the aphid fauna of the temperate regions of the southern continents is so great that only a dozen species of the undoubtedly relict genus Neophyllaphis Takahashi, Reference Takahashi1920 have been recorded on gymnosperms. None of these genera have any connection to ants. Similarly, it has been suggested that the diversification of ants was also the cause of the extinction of Limaiinae (Chrysopidae are homopteran feeding) (Khramov et al. Reference Khramov, Liu, Zhang and Jarzembowski2016).
4. Conclusions
At least since the Cretaceous Terrestrial Revolution, the geographical distribution of aphids, particularly in the Northern Hemisphere, has been strongly affected by the low thermal tolerance of their obligatory bacterial symbiont, Buchnera aphidicola, which was why the aphids switched to obligate parthenogenesis in low latitudes.
Hormaphidids and greenideids penetrated into the tropics only after the Oligocene strengthening of climate seasonality, and the specialisations of the tropical representatives of these families did not allow them to spread further south (in the case of cerataphidines), or only allowed this spread in a few cases (in the case of greenideids).
Aphids suffered from the Mesozoic–Cenozoic boundary extinction event much more strongly than other insects. The extinction roughly coincided with the establishment of the tight symbiosis of aphids with formicine and dolichoderine ants, which was accompanied by the flourishing of all three groups. Because of the low overheating tolerance of Buchnera, the mutualistic ant–aphid system failed to cross the tropical belt during the Cenozoic. As a result, the native southern temperate aphid fauna consists now of seven genera only, five of which being Late Cretaceous relicts; some of them had relatives in Late Cretaceous amber of the Northern Hemisphere.
Ole Heie (Reference Heie1994) asked 22 years ago in his title “Why are there so few aphid species in the temperate areas of the southern hemisphere?” We hope that our paper proposes a new answer.
5. Acknowledgements
We would like to thank A. P. Rasnitsyn (Paleontological Institute, Moscow), for discussing the problems and the invaluable comments on the manuscript; the late G. M. Dlussky (Moscow State University), for his continuous encouragement and support in the studies on the history of ant–aphid relationships; V. N. Makarkin (Institute of Biology and Soil Sciences, Vladivostok), for helpful discussion on aphid predators; M. Kanturski (University of Silesia) and T. M. Kodrul (Geological Institute, Moscow), for their help in the literature search; and T. Szklarzewicz (Jagiellonian University) and Ł. Depa (University of Silesia) for reading the manuscript and their suggestions, which we incorporated into the study. We would also like to thank T. Szklarzewicz for the photographs of Buchnera. The authors thank the two anonymous reviewers, whose comments improved our work considerably.