Introduction
The giant sugarcane borer, Telchin licus (Drury, 1773) (Lepidoptera: Castniidae), is an economically important sugarcane pest in several countries of Central and South America, with reports of losses averaging 0.43% in weight and 0.66% in sucrose content for each 1% of infestation intensity (Mendonça et al., Reference Mendonça, Viveiros and Sampaio1996; Almeida et al., Reference Almeida, Dias Filho and Arrigoni2007). Originally from the Amazon region, T. licus is now widely spread in countries such as Bolivia, Brazil, Colombia, Ecuador, Guyana, Paraguay, Peru, Trinidad and Tobago, and Venezuela (Ríos and González, Reference Ríos and González2011; Arboleda et al., Reference Arboleda, Grossi de Sa and Valencia2014). In Colombia, Bustillo (Reference Bustillo2013) reports attacks by the giant sugarcane borer in the eastern part of the country (Orinoco River basin) and the Andean region.
Average adult T. licus are mid-sized moths with a robust body, and forewings between 80 and 100 mm in length (Miller, Reference Miller1986; González, Reference González2003). The giant sugarcane borer shares certain characteristics with butterflies, such as their diurnal or crepuscular habits and some morphological similarities (Miller, Reference Miller and Heppner1995). Larvae are borers of sugarcane stalks, which results not only in the destruction of basal internodes and in the formation of internal galleries, but also in the reduction of the stalk's sucrose content and the eventual death of the plant, affecting the crop's established population (Arboleda et al., Reference Arboleda, Grossi de Sa and Valencia2014). Their endophytic lifestyle hinders the effectiveness of chemical, mechanical, and biological control methods (Craveiro et al., Reference Craveiro, Gomes, Silva, Macedo, Lucena, Silva, Souza Júnior, Oliveira, Magalhães, Santiago and Grossi-de-Sa2010) and despite their economic importance, studies on taxonomy and bioecology are scarce, making it difficult to develop effective management techniques and possible control mechanisms (Fonseca et al., Reference Fonseca, Firmino, Coelho, Silva-Junior, Togawa, Pappas and Grossi-de-Sá2015).
Currently, T. licus is part of a species complex that includes Telchin syphax (Fabricius, 1775) and Telchin atymnius (Dalman, 1824) (González and Cock, Reference González and Cock2004; Moraes and Duarte, Reference Moraes and Duarte2009). The distribution of this complex is sympatric throughout the neotropical region, with morphological similarities among species and a wide range of hosts (Saccharum officinarum, Heliconiaceae, and Musaceae) (González and Cock, Reference González and Cock2004). The group seems to be underrepresented in public and private entomological collections in Colombia (González et al., Reference González, Andrade, Worthy and Hernández-Baz2017), since its species have very specific distributions and very marked territorial behaviours in comparison to other Lepidoptera families (García et al., Reference García, López and Turrent2019). Studies on morphology and kinship relationships with the species that make up the complex are not abundant (Miller, Reference Miller1986; González, Reference González2003).
Reports of the distribution of Telchin species and hosts in Colombia are variable, given the scarcity of informative morphological characters in the larval stage (Miller, Reference Miller and Stehr1987), which has generated confusion in the identification of the species T. licus and T. atymnius in the field, to the point that some references have erroneously described them as synonyms (Esquivel, Reference Esquivel1981; see González and Stüning, Reference González and Stüning2007). However, morphological studies of adults have defined their taxonomic status in the genus Telchin, differentiating these two species based on their genitalia and the absence in T. atymnius of orange spots on the lateral margin of the hindwings (González and Cock, Reference González and Cock2004; González and Stüning, Reference González and Stüning2007; Moraes and Duarte, Reference Moraes and Duarte2009).
Precise identification has been difficult since the T. licus complex is extremely variable throughout its geographical distribution, which is reflected in the description of up to twelve subspecies of T. licus, and six of T. atymnius in the Neotropics (Lamas, Reference Lamas1995; Miller, Reference Miller and Heppner1995). These subspecies were proposed mainly based on their geographical distribution and subtle differences in wing colour. However, little is known about their exact geographic distribution and variation, hosts, or phylogenetic relationships, having made their taxonomy confusing (Lamas, Reference Lamas1995; Miller, Reference Miller and Heppner1995; Moraes and Duarte, Reference Moraes and Duarte2009, Reference Moraes and Duarte2014; Silva-Brandão et al., Reference Silva-Brandão, Almeida, Moraes and Cônsoli2013) and resulting in identification errors. Appropriate identification is required as a basis for developing effective control and management methods (González et al., Reference González, Domagała and Larysz2013).
Considering the economic importance of the T. licus complex in the country and the insufficient knowledge on this complex, alternative techniques are required for the adequate identification of species and subspecies. Molecular markers, such as mitochondrial DNA (mtDNA), are characterized by a high rate of evolutionary change, high genetic polymorphism among conspecifics, and availability of universal primers (Simón et al., Reference Simón, Frati, Beckenbach, Crespi, Liu and Flook1994). Therefore, molecular markers are excellent candidates for solving problems at the taxonomic level where morphological data is non-conclusive, helping define species limits and identifying the origin of new populations (Silva-Brandão et al., Reference Silva-Brandão, Almeida, Moraes and Cônsoli2013). DNA barcoding based on cytochrome c oxidase subunit I (COI), the most widely used mtDNA genes to investigate phylogeographic patterns in insects (De Jong et al., Reference De Jong, Wahlberg, Van Eijk, Brakefield and Zwaan2011), has demonstrated to be an efficient tool to rapidly identify species across different taxa in insects (Lee et al., Reference Lee, Anderson, Tran-Nguyen, Sallam, Le Ru, Conlong, Powell, Ward and Mitchell2019). This method has been proven useful in identifying sugarcane associated insects, specifically in the case of stemborers (Lange et al., Reference Lange, Scott, Graham, Sallam and Allsopp2004; Barrera et al., Reference Barrera, Villamizar, Espinel, Quintero, Belaich, Toloza, Ghiringhelli and Vargas2017; Wang et al., Reference Wang, Wang, Lin, Ali, Fu, Huang, Gao and Wang2018). Therefore, the objective of this study, which integrates molecular analysis and conventional morphology, is to determine which Telchin species are associated to sugarcane and other alternative hosts, and to update the pest's status in the country by identifying its distribution in Colombia.
Methods
Sample collection
Castniid moth samples were collected in three main regions of Colombia; in the Orinoco region, represented by the departments of Casanare and Meta; in the Andean region, in the departments of Antioquia, Caldas, Nariño, and Valle del Cauca (in mountainous areas and on the Pacific coast); and in the Amazon region, in the department of Caquetá. Larvae, pupae, and adults were collected in sugarcane, plantain, and heliconia plantations and taken to the Colombian Sugarcane Research Centre (Cenicaña) for monitoring and subsequent identification (table 1).
Table 1. Location of sampling, geographical coordinates, host plants, and number of specimens of Telchin spp. collected in each Colombian municipality

Collected individuals were kept in a breeding room at an average temperature of 25.3 °C and relative humidity of 76.3%. The larvae were fed pieces of freshly cut sugarcane of the variety CC 01-1940. The cuttings were changed every 4 days to avoid the proliferation of fungi and bacteria. All individuals were monitored during 3–4 months until they finally emerged as images allowing taxonomic identification. Larvae that failed to complete the cycle were stored in 70% alcohol for DNA extraction.
Morphologic identification
For morphological identification, at least one adult was obtained for each locality with the exception of Antioquia. Subspecies identification was based on pictures and descriptions of type specimens (Boisduval, Reference Boisduval1875; Houlbert, Reference Houlbert1918; Joicey and Talbot, Reference Joicey and Talbot1925; Lamas, Reference Lamas1995; Salazar, Reference Salazar1999; González and Salazar, Reference González and Salazar2003) before any sequencing. On the other hand, as larvae do not offer clear diagnostic characters at the species level, molecular identification methods were used to differentiate them.
The distal portion of the abdomen was removed from male Telchin spp. adults and their genitalia extracted and treated for 24 hours with 10% potassium hydroxide (KOH) solution to remove the soft tissues and clarify the exoskeleton. Subsequently, the specimens were washed with 5% acetic acid and distilled water to neutralize the effect of the potassium hydroxide (Moraes and Duarte, Reference Moraes and Duarte2009). Images of the genitalia were taken with the clear Lumenera Scientific INFINITY camera attached to the stereoscope. The interpretation and description of the observed structures were based on Moraes and Duarte (Reference Moraes and Duarte2009).
DNA extraction, amplification, and sequencing
A subsample of the specimens collected at each location was made and a tissue sample was taken for DNA extraction (table 2). In the case of the larvae, the sample was extracted from the posterior abdominal area; in the case of the adults, a pair of legs were removed from the thorax. The DNA of the individuals was extracted using a commercial kit (DNeasy Blood & Tissue Kit, QIAGEN) and the DNA concentration was quantified by spectrophotometry (Nanodrop 1000, Thermo-Fisher) and electrophoresis in a 1% agarose gel using Syber® Safe (Thermo-Fisher) for visualization.
Table 2. Estimates of genetic diversity for populations of Telchin spp. included in this study, based on COI data

N, total number of individuals tested; h, number of haplotypes in each population; S, polymorphic sites; H d, haplotype diversity (± standard deviation); π, nucleotide diversity (± standard deviation).
Sequences taken from GenBank and/or Bold System: aSilva-Brandão et al. (Reference Silva-Brandão, Almeida, Moraes and Cônsoli2013), bRatnasingham and Hebert (Reference Ratnasingham and Hebert2007).
A fragment of the mitochondrial COI gene was amplified using the primers LepF1 5′-ATTCAACCAATCATAAAGATATTGG-3′ and LepR1 5′-TAAACTTCTGGATGTCCAAAAAATCA-3′ (Ratnasingham and Hebert, Reference Ratnasingham and Hebert2007). The polymerase chain reaction (PCR) was done by using GoTaq® 1× (Promega) Master Mix, 0.25 mM of each primer and 5 μl of DNA, in 30 μl of the final volume. The amplification protocol was as follows: initial denaturation at 94 °C for 1 min, followed by 34 cycles at 94 °C for 30 s, 51 °C for 1 min, and 72 °C for 1 min, with a final extension of 72 °C for 10 min. Its amplification was then verified by electrophoresis in a 1% agarose gel. The PCR products were sent to Macrogen®, USA, for sequencing.
The sequences were edited with the Geneious® 11.1.5 program, the quality of the electropherograms was analysed and the sequences with low quality and/or ambiguous bases were excluded, generating contigs of 638 pb each. The identity of the sequences was confirmed using one BLASTn, considering only homologous sequences with an E-value <0.001 and identity percentage >98% (NCBI, available online).
Later, all sequences were aligned in the MEGA program – version 10.1 (Kumar et al., Reference Kumar, Stecher, Li, Knyaz and Tamura2018) with the ClustalX algorithm (delay divergent cut off was 30%) (Thompson et al., Reference Thompson, Gibson, Plewniak, Jeanmougin and Higgins1997) – in which sequences obtained from GenBank and the Barcode of Life Data System (BOLD) were included (table 2). The program AliView 1.26 (Larsson, Reference Larsson2014) was used to verify that there were no stop codons in the final alignment.
Genetic diversity analysis
Mean genetic distances among all combined sequences, within and between species, were calculated using Kimura 2-parameters (K2P) distances (Kimura, Reference Kimura1980) using uniform rates among sites and 1000 bootstrap replicates to calculate standard error in MEGA 10.1 program. Diversity parameters were estimated for each Telchin species under study, defining populations according to their geographical location. To reduce the population size bias in the estimates of diversity and population structure, only localities with more than five individuals were used. The results were obtained from the DnaSP program v.5.10.01 (Rozas, Reference Rozas2009), and were summarized as the number of polymorphic sites (S), the number of haplotypes (h), the haplotype diversity (H d) – the probability that two randomly sampled alleles are different –, and the nucleotide diversity (π) – the average number of nucleotide differences per site in pairwise comparisons among DNA sequences (De Jong et al., Reference De Jong, Wahlberg, Van Eijk, Brakefield and Zwaan2011).
Network of haplotypes and phylogenetic inference
A network of haplotypes for each Telchin species was built using the Median-Joining algorithm on Network 10.1.0 (Bandelt et al., Reference Bandelt, Forster and Röhl1999). In this case, all sequences obtained (including GenBank accessions) were used, for a total of 126 sequences analysed (table 2). Phylogenetic relationships among COI sequences were estimated using Bayesian inference (BI) implemented in the version 3.2 MrBayes program (Ronquist and Huelsenbeck, Reference Ronquist and Huelsenbeck2003) and neighbour-joining (NJ) clustering algorithm (Saitou and Nei, Reference Saitou and Nei1987) using 10.1 MEGA (Kumar et al., Reference Kumar, Stecher, Li, Knyaz and Tamura2018). The robustness of the NJ was evaluated using the bootstrap method with 1000 repetitions. BI analysis was run with four chains for 106 generations and the current tree was saved to file every 1000 generations. The nucleotide substitution model was applied for the sequences (TIM2 + I) analysed according to the jModelTest. The initial 10% of the trees were discarded based on the burn-in. The results were analysed in Tracer v.1.7.1 (Rambaut et al., Reference Rambaut, Drummond, Xie, Baele and Suchard2018) to confirm the convergence among chains and the development of the run. Posterior probabilities (PP) were estimated as support of the nodes, where probabilities ≥95% indicate significant support. In the analysis, Paysandisia archon Burmeister and Riechia acraeoides (Guérin-Méneville) (Lepidoptera: Castniidae) (GenBank: KF491991 and GU828835, respectively) were used as the external taxa (outgroup) (Ratnasingham and Hebert, Reference Ratnasingham and Hebert2007). The tree obtained was visualized and edited in FigTree v.1.4.2 (Rambaut, Reference Rambaut2014).
Population structure analysis
An independent analysis of molecular variance (AMOVA) was performed (Excoffier et al., Reference Excoffier, Smouse and Quattro1992) for each Telchin species (T. licus and T. atymnius). The populations grouped according to the clades found in the phylogenetic analysis were compared and ranked based on their geographic distribution and taxonomic classification, making two independent analyses: (a) genetic variation among subspecies of T. licus from Colombia and Brazil and (b) as there is only one subspecies of T. atymnius in the Bold System, genetic variation was analysed among departments in Colombia and Costa Rica. The degree of the genetic structure of the data was interpreted by the indices of fixation (Φ) (Weir and Cockerham, Reference Weir and Cockerham1984) associated with the different hierarchical levels at which the variation found is distributed: among groups (F CT), among populations within groups (F SC), and for the overall data (F ST), where statistical significance was evaluated utilizing a randomized allelic permutation test (10,000 permutations) (Excoffier et al., Reference Excoffier, Smouse and Quattro1992). All statistical parameters and tests were calculated using Arlequin 3.5 (Excoffier and Lischer, Reference Excoffier and Lischer2010).
Results
Morphological identification
Moths of the T. licus complex have a dark brown, robust, and medium-sized body (50–80 mm of forewing length); the males’ frenulum is a single bristle and the retinaculum is formed by an expansion of the subcostal vein; on the other hand, the females’ frenulum consists of several bristles and different scales form an atypical retinaculum (Houlbert, Reference Houlbert1918; Miller, Reference Miller1986; Moraes and Duarte, Reference Moraes and Duarte2009). The forewings of both male and female moths are dark brown with a white band that crosses diagonally from the subcostal vein to the anal vein (A 2); they also have five small spots on the subapical margin. Hindwings are brown and have differential characteristics between species. The posterior wings of T. licus have an irregular transverse cream-coloured band that runs from the costal margin to the anal angle. Additionally, they have a lateral margin spot band with yellow/red spots in the hindwing (fig. 1a). The latter is the most notable characteristic that distinguishes T. licus from T. atymnius, which also presents an irregular transverse cream-coloured band in the hindwings that extends from the costal margin to the anal angle, but without yellow/red spots on these posterior wings (fig. 1b) (Vélez, Reference Vélez1997; González and Salazar, Reference González and Salazar2003; Moraes and Duarte, Reference Moraes and Duarte2009).
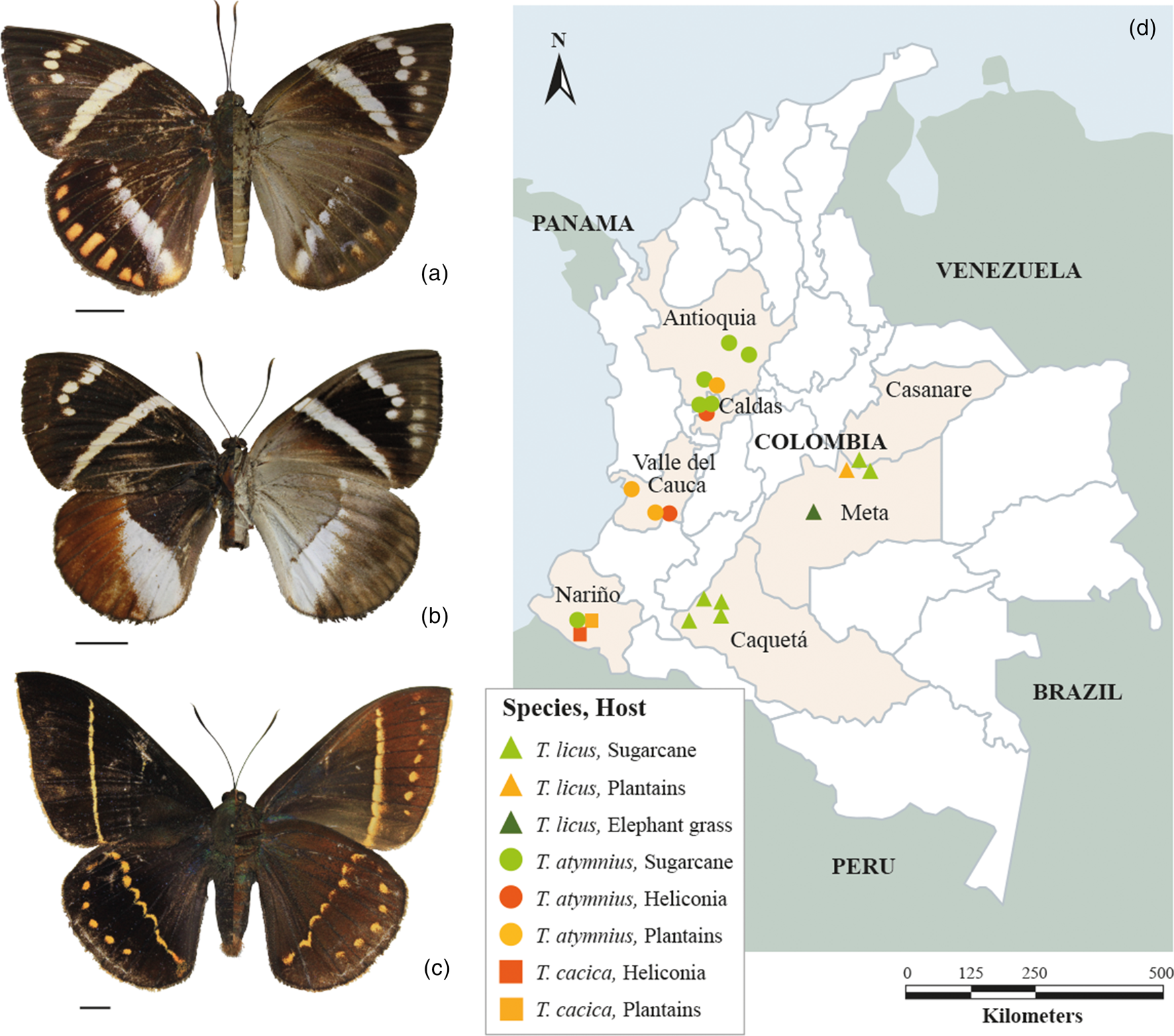
Figure 1. (a) Telchin licus magdalena (♀) dorsal and ventral view, Puerto López, Meta (Colombia). (b) Telchin atymnius humboldti (♀) dorsal and ventral view, Supía, Caldas (Colombia). (c) Telchin cacica angusta (♂) dorsal and ventral view, Ricaurte, Nariño (Colombia). Scale: 10 mm. (d) Distribution map of the species of the Telchin complex in Colombia: T. licus (triangle, ▴), T. atymnius (circle, ●) and T. cacica (square, ■). Hosts: sugarcane (green), elephant grass (dark green), heliconias (orange) and Musaceae (yellow).
The T. licus subspecies found corresponds to T. licus magdalena, originally described by Joicey and Talbot (Reference Joicey and Talbot1925). This form is distinguished by the presence of seven spots at the costal margin of the hindwing, present from the radial vein (R 1) to the anal vein (A 2). They are uniform in size and generally of yellow to orange colour. In the case of T. atymnius, the subspecies T. atymnius humboldti (Boisduval) and T. atymnius atymnius (Dalman) were found to have remarkably similar wing patterns. The difference is that the hindwings of T. a. humboldti are light brown, with a cream-coloured band that extends from the median vein (M 1) to the anal angle (A 3) (González and Salazar, Reference González and Salazar2003).
The species T. cacica angusta (Druce) was also identified. It is distinguished for having a large wingspan (up to 120 mm); the moth is black/dark brown, and the anterior wings present a straight and narrow band of yellow colour located transversally from the costal vein (R 3) to the anal margin. The posterior wing has a thin oblique postmedial band that extends from the costal margin to the yellow anal margin. The hindwing also has six small yellow spots at the costal margin, from the radial vein (R 1) to the ulnar vein (Cu2) (Miller and Sourakov, Reference Miller and Sourakov2009).
Three morphological groups of male genital structures were found. Only male genitalia was described as there are not many references describing female genitalia and the few available (Moraes and Duarte, Reference Moraes and Duarte2009) suggest little variation between characters among species. The difference between the three species begins at the level of the tenth abdominal sternum, represented by the uncus (fig. 2). From the dorsal view, the uncus is whole and triangular. Telchin licus is characterized by a marked inflection in the lateral margins of the uncus (fig. 2Ab), while T. atymnius has the limit between the tegumen and the uncus defined by a slight difference in sclerotization and the inflection in the lateral margins is not very marked (fig. 2Bb). In turn, from a lateral view, the uncus of T. cacica curves abruptly (fig. 2Ca), and from a dorsal view, the apex of the whole uncus can be observed to have a square shape (fig. 2Cb).

Figure 2. (A) Telchin licus, Puerto López, Meta (Colombia). (B) Telchin atymnius, San Roque, Antioquia (Colombia). (C) Telchin cacica, Ricaurte, Nariño (Colombia). (a) Lateral view of male genitalia. (b) Dorsal view of the tegumen and uncus. (c) Ventral view of the saccus (d) Aedeagus. T, Tegumen; U, Uncus; G, Gnathos; S, Subescafo; V, Valvae; Sa, Saccus; Vi, Vinculum; Pas, Projection of anterior saccus; By, Ejaculatory bulb.
Telchin licus has the posterior margin of the saccus (ventral view) in a ‘V’ shape (fig. 2Ac), while T. atymnius has it in a partially concave shape (fig. 2Bc), and T. cacica has the posterior margin of the saccus in the form of a ‘U’ from the ventral view (fig. 2Cc). The aedeagus is characterized by being curved and partially twisted in T. licus and T. atymnius, although T. licus presents a set of micro spicules on the right side of the distal portion of the aedeagus (fig. 2Ad), while T. atymnius lacks these spicules (Moraes and Duarte, Reference Moraes and Duarte2009). Telchin cacica presents the aedeagus deeply curved, with a complete contortion and a slightly widened ejaculatory bulb (fig. 2Cd) (Moraes and Duarte, Reference Moraes and Duarte2014). The striking difference between the genitalia of T. cacica and those of T. atymnius and T. licus, among other things (including molecular genetics, see below) is currently under study and will possibly lead to reinstating a couple of genera now synonymized under Telchin (Worthy, Zilli and González, in prep.).
Distribution of the Telchin complex in Colombia
The samples obtained in the departments of Caquetá, Casanare, and Meta correspond to T. licus and were found at altitudes between 174 and 400 metres above sea level (masl). On the other hand, in the departments of Antioquia, Caldas, Nariño, and Valle del Cauca the species found was T. atymnius, at altitudes between 12 and 1390 masl. Additionally, the subspecies T. cacica angusta (Druce, 1907) was found in the department of Nariño at altitudes between 1075 and 1338 masl (table 1, fig. 1d).
Genetic diversity index
In the entire data set (126 sequences), a total of 59 polymorphic sites and 33 haplotypes were identified, of which 18 correspond to individuals from Colombia, mostly unique haplotypes defined by the locality of the sample. Great genetic diversity was observed within each location in Colombia: the haplotype diversity (Hd) for T. licus varied between 0.591 and 0.857, being higher in the department of Caquetá (H d = 0.857); the haplotype diversity for T. atymnius varied between 0.6 and 0.77, with the highest occurring in Valle del Cauca (H d = 0.775). In contrast, nucleotide diversity (π) was relatively low for each population, ranging from 0.001 to 0.264, with the highest found in Antioquia (π = 0.264) (table 2).
Network of haplotypes and phylogenetic inference
The sequence analysis of the cytochrome COI gene, with a final length of 638 base pairs (bp), showed clear genetic variability in the populations of the Telchin species studied (GenBank accession numbers: MZ014321-MZ014357 and MZ068057-MZ068089 cox1). The average genetic distance between the sequences was 0.0556, with a range between 0 and 0.088. Mean genetic distances between Telchin species were between 0.0683 and 0.0801, with distances from the outgroups between 0.0789 and 0.1477 (table 3). Within T. licus the highest distance was between T. licus magdalena and T. licus licus (0.0251). In relation to T. atymnius, the highest distance was found between T. atymnius humboldti and T. atymnius from Nariño (0.0288). In the construction of the haplotype networks, a network with three large groups of distinct haplotypes was obtained, corresponding to each Telchin species studied (fig. 3a). Additionally, two disconnected networks were obtained with 19 haplotypes of T. licus (fig. 3b) and nine haplotypes of T. atymnius (fig. 3c).

Figure 3. (a) Median-joining network for Telchin species indicating the relationships between haplotypes defined by the variation of a region of the COI gene. Each circle represents a unique haplotype where the diameter is directly proportional to the number of specimens showing that haplotype; the parallel lines between each circle indicate the number of nucleotide changes within the sequence between the haplotypes. The colour associated with each haplotype indicates the geographical department to which the samples belong. Small black dots indicate medium vectors. (b) Haplotype network of T. licus. Brazil: CO, Coruripe; LI, Limeira; TS, Tangará da Serra; AR, Araporã; PV, Porto Velho. (c) Haplotype network of T. atymnius: Colombia: (I) Antioquia (MB: Montebello), Caldas (RS, Riosucio; SU, Supía), and Valle del Cauca (PA, Palmira; BV, Buenaventura; (II) Nariño (RC, Ricaurte); (III) Antioquia (SD, Santo Domingo). Costa Rica: GU, Guanacaste.
Table 3. Overall mean distance over COI sequence pairs among and within species groups, determined by using the Kimura 2-parameter model. Below the diagonal: the interspecific mean distances between species

Diagonal values (bold): the intraspecific mean distances within each species. Above the diagonal: standard error estimates between species.
The haplotype network for T. licus shows three main groups, where the first group corresponds to the subspecies T. l. magdalena – haplotypes representing the departments of Caquetá, Casanare, and Meta in Colombia. The second corresponds to T. l. laura and T. l. albomaculata, and the third group to T. l. licus – unique haplotypes for the São Paulo, Alagoas, Rondonia, Minas Gerais, and Mato Grosso regions in Brazil (fig. 3b).
The network formed by the individuals of T. atymnius shows a clear separation of the haplotypes of the Guanacaste province, corresponding to the subspecies T. a. futilis of Costa Rica. Additionally, within the subspecies defined as T. a. humboldti from Colombia, three groups of isolated haplotypes are shown (I–III, fig. 3). The first group is represented by the only haplotype shared within the Andean region of Colombia (Antioquia, Caldas, and Valle del Cauca), in addition to some haplotypes unique to Valle del Cauca (the Pacific coast). The second group (II) is made up of a single haplotype representing the entire Nariño region and the third group (III) consists of a haplotype, which only includes the population of Santo Domingo in the department of Antioquia (fig. 3c).
Neighbour-Joining and Bayesian inference trees showed similar topologies separating Telchin species. The samples were grouped into two main clusters supported by high bootstrap values and posterior probabilities (PP). The first clade grouped all the T. licus samples, internally defining the subspecies identified within this group (fig. 4), and the second clade grouped all the T. atymnius and T. cacica samples, showing differences between them as different species and at the same time showing an intra-species variation of some populations based on their geographical origin.

Figure 4. Bayesian inference generated under the nucleotide substitution model TIM2 + 1. The posterior probability (PP) values in which the grouped taxa have been associated are shown beside the branches. The abbreviations for each department in Colombia are: Antioquia (AN), Caldas (CA), Caquetá (CQ), Casanare (CS), Meta (MT), Nariño (NR), and Valle del Cauca (VA). GenBank accessions are indicated in bold.
Population structure
The results of the analysis of variance (AMOVA) for T. licus showed that the highest percentage of variation (91.46%) is due to the comparison between the subspecies, with a significant fixation index (Φst: 0.914) (p < 0.001). When the hierarchical level among groups was included, the highest percentage of variation was due to the difference between subspecies, while the variation among populations within subspecies was exceptionally low (3.1%) (table 4).
Table 4. Analysis of molecular variance (AMOVA) for T. licus

d.f., degrees of freedom.
Hierarchical structure tests were grouped by subspecies: T. l. magdalena, T. l. licus and T. l. albomaculata. Fixation indices (FST) ΦCT: divergence among subspecies; ΦSC: divergence among populations within subspecies; ΦST: divergence of the whole analysis.
Significance tests using 10,000 permutations.
p values: *p < 0.01; **p < 0.00001.
The analysis of variance for the T. atymnius population showed a high percentage of variation among populations with a significant fixation index (Φst: 0.718) (p < 0.0001). When the hierarchical level between groups was included, the highest percentage of variation was due to the difference among departments (46.97%) and the variation among municipalities within departments (41.07%) being significantly high (Φsc: 0.774) (p < 0.0001) and indicating an intraspecific differentiation in T. atymnius populations. This is indicative of a high genetic variability among municipalities within the same department (table 5).
Table 5. Analysis of molecular variance (AMOVA) for T. atymnius

d.f., degrees of freedom.
The genetic differentiation was examined for a total of 45 individuals from eight geographic populations (municipalities) as well as hierarchical geographic structure tests for five geographic groups: Antioquia, Caldas, Nariño, Valle del Cauca (Colombia) and Guanacaste (Costa Rica). Populations represented by one or two sequenced individuals were excluded. Fixation indices (FST) ΦCT: divergence between departments; ΦSC: divergence between municipalities within departments; ΦST: divergence of the whole analysis.
Significance tests using 10,000 permutations.
* p value: < 0.00001.
Discussion
Species distribution
In the Neotropics, some Castniidae are economically important agricultural pests that cause extensive damage to sugarcane, oil palm, pineapple, and orchid plantations (Moraes and Duarte, Reference Moraes and Duarte2014). Nonetheless, this family has received taxonomic treatment based mainly on colour patterns, making this a very diverse group throughout its distribution and in some cases, its identification has been very subjective. For this reason, efforts have been made to clarify the taxonomic status of some species in this group. Moraes and Duarte (Reference Moraes and Duarte2009) made a morphological comparison of the Telchin complex establishing that the external and internal morphological differences of T. licus and T. atymnius are sufficient to classify them as different species, despite the morphological similarities of the head, thorax, and wing pattern – similarities that could be inherited due to their sympatric distribution along the neotropical region (Miller, Reference Miller1986).
Currently, eleven species of the genus Telchin Hübner (Moraes and Duarte, Reference Moraes and Duarte2014) have been defined, of which eight are distributed in Colombia. González and Salazar (Reference González and Salazar2003) report that T. syphax (Fabricius) was located in the Andean region (Cundinamarca), T. hodeei (Oberthur) in the Caribbean (Bolivar) and southwestern Colombia (Valle del Cauca), while T. papilionaris (Houlbert) in central Colombia (Boyacá), with no indications of host plants. In addition, González et al. (Reference González, Andrade, Worthy and Hernández-Baz2017) mentioned that T. diva (Felder) was present in central Colombia (Boyacá) and T. evalthe (Fabricius) in eastern (Meta) and southern Colombia (Amazonas) feeding on Bromeliads and Heliconias (Parrales and Vargas, Reference Parrales-R and Vargas-Fonseca2017). However, the only species associated with economically important crops in the country seems to be T. licus, affecting sugarcane (Salazar et al., Reference Salazar, Rodríguez and Rodríguez2013), and T. atymnius, a common pest of plantains (Salt, Reference Salt1929; Vélez, Reference Vélez1997), but also reported as an occasional pest of sugarcane (Gallego, Reference Gallego1940; Vélez, Reference Vélez1997) and heliconias (Roth, Reference Roth1961). Based on what has been evidenced, species of the Telchin complex present a wide range of hosts (sugarcane, Musaceae, Heliconiaceae), without any particular host favouring its rapid dissemination and establishment (Salt, Reference Salt1929; González et al., Reference González, Andrade, Worthy and Hernández-Baz2017).
Observations made in this study suggest that the pest´s distribution is delimited by the geographical topology of the country. Records in entomological collections indicate the presence of species of this complex in other regions of the country in addition to those already mentioned. In this regard, Salazar et al. (Reference Salazar, Rodríguez and Rodríguez2013) reported that T. licus is restricted to the Amazon and Orinoco basins and the eastern slopes of the Eastern Cordillera, in the departments of Amazonas, Cundinamarca, Guaviare, Putumayo, and Vaupés (Salazar, Reference Salazar1999; González et al., Reference González, Andrade, Worthy and Hernández-Baz2017). Also, T. atymnius has been reported in the Andean, Caribbean and Pacific regions – in the departments of Boyacá, Cauca, Cesar, Chocó Cundinamarca, Magdalena, Risaralda, Santander, and Tolima – and in the Amazon basin (Salazar, Reference Salazar1999; González et al., Reference González, Andrade, Worthy and Hernández-Baz2017; Parrales and Vargas, Reference Parrales-R and Vargas-Fonseca2017). It seems that the geographic boundary defined by the Eastern Cordillera could be limiting the dispersion capacity of these species, which could also explain in some way the variability of the complex (Lamas, Reference Lamas1995) – allopatric speciation explained by the geographic isolation of the populations and/or sympatric speciation by the formation of races associated with the host plants (Saldamando, Reference Saldamando2010).
This diversification is reflected in the number of subspecies described, based on slight changes in their wing colouring pattern and distribution (Miller, Reference Miller and Heppner1995). Three subspecies of T. licus have been reported in Colombia: T. l. magdalena (Drury), T. l. albomaculata (Houlbert), T. l. chocoensis (Lamas, Reference Lamas1995), and three subspecies of T. atymnius: T. a. humboldti (Boisduval, Reference Boisduval1875), T. a. newmanni (Houlbert, 1917), and T. a. drucei (Schaus, 1911) (Lamas, Reference Lamas1995; Salazar, Reference Salazar1999; González et al., Reference González, Andrade, Worthy and Hernández-Baz2017). These slight morphological variations make their identification through conventional taxonomy more subjective and not so precise.
Genetic diversity
DNA barcoding is extremely useful to complement the identification of species where morphological data are not clarifying (Silva-Brandão et al., Reference Silva-Brandão, Almeida, Moraes and Cônsoli2013). In the current study, this method has allowed discrimination among Telchin species, even in the larval stage where there are no diagnostic characters. The sequences and data obtained here have been uploaded in the Barcode of Life Data System (BOLD) for future research, filling a gap as not many studies sample and report data on Telchin species. Under this approach, the analysis of genetic diversity provided information on the interconnectivity of the populations of the Telchin complex studied here, as well as on the degree of genetic variability within each species. Genetic distances on the giant sugarcane borer species are well supported according to the COI barcoding as high genetic variability within and between species was identified, without overlap between the levels of intra and inter-specific divergence (Wang et al., Reference Wang, Wang, Lin, Ali, Fu, Huang, Gao and Wang2018), consistent to previous studies on other sugarcane stemborer species from Noctuidae, Tortricidae, Crambidae and Pyralidae (Lee et al., Reference Lee, Anderson, Tran-Nguyen, Sallam, Le Ru, Conlong, Powell, Ward and Mitchell2019).
In relation to haplotype diversity, T. licus shows greater diversity in some regions, possibly due to variation in the number of municipalities sampled, with the department of Caquetá having the highest value and representing the haplotype diversity of three municipalities (Albania, Doncello, and Florencia). There were no common haplotypes in the departments of Caquetá and Meta, suggesting low gene flow between these two regions of the country (Excoffier et al., Reference Excoffier, Smouse and Quattro1992). In the case of T. atymnius, genetic diversity was greater for the area of Antioquia and the haplotype diversity reflected greater variability in the department of Valle del Cauca where it was higher. In turn, this species presented only one haplotype shared between the department of Caldas and some localities of the departments of Antioquia and Valle del Cauca. The rest of the populations were distinguished by unique haplotypes specific to each department, indicating little genetic exchange between populations of different regions (Boyer et al., Reference Boyer, Baker and Giribet2007). According to González (Reference González1999), a large part of the Castniinae subfamily is endemic to particular areas and has reduced geographical distribution, which would explain the lack of shared haplotypes among localities and the large intra-specific genetic divergences within the populations of the complex.
The phylogenetic analysis and haplotype networks revealed a high genetic differentiation among the subspecies of T. licus. The subspecies T. licus magdalena identified in Colombia is different at the molecular level from the subspecies of T. licus already defined in Brazil (Silva-Brandão et al., Reference Silva-Brandão, Almeida, Moraes and Cônsoli2013). Likewise, the significant values of the fixation index (ΦST) of the variance analysis among subspecies indicated a high genetic structure among populations, showing the haplotype differences that tend to be divergent from one geographic region to another (Hincapié et al., Reference Hincapié, Gil, Pico, Gusmao, Rondón, Vargas and Castillo2009). Thus, the study confirms the differentiation of four subspecies of T. licus, being consistent with previously reported taxonomic differences (Joicey and Talbot, Reference Joicey and Talbot1925; Lamas, Reference Lamas1995).
We found significant genetic diversity both within and among populations of T. atymnius. The phylogeny study indicated that the subspecies described as T. atymnius humboldti and T. atymnius atymnius in Colombia were different from T. atymnius futilis individuals registered in the Bold System from Costa Rica (Ratnasingham and Hebert, Reference Ratnasingham and Hebert2007), confirming differences at a molecular level among subspecies described at a morphological level (Lamas, Reference Lamas1995; González and Salazar, Reference González and Salazar2003). However, an intraspecific difference was observed in populations identified as T. a. humboldti concerning geographical distribution. The haplotype network and the Bayesian inference analysis indicated that the specimens from the department of Nariño show high variability in relation to populations present in Antioquia, Caldas, and Valle del Cauca. Additionally, within the population of Antioquia, two very divergent haplotypes were found within the network, coming from the municipalities of Montebello and Santo Domingo. This implied a significant fixation index (ΦSC) and a high percentage of variability among municipalities within departments in the analysis of variance. These results may indicate the presence of a different subspecies of T. atymnius than previously reported, as highly structured populations and marked genetic divergences may indicate the presence of cryptic species (Boyer et al., Reference Boyer, Baker and Giribet2007).
On the other hand, the subspecies identified as T. cacica angusta in the Bayesian inference analysis was differentiated from all individuals evaluated, forming a monophyletic group with the species T. cacica procera registered in GenBank – this being the closest species within the analysis. Previously known in the genus Amauta, this species has been reported in the departments of Boyacá, Nariño, and Risaralda in Colombia (González and Salazar, Reference González and Salazar2003; González et al., Reference González, Andrade, Worthy and Hernández-Baz2017). However, no records were found of its hosts in the country. Information on the bioecology of the species and its possible subspecies is scarce. So far, their larvae have been recorded boring the rhizomes of heliconias in Costa Rica (Miller and Sourakov, Reference Miller and Sourakov2009) and plantations of Musa sp. in Ecuador, causing some impact on these crops (Gonzalez et al., Reference González, Andrade, Worthy and Hernández-Baz2017). Recently, Moraes and Duarte (Reference Moraes and Duarte2014) included it within the genus Telchin, based on a morphological study of the family Castniidae. In our case, the larvae were recovered from plantain plants, constituting a new host record for Colombia. When the larvae were taken to the laboratory for observation, they finished their development feeding on sugarcane, which may point to the possibility of T. cacica having a wide range of hosts, which could eventually include crops of agricultural importance such as sugarcane.
Genetic variability in the Telchin complex could explain why both Telchin species, previously reported associated with tropical rainforest vegetation (Miller, Reference Miller1986; González and Stüning, Reference González and Stüning2007), are now found in commercial sugarcane crops in Colombia (Tarazona, Reference Tarazona2011; Vargas et al., Reference Vargas, Lastra, Ramírez and Solís2018). Despite having a reduced geographical distribution, some populations very probably have migrated to crops of economic interest due to the modification or destruction of their habitat, thus expanding their distribution over time (González, Reference González1999; Miller, Reference Miller1986; Lamas, Reference Lamas1995) and increasing the probability of pest outbreaks in other cropping systems (Goebel and Sallam, Reference Goebel and Sallam2011).
Castniidae associated with sugarcane in Colombia
In this study, taxonomic methods and COI barcoding confirmed that there are two Telchin species attacking sugarcane in different areas of Colombia, in addition to other alternative crops (i.e., Heliconiaceae, Musaceae) which increases the species’ distribution range. At the same time, the presence of new species like T. cacica have been reported in plantain crops, representing a risk for nearby sugarcane crops, given the pest's wide host range and its possible dispersion helped by the transport of planting material. In addition, the molecular study shows that the variation of the complex allowed differentiating the populations at an intraspecific level, genetically separating the subspecies described. The haplotype diversity of T. atymnius populations is closely related to their geographical distribution and this is crucial to delimit the taxonomy of the known and unknown subspecies. Understanding the taxonomy, genetic structure, host range, and distribution of this complex in Colombia will allow a deeper understanding of the bioecology of these insects, which may result in the proper identification of their natural enemies and enable management strategies. Therefore, sampling from other locations within Colombia and in neighbouring countries would help broaden the knowledge of the proposed subspecies of T. licus and T. atymnius and understand the geographic and environmental conditions that determine their distribution.
Acknowledgements
We thank Álvaro Urresti for his great help and commitment in the collection of samples in the field. We also thank Juan Gonzalo López of Agrosavia, Jennifer Román of Fedepanela, and Pedro Antonio Luna of ICA, for their technical assistance in the field. Our appreciation goes to Vanessa Muñoz for her advice on bioinformatics and molecular analysis. Finally, we would like to thank the Entomology Team and Hugo Jaimes from Cenicaña for their support and collaboration, and Alexandra Walter for valuable editorial assistance.
Conflict of interest
The authors declare none.