Introduction
In 1779, Peter Woulfe predicted the existence of a new element based upon his chemical analysis of the mineral wolframite. In 1781, Carl Wilhelm Scheele prepared tungstic acid for the first time. The starting material was a mineral at that time referred to as tungsten (from tung sten = ‘heavy stone’ in Swedish), but which we today call scheelite. Two years later, José and Fausto Elhuyar prepared the same acid from the mineral wolframite and subsequently succeeded in reducing the acid using charcoal to obtain the new metallic element that they called wolfram. Today, the element goes by this name in German and some other European languages, while in English and many other languages, it goes by the name tungsten.
In the Earth's crust, tungsten (W) combines readily with oxygen to form tungstates, such as scheelite (CaWO4), stolzite (PbWO4) and the wolframite-series minerals, ferberite (FeWO4) and hübnerite (MnWO4), or with sulfur to form sulfides, such as tungstenite (WS2). The average abundance of tungsten in the Earth's crust is estimated to be 1.25 ppm (Christy, Reference Christy2015). Concentrations of the element are generally associated with granites or medium- to high-grade metamorphic rocks and commonly occur in skarn deposits. Native tungsten is extremely rare in terrestrial rocks because of its strong tendency to form compounds with oxygen or sulfur, especially in the types of deposits in which it is generally concentrated. Until the report of its occurrence in gold placers in the Bol'shaya Pol'ya river valley, Prepolar Urals, Russia, by Novgorodova et al. (Reference Novgorodova, Nedashkovskaya, Rassdazov, Trubkin, Semenov and Koshelev1995), native tungsten was not believed to exist in Nature. Since the report by Novgorodova et al. (Reference Novgorodova, Nedashkovskaya, Rassdazov, Trubkin, Semenov and Koshelev1995), native tungsten has been confirmed from a number of localities in Russia, as well as in petroliferous rocks of Ukraine (Lukin, Reference Lukin2009), Proterozoic conglomerates of the Volyn, Ukraine (Kvasnytsya et al., Reference Kvasnytsya, Pavliuk, Kvasnytsya, Vysotskyi and Gurnenko2014), as inclusions in diamonds in the kimberlites of Shandong Province, China (Gorshkov et al., Reference Gorshkov, Titkov, Bao, Ryabchikov and Magazina2006), in the Luobusha ophiolite, Tibet (Bai et al., Reference Bai, Yang, Fang, Yan and Zhang2001) and in gold-bearing placers in South Africa (Roger Dixon, pers. comm.); it has also been found in the Krymka meteorite and the Luna 16, 20, 24 and Apollo 16 sites on the Moon (Mokhov et al., Reference Mokhov, Kartashov and Bogatikov2007, Reference Mokhov, Kartashov, Gornostaeva and Bogatikov2014; Kartashov et al., Reference Kartashov, Mokhov, Gornostaeva, Bogatikov and Ashikhmina2010).
Herein, we report the formal description of tungsten as a mineral species, based on material from its first reported occurrence in the Bol'shaya Pol'ya river valley (holotype locality), as well as on material from the Mt Neroyka rock-crystal field, Ust–Puiva, Tyumenskaya Oblast', Russia (cotype locality). The mineral and its name have been approved by the Commission on New Minerals, Nomenclature and Classification (CNMNC) of the International Mineralogical Association (IMA2011-004, Mills et al., Reference Mills, Kartashov, Kampf, Rumsey, Ma, Spratt, Rossman and Novgorodova2011a). Three cotype specimens are housed in the collections of Mineral Sciences Department, Natural History Museum of Los Angeles County (900 Exposition Boulevard, Los Angeles, California 90007, USA), catalogue number 63271 (Mt Neroyka) and 63272 (Bol'shaya Pol'ya river valley) and the Mineralogy Department, Natural History Museum, Cromwell Road, London SW7 5BD, UK, catalogue number BM 2010,122 (Dodo mine Mt Neroyka); there is no holotype as no one specimen was used for all analyses. Although the name ‘wolfram’ was not included in the new mineral proposal that was approved by the CNMNC, it can be assumed that this name has been approved for use in languages in which the element is so named, such as in German or Russian.
Occurrence
As noted above, the occurrence of tungsten in the Bol'shaya Pol'ya river valley, Prepolar Urals, Russia, was first described by Novgorodova et al. (Reference Novgorodova, Nedashkovskaya, Rassdazov, Trubkin, Semenov and Koshelev1995). Here, tungsten is very rare and occurs with inclusions of yttriaite-(Y) (Mills et al., Reference Mills, Kartashov, Ma, Rossman, Novgorodova, Kampf and Raudsepp2011b), quartz, ‘phengite’ and siderite (Fig. 1). Native tungsten was first discovered during a geological survey for gold placers in the 1980s by one of the authors (MIN) and colleagues from the Fersman Mineralogical Museum. The river valley is characterised by a 30 m thick sequence of friable sediments composed of interlayered sandstones, alevrites and pebbly sands of Pleistocene–Holocene age. These sediments are typical in the Ural erosion–structural depressions. Modern alluvial deposits in the Bol'shaya Pol'ya river valley were formed by erosion of these friable strata and also by erosion of basement gneisses, amphibolites, quartzites and conglomerates of Upper Proterozoic–Cambrian age, schists, sandstones and gravellites of Ordovician age, gabbros of Silurian age and small bodies of serpentinites. Gold placers, known in the region since the beginning of the 20th Century, derive their source from gold–sulfide–quartz veins within diabases and diabase porphyries.

Fig. 1. Back-scatter electron (BSE) photomicrograph of a polished tungsten crystal with yttriaite-(Y) inclusions from the Bol'shaya Pol'ya river valley. Specimen number 63272, Natural History Museum of Los Angeles County.
The new mineral also occurs in quartz vein #91 in the Mt Neroyka rock-crystal field, Ust–Puiva, Tyumenskaya Oblast', Russia (used for reflectance, hardness and further chemical data), where the grains are free of any inclusions. Mt Neroyka is located ~50km NW of the Bol'shaya Pol'ya river and vein #91 is a small prospecting trench located several kilometres S of the Dodo mine. Native tungsten was found in heavy concentrates obtained by the crushing of 5 tons of ultra-pure quartz (with low contents of Al and alkalis) for pure quartz glass production. In the concentrate, it was found in association with fluorapatite, ilmenite, anatase, galena, chalcopyrite and moissanite at a concentration of several hundred mg/ton of rock crystal.
Physical properties
At the Bol'shaya Pol'ya river valley locality, tungsten crystals form in a variety of morphologies: (1) porous polycrystalline crystals up to 5 mm across with a cragged surface; (2) cubo–octahedra, up to 2 mm across, exhibiting the forms {100} and {111}, twinned on {111} forming dendritic intergrown clusters; and (3) irregular globular grains up to 5 mm across. All tungsten grains from the Bol'shaya Pol'ya river valley have inclusions of yttriaite-(Y) [typically 10–12 per grain]. At Mt Neroyka, tungsten forms as polycrystalline masses with irregular outlines between 0.1 and 0.8 mm across.
Tungsten is silver white to steel grey in colour, with metallic lustre and a grey streak. No cleavage was observed. The calculated density based on the composition of the mineral from the Bol'shaya Pol'ya river valley is 19.226 g/cm3. In plane polarised light, tungsten is white with a pale yellow tint and optically isotropic. Reflectance values measured in air using WTiC as the standard are given in Table 1.
Table 1. Reflectance values (R, %) of tungsten from Mt. Neroyka.

Data for wavelengths recommended by the IMA Commission on ore microscopy (COM) are marked in boldtype.
A Vickers hardness (VHN25) of 571.45 kg/mm2 (range: 559–605) was obtained from five measurements. Metallurgical literature pertaining to the hardness of tungsten indicates that its Mohs hardness is thought to equate to ~7.5 (Samsonov, Reference Samsonov1968) and the more regularly used Vickers microhardness tests generally give values of 350 kg/mm2 (Lassner et al., Reference Lassner and Schubert1999). Equating these two values using the formula of Young and Millman (Reference Young and Millman1964), as is common in mineralogical literature, leads to an obvious mismatch whereby 350 kg/mm2 is ~4.1 on the Mohs scale. This indicates that this conversion method is inappropriate for native tungsten. Due to the nature of the material, it was not possible to undertake a simple scratch test upon the single grain and determine the Mohs hardness by reference with other minerals; therefore, it is set to 7.5 as reported in the metallurgical literature.
The measured Vickers value (571.5 kg/mm2) is still clearly somewhat different to that reported in the metallurgical literature. It is noted by a number of authors that many factors can lead to an increase in this value, all of which are present in the current example. For instance, it is widely reported that the use of smaller load weights (<100 g) gives an increase in Vickers hardness, as does the process of polishing, which acts to work harden the material studied (Craig and Vaughan, Reference Craig and Vaughan1981). The polycrystalline nature of the grain studied, with its small grain size, also leads to an increase in the hardness value according to the Hall–Petch relationship. Values as high as 1200 kg/mm2 have been recorded on materials with a sub-μm grain size, while values of ~500 kg/mm2 are common on swaged polycrystalline tungsten metal objects and can be as high as 650 kg/mm2 (macrohardness) after deformation (Lassner, Reference Lassner and Schubert1999). These figures clearly indicate that the value obtained is well within limits that might be expected for a worked, small and minutely polycrystalline tungsten specimen.
Chemical composition
Six chemical analyses of tungsten from the Bol'shaya Pol'ya river valley were undertaken using a JEOL 8200 electron microprobe in wavelength–dispersive (WDS) mode (25 kV, 300 nA and focused beam) at the Division of Geological and Planetary Sciences, California Institute of Technology. Quantitative elemental microanalyses were processed with the CITZAF correction procedure. The standards used were W metal, Mo metal, Mn metal and Fe metal. The results (mean, range, standard deviation) were W 99.27, 98.39–99.96, 0.59; Mo 0.06, 0.05–0.08, 0.01; Mn 0.04, 0.03–0.04, 0.01; Fe 0.01, 0.00–0.02, 0.01; total 99.38 wt.%. Novgorodova et al. (Reference Novgorodova, Nedashkovskaya, Rassdazov, Trubkin, Semenov and Koshelev1995) reported W analyses between 99.93 and 101.19 wt.%, with Ca, Al, Fe, Mn, Mg, Ti between 0.01 and 0.06, and up to 0.11 wt.% Mo.
Nineteen chemical analyses of material from Mt Neroyka were done using a Cameca SX100 electron microprobe at the Natural History Museum, London, UK, operating in WDS mode (15 kV, 20 nA and 20 μm beam diameter). Peak overlap corrections were taken into account within the matrix correction. Mo, Cr and Fe were all sought for, but were found to be below detection limits and no other elements were detected using energy dispersive spectroscopy (EDS). Tungsten metal was used as the standard. The result (mean, range, standard deviation) was W 100.04, 98.79–102.34, 0.92; total 100.04 wt.%.
X-ray crystallography
Powder X-ray diffraction data for tungsten from the Bol'shaya Pol'ya river valley were obtained on a Rigaku R-Axis Rapid II curved imaging plate microdiffractometer utilising monochromatised MoKα radiation. Observed d values and intensities were derived by profile fitting using JADE 9.1 software. The observed powder data are presented in Table 2. The unit-cell parameters (space group Im $\bar{3}$m) refined from the powder data using JADE 9.1 with whole-pattern fitting are a = 3.1648(4) Å, V = 31.69(4) Å3 and Z = 2.
Table 2. X-ray powder diffraction data (d in Å) for tungsten from the Bol'shaya Pol'ya river valley.
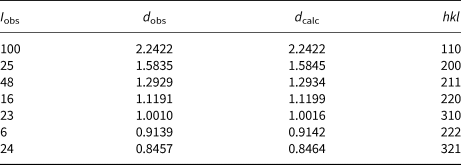
All tungsten grains examined were found to be polycrystalline, so single-crystal X-ray studies could not be conducted. Electron back-scatter diffraction (EBSD) analyses were performed on the vibro-polished section using methods described in Ma and Rossman (Reference Ma and Rossman2008). An HKL EBSD system on a ZEISS 1550VP scanning electron microscope (SEM) was used for these measurements and operated at 20 kV and 6 nA in a focused beam configuration with a 70° tilted stage and variable pressure (20 Pa) mode, which allows the study of uncoated specimens. The structure was constrained by comparing the observed EBSD pattern with synthetic tungsten (Fig. 2). Native tungsten is I-centred cubic (e.g. Deshpande and Pawar, Reference Deshpande and Pawar1962) and is isostructural with native Cr. There is one W atom located on the origin.

Fig. 2. EBSD pattern for tungsten from the Bol'shaya Pol'ya river valley (upper) and the pattern indexed with the synthetic structure (lower).
Additional tungsten finds
The Tabornoe gold deposit located in the Olekma area of the Sakha Republic, Russia occurs at the western boundary of Aldan crystalline shield. Gold ores are found in mica–quartz–K-feldspar metasomatites of Mezozoic age, superimposed on lower-Proterozoic sandstones and middle-Jurassic syenite–porphyres. Content of ore minerals (pyrite, marcasite, arsenopyrite, chalcopyrite, sphalerite, galena, molybdenite and acanthite) is < 1% (Dvurechenskaya and Kryazhev, Reference Dvurechenskaya and Kryazhev2005). The ore metasomatites are significantly oxidsed and weathered up to red hematitised clays. Some gold placers were formed during rewashing of these rocks (Glushkova and Nikiforova, Reference Glushkova and Nikiforova2014).
The deposit is located within a graben-like structure. Two prospecting drill-holes were made on a fault in the northern part of the structure. A high Ag geochemical anomaly was detected in both holes at a depth of 80–90 m. The drill-cores at this depth were composed of red hematitised montmorillonite–hydromica clay material. Heavy concentrates from this red clay contain abundant copper–silver micronuggets up to 3 mm, where crystals of native copper were cemented by native silver or vice versa. This copper–silver matrix contains abundant inclusions and well-formed crystals of a grey (in reflected light) W mineral with elevated hardness (Fig. 3).

Fig. 3. Copper–silver nuggets with native tungsten inclusions from the Tabornoe gold deposit, Olekma, Sakha Republic, Russia. (a) Reflected-light micrograph showing copper matrix and native tungsten (light-grey with violet tinge), grain size ~400 μm across; and (b) SEM–BSE photomicrograph. Photos and specimens: Pavel Kartashov.
Initially we thought that the mineral was tungsten carbide, WC, known as the mineral qusongite (Fang et al., Reference Fang, Bai, Yang, Xu, Li, Shi, Xiong and Rong2009). Indeed, its colour in reflected light (light-grey with violet tinge) was very different from the white colour of metallic tungsten from the Prepolar Urals. However, EBSD performed on these crystals in Italy by Anatoly Kasatkin (pers. comm.) confirms that the mineral is metallic tungsten. The change in the colour of the rotation tints is explained by the influence of the matrix on the colour of the mineral as described by Ramdohr (Reference Ramdohr1969). Native tungsten both from Bol'shaya Pol'ya river and Mt Neroyka. were pure and in direct contact with only epoxy wax and this allows us to observe its true colour in reflected light.
Technogenic tungsten
Two W-rich minerals exist, metallic tungsten and qusongite. The later contains 6 mas.% C and can be easily confused with metallic tungsten especially in cases of micrometre-size particles in microprobe sections coated by carbon. Tungsten carbide is significantly more widespread as a component of so called ‘hard alloys’ (also known as ‘pobedite’). Such ‘hard alloys’ have wide uses in metalworking and drilling from construction to mining. These ‘hard alloys’ are composite materials, produced from fine powders of synthetic WC and metallic bonding materials – usually cobalt (due to similarity of their thermal expansion coefficients). These ‘hard alloys’ have been used in large quantities since the 1920s, while Co-bearing WC has been used since the 1930s (Trent and Wright, Reference Trent and Wright2000).
Many ‘mineral samples’, especially those obtained by core drilling, are contaminated by tungsten carbide. The presence of Co in the EDS spectra of ‘tungsten’ microparticles indicates the presence of synthetic WC from ‘hard alloys’, rather than metallic tungsten. The difference between the two can be seen in Lukin (Reference Lukin2009), their figure 2c, d and e and figure 3c and d show Co in the spectra, while the others show mostly minor Ti. These samples were obtained from drill cores from boreholes obviously drilled with ‘hard alloy’ bits, so the native tungsten was confused here by WC from drilling.
Metallic tungsten has fewer applications than WC. The high melting point of tungsten, the highest of any metal, is the reason it is used for the spiral filaments in incandescent lamps – its principal use. Fragments of such spirals can be found in any placer mines that use illumination by incandescent lights. Due to its high specific gravity, metallic tungsten from lamps is accumulated in heavy concentrates and is commonly found in gold and platinum concentrates (Fig. 4). Here, we show metallic tungsten wire associated with Ru–Os–Ir alloys from a gold placer in the Uchalinsky District, Bashkortostan, Russia. Tamana (Reference Tamana1994) similarly reported the occurrence of tungsten lamp filaments in Pt placers from the USA.

Fig. 4. Tungsten wires of different diameters (technogenic) from two different lamp spirals associated with Ru–Os–Ir alloys from a gold placer in the Uchalinsky District, Bashkortostan, Russia. Field of view ~1 cm. Photo and specimen: Pavel Kartashov.
The high-refractory nature of metallic tungsten has led to its use in the nozzles, jets and heads of rockets (cosmic and military). Fragments and particles from them constantly precipitate from the atmosphere over land and the ocean surfaces. These tungsten particles can be recognised by the presence of Ce, Y and other REE oxides and by a linear eutectic texture, which is due to exsolution during their high-temperature manufacture (generally over 2200°C). Such material has been described since the 1990s (e.g. Itoh and Ishiwata, Reference Itoh and Ishiwata1996), so it is important to benchmark finds of native tungsten (and likewise qusongite) with technological developments and the location of manufacture and use. Our finds of native tungsten predate these technological developments.
Formation of tungsten
The native tungsten from the Bol'shaya Pol'ya river contains inclusions of both siderite and ‘phengite’. The thermal stability of both ‘phengite’ and siderite gives us a formation temperature below ~400°C (e.g. Pan et al., Reference Pan, Zhu, Banerjee, Gill and Williams2000) for this occurrence. At higher temperatures, siderite decarbonises, while ‘phengite’ breaks down with formation of muscovite + clinochlore. This temperature of formation is much lower than that for Lunar tungsten, which is formed by fractional condensation from high-temperature (2500–5000°C) gas–plasma clouds during impact events (Kartashov et al., Reference Kartashov, Mokhov, Gornostaeva, Bogatikov and Ashikhmina2010). Mokhov et al. (Reference Mokhov, Kartashov, Gornostaeva and Bogatikov2014) also reported native tungsten in association with the Hg-bearing complex sulfide (Cu10Ag2Hg)13S14 from the Lunar regolith of Mare Fecunditatis (Fig. 5), indicating that tungsten can also form from exhalative gasses, presumably at temperatures below ~1000°C. The presence of tungsten inclusions in diamond (Gorshkov et al., Reference Gorshkov, Titkov, Bao, Ryabchikov and Magazina2006) also points to high-pressure high-temperature formation deep within the Earth's crust. Clearly, there are a variety of possible natural processes that can produce native tungsten.

Fig. 5. SEM–BSE images of native tungsten (W) on particles of Lunar regolith from Mare Fecunditatis. (a) Exhalative origin, W in association with (Cu10Ag2Hg)13S14 (CuAgHgS) (after Mokhov et al., Reference Mokhov, Kartashov, Gornostaeva and Bogatikov2014); (b) (b,c) impact origin, W surrounded by glass agglomerations, full scale in (b) and close up (c). Photos: Pavel Kartashov.
Acknowledgements
This paper is dedicated to Frank Reith who's spirit and endeavour to discover information about metals has inspired us to explore the world and discover more about the origin of precious metals and deposits. The paper benefited from constructive reviews by Daniel Atencio and an anonymous reviewer. We thank Elena Anikina for providing samples from the Tabornoe deposit and Anatoly Kasatkin for confirmation of native tungsten from Tabornoe by EBSD. A portion of this study was funded by the John Jago Trelawney Endowment to the Mineral Sciences Department of the Natural History Museum of Los Angeles County.