1 Introduction
Although Ghana and Côte d'Ivoire produce over 60% of the world's Theobroma cacao (cocoa) beans, productions in these countries are constrained by declining soil fertility which has resulted in poor cocoa yield (Wessel and Quist-Wessel, Reference Wessel and Quist-Wessel2015; FAO, 2017). Declining soil fertility under cocoa farms has been cited as a driver of deforestation in Ghana as farmers tend to abandon old cocoa farms and establish new ones in existing forests (FAO, 2017; Benefoh, Reference Benefoh2018). The regional frontiers of cocoa production in Ghana is associated with forest clearance by farmers in search of rich soils for the establishment of cocoa plantations (Knudsen and Agergaard, Reference Knudsen and Agergaard2016; Benefoh, Reference Benefoh2018). Continuous production without proper soil fertility management coupled with intensification (i.e. replacing the shade trees with cocoa trees to increase cocoa yield) leads to nutrient depletion and poor soil quality. Attempts to resolve this problem with synthetic chemical fertilizers have not been successful due to the socio-economic context of cocoa farmers (Gockowski et al., Reference Gockowski, Afari-Sefa, Sarpong, Osei-Asare and Agyeman2013; Hütz-Adams et al., Reference Hütz-Adams, Huber, Knoke, Morazán and Mürlebach2016). Cocoa is predominantly produced by poor rural smallholder farmers who are unable to access or afford synthetic fertilizers (Hütz-Adams et al., Reference Hütz-Adams, Huber, Knoke, Morazán and Mürlebach2016; Benefoh, Reference Benefoh2018).
Faced with the challenge of ensuring soil fertility and the lack of robust data on soil physico-chemical properties of cocoa farms under organic and conventional management in West Africa, there is an urgent need to provide such data to guide decision making and policy formulations. Our study which compared two cocoa farming systems in Ghana (organic vs conventional) would contribute in bridging the research gap. We evaluated the influence of organic management on soil physico-chemical properties of cocoa agroforestry systems across the entire crop production stages. We examined cocoa pod, banana (Musa sapientum L. f. thomsonii King ex Baker) and plantain (Musa paradisiaca L.) production in the two farm types as an assessment of productivity. Finally, we explored the relationship between soil nutrient stocks, stand characteristics and productivity. We posit that organic systems would manifest greater soil nutrient stocks as well as cocoa pod, banana and plantain yields compared to conventional systems and that stand characteristics will influence soil parameters and productivity.
2 Methods
2.1 Study area
Suhum Municipality (Fig. 1; located at N 6o 5′ and W 0o 27′ and about 400 km2) has an agrarian economy based on rain-fed agriculture with cocoa being the main cash crop; other cultivated crops include banana, plantain and cocoyam (Cocos nucifera L.). Located in the semi-deciduous forest zone, Suhum has a mean annual temperature and precipitation range of 24–29°C and 1270–1651 mm, respectively (Ghana Statistical Service, 2014). The relative humidity is low during the dry season (48–52%) and high during the wet or rainy season (87–91%). The soils are classified as forest ochrosols (Nsaba-Swedru series), formed from granite parent material and are well-drained, deeply weathered, moderate fine granular in structure and friable in consistency (Cartographic Staff of Soil Research Institute, 1990; Adjei-Gyapong and Asiamah, Reference Adjei-Gyapong and Asiamah2002).

Fig. 1. Map of the study area (modified from Asigbaase et al., Reference Asigbaase, Sjogersten, Lomax and Dawoe2019).
Cocoa plantations in the district were established as agroforestry systems (cocoa cultivated under shade trees/shrubs) and are managed with or without synthetic chemical inputs such as fertilizer, pesticides and herbicides. Whereas the conventional systems use synthetic chemicals to improve soil fertility, control pest and suppress weeds, the organically certified cocoa systems use an integration of diverse shade tree species, neem (Azadirachta indica A. Juss.) extracts and organic fertilizer to maintain soil fertility, control pests and suppress weeds (Appendix Table 1). Specifically, the conventional systems applied ‘Asaasewura’, a specially formulated cocoa fertilizer (0-22-18 + 9Ca + 7S + 6MgO) and ‘Nitrobor’ (Nitrogen + Boron) at the rate of 375 kg ha−1 and 125 kg ha−1, respectively (Ghana Cocoa Board, 2018; Asigbaase et al., Reference Asigbaase, Sjogersten, Lomax and Dawoe2019). The organic farmers were encouraged to maintain shade trees on their farms to exploit their ecological benefits and to use organic fertilizer (e.g. Elite organic fertilizer, NPK 3:4:4 + 9Ca + 1Mg + 0.04B + 0.08Zn + 11organic matter) at a rate of 988 kg ha−1 as an alternative to synthetic chemical input (Djokoto et al., Reference Djokoto, Owusu and Awunyo-Vitor2016; Ghana Cocoa Board, 2018; Asigbaase et al., Reference Asigbaase, Sjogersten, Lomax and Dawoe2019). The cultivation practices and biophysical characteristics of the two systems are presented in detail in Asigbaase et al. (Reference Asigbaase, Sjogersten, Lomax and Dawoe2019) and Appendix Tables 1 and 2. The organic farms obtained their certification from Control Union, an international certification body that is present and active in over 70 countries.
2.2 Selection of cocoa farms
Suhum Municipality was purposely selected because organic cocoa certification in Ghana was pioneered in the Municipality, thus it has the oldest certified organic cocoa farms. A list of organic and conventional cocoa farming communities within the Municipality was obtained from Ghana Cocoa Board (COCOBOD), the regulator of the cocoa sector in Ghana and seven cocoa communities were randomly selected (Fig. 1). Separate lists of organic and conventional cocoa farmers in the selected communities were obtained from the local offices of the regulator and 24 organic and 24 conventional cocoa farms (i.e. eight farms per farm type per cocoa temporal phase) were randomly selected for the study. The selected organic and conventional farms were categorized into three cocoa temporal phases, young (≤15 years), mature (16 to 30 years) and old (≥31 years). One plot (25 m × 25 m) was established per farm; mean farm size in organic systems was 1.9 ha and that of conventional systems was 1.2 ha. The location of each plot was determined by dividing each selected farm into six equal parts and randomly selecting one. The research was conducted on private land and farmers gave oral consent; there was no need for additional permission.
2.3 Soil sampling
Soil samples were collected from two depths (0–15 cm and 15–30 cm). In each plot and for each depth, five soil samples were collected using an Eijkelkamp soil auger with a 5 cm blade diameter; the soil auger was marked at the respective soil depths and manually driven into the soil to collect samples. The five samples for each layer in each plot were pooled, thoroughly mixed for each soil depth and subsampled for chemical analysis. Two soil samples per plot per depth were collected with 139 cm3 bulk density cylinders for soil bulk density determination. The samples were collected from the soil wall after digging. Soil subsamples were oven-dried (105°C for 48 h), sieved (2 mm mesh) and milled (agate ball mill at 290 rpm for 15 min) prior to chemical analysis. The collection of soil samples was conducted on the same day.
2.4 Crop yield and stand characteristics
We used data collected by Asigbaase et al. (Reference Asigbaase, Sjogersten, Lomax and Dawoe2019) to estimate the stand characteristics (cocoa and shade tree species densities and shade tree basal area, richness and Shannon diversity) of the two farm types. Surface litter was collected by randomly throwing a 50 cm × 50 cm wooden quadrat five times within the plots (e.g. Soto-Pinto and Aguirre-Dávila, Reference Soto-Pinto and Aguirre-Dávila2015). The cocoa yield was estimated in terms of cocoa pod production by counting the number of small (<5 cm long), medium (5–10 cm) and large (>10 cm) cocoa pods in August and January which coincides with the major and minor cocoa seasons, respectively. The cocoa pod census was conducted in 25 m × 25 m plots established in the selected organic and conventional farms. The annual amount of banana (M. sapientum) and plantain (M. paradisiaca) production was estimated as the sum of the weight of each harvested bunch per farm over a 12-month period. In Ghana, including Suhum, cocoa trees are generally planted at 3 m × 3 m (resulting in a planting density of approximately 1100 trees ha−1) with the majority of the farms having the recommended 12–18 shade trees ha−1 (Asare and Anders, Reference Asare and Anders2016). Thus, we estimated cocoa pod production at the plot level and the production of banana and plantain at the farm level.
The gross potential income for both organic and conventional farmers was estimated assuming: 10% premium for organic farmers, GH₵ 7.6 (equivalent to US$ 1.53) kg−1 (for the 2017/2018 and 2018/2019 cocoa seasons), and a conversion factor of cocoa pods to beans of 0.04 (Mahrizal et al., Reference Mahrizal, Nalley, Dixon and Popp2012; Rainforest Alliance, 2018). A price range of GH₵ 10.00–GH₵ 25.00 (US$ 2.01 - US$ 5.03) for a bunch of bananas and a range of GH₵ 20.00–GH₵ 30.00 (US$ 4.03–6.04) for a bunch of plantains provided by farmers was applied. Based on our data, an average bunch of banana and plantain were 13.3 kg and 13.1, respectively; thus, unit price ranges of GH₵ 0.75–GH₵ 1.88 (banana) and GH₵ 1.53–GH₵ 2.29 (plantain) were estimated. Gross potential income from cocoa was estimated as a product of an overall number of cocoa pods, a factor for conversion of cocoa pods to beans and unit price of cocoa plus premium for organic farmers and without the premium for conventional farmers. Gross potential income from banana and plantain were estimated as a product of their unit price and quantity. Overall gross potential income was estimated as sum of income from cocoa, banana and plantain.
2.5 Data processing and chemical analysis
2.5.1 Soil moisture content and physical properties
Soil water content (%WC) was calculated as WC = [(S1 – S2)/S2] × 100, where S1 is the fresh weight of soil and S2 is the weight of oven-dried (at 105°C for 48 h) soil. Soil bulk density was estimated as BD (g cm−3) = [(W1 - W2)/V] × (100 - %CF)/100, where CF is coarse soil fraction, BD is bulk density, W1 and W2 are the weights of empty trays and oven-dried soils in trays respectively, and V is the volume of the bulk density cylinder. Soil particle size distribution was assessed via laser ablation (Bechman Coulter LS 200) and classified into textural classes based on the USDA soil triangle (Soil Survey Division Staff, 1993).
2.5.2 Soil chemical analysis
Soil pH was determined in a 1:2.5 soil:solution slurry with a pH meter (pH 209, Hanna Instruments), calibrated with pH 4.01 and 7.00 buffer solutions. The electrical conductivity (EC, mS cm−1) of the soil was measured in the same soil slurry using a portable electrical conductivity meter (Combo pH and EC, Hanna Instruments) calibrated with 1413 μS cm−1 standard solution. To determine Olsen extractable P (hereafter P), soil samples (2 g) were extracted with 30 ml of 0.5 M sodium bicarbonate thoroughly mixed with 0.05% w/v polyacrylamide, well shaken and centrifuged at 3500 rpm for 15 min. Phosphorus in the extract was estimated at 880 nm in a 1 cm cell using a spectrophotometer and the blue phospho-molybdate method with ascorbic acid as reducing agent. Total soil organic carbon (SOC) and total nitrogen (N) were determined using a CN analyzer [Thermo Scientific™ Flash™ 2000 Organic Elemental Analyzer (OEA)]. Extractable Ca, Mg, K and Na and the concentrations of Mn, Al, Cu and Zn were determined using ICP-MS (Thermo Scientific™ iCAP™ TQ) after extracting 2 g of each soil sample with 20 ml of 1 M NH4NO3, centrifuging at 3500 rpm for 30 min, filtering and diluting 1 ml of the supernatant with 9 ml of 2% HNO3. Effective cation exchange capacity (ECEC) was determined as the sum of exchangeable bases and exchangeable acidity. Nutrient stocks (Mg or kg ha−1) were estimated as the product of nutrient concentration, bulk density, depth and unit conversion factor.
2.6 Data and statistical analysis
Soil physical and chemical properties were analyzed using two-way analysis of variance (ANOVA) with farm type and cocoa temporal phase as factors. A general analysis of variance was conducted with soil depth as a factor. Where applicable, the ANOVA was followed by Fisher's Least Significant Difference (LSD). Yield and gross potential income data (cocoa, banana, plantain and overall) and farm characteristics (farm size, standing litter stock, cocoa and shade tree species densities, basal area, richness and diversity) were analyzed via two-way ANOVA with farm type and cocoa temporal phase as the independent factors. All variables, which were not normally distributed, were Box-Cox transformed to meet the normality assumption and those which remained non-normally distributed were analyzed using Kruskal–Wallis ANOVA. Where interaction terms were not significant, only main effects were considered in results and discussion. Spearman's rank correlation was used to analyze the relationship between soil physico-chemical properties and nutrient concentrations as well as between nutrient stocks, crop yield, and stand characteristics (standing litter stock, cocoa tree density and shade tree species density, basal area, richness and diversity). Differences in means were considered significant at p < 0.05.
3 Results
3.1 Farm characteristics, soil moisture content and soil physical properties
The organic systems had greater shade tree species richness, diversity, density and basal area than conventional systems (Appendix Table 2). Standing litter stock, total basal area and the density of food and fruit shade trees/shrubs were greater on organic farms whilst cocoa tree density and total shade tree density were greater on conventional farms. Although organic farms were larger than conventional systems, it did not differentiate stand characteristics, soil properties or farm productivity.
The water contents of the soils were influenced by farm type and depth, but not the temporal phase of cocoa. Soil water content was 33% and 13% greater on organic farms than conventional farms in topsoils (p < 0.001) and subsoils (p = 0.025), respectively, and decreased with soil depth (p < 0.001) (Table 1). Soil gravel content (> 2 mm) was significantly higher in the topsoils of conventional farms compared to organic farms (p = 0.043), subsoil compared to topsoil (p = 0.006) and similar for cocoa temporal phases. Soil bulk density was similar between organic and conventional farms in the topsoils, greater in subsoils of conventional farms (p = 0.029), similar across cocoa temporal phases and increased with depth (p < 0.001). Clay, silt and sand contents were similar in both organic and conventional farms and were classified as loamy for the 0–30 cm depth.
Table 1. Soil water content and selected soil physical properties (Mean ± SEM) of farm types (organic vs conventional) and cocoa temporal phases (young, ≤15 years; mature, 6–30 years; old, ≥31 years) at 0-30 cm as well as the topsoil (0–15 cm) and subsoil (15–30 cm) of cocoa agroforestry systems at Suhum.
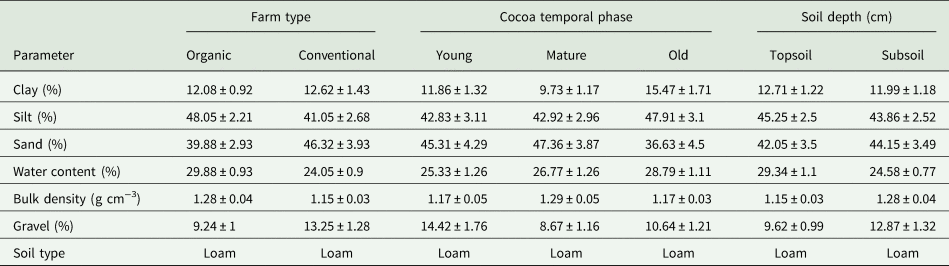
3.2 Soil chemical properties
The effect of farm type on soil electrical conductivity was more pronounced than pH (Fig. 2). Both pH (p = 0.010) and electrical conductivity (p < 0.001) were higher on organic farms than conventional farms in topsoils and decreased with soil depth (pH; p = 0.002; EC, p < 0.001). Effective ECEC was similar for cocoa farms under organic and conventional management, and it was similar across cocoa temporal phases. However, ECEC decreased with soil depth (p < 0.001). The ratios C:N, Ca:Mg, Ca + Mg:K and Ca + Mg:Na + K were similar for both farm types, cocoa temporal phases and soil depths (Appendix Tables 3 and 4). Extractable cations (Na+, Mg2+, Ca2+ and K+) were similar on both farm types and across cocoa temporal phases. The cations Ca2+ (p < 0.001), K+ (p = 0.035) and Mg2+ (p < 0.001) decreased with soil depth.

Fig. 2. Soil chemical properties on organic and conventional cocoa farms (0–15 cm and 15–30 cm) and across cocoa temporal stages (0–30 cm) at Suhum. Panels: 1) electrical conductivity (EC), 2) effective cation exchange capacity (ECEC), 3) pH and 4) C:N ratio. Mean ± SEM are shown and bars with different letters indicate a significant difference (p < 0.05).
3.3 Soil nutrient stocks
Cocoa farms under organic management had 20, 81, 88 and 323% higher stocks of soil organic carbon (p = 0.040), P, Mn and Cu (p < 0.001 in each case), respectively, compared to those under conventional management (Fig. 3). The differences in nutrient stock between organic and conventional farms were more pronounced in the topsoils than subsoils, with significant differences in SOC, Ca, Cu, Mn, and P (Appendix Tables 5 and 6). That notwithstanding, organic farms had higher stocks of P, Mn and Cu in subsoils than the subsoils of conventional systems. The stocks of soil organic carbon were 45% greater in topsoil than subsoil and similar across the different cocoa temporal phases, P was 16% greater in topsoil than subsoil (p = 0.015) and 44–54% greater on mature and old cocoa farms compared to young systems (p = 0.006), and Mn was 19% higher in the subsoil than topsoil (p = 0.021) and similar across cocoa temporal phases. Soil depth had a significant influence on Cu stocks (p < 0.001). Cocoa farms under organic management had similar overall N, Ca, Mg and K stocks as those under conventional management and there were no differences in mean stocks of these nutrients across cocoa temporal phases. That notwithstanding, Mg (p = 0.041) and Ca (p = 0.024) stocks in subsoils were both greater in mature and old cocoa farms compared to young farms (Appendix Tables 5 and 6). Nitrogen stocks decreased with soil depth (p = 0.026) and so was Mg (p < 0.001) and Ca (p = 0.003), but K increased with depth (p = 0.001). Whereas overall Zn stocks were independent of farm type, greater in young cocoa farms than both mature and old farms (p = 0.024), and increased with soil depth (p = 0.035), Na stocks were independent of farm type, cocoa temporal phase and soil depth.

Fig. 3. Nutrient stocks (Mean ± SEM) to the depth 0–15 cm and 15–30 cm in organic and conventional cocoa agroforestry systems at Suhum. The panels represent soil nutrient stocks of 1) P, 2) soil organic carbon (SOC), 3) Mn, 4) N, 5) Cu, 6) Ca, 7) K, 8) Mg, 9) Zn and 10) Na, respectively.
Spearman's rank correlation between soil nutrient stocks and stand characteristics (cocoa density and shade tree species densities, and shade tree species basal area, richness and diversity) showed that P was negatively related to the cocoa density and positively correlated with shade tree basal area (Fig. 4). Mn was positively correlated with shade tree species diversity (Shannon diversity) while Cu was negatively related to cocoa tree density but positively correlated with total standing litter stocks and shade tree species density (r = 0.296, p = 0.041), basal area and richness (r = 0.309, p = 0.033).

Fig. 4. Correlations between soil nutrient stocks and stand characteristics of organic and conventional cocoa systems at Suhum. The panels represent correlations between 1) soil Cu stocks and cocoa tree density, 2) soil P stocks and shade tree basal area, 3) soil Mn stocks and Shannon diversity, 4) soil P stocks and cocoa tree density, 5) soil Cu stocks and shade tree basal area and 6) soil Cu stocks and standing litter stock.
3.4 Crop yield and potential income
Although the production of the medium, large and total (sum of small, medium and large) cocoa pods (number ha−1 yr−1) was 28, 58 and 30% respectively, greater in conventional cocoa farms than organic cocoa systems (Fig. 5, p = 0.010, p < 0.001, p = 0.002, respectively), the production of these cocoa pods per tree were similar between organic and conventional farms. The mean annual production of banana was 570% greater in cocoa systems under organic management compared to those under conventional management (Fig. 5, p < 0.001). However, plantain production was similar in both farm types. The total annual production of banana and plantain was 490% greater in organic farms than conventional farms (Fig. 5, p < 0.001). The mean annual banana production per plant was more than two times greater on organic farms than conventional farms (Org. 0.69 ± 0.14 kg plant−1 vs Con 0.27 ± 0.12 kg plant−1; p < 0.001) while plantain production was similar. Thus, the gross potential income of organic farmers was four times higher for banana production than conventional farmers (Table 2). Although the gross potential income from cocoa was 18% lower on organic farms, the overall gross potential income (sum of income from cocoa, banana and plantain) was similar for both farm types.

Fig. 5. Annual cocoa pod (panel 1), banana and plantain (panel 2) production (Mean ± SEM) in organic and conventional cocoa agroforestry systems at Suhum. Small (< 5 cm), medium (5–10 cm), large (> 10 cm) and total (sum of small, medium and large).
Table 2. Potential gross income from cocoa, banana and plantain production under conventional and organic management in agroforestry systems at Suhum.

Spearman's rank correlation between total cocoa pod production and annual banana and plantain yield with stand characteristics and soil nutrient stocks showed that annual banana and plantain yield was positively correlated to P (r = 0.335, p = 0.020) and Cu (r = 0.432, p = 0.002) stocks. Shade tree basal area (r = 0.518, p < 0.001) and fruit species density (r = 0.680, p < 0.001) positively correlated with annual banana and plantain yield, but cocoa tree density was negatively related to banana and plantain yield (r = −0.413, p = 0.004). Total cocoa pod production was negatively correlated to Cu stocks (r = −0.335, p = 0.020) and shade tree species basal area (r = −0.367 p = 0.010).
4 Discussion
4.1 Effect of organic management on selected soil physical and chemical properties
We found that soil water content was greater (24%) in organic systems than conventional systems and decreased (by 19%) with soil depth as reported by other workers (Reganold, Reference Reganold1988; Suja et al., Reference Suja, Byju, Jyothi, Veena and Sreekumar2017; Di Prima et al., Reference Di Prima, Rodrigo-Comino, Novara, Iovino, Pirastru, Keesstra and Cerdà2018). Soil water content affects the moisture and amount of nutrients available to plants (Appendix Table 7; Kyereh, Reference Kyereh2017). Cocoa is sensitive to drought hence high soil water content is crucial in regions with pronounced dry seasons, such as our study area (Wessel, Reference Wessel1971; Zuidema et al., Reference Zuidema, Leffelaar, Gerritsma, Mommer and Anten2005; Kyereh, Reference Kyereh2017); the organic farms are promising in this context. That notwithstanding, it should be noted that soil moisture content varies with time/season. The application of organic fertilizer, high in organic matter content, coupled with the greater levels of standing litter stock on the organic farms (Appendix Tables 1 and 2) possibly enhanced moisture retention. Organic farmers were keen in maintaining greater levels of shade tree species density, richness and diversity, possibly with the aim of exploiting their ecological benefits (e.g. suppression of weeds and contribution to soil fertility through litterfall) (Asigbaase, Reference Asigbaase2019). The integration of shade trees might have enhanced microclimatic conditions and reduced soil moisture loss through evaporation.
Cocoa farms under organic management had higher (39%) electrical conductivity than those under conventional management, indicating a greater potential for nutrient uptake since increasing electrical conductivity when it is below the range 1–4 dS m−1 means a higher amount of nutrients availability for crop uptake (Fig. 2; Heinen et al., Reference Heinen, Marcelis, Elings, Figueroa and del Amor2002; Aban, Reference Aban2014; Buggenhout, Reference Buggenhout2018). Electrical conductivity (EC) is an indicator of soil health, suggesting improved soil health for the organic farms. EC influences crop yields as well as the activity of soil microorganisms, which play a critical role in nutrient recycling (Heinen et al., Reference Heinen, Marcelis, Elings, Figueroa and del Amor2002; Aban, Reference Aban2014; Brito-Vega et al., Reference Brito-Vega, Salaya-Domínguez, Gómez-Méndez, Gómez-Vázquez and Antele-Gómez2018). The greater levels of soil moisture and P concentration on organic farms than conventional farms as well as their interaction with other physico-chemical properties may explain the differences in mean electrical conductivity (Peralta and Costa, Reference Peralta and Costa2013).
Organic management enhanced pH at the 0–15 cm soil depth than conventional management. The integration of diverse woody plants with dense and deep root system found in the organic systems (Asigbaase et al., Reference Asigbaase, Sjogersten, Lomax and Dawoe2019) possibly captures leached nutrients and return them to the soil through litterfall and decomposition thereby offering a buffer capacity, which enhances soil pH (Hartemink and Donald, Reference Hartemink and Donald2005; Arévalo-Gardini et al., Reference Arévalo-Gardini, Canto, Alegre, Loli, Julca and Baligar2015). Furthermore, abundant litter on the floor, a characteristic of cocoa systems, acts as a permanent soil cover thereby reducing nutrient loss through surface runoffs and leaching (Dawoe et al., Reference Dawoe, Quashie-Sam and Oppong2014; Arévalo-Gardini et al., Reference Arévalo-Gardini, Canto, Alegre, Loli, Julca and Baligar2015). Contrary to the report that cocoa cultivation leads to soil acidification, i.e. lowering of pH (e.g. Ahenkorah et al., Reference Ahenkorah, Halm, Appiah, Akrofi and Yirenkyi1987; Hartemink and Donald, Reference Hartemink and Donald2005), our results showed that pH remained similar across the different cocoa temporal phases (Appendix Tables 3 and 4) which is consistent with other workers (Ofori-Frimpong et al., Reference Ofori-Frimpong, Asase, Mason and Danku2007; Arévalo-Gardini et al., Reference Arévalo-Gardini, Canto, Alegre, Loli, Julca and Baligar2015). The lack of consensus in these reports in relation to the effect of cocoa cultivation on soil pH as the cocoa system mature may be due to differences in soil fertility management regimes.
4.2 Effect of farm management type on the stocks of soil nutrients
Cocoa farms under organic management had greater stocks of the macro-nutrient P compared to those under conventional management (Fig 3; Appendix Table 6); this corroborates with literature (Aban, Reference Aban2014; Wortman et al., Reference Wortman, Galusha, Mason and Francis2012; Suja et al., Reference Suja, Byju, Jyothi, Veena and Sreekumar2017). Adequate P is important for increased cocoa root development, which in turn increases the capacity of cocoa trees to absorb nutrients for growth and productivity (van Vliet et al., Reference van Vliet, Slingerland and Giller2015). West African soils are known to have inherently low P levels, limiting its availability to plants (Boyer, Reference Boyer1973; Nziguheba et al., Reference Nziguheba, Zingore, Kihara, Merckx, Njoroge, Otinga, Vandamme and Vanlauwe2016) but organic management of cocoa agroforestry systems demonstrate the potential to enhance its availability possibly due to increased P mineralization resulting from greater soil moisture content (Table 1), diverse litter as a result of diverse shade tree species (Asigbaase et al., Reference Asigbaase, Sjogersten, Lomax and Dawoe2019) and well-adapted soil biota (Stockdale and Watson, Reference Stockdale and Watson2009; Kremer and Hezel, Reference Kremer and Hezel2013; Domínguez et al., Reference Domínguez, Bedano, Becker and Arolfo2014; Lori et al., Reference Lori, Symnaczik, Mäder, De Deyn and Gattinger2017). The fact that P stocks was positively correlated with shade tree basal area, but negatively related to cocoa tree density may indicate the role shade tree species play in contributing to P stocks in the two systems through litterfall. Furthermore, P availability in the soil depends on several factors such as SOC, pH, EC and moisture content (Appendix Table 7; Ahenkorah, Reference Ahenkorah1981); these factors and their interaction possibly explain the greater availability of P on organic farms. That notwithstanding, soil fertility management in the organic systems such as the application of organic fertilizer which slowly release nutrients into the soil may account for the greater levels of P. The fact that organic cocoa farms maintained greater stocks of SOC compared to conventional farms (Fig 3) show their potential to sustain cocoa production.
According to Boyer (Reference Boyer1973), 30-year-old cocoa trees required 3–4, 0.1, 4–5, 4.5–6 and 1–1.5 kg ha−1 yr−1 of N, P, K, Ca and Mg respectively, for growth. Landon (Reference Landon2014) estimated that to produce 560 kg ha−1 of dry beans, which is relatively closer to the average cocoa yield of 450 kg ha−1 in Ghana, the nutrient uptake of the crop is 25 kg ha−1 N, 4.5 kg ha−1 P, 36 kg ha−1 K. Based on these figures and assuming similar nutritional demand and cocoa planting density, the nutrient stocks reported in our study (Fig. 3) could sustain cocoa growth and production for several years with P and K contents possibly being the main limiting factors (Boyer, Reference Boyer1973; van Vliet et al., Reference van Vliet, Slingerland and Giller2015; Arthur et al., Reference Arthur, Afrifa and Dogbatse2017; Kongor et al., Reference Kongor, Boeckx, Vermeir, Van de Walle, Baert, Afoakwa and Dewettinck2019). The incorporation of cocoa pod husk compost, wood ash, incorporation of pruned materials and management practices (e.g. cover cropping or zero-tillage) which protect the soil from all forms of leaching and erosion might minimize the limiting effects of P and K (Boyer, Reference Boyer1973; van Vliet et al., Reference van Vliet, Slingerland and Giller2015; Arthur et al., Reference Arthur, Afrifa and Dogbatse2017; Kongor et al., Reference Kongor, Boeckx, Vermeir, Van de Walle, Baert, Afoakwa and Dewettinck2019). Nutrient recycling through litterfall, root turnover and rainwash (i.e. stemflow and throughfall) may potentially reduce the limiting effect of P and K contents (van Vliet et al., Reference van Vliet, Slingerland and Giller2015), making the systems sustainable in the long run without synthetic fertilizers.
Organic management enhanced the stocks of the micro-nutrients Mn and Cu compared to those under conventional management (Fig 3) possibly due to the greater mobilization of these nutrients on the organic farms or greater immobilization in cocoa trees on conventional farms (Arévalo-Gardini et al., Reference Arévalo-Gardini, Canto, Alegre, Loli, Julca and Baligar2015). The combined effect of micro-nutrients scarcity in the soil and other factors such as pH affects their availability for uptake by plants and their deficiencies in African soils have been reported (Baligar et al., Reference Baligar, Fageria, Machado and Meinhardt2006; Zingore et al., Reference Zingore, Delve, Nyamangara and Giller2008). Organic management demonstrates the potential to increase the availability of micro-nutrients especially Cu and Mn probably as a result of the use of compost from organic residues such as cocoa pod husks (Zingore et al., Reference Zingore, Delve, Nyamangara and Giller2008; van Vliet et al., Reference van Vliet, Slingerland and Giller2015; Buggenhout, Reference Buggenhout2018). Furthermore, the fact that Mn was positively correlated with shade tree species diversity and Cu was positively related to shade tree species density, basal area and richness possibly shows the importance of rich and diverse shade tree species in increasing the availability of micro-nutrients. Given the fact that decline in soil fertility is a major driver of low cocoa yield and deforestation in West Africa (Wessel and Quist-Wessel, Reference Wessel and Quist-Wessel2015; FAO, 2017; Benefoh, Reference Benefoh2018), the potential of organic management to enhance soil nutrients seems promising in this context.
4.3 Farm management type and crop yield
Our results demonstrate that although total cocoa pod production was 30% lower in organic systems compared to conventional farms, cocoa pod production per tree was similar on both farms, which indicates that the greater total cocoa pod production on conventional farms was due to differences in cocoa tree density (Fig. 5; Appendix Table 2; Asigbaase et al., Reference Asigbaase, Sjogersten, Lomax and Dawoe2019). Moreover, the fact that total cocoa pod production was negatively correlated to shade tree species basal area possibly further suggests that conventional cocoa farmers replaced shade tree species with cocoa trees, which resulted in an overall greater cocoa pod production. The replacement of shade trees with cocoa trees reduces their contribution to soil nutrient replenishment (Asigbaase, Reference Asigbaase2019) and their removal has been linked to increased use of synthetic chemicals (Nunoo et al., Reference Nunoo, Owusu and Darko2014). In the cocoa systems we evaluated, Asigbaase (Reference Asigbaase2019) reported that shade trees accounted for 30–47% of all annual macro-and micro-nutrient return (except Ni and Zn) on organic farms vs 20–35% on conventional systems. Schneider et al. (Reference Schneider, Andres, Trujillo, Alcon, Amurrio, Perez, Weibel and Milz2017) reported similar cocoa yield on both organic and conventional agroforestry farms in Bolivia whilst Jacobi et al. (Reference Jacobi, Schneider, Mariscal, Huber, Weidmann, Bottazzi and Rist2015) reported greater yields in organic agroforestry systems compared to conventional systems in Bolivia. Organic farmers, on the other hand, had 490% greater annual banana and plantain yield compared to conventional farmers indicating the potential for a more diversified approach to cocoa production (Fig. 5; Badgley et al., Reference Badgley, Moghtader, Quintero, Zakem, Chappell, Avilés-Vázquez, Samulon and Perfecto2007; Jacobi et al., Reference Jacobi, Schneider, Mariscal, Huber, Weidmann, Bottazzi and Rist2015).
The idea that organic farmers sought to diversify farm output is confirmed by the significant positive correlation of annual banana and plantain production with shade tree species basal area and fruit species density and its negative correlation with cocoa tree density. That is, conventional cocoa farmers replaced shade tree species with cocoa trees, whereas organic farmers incorporated more fruit species and shade tree species. Our study demonstrates that organic cocoa management was achieved with a higher overall yield of co-products (i.e. banana and plantain) and lower overall cocoa pod production. Other workers have described organic farming as climate smart (Bandanaa et al., Reference Bandanaa, Egyir and Asante2014) and resilient farming systems (Jacobi et al., Reference Jacobi, Schneider, Mariscal, Huber, Weidmann, Bottazzi and Rist2015) due to their greater shade tree species diversity. The organic systems we evaluated had greater shade tree densities, basal area, richness and diversity than conventional farms (Appendix Table 2). Furthermore, the production of co-products such as banana and plantain which has the potential to diversify income and reduce shocks is important in the discussion about adaptation to climate change and climate change mitigation (Scialabba and Müller-Lindenlauf, Reference Scialabba and Müller-Lindenlauf2010; Jacobi et al., Reference Jacobi, Schneider, Mariscal, Huber, Weidmann, Bottazzi and Rist2015; Schneider et al., Reference Schneider, Andres, Trujillo, Alcon, Amurrio, Perez, Weibel and Milz2017). For example, organic farmers’ overall potential income was comparable to conventional farmers when banana and plantain production was considered (Table 2). That notwithstanding, the net ecological and economic benefits of organic cocoa cultivated under diverse shade trees must outweigh that of the conventional farms to make them attractive to farmers (Badgley et al., Reference Badgley, Moghtader, Quintero, Zakem, Chappell, Avilés-Vázquez, Samulon and Perfecto2007).
5 Conclusions
Organic management of cocoa agroforestry systems enhanced the moisture content and chemical properties of the soil than conventional management. The soils of organic cocoa agroforestry systems had high nutritional content and were suitable for sustainable cocoa production. Organic cocoa producers diversified farm output by incorporating more shade tree and fruit species which resulted in greater annual banana and plantain production while conventional farmers replaced shade tree and fruit species with cocoa trees which resulted in greater total cocoa pod production. Organic management demonstrated the potential to enhance P stocks, a major nutrient which is often limited in African soils. We conclude that organic management of cocoa agroforestry systems results in soils with the greater overall quality for cocoa production than conventional management and that it increases the yield of co-products from shade trees/plants which farmers were keen to maintain on their farms as a soil fertility management strategy. Future studies may explore the impact of organic management of cocoa agroforestry systems at the landscape and regional scales to further improve our understanding and support policy.
Acknowledgment
Special thanks to the cocoa farmers and field assistants who supported us during field data collection. We thank Mr. Kofi Mark and Mr. Eric Odei for facilitating our engagement with the farmers. We also thank Saul Vazquez Reina and Li Dongfang for offering technical support on chemical analysis. We appreciate COCOBOD for facilitating our contact with cocoa farmers.
Funding
This work was supported by the Ghana Education Trust Fund (GET FUND, http://www.getfund.gov.gh/) as part of a doctoral study at the University of Nottingham, UK. The funder had no role in study design, data collection and analysis, decision to publish, or preparation of the manuscript.
Conflict of interests
On behalf of all authors, the corresponding author states that there is no conflict of interest.
Supplementary material
The supplementary material for this article can be found at https://doi.org/10.1017/S1742170520000290