Introduction
Sugarcane (Saccharum spp.) is one of the most agronomical and economically suitable forage sources for ruminants in tropical and sub-tropical regions. It shows high dry matter (DM) production (25–40 t/ha; Ávila et al., Reference Ávila, Pinto, Figueiredo and Schwan2009) and presents up to 571 g/kg total digestible nutrients after being ensiled. However, the fibre content of sugarcane exceeds 600 g/kg of DM (de Andrade et al., Reference de Andrade, Rodrigues, Detmann, Valadares Filho, Castro, Trece, Silva, Fischer, Weiss and Marcondes2016), which impairs digestibility (Aroeira et al., Reference Aroeira, Lizieire, Matos and Figueira1993) and compromises animal feed intake and performance (Corrêa et al., Reference Corrêa, Pereira, de Oliveira and Ramos2003).
Among enzymatic supplements, fibrolytic enzymes are an emerging additive used in ruminant diets to increase fibre digestion. This has been observed both in vitro (Bowman et al., Reference Bowman, Beauchemin and Shelford2002; Kung et al., Reference Kung, Cohen, Rode and Treacher2002) and in vivo (Arriola et al., Reference Arriola, Oliveira, Ma, Lean, Giurcanu and Adesogan2017; Gandra et al., Reference Gandra, Miranda, Goes, Takiya, Del Valle, Oliveira, Freitas Junior, Gandra, Araki and Santos2017) trials. However, some studies (Sheperd and Kung, Reference Sheperd and Kung1996; Daniel et al., Reference Daniel, Queiroz, Arriola, Staples, Romero, Shin, Paschoaloto, Nussio and Adesogan2016) show no effects of exogenous enzymes on animal digestion and performance. Inconsistent results could be associated with enzyme dose, basal diet (Bowman et al., Reference Bowman, Beauchemin and Shelford2002) and application method (total mixed ration, concentrate or silage) (Yang et al., Reference Yang, Beauchemin and Rode1999).
Gandra et al. (Reference Gandra, Miranda, Goes, Takiya, Del Valle, Oliveira, Freitas Junior, Gandra, Araki and Santos2017) reported positive effects of xylanase (XYL) and cellulase addition on fibre digestibility when animals were fed with sugarcane silage, whereas no effect of these enzymes was observed in animals fed a maize silage diet. On the other hand, the addition of enzymes in grass (Mandebvu et al., Reference Mandebvu, West, Froetschel, Hatfield, Gates and Hill1999; Dean et al., Reference Dean, Adesogan, Krueger and Littell2005), sorghum (Xing et al., Reference Xing, Chen and Han2009) and maize (Sheperd and Kung, Reference Sheperd and Kung1996; Ying et al., Reference Ying, Borjigin and Zhu2017) at ensiling increases silage digestibility. Degradation of xylan produces mainly acetic acid as an end-product during fermentation (Fred et al., Reference Fred, Peterson and Davenport1919; Dehghani et al., Reference Dehghani, Weisbjerg, Hvelplund and Kristensen2012). Acetic acid reduces pH and inhibits the growth of spoilage organisms, being associated with increased aerobic stability (Danner et al., Reference Danner, Holzer, Mayrhuber and Braun2003). To the authors’ knowledge, there is no study in the literature evaluating the effect of XYL on ensiled sugarcane.
The aim of the current study was to determine the effects of increasing levels of XYL on sugarcane silage fermentation, gases and effluent losses, chemical composition, DM and neutral detergent fibre (NDF) degradation and aerobic stability. It was hypothesized that XYL improves DM and NDF degradation, alters parameters of fermentation and improves chemical composition and aerobic stability of sugarcane silage.
Materials and methods
The experiment was conducted between July and September of 2016, at the Group of Agriculture Study and Labor (GETAP) of Agricultural Sciences Center (CCA), Federal University of São Carlos (UFSCar), in Araras, Brazil: 22°18′ S latitude, 47°22′ W longitude and 665 m asl.
Treatments and experimental design
Sugarcane (Cultivar RB02-5799) was harvested and ensiled 11.5 months after planting (first cut), and solids concentration of sugarcane juice (BRIX) was 210 g/kg (Table 1). Evaluation of BRIX was performed using the refractometric method (990.35 of AOAC, 2000). Sugarcane was harvested manually from five batches and chopped in a forage harvester (90 z-10, JF, Itapira, Brazil). Particle size of processed sugarcane was analysed using the Penn State Particle Separator (Maulfair et al., Reference Maulfair, Fustini and Heinrichs2011). Immediately before ensiling, lyophilized enzyme (powder) was individually weighed, top-dressed to chopped sugarcane and hand mixed. The sugarcane was ensiled with specific density of 600 kg/m3 in silos comprising of a plastic bucket, 28 cm in diameter, 25 cm high and equipped with a Bunsen valve to allow gas to escape. The trial was performed in a completely randomized design with five treatments and ten repetitions per treatment. Treatments were: 0, 100, 200, 300 and 400 mg of XYL/kg DM of sugarcane. Enzyme contained 10 000 U/g (Beijing Smile Feed Sci. & Tech. Co., Ltd.).
Table 1. Chemical composition of sugarcanea

a First cut of RB025799 cultivar sugarcane, which showed 210 g/kg of soluble carbohydrates, at 11.5 months after plantation.
b NFC = 1000 − [(CP − neutral detergent insoluble protein) + EE + ash + NDF].
c Net energy of lactation, calculated according to NRC (2001).
Fermentative profile and loss evaluation
Five kilograms of sand were placed at the bottom of a plastic bucket with a nylon screen separating the chopped sugarcane to be ensiled. The weight of empty and sealed silos at ensiling and after 60 days was recorded using a 5-g sensitivity scale (Mettler Toledo, Barueri, Brazil). Gas losses (GL) were obtained by difference between silo weight at ensiling (ESW) and opening (OSW), according to the following equation:

where EDM was the ensiling DM. The difference between weight of empty silo before ensiling (EESW) and after opening (OESW) was considered effluent losses (EL), according to the following equation:

Total DM losses were obtained by sum of gas and effluent production according to Jobim et al. (Reference Jobim, Nussio, Reis and Schmidt2007). Dry matter recovery (DMR) was calculated by the ratio between DM at silos after silos opening (ODM, kg) and ensiled DM:

After opening silos, a sample of 15 g was collected from each silo and diluted in 150 ml of distilled water (ratio 1 : 10). It was processed in a blender for 30 s (Cherney and Cherney, Reference Cherney, Cherney, Buxton, Muck and Harrison2003) and then squeezed through four layers of cheesecloth to extract fluid for pH measurement using a digital potentiometer (LUCA-210, Lucadema, Sao José do Rio Preto, Brazil). Filtered samples were frozen for subsequent evaluation of ammonia-N (NH3-N), acetate, propionate, ethanol and lactate.
Samples of silage extract were thawed to room temperature and centrifuged (500 g for 15 min). For NH3-N content evaluation, extracts (2 ml) were diluted with sulphuric acid (1 N; 1 ml) and analysed by the colorimetric phenol-hypochlorite method (Broderick and Kang, Reference Broderick and Kang1980). Samples were analysed for lactic acid concentration using a spectrophotometric method (Pryce, Reference Pryce1969): samples were diluted in phosphoric acid solution and centrifuged (3000 g for 5 min); supernatant was homogenized with sulphuric acid and heated to 75 °C for 2.5 min; after cooling, colour reagent (4-phenylphenol, Sigma Aldrich, St. Louis, MO, USA) was added; the sample was heated to 90 °C for 1.5 min and, after cooling, read in the spectrophotometer at 560 nm. Other samples were acidified using formic acid in a 1 : 4 ratio for further ethanol, acetic, propionic and butyric acids analyses. Organic acids were determined by gas chromatography (GC-2010 Plus chromatograph, Shimadzu, Barueri, Brazil), equipped with an AOC-20i auto-sampler, Stabilwax-DA™ capillary column (30 m, 0.25 mm internal diameter, 0.25 µm df, Restek©) and a flame ionization detector. A 1 µl aliquot of each sample was injected with a split ratio of 40 : 1, using helium (He) as the carrier gas at linear velocity of 42 cm/s, in a chromatographic run of 11.5 min. The injector and detector temperatures were 250 and 300 °C, respectively, and the initial temperature of the column was 40 °C. The temperature ramp of the column started with a gradient from 40 to 120 °C at 40 °C/min rate, followed by a gradient from 120 to 180 °C at 10 °C/min rate and from 180 to 240 °C at 120 °C/min, keeping temperature at 240 °C for 3 min at the end. For quantification, a calibration of the method was made using diluted solutions of the WSFA-2 standard (Ref. 47056, Supelco©) and ethanol (Ref. 459828, Sigma-Aldrich©) analysed under the conditions described above. Peak detection and integration were performed using the GCsolution v. 2.42.00 software (Shimadzu©).
Chemical composition and in situ degradation
A sample (100 g) was collected from the centre of silos immediately after opening and frozen until analysis. Samples were pre-dried at 60 °C in a forced-air oven for 72 h and ground in a Wiley mill (SL-31, SolabCientífica, Piracicaba, Brazil) either through a 2-mm or a 1-mm sieve. Samples (1-mm processed) were analysed for DM (method 950.15, AOAC, 2000), ash (method 942.05, AOAC, 2000), crude protein (CP) (N × 6.25; method 984.13, AOAC, 2000), ether extract (EE, method 920.39, AOAC, 2000), NDF using α-amylase without addition of sodium sulphite (Van Soest et al., Reference Van Soest, Robertson and Lewis1991) and acid detergent fibre (ADF) according to Van Soest et al. (Reference Van Soest, Robertson and Lewis1991). Net energy concentration of sugarcane was estimated according to NRC (2001) and non-fibre carbohydrate (NFC) according to Hall (Reference Hall2000). DM and NDF degradation were determined in situ. Samples (processed at 2-mm) were placed in bags of non-woven fabric tissue (5 × 5 cm and 100 g DM/m2; Casali et al., Reference Casali, Detmann, Valadares Filho, Pereira, Henriques, de Freitas and Paulino2008) and incubated for 96 h in the rumen of two Holstein cows. After incubation, samples were washed in running tap water and analysed for NDF content as described previously.
Aerobic stability
For aerobic stability, samples of silage (3 kg) were placed in a plastic bucket and maintained in a controlled temperature room (22 ± 1.7; mean ± s.d.) for 5 days. Temperature of the silage centre was recorded every 8 h using an infrared digital thermometer (DT-8380, Tianjin Cheerman Technology Co. Ltd., Tianjin, China). Silage pH was evaluated every 24 h, using the method described previously. Aerobic stability was defined as the number of hours the silage remained <2 °C above the ambient temperature (Ranjit and Kung, Reference Ranjit and Kung2000).
Statistical analysis
Data were analysed using PROC MIXED of SAS 9.3 (SAS Inst. Inc., Cary, NC, USA), according to the following model:

with $e_{ij}\approx N(0,\sigma _{\rm e}^2 )$, where Y ij is the value of dependent variable; μ is the overall mean; X i is the fixed effect levels of XYL (i = 1 to 5); e ij is the residual error and N stands for Gaussian distribution. Degrees of freedom were corrected by Kenward and Rogers (Reference Kenward and Roger1997) method. XYL enzyme levels were tested for linear and quadratic effects using polynomial regression. Equations that describe the effect of XYL level were obtained for every variable.
Silage pH and temperature after aerobic exposition were analysed as repeated measures, according to the following model:

with $\omega _{ijk}N(0,\; \sigma _\omega ^2 )$ and e ijkMVN(0, R); where Y ijk is the value of dependent variable; μ is the overall mean; X i is the fixed effect levels of XYL enzyme (i = 1 to 5); T k is time fixed effect (k = 1 to 5 for pH and k = 1 to 15 for temperature); ω ij is the random effect of silo; T × X ki is the interaction effect of time and XYL enzyme; e ijk is the residual error; N stands for Gaussian distribution;
$\sigma _\omega ^2 $ is the variance associated with experimental silo; MVN stands for multivariate normal and R is the variance–covariance matrix of residuals due to the repeated measurements. It was evaluated the following variance–covariance matrix (CS, CSH, AR(1), ARH(1), TOEP, TOEPH, UN, FA(1) and ANTE(1)). The matrix was chosen using a Bayesian method. Statistical differences were defined as P ⩽ 0.05 and tendency towards significance was considered at 0.05 < P ⩽ 0.10.
Results
The addition of XYL showed a quadratic effect (P ⩽ 0.001) on silage pH and acetic acid concentration (Table 2). According to regression, the lowest level of silage pH was found at an enzyme inclusion of 211 mg/kg DM (Table 3). Similarly, the highest acetic acid concentration was found at 185 mg/kg DM of XYL. Enzyme had no effect (P ⩾ 0.445) on ethanol concentration but linearly decreased lactic acid concentration (P < 0.001). Furthermore, there was a quadratic effect (P = 0.003) of XYL on effluent and total losses. The highest effluent and total losses were obtained at 178 and 184 mg/kg DM of XYL, respectively. However, there was no effect (P ⩾ 0.153) of enzyme on GL and DM recovery.
Table 2. Effect of XYL level on sugarcane fermentation profile and losses

a Probabilities: Lin, linear; Qua, quadratic.
Table 3. Regression coefficients and quadratic maxima for variables with linear and quadratic effects of XYL level

a Level of XYL for maximal or minimal response = −linear coefficient/(2 × quadratic coefficient).
Although XYL had no effect (P ⩾ 0.141) on DM, ADF or EE content, it showed a quadratic affect (P ⩽ 0.027) on organic matter (OM), NFC and CP and tended to have a quadratic effect (P = 0.063) on NDF content (Table 4; Fig. 1). Intermediary levels of enzyme showed the highest concentration of OM (186 mg/kg DM) and NFC (218 mg/kg DM), with the lowest values of NDF (227 mg/kg) and CP (184 mg/kg DM; Table 3). Additionally, XYL had no effect (P ⩾ 0.216) on DM degradation and tended to quadratically affect (P = 0.053) NDF degradation (Fig. 1).

Fig. 1. Effect of XYL level on sugarcane silage NDF and degradation (mean ± SEM).
Table 4. Effect of XYL level on sugarcane silage chemical composition and ruminal in situ degradation

DM, dry matter; NDF, neutral detergent fibre; ADF, acid detergent fibre; NFC, non-fibre carbohydrates; CP, crude protein; EE, ether extract.
a Probabilities: Lin, linear; Qua, quadratic.
Besides the quadratic effect (P = 0.001) of XYL on silage pH at opening, which was still apparent (P ⩽ 0.006) 48 h after aerobic exposure (Fig. 2), in general, there was no XYL effect (P ⩾ 0.543) on silage pH after aerobic exposure (Table 5). Similarly, treatments showed no effect (P = 0.791) on silage temperature after aerobic exposure (Fig. 3) and, consequently, the time of stability was not affected (P ⩾ 0.658) by XYL (Table 5).

Fig. 2. Effect of XYL level on sugarcane silage pH after aerobic exposure (mean ± SEM).
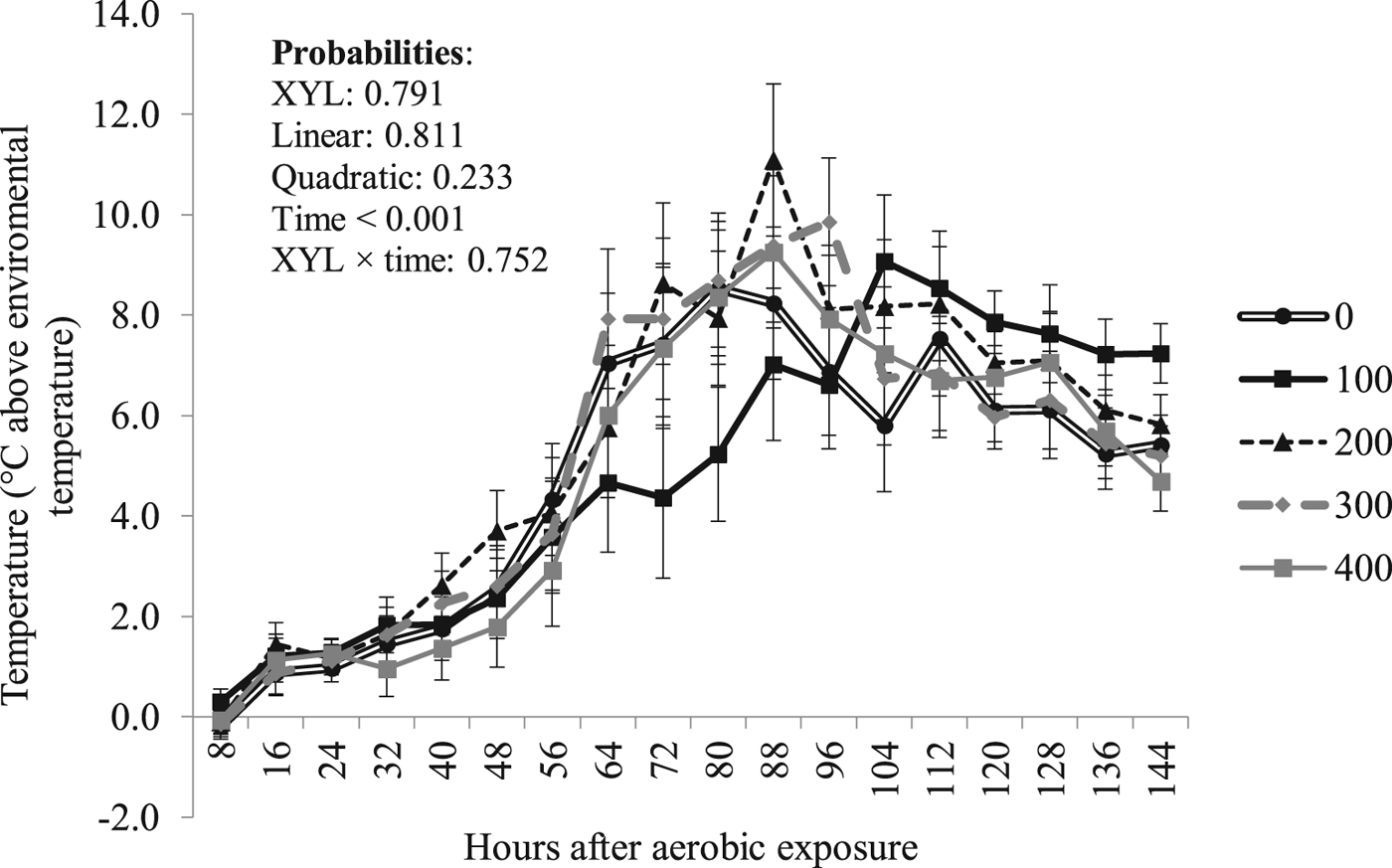
Fig. 3. Effect of XYL enzyme level on sugarcane silage temperature after aerobic exposure (mean ± SEM).
Table 5. Effect of XYL level on sugarcane silage aerobic stability

a Probabilities: Lin, linear; Qua, quadratic; INT, treatment and time interaction effect.
Discussion
Ensiled sugarcane had 426 g/kg of NFC, in the current study. According to McDonald et al. (Reference McDonald, Henderson and Heron1991), NFC is mainly sucrose, which can be fermented by lactic acid bacteria to produce organic acids and decrease pH. XYL had a quadratic effect on many variables related to silage fermentation, fermentative losses and chemical composition. He et al. (Reference He, Yang, Yang, Beauchemin, Tang, Huang, Zhou, Han, Wang, Kang, Odongo and Tan2015) previously reported a quadratic effect of XYL activity on degradation of some forages. These authors highlighted that the main factors associated with negative effects of elevated XYL levels are: blocking the enzyme binding sites (Nsereko et al., Reference Nsereko, Morgavi, Rode, Beauchemin and McAllister2000) and decreasing microbial attachment to substrate (Morgavi et al., Reference Morgavi, Beauchemin, Nsereko, Rode, McAllister and Wang2004). These mechanisms could have negated the enzyme effect at higher levels of application. Beauchemin et al. (Reference Beauchemin, Rode and Sewalt1995) also observed improved fibre digestibility in cattle fed intermediate levels of XYL and cellulase but not at high levels of inclusion.
In the current study, intermediate levels of XYL increased acetic acid and decreased silage pH. Increased acetic acid could be associated with degradation of xylan (Fred et al., Reference Fred, Peterson and Davenport1919; Dehghani et al., Reference Dehghani, Weisbjerg, Hvelplund and Kristensen2012), with a consequent decrease in NDF content. However, enzyme-induced release of polysaccharides available for microbial fermentation increased acid production (Eun and Beauchemin, Reference Eun and Beauchemin2008), resulting in decreased silage pH (Khota et al., Reference Khota, Pholsen, Higgs and Cai2016). Similar reduction in pH was obtained when fibrolytic enzyme was applied to maize silage (Sheperd and Kung, Reference Sheperd and Kung1996; Colombatto et al., Reference Colombatto, Mould, Bhat, Phipps and Owen2004), bermudagrass (Dean et al., Reference Dean, Adesogan, Krueger and Littell2005) and alfalfa silages (Nadeau et al., Reference Nadeau, Russell and Buxton2000).
Although it was hypothesized that increased acetic acid could prevent the growth of spoilage microorganisms (Daniel et al., Reference Daniel, Checolli, Zwielehner, Junges, Fernandes and Nussio2015), no effects were observed in ethanol concentration, and XYL decreased lactic acid concentration linearly in the silage. Other studies reported positive effects (Dehghani et al., Reference Dehghani, Weisbjerg, Hvelplund and Kristensen2012; Ying et al., Reference Ying, Borjigin and Zhu2017) or no effect (Sheperd and Kung, Reference Sheperd and Kung1996; Mandebvu et al., Reference Mandebvu, West, Froetschel, Hatfield, Gates and Hill1999) of fibrolytic enzymes in maize and grass silages. Differences between cited studies and the current work could be explained by the ensiled material. Higher levels of water-soluble carbohydrates, at intermediary enzyme levels could increase fermentative losses, following rapid fermentation (McDonald et al., Reference McDonald, Henderson and Heron1991).
According to Kung et al. (Reference Kung, Schmidt, Ebling and Hu2007), rapid lactic acid production and silage pH decline results in a favourable environment for the proliferation of yeasts, increasing ethanol production and EL. In the current study, XYL had no effect on ethanol concentration; however, there was a quadratic effect of XYL on effluent and total losses, probably due to higher carbohydrate solubilization and fermentation by yeast. Dean et al. (Reference Dean, Adesogan, Krueger and Littell2005) reported decreased DM losses on bermudagrass silage treated with a specific type of fibrolytic enzyme. However, it is important to highlight that increased acid production and decreased pH generally results in increased fermentative losses in sugarcane silage (Pedroso et al., Reference Pedroso, Nussio, Paziani, Loures, Igarasi, Coelo, Packer, Horii and Gomes2005).
When applied before feeding, XYL enzyme could alter the structure of the feed, making it more favourable to degradation (Beauchemin et al., Reference Beauchemin, Rode and Sewalt1995). XYLs and cellulases have been found to be effective in degrading cell wall carbohydrates in forage and, therefore, reducing NDF content in silage (Sheperd and Kung, Reference Sheperd and Kung1996; Colombatto et al., Reference Colombatto, Mould, Bhat, Phipps and Owen2004; Desta et al., Reference Desta, Yuan, Li and Shao2016; Khota et al., Reference Khota, Pholsen, Higgs and Cai2016; Liu et al., Reference Liu, Li, Desta, Zhang and Shao2016). In the current study, intermediate levels of XYL decreased NDF content and degradation. Enzymes act on the most digestible content of the NDF (xylan), resulting in a silage with lower NDF, similar content of indigestible fraction, and proportionally higher indigestible fraction. Therefore, no improvement in nutritive value of silage was observed with XYL supply. Besides treatment effects on NDF silage concentration, contents of NDF and CP were higher in silage than in fresh sugarcane. This increased concentration is a consequence of NFC losses, increasing fibrous components of silage.
Aerobic deterioration of silage is a complex process that depends on many factors. During aerobic exposure, acids and other substrates are oxidized by aerobic bacteria, yeasts and moulds (McDonald et al., Reference McDonald, Henderson and Heron1991). Ying et al. (Reference Ying, Borjigin and Zhu2017) reported increased aerobic stability in maize silage treated with fibrolytic enzyme and associated the increase in acetic acid concentration with improvements in aerobic stability. Danner et al. (Reference Danner, Holzer, Mayrhuber and Braun2003) reported that the existence of acetic acid was able to inhibit the growth of yeasts and moulds to improve aerobic stability. Therefore, despite increased acetic acid concentration, XYL showed no effect on aerobic stability in the current study. Evaluating silage pH after aerobic exposure, it was observed that the quadratic effect found at silo opening was still apparent at 24 and 48 h after aerobic exposure, without major physiological and practical implications.
Conclusion
The use of fibrolytic enzymes in sugarcane silage alters fermentative profile and chemical composition, without affecting aerobic stability. Contrary to expectations, intermediate levels of XYL (around 200 mg/kg) decreases NDF degradation, despite reducing NDF content.
Author ORCIDs
T. A. Del Valle, 0000-0001-8093-7132
Acknowledgements
The authors wish to thank Prof. Dr. Reinaldo G. Bastos and Luiz F. A. Mattos, from UFSCar, for providing the physical infrastructure and staff to organic acids evaluations.
Financial support
The authors are grateful for institutional support from Fundação de Apoio Institucional (FAI/UFSCar).
Conflict of interest
The authors declare that there are no conflicts of interest.
Ethical standards
Not applicable.