INTRODUCTION
This review focuses on the digenetic trematodes Schistosoma japonicum, Fasciola hepatica and F. gigantica as each is an important zoonosis of man, particularly in Asia. Vaccines against S. mansoni have been reviewed extensively elsewhere (Pearce, 2003; Bergquist, Leonardo and Mitchell, 2005; Capron et al. 2005). Because of their relatedness and similarities in life cycle the reader will notice a clear parallel in the approaches taken towards vaccine development (choice of antigens, technology to produce vaccines etc.) for each of these parasites. In addition, the concept of producing a transmission blocking vaccine, i.e. one that lowers the level of parasites in the environment and thus prevents infection to other animals and humans, has been suggested as an important component of disease control (Dalton et al. 1996; Williams et al. 2002). This is particularly pertinent as in some regions, Asia and Egypt in particular, where both schistosomiasis and fasciolosis are endemic in animals and/or humans and, therefore, disease control programmes will need to take both into account.
VACCINES AGAINST SCHISTOSOMA JAPONICUM
Over 250 million people and significant numbers of domestic livestock animals are infected and 280000 individuals die as a direct result of schistosomiasis infection in sub-Saharan Africa alone annually (van der Werf et al. 2003). A recent important article by King, Dickman and Tisch (2005) indicates that, in terms of morbidity and mortality, schistosomiasis represents an even greater disease burden than previously appreciated. The construction of dams and the development of important irrigation schemes are often followed by epidemic outbreaks of the disease. Despite the availability of the highly efficient drug, praziquantel (PZQ), there is considerable spreading of schistosomiasis into new endemic areas (Ross et al. 2002). After many years of experience it is generally agreed that mass PZQ chemotherapy, although the mainstay of current control programmes, does have significant limitations. In particular, mass treatment does not prevent re-infection. Furthermore, despite the fact there is not yet clear-cut evidence for the existence of PZQ-resistant schistosome strains, decreased susceptibility to the drug has been reported in several countries. Although schistosomiasis is effectively treated with PZQ, rapid reinfection with rebound morbidity precludes effective control based on chemotherapy alone and justifies current efforts to develop vaccines for these parasites.
Unlike the African schistosomes (S. mansoni, S. haematobium), schistosomiasis japonica is a zoonosis and a major disease risk for more than 40 million people living in the tropical and sub-tropical zones of China (Chen and Feng, 1999) and several millions more in the Philippines (Blas et al. 2004). Despite extensive and concerted control efforts, it is estimated that over one million Chinese and several hundred thousand livestock, including over 200000 bovines, are currently infected (Ross et al. 2001) with the major endemic foci being the marsh and lake regions of Southern China. Construction of the massive Three Gorges Dam across the Yangtze River in Southern China is expected to increase schistosomiasis japonica transmission significantly and introduce the infection into areas currently unaffected (Ross et al. 2001). Vaccines (for use in livestock animals, particularly bovines and humans) in combination with other control strategies, including the use of new drugs, are needed to make elimination of the disease possible (Todd and Colley, 2002). The approach that several groups (Shi et al. 2001; He et al. 2003; Zhang et al. 2006; Zhu et al. 2006) are taking incorporates the development of a transmission blocking veterinary vaccine. The vaccine would be used in reservoir hosts of S. japonicum that potentially will reduce transmission to humans at least in China. Bovines (cattle and water buffalo) are the major reservoirs for S. japonicum infection in China with estimates that 90% of egg contamination comes from this source (Chen and Lin, 2004). Schistosomiasis japonica was once highly prevalent in other domestic animals such as pigs but, in recent years, these animals have been of less importance because they are usually restricted to pens, with limited access to the marshland areas. Sheep and goats are also infected but to a far lesser extent and, as wild animals become rarer and rarer, their involvement in transmission can probably be ignored (Chen and Lin, 2004).
The results of control technology advances, including the success of recent World Bank inputs, were used by Willams et al. (2002) to mathematically model prospects for the future control of schistosomiasis in China. Another mathematical model of the dynamics and control of S. japonicum transmission on Bohoiol island, the Philippines was developed by Ishikawa et al. (2006). Furthermore, a bovine drug intervention trial (Guo et al. 2006) was recently concluded in schistosome-endemic communities in Jiangxi Province, China, which indicated that buffalo are responsible for 75–80% of schistosomiasis transmission in the marshland areas.
A recently published special issue of Acta Tropica entitled “Control of Schistosomiasis in China” (Volume 96, issue 203, 2005) provides a major overview of control strategies, including vaccine development, and provides a unique insight into various facets of the disease that are entirely complementary with this review.
S. japonicum vaccine development – basic considerations
Convincing arguments support the likelihood that effective vaccines against schistosome infection can be developed. Recent progress has provided the basic framework for the development of an effective vaccine against S. japonicum and S. mansoni and comprehensive reviews of the area are available (Pearce, 2003; Hagan and Sharaf, 2003; McManus and Bartley, 2004; Lebens et al. 2004; Capron et al. 2005; Da'dara and Harn, 2005; McManus, 2005; Wu, Lu and Yu, 2005). Schistosomulum life cycle stage antigens are likely to be major vaccine candidate targets of protective immune responses. Zoonotic transmission adds to the complexity of S. japonicum control programmes, but provides a unique opportunity to develop a transmission blocking veterinary vaccine to help prevent human infection and disease. Vaccination with radiation-attenuated cercariae induces significant levels of resistance to S. japonicum challenge in mice, rats, rabbits, sheep and bovines; in the case of water buffalo, greater than 95% protection has been achieved (Shi et al. 1990) by immunization with an ultaviolet-irradiated cercarial vaccine. The initial protection in mice is conferred by Th1 cellular immunity with lymphoid proliferation in regional and mediastinal lymph nodes. Appropriately timed passive transfer of sera from vaccinated mice can also confer protection. This protection is antibody mediated, with predominantly IgG (less so IgM) antibodies acting on the lung schistosomula stage. IgE depletion did not influence protection in these studies. Later, extensive studies both in animal models and in humans suggest that both Th1 and Th2 responses may contribute to protection (Pearce and MacDonald, 2002; Capron et al. 2005; Leenstra et al. 2006). Unfortunately, studies of protective immunity, in bovine schistosome infections are few (De Bont and Vercruysse, 1998), and, consequently, our knowledge of the immunology of S. japonicum infections in bovines is extremely limited. This is particularly the case for water buffalo where immunological reagents for studying immune responses are scarce. Studies on the immunology of buffaloes represent an important area for future research as such information may prove useful in candidate S. japonicum vaccine antigen selection and in defining the optimum route of immunization.
VACCINE CANDIDATES AND PROTECTIVE POTENCY
Considerable efforts have been aimed at the identification of relevant S. japonicum antigens that may be involved in inducing protective immune responses, with a view to developing them further as viable vaccines. Vaccination can be either targeted towards the prevention of schistosome infection or to the reduction of parasite fecundity. A reduction in worm numbers is the gold standard for anti-schistosome vaccine development but, as schistosome eggs are responsible for both pathology and transmission, a vaccine targeted on parasite fecundity and egg viability appears is also relevant.
Some of the leading S. japonicum vaccine candidates (as recombinant protein and/or DNA vaccines) are discussed below and their protective efficacy in different animal models summarised. The majority are membrane proteins, muscle components or enzymes and further details of the characteristics and efficacy of these and other molecules can be found in Wu et al. (2005) and McManus (2005).
Glutathione S-transferase (Sj26GST)
Gluathione S-transferases (GSTs) are a group of enzymes that catalyse the detoxification of lipophilic molecules by thio-conjugation. In light of their physiological importance, a number of research groups have investigated the potential of the GSTs as vaccine targets. Some encouraging data are available for the protective efficacy of the Sj28GST against S. japonicum in China (McManus, 2005) but most work has concentrated on the 26 kDa isoform. Recombinant (r) Sj26GST induces a pronounced anti-fecundity effect, as well as a moderate but significant level of protection in terms of reduced worm burden in mice, sheep, cattle and pigs following challenge infection with S. japonicum (Wu et al. 2005). Similar levels of vaccine efficacy were obtained in water buffaloes vaccinated with purified reSj26GST (Wu et al. 2005). Anti-Sj26GST antibodies were produced in the immunised buffaloes and, following challenge with S. japonicum cercariae, the typical anti-fecundity effect was manifest, characterized by a significant decrease in faecal egg output and eggs deposited in host tissues with those in the liver and intestine being reduced by about 50%. In addition to the anti-fecundity effect, reSjc26GST reduced by nearly 40% the egg-hatching capacity of S. japonicum eggs into viable miracidia. Recent field trials have demonstrated that the protective effect of the reSjc26GST vaccine against S. japonicum can be maintained in cattle and buffaloes for at least 12 months (Wu et al. 2005; McManus, 2005).
Paramyosin (Sj97)
Paramyosin (pmy) is a 97 kDa myofibrillar protein with a coiled-coil structure and is found exclusively in invertebrates. Expressed on adults and the tegumental surface of lung-stage schistosomula, it appears to be multifunctional. It may act as a receptor for Fc, and an exogenous form inhibits activation of the terminal pathway of complement implying an important immunomodulatory role in schistosomiasis (Gobert and McManus, 2005). Native and recombinant pmy (Sj97) confer protection against S. japonicum in mice, buffalo and other mammalian hosts (Wu et al. 2005). Furthermore, recent studies of human T helper2 (Th2) cytokine responses to Sj97 add further support to this molecule as a leading vaccine candidate against S. japonicum (Leenstra et al. 2006). Unfortunately, a major challenge with Sj97 is its poor expression in soluble form, probably due to its coiled coil structure and its large size. To improve its expression and to identify protective epitopic regions on paramyosin, the published Chinese Sj97 cDNA sequence was recently redesigned using Pichia codon usage and divided into four overlapping fragments (fragments 1, 2, 3, 4) of 747, 651, 669 and 678 bp, respectively (Zhang et al. 2006). These gene fragments were synthesized and expressed in Pichia pastoris (fragments 2 and 3) or E. coli (fragments 1 and 4). The recombinant proteins were produced at high level and purified using a two-step process involving Ni-NTA affinity chromatography and gel filtration. BALB/c mice were immunized subcutaneously three times at 2 week intervals with the purified proteins formulated in adjuvant Quil A. The protein fragments were highly immunogenic, inducing high, though variable, ELISA antibody titres, and each was shown to resemble native paramyosin in terms of its recognition by the anti-fragment antibodies in Western blotting. The immunized mice were subjected to cercarial challenge 2 weeks after the final injection and promising protective efficacy in terms of significant reductions in worm burdens, worm-pair numbers and liver eggs in the vaccinated mice resulted. There was no apparent correlation between the antibody titres generated and protective efficacy, as all fragments produced effective but similar levels of protection. These fragments now need to be tested further for protective potency both separately and in combination in larger animals including buffaloes.
Very low density lipoprotein-binding protein (SVLBP)
An expressed sequence tag (EST) of S. japonicum encoding a S. japonicum very low-density lipoprotein binding protein (SVLBP; molecular size, 20 kDa) was reported to be membrane-associated, and located in the tegument and sub-tegument of adult male schistosomes (Fan et al. 2003). Given that the SVLBP may play an essential role in lipid acquisition by the parasite, and/or in signal transduction pathways, its further investigation for development as a novel anti-schistosomal intervention was warranted. Accordingly, Gan et al. (2005) used affinity purified recombinant (r) SVLBP to vaccinate mice. The worm numbers and egg deposition recovered from the livers and veins of the immunized mice were 33·5% and 47·6% less than those from control mice, respectively. There was also a marked increase in the antibody response in vaccinated mice: the titre of IgG1 and IgG2a, IgG2b in the vaccinated group was significantly higher than that in the controls. In a comparison of the reactivity of sera from healthy individuals and patients with rSVLBP, recognition patterns against this parasite tegumental antigen varied among different groups of the individuals. Notably, the average titres of anti-rSVLBP antibody in sera from faecal egg-negative individuals was significantly higher than that in sera from the faecal egg-positives, which may reflect SVLBP-specific protection. These results suggest that the parasite tegumental protein SVLBP is a promising candidate for further investigation as a vaccine antigen for use against Asian schistosomiasis but further testing is now required to assess its true value.
Serine protease inhibitor (Sj serpin)
Serine proteinase inhibitors (serpins) represent an important superfamily of endogenous inhibitors that regulate proteolytic events active in a variety of physiological functions. Yan et al. (2005) used immunological screening of a S. japonicum adult worm cDNA expression library with sera of Microtus fortis, a naturally resistant vertebrate host, and identified one clone that encoded for a sequence homologous to those of the serpin superfamily. The full-length sequence encoding S. japonicum serpin (Sj serpin) was amplified from adult worm cDNA by using 5′-RACE-PCR and subsequently cloned into the prokaryotic expression vector pET28c. The full-length Sj serpin fusion-protein with his-tag was expressed in E. coli, purified by affinity chromatography and used to immunize New Zealand white rabbits. Sj serpin is located on the tegument in S. japonicum adult worms. C57BL/6 mice immunized with Sj serpin induced the production of high levels of specific IgE and IgG1 subclass antibodies as well as a marked IL-4 response. Lymphocyte surface marker analysis revealed proliferation of CD19-expressing B cells, indicating a predominant Th2-type response to Sj serpin. Immunized mice developed moderate protection against infection of S. japonicum as demonstrated by a 36 and 39% reduction in the recovery of adult worms and eggs, respectively. Further vaccine-challenge experiments with Sj serpin, modifying the delivery of the vaccine and testing different adjuvant formulations, will enable a better assessment of its potential as a vaccine candidate.
Triose-phosphate isomerase (SjTPI)
The glycolytic pathway enzyme triose-phosphate isomerase (TPI) is found in each cell of each stage of the schistosome life cycle and the S. mansoni enzyme (SmTPI) has long been targeted as a schistosomiasis vaccine candidate. As the two schistosome TPI sequences are very similar (84% identity), it was logical to assess the protective efficacy of SjTPI both as a recombinant protein and plasmid DNA vaccine. Encouraging results were obtained in early experiments on mice but to examine the transmission blocking potential in large animals, Zhu et al. (2004) determined the vaccine efficacy of Chinese SjTPI (SjCTPI) plasmid DNA in naive pigs. Pigs were vaccinated with the TPI DNA-plasmid alone, or in conjunction with IL-12 as pcDNA3.1-P35, pcDNA3.1-P40 plasmids via intramuscular injection. Control pigs were immunized with equivalent amounts of pcDNA3.1. Pigs were immunized 3 times at 21-day intervals and challenged 30 days after the final boost. Forty-five days post-challenge, pigs were sacrificed and perfused to compare adult worm burdens, female worm burdens, liver egg burdens and granuloma size. They found that pigs vaccinated with SjCTPI DNA alone had adult worm burdens reduced by 48·3% and that a further decrease in adult worm burdens was not seen in the group vaccinated with SjCTPI DNA in conjunction with IL-12 (46·2% reduction). The SjCTPI DNA vaccines had a more pronounced effect on reducing female worm burdens i.e. 53·6% SjCTPI alone and 59·6% for SjCTPI+IL-12. Vaccination with SjCTPI-DNA reduced liver eggs by 49·4% and this response was significantly enhanced by the addition of IL-12 (65·8% reduction in liver eggs). In addition to the dramatic protective effects seen in vaccinated pigs, they also noted that granuloma size was reduced by 42% in both groups. Though encouraging, further extensive experimental and field-based trials on bovines, particularly buffaloes, are now required in China to determine whether vaccination with the SjCTPI DNA vaccine will likely reduce transmission by reducing adult worm burdens and worm egg output, with simultaneous reduction of hepatic egg-associated pathology.
23 kDa integral membrane protein (Sj23)
As with TPI, the schistosome integral membrane protein (Sm/Sj23) was identified as a major vaccine candidate some years ago, first against schistosomiasis mansoni and then schistosomiasis japonica. The S. japonicum Chinese strain form (SjC23) was initially shown to induce protection in mice as a synthetic peptide vaccine and then as a plasmid DNA vaccine to induce protection in mice, sheep, pigs and water buffalo. Overall, the results from the extensive plasmid DNA vaccine studies indicated that vaccination with SjC23 DNA induced not only a significant reduction in worm and egg burdens, but also significantly reduced the size of egg granulomas; thus, like SjCTPI, SjC23 produced an anti-pathology effect as well. The protective effect of the SjC23 plasmid DNA vaccine was enhanced with IL-12 in pigs (Zhu et al. 2004) and mice (Gan et al. 2005), and by CpG immunostimulatory sequence in mice (Zhao et al. 2005). As with the other candidate vaccines, extensive large animal field trials are now required to determine the precise protective potency of SjC23 with or without IL-12 or CpG.
Fatty acid-binding protein (SjFABP)
Like other parasitic helminths, schistosomes are unable to synthesise long chain fatty acids or sterols and hence are completely dependent on the host for these components. Fatty acid binding proteins (FABPs) are critical for schistosomes to take up fatty acids from host blood as essential nutrients, and are thus prime targets for both vaccination and drug development. The 14 kDa FABP of Chinese strain S. japonicum (SjFABPc) has at least eight different variants encoded by a single-copy polymorphic gene, and it is particularly important to S. japonicum for uptake, transport and compartmentalisatioin of host-derived fatty acids, playing a vital role in the physiology and survival of the parasite. Several Chinese groups have obtained encouraging protection with (SjFABPc) in mice both as a recombinant protein and plasmid DNA vaccine. Especially noteworthy are the studies by Liu et al. (2004) who expressed SjFABPc in Escherichia coli and in Baculovirus/silkworm systems. The recombinant protein from E. coli was a 41 kDa GST fusion protein (rSj14/GST), which could be purified by glutathione agarose affinity chromatography, with a yield of 25 mg/L E. coli culture. The recombinant protein from the Baculovirus/silkworm system was an 18 kDa fusion protein (rSj14/His), which could be purified by Ni-NTA resin chromatography column with a yield of 3·5 mg per silkworm larva. Both rSj14/GST and rSj14/His were recognized by S. japonicum-infected mouse sera and anti-rSj14/GST mouse sera in Western blotting. The purified recombinant protein was immunogenic in mice, rats and sheep, and 34·3%, 31·9% and 59·2% worm reductions, respectively, were obtained in vaccinated Kunming mice, Wistar rats and sheep vaccinated with Sj14/GST, compared to non-vaccinated control groups. Worm reductions of 48·8% and 49·0% were recorded in BALB/c mice immunized with Sj14/His, compared to non-vaccinated and BCG-vaccinated groups, respectively. Taken together, these results emphasise the promise of SjFABPc as a candidate vaccine for schistosomiasis japonica, particularly as in the rat and sheep vaccination experiments, no adjuvant was used.
Another group (Zhu et al. 2005) studied the protective efficacy of SjFABPc as a DNA vaccine enhanced by IL-12 in mice challenged with S. japonicum. They showed that IL-12 drives the immune response toward a Th1 direction, and enhanced the protective effect of the vaccine.
VACCINES AGAINST FASCIOLA HEPATICA AND F. GIGANTICA
While certainly not responsible for as great a toll on human mortality and morbidity as schistosomiasis, fasciolosis is more important in terms of economic loss to the global agricultural community. This loss is estimated at >$2000 million annually, as >600 million animals are infected (Hillyer and Apt, 1997; Spithill et al. 1999b). Like schistosomes, liver flukes are digenetic trematodes and therefore display similar life cycles. However, infection is acquired following ingestion of the infective larvae that lie dormant within cysts rather than by penetration of the skin by free-swimming larvae. Depending on the climate, these cysts can remain viable in the environment for up to one year. Following excystment in the intestine of the host the parasite burrows through the intestinal wall, migrates through the liver parenchyma for approximately two months before moving into and taking up residence in the bile ducts. These early migratory stages cause extensive perforations and haemorrhaging of the liver which can result in death in cases of heavy infection, particularly in susceptible species such as sheep. Chronic disease can persist for several years in cattle and many years (as long as 20) in sheep. Within the bile ducts mature hermaphroditic flukes liberate up to 20–50000 eggs per day per parasite that are carried with the bile juices into the intestine and are passed onto the pastures in the faeces (Whitfield, 1979; Andrews, 1999).
Fasciolosis is caused by two species of liver fluke, Fasciola hepatica and Fasciola gigantica. The main intermediate host for F. hepatica in Europe is Galba truncatula but other varieties such as L. tomentosa are more important in Australia and New Zealand and Pseudosuccinia columella in South America. The intermediate hosts of F. gigantica, the Eurasian Radix auricularia and African R. natalensis, are resistant to infection with F. hepatica and, conversely, F. gigantica does not appear to develop in Galba truncatula (Graczyk and Fried, 1999; Mas-Coma, Barques and Valero, 2005).
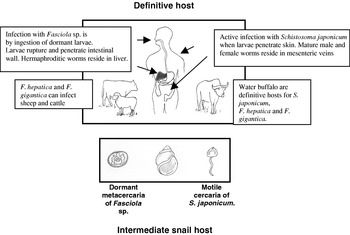
Fig. 1. The life cycles of the zoonotic trematodes S. japonicum, Fasciola hepatica and Fasciola gigantica have certain similarities; the figure above highlights some important differences. The parasites use similar molecules (proteases, anti-oxidants etc.) in key aspects in host-parasite interaction. It is not surprising, therefore, that similar strategies for vaccine discovery for the prevention and transmission of infection have been employed by researchers and, consequently, that many of the lead vaccine candidates are homologs in these parasites. Figure drawn by JPD.
Although believed to be of European origin, due to the capacity of F. hepatica to infect many different species and to the ability of the intermediate snail host to adapt to a wide range of ecological niches, the parasite has now a cosmopolitan distribution (Mas-Coma et al. 2005). Fasciolosis is still generally associated with more temperate climates and besides being highly prevalent throughout Europe, it is now found in the southern states of the US, Mexico, North Africa, New Zealand and small regions within Australia. The parasite infects primarily cattle and sheep, although other domesticated animals such as goats, horses and pigs can be infected. Other hosts include llamas in South America, camels in Africa and marsupials in Australia which may play important roles as reservoir hosts in these regions. The introduction of F. hepatica to South America by Europeans since the 18th Century has seen it become a major problem in Brazil, Argentina, Uruguay and in several Andean countries such as Bolivia, Peru and Equador (Mas-Coma et al. 2005).
In Europe and Australia where chemical products are used as the almost sole means of disease control parasites resistant to the major anti-flukicide, triclabendazole (FasinexR, Novartis Animal Health), have emerged. Problems of drug-resistance will increase as this drug has recently come off patent protection and various generics are currently being sold. Some less effective flukicide products are still available (e.g. clorsulon) and new mixtures of old products have being recently marketed, but the likelihood of novel chemical products being developed is small given the heightened regulations and costs associated with drug research and development. Thus, the future control of fasciolosis hepatica will depend on the development of an effective vaccine.
Disease caused by F. gigantica, a larger but more slender fluke, has a relatively restricted distribution due to the reduced ability of the intermediate hosts to invade new niches and is generally found in tropical regions, being recorded in Africa, South and East Asia, the Middle East and eastern Europe. This parasite has been largely neglected even though, as highlighted by Spithill et al. (1999b), it is regarded as one of the most important single helminth infections of ruminants in Asia and Africa. Since the disease is more prevalent in less well-off communities it impacts significantly on local or family economies. For example, in some regions of Indonesia the prevalence in some areas can reach 90% and annual losses to the country as a whole are in excess of US$100 million. Similar to infection with F. hepatica, this disease results in lower weight gains, reduced milk production, poorer quality of meat, reduced fertility and reduce work capacity. The parasite infects mainly cattle and sheep but in Asia infection of water buffalo, the main source of draught power, is of greater significance.
The distribution of F. hepatica and F. gigantica overlap in various regions in the Far East where fasciolosis is important, including Japan, Korea, Taiwan and the Phillipines. Significantly, this has given rise to mixed parasite forms, seemingly by natural hybridisation (Agatsuma et al. 2000), with morphological and genetic features that range in the two extremes; for example, forms often referred to as Fasciola spp. in Japan have mtDNA NDI and COI sequences in common with F. gigantica and ITS-2 sequences of nuclear rDNA identical with either F. gigantica or F. hepatica (Itagaki and Tsutsimi, 1998).
HUMAN FASCIOLOSIS – A RE-EMERGING PROBLEM
Since infection is acquired through ingesting infective larvae fasciolosis in humans is considered a food-borne zoonotic disease. However, the importance of human fasciolosis has only been recognised in the last decade. Prior to 1992 total reported cases of human fasciolosis was estimated to be <3000 but more recent studies provide figures of 2·4 million (Rim et al. 1994; Curtale, Hassanein and Savioli, 2005) and 17 million (Hopkins, 1992). While the actual situation remains unclear it is certain that, due to the increased number of surveys carried out with better diagnostic assays, human fasciolosis is highly prevalent in certain regions of the world. Human infection is always associated with local endemic animal fasciolosis, although the distribution of human infection and level of prevalance may not always correlate with that observed in animals (Mas-Coma, 2004; Mas-Coma et al. 1999a, 2005). Moreover, parasites in humans produce viable eggs and thus play a part in the overal transmission of diseases (Mas-Coma et al. 2005).
After initial reports of human infection in Bolivia (Hillyer et al. 1992; Bjorland et al. 1995) several major epidemiological studies revealed that human infection is highly prevalent (100% in some areas) in the high altitude Andean rural regions stretching from northern Bolivia, through Peru, to Equador (Mas-Coma et al. 1999a,b; O'Neill et al. 1998, 1999a). Infection is found in mainly indigenous peoples, subsistance farmers who regularly share the same water sources with their animals. Infection is more prevalant in children but is present in all ages of both sexes (Mas-Coma et al. 2005). Since the parasites can live in humans for up to 13 years and produce large numbers of eggs (up to 5000 eggs per gram faeces in some Bolivian children) that are infective to other hosts, humans may play a significant part in disease transmission (Mas-Coma et al. 1999b), as observed for schistosomiasis japonicum in China (Williams et al. 2002; Guo et al. 2006; Ishikawa et al. 2006).
Human infection is also prevalent, with regular outbreaks involving up to 10000 infections, in the Guilan and Mazandaran provinces of northern Iran that border the southern end of the Caspian Sea (Rokni et al. 2002; Moghaddam et al. 2004). Esteban et al. (2003) recently reported hyperendemic human disease in the Nile Delta region between Cairo and Alexandria in Egypt (ranging in prevalence from 5 to 19%) and estimate that as many as 830000 individuals are infected here. Schistosomiasis mansoni is also endemic in this region (more than double that of fasciolosis) and the relationship of its prevalence and intensity with sex and age showed patterns similar to that found with fasciolosis. The intermediate hosts of both parasites have penetrated the irrigation streams and, therefore, control programmes here will need to consider both diseases (Esteban et al. 2003; Curtale et al. 2005). Immunosupression induced by liver flukes (and schistosomes) may contribute to the susceptibility of humans to other infections as multiparasitism, particularly with other food-borne pathogens such as Giardia intestinalis, has been observed in surveys in Egypt and South America (Brady et al. 1999; Mas-Coma et al. 2005).
Human infection in Europe occurs more sporadically, but countries such as France, Spain and Portugal are experiencing significant and consistent cases of infections, approximately 50 to 100 per year (Mas-Coma et al. 2005). Infection is usually due to the ingestion of various freshwater aquatic plants, such as watercress, on which the metaceracariae have settled. Farm management practices and the culturing of edible aquatic plants in geenhouses has limited the extent of human infection in more industrialised regions but in South America, Egypt and Iran wild aquatic plants or plants grown in fields where infected animals can freely roam form a regular part of the daily diet. Since these are often sold at local or distant food markets outbreaks of human infection, such as that experienced in the town of Rasht in northern Iran (Rokni et al. 2002), can occur far from the site of vegetable contamination with metacercariae. Metacercariae can also be found floating in water and, therefore, infection can be acquired through drinking water, even from piped sources (Mas-Coma, 2004).
Most human cases are with F. hepatica, with a few reports of F. gigantica infection in the tropics (see Mas-Coma et al. 1999a). Several recent reports are indicating a rising problem with fasciolosis in Vietnam, although the complete picture remains unclear (Hien et al. 2001). It is likely, however, that a detailed survey of rural liver fluke-endemic regions in the Asia would reveal greater human infection since the lack of farm management, the sharing of water sources by animals and humans and the likelihood of contamination of edible aquatic plants and rice with infective fluke metacercariae is similar to that observed in other regions where fasciolosis is an important zoonosis.
Theoretically, human fasciolosis could be controlled by introducing strict farm management practices, prevention of food contamination and a change of eating habits but, for obvious reasons, this is not practical in areas where disease in prevalent. However, two recent advances will be important for the future detection and control of human disease (in both edemic and non-endemic regions); firstly, a cheap sensitive and specific immunodiagnotic ELISA test that detects antibodies in human serum or plasma (or in blood taken onto filter paper) has been developed (O'Neill et al. 1998; Strauss et al. 1999; Rokni et al. 2002) and second, the flukicide triclabendazole has been formulated into tablets for human use (EgatenR, Novartis) and is highly effective (80%) against both F. hepatica and F. gigantica infection when given in dose of 10 mg per kilogram and almost 100% effective when given in two doses (Curtale et al. 2003, 2005; Savioli, Chitsulo and Montresor, 1999).
PROGRESS TOWARDS VACCINE DEVELOPMENT
The development of vaccines for controlling animal and human fasciolosis is of critical importance. The lack of protection against the spread of drug-resistant F. hepatica in the major agricultural economies will be the main force that will drive this development and its commercialisation. Moreover, there appears to be a increase in animal fasciolosis in Europe, possibly due to weather changes (Fairweather and Boray, 1999). Support from the EU Commission under the Sixth Framework Programme (FP6) for a new major global project (DELIVER) that will focus on the control of fasciolosis and the understanding of drug resistance is an acknowledgement of the growing importance of this disease and should give research in this area a major boost (Jose Perez, University of Cordoba, Cadiz, Spain, personal communication). Additionally, there has been a noticeable renewed interest in fasciolosis amongst the major animal health multinationals and now that the costs and legislation associated with new drug development have become more onerous the notion of exploiting recombinant vaccines is becoming a realisitic option from a commercial point of view.
Substantial strides have taken place in our understanding of the molecular biology of F. hepatica and F. gigantica and there is now a rich source of more than 4000 expressed sequences tags (EST) (www.sanger.ac.uk/Projects/S_mansoni/) from which vaccine candidates can be fished using random or rational criteria. The diffculty in employing high-throughput techniques for identifying putative vaccine candidates, such as signal sequence trap that identifies secreted or membrane-bound antigens (see Dalton et al. 2003a), is the lack of consensus on a biological property or molecular characteristic that is predictive of vaccine potential. Additionally, there is no suitable in vitro assay or even laboratory model that lends itself to testing large numbers of vaccines. Accordingly, most of our present vaccine candidates for fasciolosis have been known for some time and were discovered using basic biochemical and/or immunological methods for investigating parasite excretory-secretory (ES) products or by systematically analysing chromatographically separated fractions of parasite extracts that provide protection in experimental models. These candidates have been reveiwed in a excellent recent paper by Hillyer (2005) so in the present report we will summarise these in terms of their phase of development.
CANDIDATES PROGRESSED TO RUMINANT VACCINE TRIALS
Fatty acid-binding proteins
The potential of using immunologically cross-reacting antigens to target both F. hepatica and S. mansoni was pursued by Hillyer and colleagues in a series of vaccine trials in mice since the early 1970s (see Hillyer, 2005) and led to the discovery of the fatty acid binding proteins (FABP). FABPs are major components of whole fluke extracts and are also found in ES products. They represent a heterogeneous family of proteins as revealed by 2-D electrophoresis (Jefferies et al. 2001) and several different genes are deposited in the public database. FABP function in the transport of hydrophobic ligands and since trematodes cannot synthesis long chain fatty acids de novo the parasite may use FABPs to sequester these from host serum (Spithill, Smooker and Copeman, 1999a).
One of the native FABP forms of 12 kDa (nFh12) was selected from a gel permeation fraction of adult liver flukes for their immunoprophylactic potential against schistosomes and liver flukes following extensive vaccine studies in mice (see Hillyer, 2005). The recombinant counterpart (rFh15) of this molecule has given impressive vaccine results in rabbits against a heterologous challenge with F. hepatica; levels of protection of 40%, 43% and 76% were observed in various experiments which varied the time between vaccination and challenge. Protection was positively correlated with longer periods between vaccination and challenge which may be important in sufficient high titres of specific high-affinity antibody. The vaccine also had similar large reductive effects on fluke size, egg production (anti-fecundity) and pathology (Muro et al. 1997; Casanueva et al. 2001). In subsequent vaccination trials in sheep (Castillian breeds in Spain) using nFh12 and rFh15 similar high levels of protection were not observed but the results highlighted the importance of the adjuvant in which the vaccine was formulated (Ramajo et al. 2001; Martinez-Fernandez et al. 2004). For example, when formulated in Freund's adjuvant no reduction in fluke burden or development was seen although effects on faecal eggs counts were significant. However, when the native molecule was formulated in saponin from Quillaja saponaria or a hydroalcoholic extracts of Polypodium leucotomos and emulsified in a non-mineral oil (Montanide), fluke recoveries in vaccinated sheep where significantly lower (23·5%), fluke development was retarded (by approximately 35%) and egg counts in bile and faeces were reduced by 58·1% and 40·3%, respectively.
A 12 kDa protein from F. hepatica which showed sequence homology to FABP but was different to rFh15 was described by Bozas and Spithill (1996). The native molecule, termed FABP3, was isolated by reverse phase HPLC and tested in cattle, but no protection was observed. The negative result could be contributed to the destruction of protective epitopes by denaturing conditions used during purification, and it is possible that the adjuvant used (DEAE) was not appropriate to induce the required immunological responses (Spithill et al. 1999b).
Recent studies in mice by Lopez-Aban et al. (1999, 2000) have shown the potential of nFh12 and rFh15 to protect against S. bovis in mice (as high as 96%), albeit this protection was strain dependent. Tendler and colleagues (Tendler et al. 1996) characterized a 14·8 kDa S. mansoni FABP (Sm14) which, in recombinant form, could induce protection in mice to both S. mansoni and F. hepatica (Almeida et al. 2003). The schistosome molecule is homologous to rFh15 and certain peptides (EKNSESKLTQ and VTVGDVTA) that match sequences in both molecules exhibit protective property similar to that of recombinant Sm14 (Vilar et al. 2003). Sm14 was also reported to protect sheep from a challenge infection of F. hepatica and, while the numbers of animals used in this single trial were low, the effects on fluke burdens were very high (59·2%) (Almeida et al. 2003).
Cysteine peptidases
The most abundant proteins secreted by adult liver flukes are cathepsin L cysteine peptidases (approximately 24·5 kDa). These belong to a large heterogenous family encoded by at least seven different genes (Tort et al. 1999; Dalton et al. 2003a). They are synthesised and secreted by the epithelial cells lining the parasite gut and function in the degradation of the blood meal in the gut lumen (Collins et al. 2004). Since the contents of the gut are emptied approximately every three hours, these peptidases are also delivered to the exterior in large quantities where they perform additional functions such as facilitating penetration of host tissue (intestinal wall, liver parenchyma and bile duct) by the migrating stages of the parasites and preventing host immune attack by cleaving immunoglobulins and suppressing the development of protective Th1 responses (Dalton and Mulcahy, 2001; Dalton et al. 2003b).
Two cathepsin L peptidases, cathepsin L1 and cathepsin L2, have been the most extensively studied vaccine candidates and trials have been carried out in both sheep and cattle. These vaccines have given consistent protection in cattle that ranges from 38·2%–68·5%. A cocktail of the two enzymes has never been tested in cattle but when cathepsin L1 and cathepsin L2 were combined with another antigen, a fluke haemoglobin, protection levels of 51·9% and 72·4% were obtained, respectively (Dalton et al. 1996, 2003b). In all of these trials a large reduction in egg production and egg viability was observed in the the peptidase-vaccinated animals; the anti-fecundity effect observed using cathepsin peptidases ranged from 40% to 65% while the combination of these with haemoglobin ranged from 80% to 98%.
Both cathepsin L1 and cathepsin L2 vaccines also induced significant protection in Uruguayan sheep breeds (34% and 33% lower fluke burdens, respectively), and elicited strong anti-fecundity effects (71 and 81%, respectively). A combination of the two peptidases showed that a cocktail can enhance protection to 60% (anti-fecundity effects were not measured) (Piacenza et al. 1999). In contrast, vaccination of Australian Merino sheep with a preparation of adult fluke cysteine peptidases (possibly included both cathepsin L1 and cathepsin L2) did not elicit a protective response, although a large, 69·4%, reduction in egg output was observed (Spithill et al. 1999a).
The above results have been obtained using native cathepsin L peptidase. Recently, Collins et al. (2004) described the production of recombinant cathepsin Ls in the methylotrophic yeast, Pichia pastoris, that exhibit identical enzymatic and molecular characteristics to the native peptidases. These molecules are currently under investigation in vaccination trials in sheep and recently data using cathepsin L1 revealed that this recombinant delivers protection levels (35–45%) and anti-fecundity effects (50%) that are similar to those observed with the native molecules (Dalton and Mulcahy, unpublished).
Leucine aminopeptidase
Leucine aminopeptidase is a 54 kDa metallo-exopeptidase also secreted from the gut of liver flukes; its primary role is likely in food digestion (Acosta, Goni and Carmona, 1998; Piacenza et al. 1999). When used together with cathepsin L1 and cathepsin L2 the levels of protection were increased over the cathepsin L peptidases alone (see above) and reached 79%. However, and perhaps surprisingly, when the leucine aminopeptidase was used as a vaccine alone it induced even higher levels of protection (89·6%). Recently, Carmona (2005) reported the production of a recombinant protein expressed in Escherichia coli that induced a statistically significant 81% protection in rabbits compared to adjuvant control.
Glutathione-S-transferase
Once the focus of intense vaccine research with substantial commercial backing, the family of glutathione-S-transferase (GST) isoenzymes have now been rather neglected. The original rationale for choosing GST as vaccines against F. hepatica was their demonstrated efficacy as vaccines against S. haematobium. Additionally, the enzymes are secreted by liver flukes and may play important chemical neutralisation functions outside the parasite as well as household intracellular detoxification roles (Spithill et al. 1999a,b). The inconsistent efficacy of these enzymes in both sheep and cattle trials (even in direct repeat trials) has reduced optimism in their use as vaccines. The native vaccine was tested in a range of adjuvants with an overall average protection level of 29% (lowest 0% and highest 65%) in sheep and 45% (lowest 19% and highest 69%) in cattle (Dalton and Spithill, 1998; Spithill et al. 1999a). In most experiments, significant reduction in faecal eggs counts was observed (up to 65%), but this effect was also highly variable. The most effective adjuvant in cattle, QuilA/Squalene Montanide, was not effective in sheep suggesting that each species may require distinct vaccine formulations. Vaccination of cattle with a S. bovis-derived GST formulated in QuilA, Alum and Freund's adjuvants did not induce protection in cattle challenged with metacercaraie of F. hepatica (De Bont et al. 2003).
CANDIDATES IN THE PIPELINE
A high molecular sized fraction which contained fluke haemoglobin enhanced the efficacy of vaccines containing cathepsin L1 or cathepsin L2 (see above) and alone induced 43·8% protection in cattle (Dalton et al. 1996). While haemoglobin was the major component of this protective fraction (McGonigle and Dalton, 1995) immunological screening of cDNA libraries with the sera taken from protected cattle prior to challenge led to the isolation of a cDNA encoding a novel anti-oxidant termed thiol specific anti-oxidant (now termed thioredoxin peroxidase or peroxiredoxin) (McGonigle et al. 1997, 1998). Thioredoxin peroxidase (TPx) is secreted in low amounts by the parasites and while not immunogenic in naturally infected animals it is highly antigenic when used as a vaccine in Freund's and QuilA adjuvants. Recent data revealed that TPx is involved in subverting the immune response of the host by stimulating the recruitment and activation of alternatively activated macrophages and thus offers the possiblity of developing a vaccine that intervenes in the host-parasite immunological relationship (Donnelly et al. 2005). Recombinant TPx with functional anti-oxidant activity has been produced in E. coli and is currently under trials as a vaccine against fasciolosis in sheep (Dalton and Mulcahy, unpublished).
A F. hepatica saposin-like or NK-lysin-like molecule that may be involved in lysing host erythrocytes as they enter the parasite gut was isolated using sera taken from rabbits four weeks after an experimental infection (Reed et al. 2000; Espino and Hillyer, 2003). The recombinant form of the protein, FhSAP-2, induced an impressive 81·2% protection against worms in rabbits, a reduction in faecal and gall bladder egg counts of 83·8% and 73%, respectively, and concomitant alleviation of liver pathology (Espino and Hillyer, 2003, 2004; Espino et al. 2005).
A different isotype of cathepsin L (termed cathepsin L3; Harmsen et al. 2004) and cathepsin L DNA vaccine contructs (Kofta et al. 2000; Wedrychowicz et al. 2003) have been tested in rats and elicited >90% and 61–75% protection, respectively. Additional molecules that are being characterized from F. hepatica with vaccine potential include cathepsin B cysteine proteases (Law et al. 2003; Meemon et al. 2004), thioredoxin reductase (Maggioli et al. 2004) and enolase (Bernal et al. 2004).
CANDIDATE VACCINES FOR F. GIGANTICA
The development of vaccines for F. gigantica depends, unfortunately, on the transfer of know-how and technology from research performed on F. hepatica. Studies on water buffalo, the main source of income for many in Asia, have not been reported mainly because animals are expensive and trials are logistically difficult to organise. One report of a major vaccine trial in Braham-cross cattle using native and recombinant FABP (rFh12), native GST, native cathepsin L and native paramyosin was published over eight years ago (Estuningsih et al. 1997). In this trial, only native FABP appeared to induce protection (31%) but this was with low significant values (P<0·026). A recent trial also did not observe protection in sheep using native F. gigantica GST formulated in saponin or alum hydroxide (Paykari, Dalimi and Madani, 2002).
DISEASE TRANSMISSION – BLOCKING EFFECT OF LIVER FLUKE VACCINES
A common feature of all the vaccines described above is that concurrent with protection against parasites a reduction of faecal eggs counts was also induced. While it would seem obvious that a reduction of parasite numbers would have a corresponding knock-on effect on the number of eggs produced and released onto pastures, in many cases the effects on egg production is greater than the effect on worm burden; for example, vaccination with FABP and cathepsin L proteases (see above). In some cases, negative effects on egg production have been observed with little or no concurrent protection against flukes; for example, vaccination of sheep with native cathepsin L proteases (Spithill et al. 1999a,b). The results indicate that egg production is particularly vulnerable to immunological responses generated by vaccination, which is not so surprising given that most of the catabolic (feeding and breakdown of nutrient) and biosynthetic (synthesis of egg and eggshell components) energy of mature adult flukes is devoted to this process. Accordingly, it is easy to understand that blocking of the uptake of host fatty acids by anti-FABP antibodies or the digestion blood components by anti-cathepsin L and anti-leucine aminopeptidases would have a immediate negative impact on the parasites' ability to synthesis viable eggs. Interestingly, immunological responses induced by vaccination with GST appear to have no consistent correlation with blocking of egg production (Dalton and Spithill, 1998; Spithill et al. 1999b).
Presently, there is no means of knowing how much impact a vaccine that reduces egg output would have on the transmission of fasciolosis, particularly since adult flukes produce an estimate 20–50000 eggs per hour and that infection of the intermediate snail host with a single miracidium gives rise to approximately 600 infective metacercariae (Whitfield, 1979). Mathematical models, similar to those developed for schistosomiasis japonicum (Williams et al. 2002; Guo et al. 2006; Ishikawa et al. 2006), have not yet been reported for fasciolosis but are needed to predict the impact of vaccines on disease transmission. If we can draw an analogy with the study of Williams et al. (2002) it is probable that a vaccine delivering a 75% anti-fecundity affect would ensure mid-term reduction of parasite transmission and long-term elimination. Dalton et al. (1996) showed that vaccination of cattle with a combination of cathepsin L2 and haemoglobin resulted in a 98% reduction in egg output, and that the majority of the eggs produced did not embyonate. This study thus demonstrated that it is theoretically possible to develop a highly efficacious vaccine that could also prevent the contamination of pastures with viable fluke eggs and thus have a profound blocking effect on disease transmission.
IMMUNE MECHANISMS OF VACCINE PROTECTION AGAINST LIVER FLUKES
Although quite a number of vaccine trials have been carried out by various research groups, no consensus on the factors required for immunological protection has emerged. Most immunological analysis has focused on antibody isotypic responses due to the earlier lack of reagents for cytokine analysis and, hence, protective cellular components can only be inferred. Recent advances in the analysis of ruminant cytokines should provide the tools to generate more informative data on the immunological phenotype in naturally-infected and vaccinated animals (for example see Hein et al. 2004) which will be critical to the design and implementation of future vaccine formulations.
Neither sheep nor cattle develop strong immunity to infection with F. hepatica or to subsequent re-infections (Mulcahy, Joyce and Dalton et al. 1999; Hoyle et al. 2003). Moreover, unlike for other helminth infections such as schistosomes, hookworms and lungworms, vaccines using irradiation-attenuated larvae do not induce protective immune responses (irradiated metacercariae of F. hepatica can survive in the host for up to 28 days but do not develop beyond 10 day-old immature flukes.) The studies of Piedrafita et al. (2000, 2001) suggest that the lack of resistance in ruminants is related to the inability of their macrophages to produce nitric oxide. Peritoneal lavage cells (mainly macrophages) obtained from rats, which exhibit high levels of resistance to fluke re-infection, can bind to antibody-coated newly excysted juveniles (NEJ) in vitro and induce killing by producing high levels of reactive nitrogen species. In contrast, macrophages of Merino and Indonesion Thin Tailed (ITT) sheep did not produce reactive nitrogen species and failed to kill antibody-coated NEJ, regardless of whether the source of antibody was infected rats or sheep.
Collective data from studies in ruminants indicate that liver flukes elicit immune responses involving the typical hallmarks of the Th2-type, namely eosinophilia, IgE and the production of IgG1 antibody isotypes (Mulcahy et al. 1999; Estes and Brown, 2002). The current general belief is that helminths can withstand the onslaught of components of the Th2 arm of the host immune response and that these responses cause pathological damage to the host that is tolerated over a long and sustained period (Pearce and MacDonald, 2002). Migrating and adult F. hepatica not only secrete molecules that induce Th2 responses but also others, including cathepsin L proteases, that appear to actively down-play Th1 responses (O'Neill et al. 1999b, 2001). Once the flukes reach the bile ducts of their host, a site that is considered to be immunologically safe, they can survive for many years. However, being blood feeders, they still have contact with the circulation and secrete antigens from their guts that may be involved in maintaining the Th2-polarised immune response during chronic fasciolosis (Dalton et al. 2003b).
A consistent feature observed in vaccine trials with cathepsin L proteases is the correlation between antibody isotypic responses and protection. Naturally-infected animals elicit high IgG1 antibody titres, which are controlled by the Th2 cytokine IL4, but little or no IgG2. In contrast, vaccinated animals elicited both IgG1 and IgG2. Moreover, in trials involving both cattle and sheep, the titre of IgG2 antibodies generated directly correlated with the fluke burden of animals within vaccinated groups i.e. those animals with the lowest liver fluke numbers exhibited the highest IgG2 levels. Adjuvant such a Freunds' incomplete adjuvant which do not induce high titres of these antibodies do not induce significant protection (Mulcahy et al. 1998, 1999; Dalton et al. 2003b). The interpretation is that cathepsin L vaccines induce Th1 or mixed Th1/Th2 responses with mixed antibody isotypes that bind and inactivate the parasite enzymes and hence block parasite migration, feeding and the abilty to skew the host immune response to a fully-polarised Th2-type. The level of enzyme inactivation, therefore, depends on the total antibody titre induced by the vaccines but in particular IgG2. IgG2 antibodies may be involved in direct enzyme inactivation but may be more detrimental to the parasites since these are also efficient activators of complement and phagocytosis by macrophages (Corbeil, 2002; Crawley and Wilkie, 2003). While sheep macrophages do not produce nitric oxide following binding to sera from infected animals (largely IgG1) (Piedrafita et al. 2001) they may become activated in the presence of IgG2. Other effector cells such as eosinophils and neutrophils may contribute to the killing process of already damaged flukes. Although no difference in the level of eosinophilia between protected and cathepsin L-vaccinated animals is observed, a protective role for these cells cannot be excluded since their activation status was not examined. Meeusen and colleagues have proposed that ‘activated’ eosinophils are responsible for the elimination of infective Haemonchus contortus larvae in protected sheep (Meeusen and Balic, 2000).
Isotypic analyses were not reported for the FABP and leucine aminopeptidases and immunological correlates of protection are therefore still unknown. In the latter case, enzyme-inhibitory antibodies have been implicated in the protective responses and killing may be thus solely due to blocking of nutrient uptake. GST vaccines formulated in Freund's Complete Adjuvant induced both IgG1 and IgG2 isotypes but correlations between the reactivity of these antibodies to native GST and protection were not reported. Responses to overlapping peptides representing linear epitopes were analysed but revealed no correlation with protection (Spithill et al. 1999a). Since GST formulated in QuilA/SM gave the most consistent, albeit variable, protection in cattle it would be worthwhile investigating the type of responses generated with this adjuvant.
Lessons we learn regarding immunological responses following natural infection and vaccination with F. hepatica cannot be applied directly to F. gigantica. Merino breeds are susceptible to infection with F. gigantica whereas ITT show high levels of resistance. In this case, susceptibility to infection in Merino sheep was positively correlated with the induction of IgG2 isotypes, the opposite to that observed in F. hepatica infections of sheep. Correlates of protection in ITT are Th2 markers including higher levels of eosinophils, IgM, IgG1 and IgE (see Meeusen and Piedrafita, 2003) and suggest that F. hepatica and F. gigantica have different immunomodulation and immunoevasion strategies. Moreover, biochemical analyses show that the parasites express different levels of anti-oxidants, such as glutathione-S transferase/peroxidase, that may protect them to different levels from reactive oxygen intermediates released by host immune effector cells, particularly macrophages (Meeusen and Piedrafita, 2003). Zhang et al. (2004a,b, 2006) showed that Bellilois male sheep are more susceptible to infection with F. hepatica compared to F. gigantica (infection rates of 18·7% and 5·4% following an infection dose of 250 metacariae of each parasite); they concluded from their immunological analysis that humoral and eosinophil/cell mediated responses to F. gigantica were greater and, hence, more protective. The take-home message for vaccine developers is that F. gigantica homologues of antigens with protective properties against F. hepatica may not neccessarily protect against F. gigantica or, at the very least, will require presentation in a different adjuvant formulation or administration regime.
HOW DO WE GO FORWARD WITH LIVER FLUKE VACCINES?
The spread of fasciolosis in Europe, the appearance of triclabendazole-resistant liver flukes and its emergence as an important zoonosis of humans have led to an increased interest in this disease. Interest is not confined to academia but is also developing in the animal health industrial sector, not only because of tighter regulations and costs surrounding drug development and commercialisation, but also because advances in recombinant protein technology has meant that, in terms of cost of production, vaccines can now compete with drugs. However, both the academic and the industrial community need to be convinced that vaccines can reach an efficacy that is ‘commercially-viable’ i.e. can provide a obvious benefit to farmers who have now become used to, and even dependent on, highly effective chemical products. As it presently stands, the data derived from the few antigens described in this review show some promise but are still at an early-stage level.
The cost and logistics associated with performing vaccine trials in ruminants has been a major obstacle for most research teams and, hence, the majority of vaccine data has been derived from laboratory models such as rabbits, rats and mice. Laboratory models may be useful for initial vaccine screening purposes but results obtained have to be treated with some caution since each of these models: (1) Show different levels of natural resistance; (2) Use different mediators/mechanisms for resistance; (3) May resist infection at different immunological compartments e.g. rats at both the intestine and liver whereas mice show no resistance at either; (4) Differ in their capability of withstanding the pathology of infection e.g. mice will die within 28 days following infection of more than two metacercariae, whereas rats and rabbits will survive infections of 50 metacercariae or more; and (5) Respond differently to various adjuvants. Despite these limitations, several vaccine candidates, such as FABP, cathepsin L and leucine aminopeptidase, have been shown to induce protection in both rodents and ruminants. More trials in ruminants are required to demonstrate that these can provide consistent protection in the target species.
As pointed out by Meeusen and Piedrafita (2003), the development of a vaccine for any helminth parasite will depend on a better understanding components of the protective process including: (1) Recognition of the antigen; (2) Induction of the protective immune phenotype; and (3) Activation of appropriate immune effector mechanisms. Unfortunately, we have not gathered sufficient data on the present vaccine candidates to identify the cellular components and their means of protection, and this must be of high priority in future studies. Studies on natural immune responses in sheep and cattle, which are Th2-driven and non-protective compared with vaccine-induced protective responses, are imperative to elucidate the mechanism of activation and propagation of cellular and soluble immune factors. If vaccine-induced protection is dependent on inducing factors not normally induced by natural infection, for example a mixed Th1/Th2 response as indicated by the studies with cathepsin L vaccines (Mulcahy et al. 1998, 1999; Dalton and Mulcahy, 2001; Dalton et al. 2003b), then it will be necessary to determine the longevity of this ‘altered’ immune response in the face of continual exposure to Th2-inducing natural infection (and other Th2-inducing pathogens). Also, some vaccines may not be naturally immunogenic (often referred to as hidden antigens) and will not receive boosting following natural infection regardless of the type of immune response induced by the vaccine. In both cases, repeat vaccinations (six-monthly or yearly) may be required to prevent natural infection reversing or even suppressing the vaccine effect. In contrary cases, for example the leucine-aminopeptidase vaccine, it may be sufficient to induce high enzyme-blocking antibody titres of any isotype, and boosting of antibody responses by natural infection may maintain or even enhance protection.
To date there has not been a report of a successful field trial of any liver fluke vaccine. Because of the obvious differences in parasite exposure in experimental vaccine trials and in field conditions (not to mention possible genetic variation in wild parasites compared to laboratory strains) it cannot be predicted how well the current vaccine candidates would perform in the ‘real situation’ and under variable environmental and disease-transmission pressures. Moreover, the immune responses of sheep and cattle (and other species) to natural infection and to vaccination will clearly differ and vaccine design and formulation will likely need to be unique to each species. An additional challenge to overcome will be internal variation in immune responses due to host genetics that may confer some breeds with a better ability to develop protective responses to some antigens and/or to certain formulations. To surmount these obstacles it is likely that the final liver fluke vaccine will be composed of several protective antigens that elicit protection through different mechanisms, involving both antibody and cellular components. The mixture of such a cocktail vaccine could include the antigens described in the present review and a critical next step would be to empirically assess combinations of these in sheep and cattle.
There is an expanding range of adjuvants, commercially available and reported in the literature, with various abilities to activate different components of the immune system. Arriving at the appropriate adjuvant for commercialisation will require systematic testing in trials that investigate site of injection and timing between vaccinations. Ultimately requirements and restrictions related to on-farm/animal-side vaccine use will dictate that vaccines be prepared in easily deliverable, stable adjuvants in a user-friendly way and convenient time (e.g. injectable, given twice within a four-week period with possible, as mentioned above, six-monthly or yearly boosting).
The development of a liver fluke vaccine for animals could play a major role in eliminating human infection in endemic regions. Human disease is always associated with endemic animal disease and, therefore, vaccination of animals (including reservoir hosts) to prevent infection and block disease transmission should be incorporated alongside the administration of chemotherapy to humans in any future control programme. Because the regions where human fasciolosis is endemic tend to be relatively small and geographically confined e.g. the Andean altiplano, the Nile Delta or the northern provinces of Iran, the chance of a well-designed control programme of having success is high. Curtale et al. (2005) recently reported a successful intervention programme in the Nile Delta, Egypt, that involved screening almost 36000 schoolchildren between 1998 and 2002 and treating 1280 cases of fasciolosis with triclabendazole (Egaten). The result of this ‘targeted selective chemotherapy’ in this endemic area was the reduction of prevalence from 5·2% to 1·2%, in addition to lowering infection intensity. This study demonstrates that, with the proper local support, a similar programme could be successful if introduced in other endemic regions and it also raises the possibility of greater success if a parallel animal vaccination programme was put in place. Since fasciolosis is a food-borne disease educational programmes also have a major role to play, although the likelihood of changing current agricultural traditions and eating habits in regions that are generally under-developed and rural is slim. Considering that human behaviour is difficult to change Macpherson (2005) suggests that for educational programmes to succeed the correct message regarding how disease is spread needs to be delivered to the at-risk group, and a direct link between the cause of disease and its resulting pathogenesis has to be made. In summary, a good control programme will require chemotherapy, vaccination, education and local government support.
CONCLUSION
While progress in vaccine development for two of the most important flatworm taxa, schistosomes and fasciolids, has been slow recent results are optimistic for the future implementation of first generation recombinant vaccines. The current schistosome and fasciolid vaccine candidate antigens and prototype vaccine formulations induce significant levels of protection in animals using the standard readouts of reduced worm burden or egg production and viability but are not yet ready for application in the field. It is important to continue to identify new target antigens and to explore alternative vaccination strategies to improve vaccine efficacy. One approach to progressing the challenge is through the establishment of an antigen discovery pipeline. Molecules containing signal peptides and signal anchors as predictors of excretory-secretory products, including enzymes, and components exposed on the schistosome epithelial surfaces (including receptors) that interact directly with the host immune system, are relevant targets for study. Mining, functional annotation and gene ontology categorisation of the greatly expanded genomic databases (Dalton et al. 2003a; Hu et al. 2003; Verjovski-Almeida et al. 2003) has allowed identification of potential vaccine candidates such as secreted toxins/allergens, cell surface adhesion receptors, surface-exposed membrane proteins, receptors for host factors and surface exposed enzymes. Whereas the prediction of vaccine potency from sequence information is somewhat speculative, immunological and vaccine/challenge studies in animal models should confirm the identity of key antigens for further study. Additional vaccine candidates will also likely be discoverd by proteomics (Jefferies et al. 2001; Curwen et al. 2004, 2006; Cheng et al. 2005; van Balkom et al. 2005; Knudsen et al. 2005) and microarrays (Fitzpatrick et al. 2004, 2005; Vermeire et al. 2006; Chai et al. 2006; Gobert et al. 2006; Moertel et al. 2006) approaches.
Difficulties in obtaining good expression levels and scaling up production according to GLP/GMP standards of the limited number of antigens selected have turned out to be another major obstacle. Feasibility of large-scale production should be a prime selection criterion in assessing the vaccine candidacy of schistosome and fasciola antigens (Dalton et al. 2003a; Bergquist et al. 2005). In addition, the selection of a suitable adjuvant to aid in the stimulation of the appropriate immune response is a critical step in the path to the development and employment of a successful transmission-blocking veterinary vaccine for S. japonicum, F. hepatica and F. gigantica.