Introduction
Insect growth and developments are regulated by hormones. Steroid hormones play key roles in the coordinated regulation of many developmental and physiological events such as molting, metamorphosis and diapause in insects (Niwa & Niwa, Reference Niwa and Niwa2014). For example, the precise timing of molting is strictly guided by precisely timed changes of the molting hormone 20-hydroxyecdysone (20E) (Iga & Smagghe, Reference Iga and Smagghe2010). Insect metamorphosis from larva over pupa to a reproductive adult is under the control of 20E as well (Iga & Smagghe, Reference Iga and Smagghe2010). It is generally recognized that insects synthesize ecdysone in the prothoracic gland (PG) from dietary cholesterol or phytosterol, and the synthesized ecdysone (E) is secreted in the hemolymph, and then converted into 20E in various peripheral tissues predominantly in midgut (MG) or fat body (FB) (Gilbert et al., Reference Gilbert, Rybczynski and Warren2002).
Ecdysteroids are biosynthesized from the embryonic to the adult stage. Ecdysone biosynthesis involves a series of enzymatic oxidation reactions (fig. S-1) (Niwa & Niwa, Reference Niwa and Niwa2014). It has demonstrated that Neverland (Nvd) (the [2Fe–2S] Rieske oxygenase) catalyzes the first step from cholesterol to 7-dehydrocholesterol (7-DC) (Yoshiyama-Yanagawa et al., Reference Yoshiyama-Yanagawa, Enya, Shimada-Niwa, Yaguchi, Haramoto, Matsuya, Shiomi, Sasakura, Takahashi, Asashima, Kataoka and Niwa2011). However, the conversion process from 7-DC to ketodiol remains unclear, commonly referred as the ‘Black Box’. The ‘Black Box’ is hypothesized to be catalyzed by at least three enzymes, including the cytochrome P450 monooxygenases Spook (Spo) and CYP6T3, and the short-chain dehydrogenase/reductase Shroud (Sro) (Niwa & Niwa, Reference Niwa and Niwa2014). In addition, it is still possible that other uncharacterized enzymes play a role in ecdysteroid biosynthesis. Recent studies have demonstrated that a glutathione S-transferase encoding gene, noppera-bo (nobo), plays a crucial role in regulating ecdysone biosynthesis in insects (Enya et al., Reference Enya, Ameku, Igarashi, Iga, Kataoka, Shinoda and Niwa2014, Reference Enya, Daimon, Igarashi, Kataoka, Uchibori, Sezutsu, Shinoda and Niwa2015).
Up to now, four cytochrome P450 enzymes encoded by Halloween genes (phm, dib, sad and shd) have been characterized in Drosophila melanogaster, Bombyx mori and Manduca sexta. Phm (CYP306A1), Dib (CYP302A1) and Sad (CYP315A1) sequentially convert the precursor of ecdysone, 2, 22, 25-trideoxyecdysone (ketodiol), to 22, 2-dideoxyecdysone (ketotriol), 2-deoxyecdysone and E. The product of shd (CYP314A1) hydroxylates E–20E, an active form of ecdysteroid. During the past decade, the orthologs of these Halloween genes have been also identified or inferred in several other insects (Christiaens et al., Reference Christiaens, Iga, Velarde, Rougé and Smagghe2010; Iga & Smagghe, Reference Iga and Smagghe2010; Jia et al., Reference Jia, Wan, Zhou, Mu and Li2013; Wan et al., Reference Wan, Jia, Li, Fan and Li2014a ; Cabrera et al., Reference Cabrera, Shirk, Evans, Hung, Sims, Alborn and Teal2015). Although many ecdysteroidogenic enzyme-encoding genes are well conserved in the genomes of insects (Niwa & Niwa, Reference Niwa and Niwa2014), previous studies also raise the possibility that ecdysteroid biosynthesis is differentially regulated among various insect species (Enya et al., Reference Enya, Ameku, Igarashi, Iga, Kataoka, Shinoda and Niwa2014). This situation highlights the necessity to know the specific molecular mechanism of ecdysteroid synthesis in certain insects of interest.
From the aspect of pest control, P450 enzymes that catalyze physiologically important reactions offer insect-selective targets for discovering and developing new insecticides (Niwa & Niwa, Reference Niwa and Niwa2014; Feyereisen, Reference Feyereisen2015). Several studies have showed that such gene can serve as a potential target gene for RNA-interference-based pest management (Luan et al., Reference Luan, Ghanim, Liu and Czosnek2013; Kong et al., Reference Kong, Liu, Wan, Shi, Guo and Li2014; Wan et al., Reference Wan, Jia, Li, Fan and Li2014b ; Wan et al., Reference Wan, Jia, Li, Fan and Li2015). For example, knockdown of CYP314A1 in Leptinotarsa decemlineata by dietary introduction of double-stranded RNA into the secondary instar larvae caused larval lethality, delayed development and reduced pupation ratio (Kong et al., Reference Kong, Liu, Wan, Shi, Guo and Li2014). Similarly, knockdown of CYP306A1 in the white-backed planthopper Sogatella furcifera through dietary delivery into the 2nd instars caused lethality and slowed down ecdysis during nymphal stages (Wan et al., Reference Wan, Jia, Li, Fan and Li2014b ), and oral delivery of double-stranded RNA of Laodelphax striatellus CYP315A1 at the nymph stage caused nymphal lethality and delayed development in a dose-dependent manner (Wan et al., Reference Wan, Jia, Li, Fan and Li2015).
The cotton bollworm Helicoverpa armigera is a dreaded pest worldwide (Fitt, Reference Fitt1989). Although molting and metamorphosis are known to be controlled by 20E in this holometabolous insect, the enzymes in ecdysteroid synthetic pathway remain to be characterized. As the first step toward characterizing the enzymes involved in ecdysteroid biosynthesis, in this study, we tried to probe H. armigera for Halloween gene orthologs, and investigated their transcriptional patterns. This effort may advance our knowledge about the molecular details of ecdysteroid biosynthesis in insects and may contribute to developing new insecticides targeting these ecdysteroidogenic enzymes for a smart control of this important pest.
Materials and methods
Insects
A colony of cotton bollworm H. armigera (Hübner) was established from a field collection from Henan Province, China in 2005 and was maintained in the laboratory. Larvae were individually reared in glass tubes on wheat germ-based artificial diets (Wu & Gong, Reference Wu and Gong1997), at 25 ± 1°C and relative humidity of 70% with a photoperiod of 16-h light/8-h dark. Adults were kept under the same temperature and light conditions, and provided with a 10% honey solution.
Cloning of full-length transcripts of Halloween genes
Total RNA was extracted from entire 2nd-instar larvae of H. armigera by using TRIzol (Invitrogen, CA, USA) according to the manufacture's protocol. First-strand cDNA was synthesized from total RNA (1 µg) using PrimeScript First Strand Synthesis Kit according to the manufacturer's instructions (Takara, Dalian, China).
All the oligonucleotide primers (table 1) used in this study were commercially synthesized by Invitrogen (China). The cloning of each gene was performed by three steps. Firstly, a fragment of cDNA was amplified by PCR using degenerate primers. Then the 3′-end and 5′-end of this cDNA fragment were obtained respectively via rapid-amplification of cDNA ends (RACE) using SMART™ RACE cDNA amplification kit according to the manufacturer's protocols (Clontech, CA, USA) with gene-specific primers used for 3′- and 5′-RACE. Lastly, the full-length open reading frames were amplified for sequence confirmation using corresponding primers (table 1), which were designed based on the sequence information obtained from 3′- and 5′-RACE ends. Products which amplified using the high-fidelity DNA polymerase (Pfu) were gel purified (Takara, Dalian, China) and then subcloned into pEasy-T1 and transformed the Escherichia coli Trans 5α strain (Transgen, Beijing, China). At least three positive clones were sequenced for each gene.
Table 1. Oligonucleotide primers used in this study.
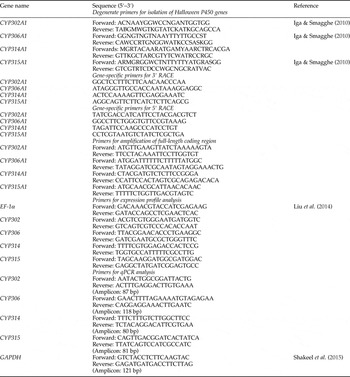
Sequence identification and phylogenetic analysis
Bioinformation analysis was conducted using on line software (http://web.expasy.org/protparam/). Alignment of amino acid sequence was performed by using Sequence Alignment tool Clustal W program, and a neighbor-joining phylogenetic tree was generated by MEGA 6 (Tamura et al., Reference Tamura, Stecher, Peterson, Filipski and Kumar2013).
Spatial and temporal expression analyses using RT–PCR
The expression profiles were evaluated in various tissues and at various developmental stages of H. armigera by RT–PCR. Total RNA from eggs (12 h old), selected tissues (head, MG, Malpighian tubules, FB and epidermis) of the 5th-instar larvae (1 day old), female and male pupae (4–5 days old), and different parts from female and male adults (3–4 days old), were prepared using TRIzol according to the manufacturer's protocol (Invitrogen, Carlsbad, CA). Ten individuals were used for each preparation. To remove potential genomic DNA contamination, All RNA samples were treated with RNase-free DNase I (Takara, Dalian, China).
The first-strand cDNA was synthesized from 1 µg RNA with an oligo (dT) primer using the MLV reverse transcriptase. At the same time of cDNA synthesis, samples without reverse transcriptase were prepared for evaluating the genomic contamination. The RT–PCR amplifications were carried out in a final volume of 25 µl reaction containing 2 µl of 10× diluted template cDNA, 12.5 µl Taq Master Mix (Tiangen, Beijing, China), 0.5 µl (10 µM) of each primer and sterilized water up to the final volume. The primers used for RT–PCR analysis were listed in table 1. The elongation factor-1α gene (EF-α) was used as a reference gene (Zhou et al., Reference Zhou, Ma, Li, Sheng, Liu and Qiu2009). The thermal cycling profile consisted of an initial step of denaturation at 95°C for 5 min, followed by 30 cycles of 95°C for 30 s, 55–60°C for 30 s and 72°C for 30 s, and a final extension step of 72°C for 5 min. Aliquots of PCR products were analyzed on a 2% agarose gel. In order to confirm the fidelity of the RT–PCR, the PCR products were gel purified and sequenced.
Quantitative real-time PCR (qPCR) analysis of gene expression
A sensitive quantitative analysis was performed to compare the expression profile of each Halloween gene in the PG and two main peripheral tissues (the MG and FT) during larval development. Three independent cDNA samples originating from different tissues of 5–10 animals for each time point were used. qPCR assays were designed and performed according to the MIQE guidelines (Bustin et al., Reference Bustin, Benes, Garson, Hellemans, Huggett, Kubista, Mueller, Nolan, Pfaffl, Shipley, Vandesompele and Wittwer2009), using SYBR premix Ex Taq II (Perfect Real Time) kit (Takara) on MX3005P machine (Strategene). The primers used for qPCR, amplifying a product with a size between 80 and 121 bp, were listed in table 1. The reaction of 20 µl in total volume contained 10 µl 2xSYBR premix Ex Taq™ II, 0.4 µl 50× ROX II and 0.4 µl each primer and 2 µl cDNA template using the cycling parameters: 95°C for 15 s, followed by 40 cycles of 95°C for 5 s, 56°C for 34 s and 68°C for 30 s, and a cycle of 95°C for 15 s and 60°C for 1 min. The melting curves of amplicons were measured by taking continuous fluorescence reading while increasing temperature from 56 to 95°C with 0.5°C increments for 10 s. Potential contamination was checked by including non-reverse transcriptase preparation and non-template controls. PCR fidelity was confirmed by checking the amplicon size and sequencing. PCR product homogeneity was evaluated by melting curve analysis. Amplification efficiency was calculated by serial dilutions from 1- to 1000-fold of each cDNA templates. mRNA levels of the target genes were normalized to GAPDH (encoding glyceraldehyde-3-phosphate dehydrogenase) after correcting for differences in amplification efficiency. In a pilot study, we considered several housekeeping genes encoding GAPDH, EF-1α, ribosomal protein L28, ribosomal protein S3 as the candidate reference genes based on a literature review, and found that GAPDH gene was stably expressed. Under the conditions described in this study, the amplification efficiency for each gene was between 102 and 108%, and the gene-specific PCR products were achieved. All qPCR analyses were performed with three independent biological replicates and three technical replicates. The relative expression levels of target genes were calculated by the comparative C t method as described by Livak & Schmittgen (Reference Livak and Schmittgen2001). Gene expression data (relative expression) were statistically analyzed by the analysis of variance (ANOVA) followed by Tukey's HSD multiple comparisons for mean separation among different tissues using SPSS for Windows (PASW 18, Chicago, IL, USA). A P-value of <0.05 was considered significant.
Results
Cloning and identification of Halloween P450 homologs from H. armigera
Full-length transcripts of four Halloween P450 orthologs from H. armigera, namely HarmCYP302A1 (GenBank number: KP764853), HarmCYP306A1 (KP764854), HarmCYP314 (KP764856) and HarmCYP315A1 (KP764855) respectively, were obtained. Overall, these transcripts encode proteins with a size consistent with the character of cytochrome P450s. They possessed the conserved structural attributes of many P450s (Feyereisen, Reference Feyereisen and Gilbert2012), for example the WxxxR motif in Helix-C, the GxE/DTT/S in Helix-I, the ExLR in Helix-K and the heme-binding domain (PFxxGxRxCxG/A) (figs 1–4). Although the PERF motif (PxxFxPE/DRF/W) was easily discernible in HarmCYP302A1, this motif in other three proteins was not very typical. The PERF motif in HarmCYP306A1 was modified by a substitution of E/D with S, while only PERW and PYRW were visible in HarmCYP314A1 and HarmCYP315A1, respectively. In addition, the N-terminals of HarmCYP302A1 and HarmCYP315A1 carried the distinctive mitochondrial import sequence consisting of several charged residues. HarmCYP306A1 contained the microsomal P450 characteristic N-terminal string consisting of hydrophobic residues followed by a proline–glycine (P/G)-rich region (figs 1–4). The two to three positively charged residues interacting with adrenodoxin (Feyereisen, Reference Feyereisen and Gilbert2012), a signature of mitochondrial enzymes, were observed in HarmCYP302A1, HarmCYP314A1 and CYP315A1. Additional two positively charged residues near the heme-binding site were found in HarmCYP302A1, HarmCYP314A1 and CYP315A1. Notably, HarmCYP314A1 has a P/G-rich region and lack some of the positively charged residues, indicating that HarmCYP314A1 can be both microsomal and mitochondrial.
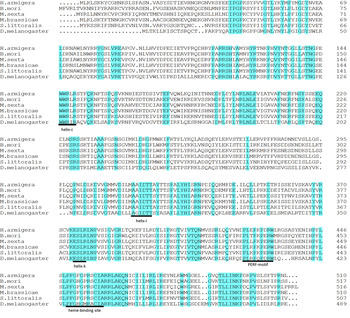
Fig. 1. Alignment of selective CYP302A1 sequences using the Clustal W program. Identical residues are highlighted. Dots indicate gaps inserted for optimizing the alignment. The conserved motifs are underlined.
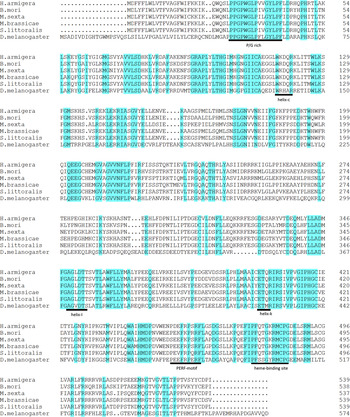
Fig. 2. Alignment of selective CYP306A1 sequences using the Clustal W program. Identical residues are highlighted. Dots indicate gaps inserted for optimizing the alignment. The conserved motifs are underlined.
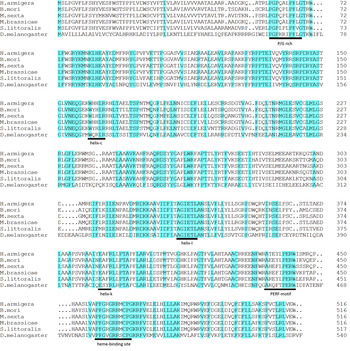
Fig. 3. Alignment of selective CYP314A1 sequences using the Clustal W program. Identical residues are highlighted. Dots indicate gaps inserted for optimizing the alignment. The conserved motifs are underlined. Two positively charged residues, a signature of mitochondrial enzymes, are boxed.
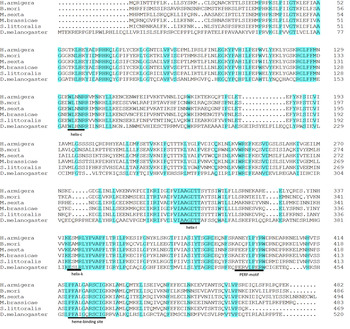
Fig. 4. Alignment of selective CYP315A1 sequences using the Clustal W program. Identical residues are highlighted. Dots indicate gaps inserted for optimizing the alignment. The conserved motifs are underlined.
The identified Halloween P450 genes are highly conserved in Lepidoptera. Helicoverpa armigera orthologs show 66–80% (CYP302A1), 80–92% (CYP306A1), 85–93% (CYP314A1) and 58–80% (CYP315A1) identity to lepidopteran orthologs (tables S1 and S2). On the other hand, the identity between H. armigera and insects of other orders were much lower (<46%) (tables S1 and S2).
A phylogenetic analysis was performed using the putative amino acid sequences of Halloween P450 genes available in GenBank. The phylogenetic tree clearly clustered into two groups, the CYP2 clan (CYP306A1) and the mitochondrial clan (CYP302A1, CYP314A1 and CYP315A1) (fig. 5).

Fig. 5. Phylogenetic tree of the four Halloween P450 genes generated using the neighbor-joining method performed with the amino acid sequences by Mega 6. The scale bar represents the evolutionary distances computed using the Poisson correction method. The information (the full name of animal species and the GenBank access number of the used sequences) was listed in table S1.
General spatial and temporal patterns of H. armigera Halloween P450 genes by RT–PCR
The general spatial and temporal expressions of the four Halloween genes were determined using the RT–PCR approach with the EF-α as a reference. Using templates without the addition of reverse transcriptase, no amplification was observed (data not shown). Transcripts of all the four genes were detected during all stages of development (fig. 6).
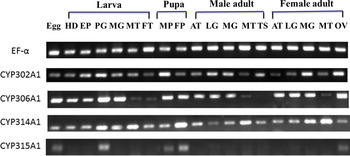
Fig. 6. Expression profile of Halloween genes in various tissues at different developmental stages. EF-α = the elongation factor-1α; HD, head; EP, epidermis; PG, prothoracid gland; MG, midgut; MT, Malpighian tubules; FT, fat body; MP, male pupae; FP, female pupae; AT, antenna; LG, leg; TS, testes; OV, ovary.
HarmCYP302A1 and HarmCYP314A1 were detected in all the selected tissues of both larvae and adults. HarmCYP306A1 was detected in all the selected tissues except that its expression in adult testis was below the reliable detection level. Notably, HarmCYP315A1 was expressed specifically in egg, larval PGs, pupae and adult ovaries.
Spatial and temporal patterns of H. armigera Halloween P450 genes in the PG and peripheral tissues during larval development by qPCR
A more sensitive quantitative analysis was performed to compare the expression profile of each Halloween gene in the endocrine tissue (PGs) and two main peripheral tissues (the MG and FT) during the fifth larval instar (fig. 7) and the final instar (fig. 8). HarmCYP302A1 was predominantly expressed in PG at both 5th instar (L5) and the final (6th) instar larvae (L6), and the expression level in PG in L5 is higher than in L6 in PG. Furthermore, developmental variation in the expression of CYP302A1 in PG was obvious. There was an increase from day 0 to day 1 in L5 and then a sharp reduction from day 2 in L5 to L6 (fig. 7), and an increase later on at day 3 in L6 again (fig. 8). For HarmCYP306A1, higher expression was observed in PG at day 1 and day 2 in L5, while the comparable level of the expression was detected between PG and MG except at day 1 in L6. In addition, the maximal expression level of HarmCYP306A1 was lower in L6 than L5 (L5D2/L6D3 = 3.3-fold). The lowest abundance of HarmCYP314A1 was observed in MG, while the HarmCYP314A1 levels were comparable between PG and FB in L5 except at day 2 (the highest expression was detected in FB). Overall there was no significant difference in the HarmCYP314A1 level among the three selected tissues except at day 3 in L6. HarmCYP315A1 was predominantly expressed in PG of both the 5th instar and the final instar larvae.
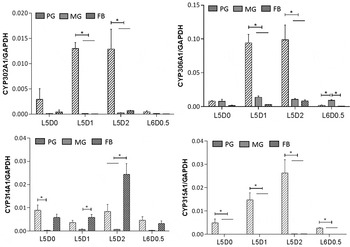
Fig. 7. Developmental expression profile of four Halloween P450 genes of Helicoverpa armigera in three tissues during 5–6th instar larval transitions. The expression levels were measured by qPCR. Each datum point represents the mean ± SEM of the expression level relative to GAPHD. The relative expression levels were compared among three tissues at each time point. Significant difference is marked with *(P < 0.05). PG, prothoracic glands; MG, midguts; FB, fat bodies; L, larval stage; D, day.
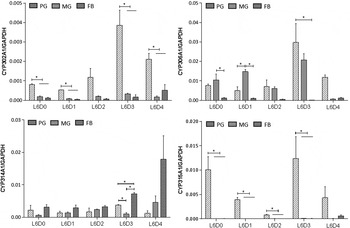
Fig. 8. Developmental expression profile of four Halloween genes in three tissues of 6th larvae of Helicoverpa armigera. The expression levels were measured by qPCR. Each datum point represents the mean ± SEM of the expression level relative to GAPHD. The relative expression levels were compared among three tissues at each time point. Significant difference is marked with *(P < 0.05). PG, prothoracic glands; MG, midguts; FB, fat bodies. L, larval stage; D, day.
Although HarmCYP315A1 and CYP302A1 were dominantly expressed in PG, their expression were detectable in MG and FB by the very sensitive qPCR measurement, with an abundance being much lower compared with that in PG (figs 7 and 8). For example, the relative expression levels of CYP302A1 in MG and FB to that in PG were 5.6 × 10−3 and 2.7 × 10−3, respectively, while the abundance of HarmCYP315A1 in PG was about 1000-fold higher than that in MG or FB at day 1 in L5 (fig. 7).
Discussion
Here, by using RT–PCR with degenerate primers and RACE approaches, four Halloween P450 genes (HarmCYP302A1, HarmCYP306A1, HarmCYP314A1 and HarmCYP315A1) putatively encoding enzymes involved in the final hydroxylation steps in the synthesis of ecdysone from the cotton bollworm H. armigera were identified. The proteins encoded by these four genes possess typical P450 motifs, and exhibit high similarity to those previously identified from other lepidopteran insects (table S2).
Previous studies showed that the expression of ecdysteroidonenic genes, including CYP302A1, CYP306A1 and CYP315A1 is restricted to the PG cells at the larval stage in Drosophila, Manduca and Bombyx (Chavez et al., Reference Chavez, Marques, Delbecque, Kobayashi, Hollingsworth, Burr, Natzle and O'Connor2000; Warren et al., Reference Warren, Petryk, Marques, Jarcho, Parvy, Dauphin-Villemant, O'Connor and Gilbert2002, Reference Warren, Petryk, Marques, Parvy, Shinoda, Itoyama, Kobayashi, Jarcho, Li, O'Connor, Dauphin-Villemant and Gilbert2004; Namiki et al., Reference Namiki, Niwa, Sakudoh, Shirai, Takeuchi and Kataoka2005; Niwa et al., Reference Niwa, Sakudoh, Namiki, Saida, Fujimoto and Kataoka2005; Reference Niwa, Namiki, Ito, Shimada-Niwa, Kiuchi, Kawaoka, Kayukawa, Banno, Fujimoto, Shigenobu, Kobayashi, Shimada, Katsuma and Shinoda2010; Ono et al., Reference Ono, Rewitz, Shinoda, Itoyama, Petryk, Rybczynski, Jarcho, Warren, Marques, Shimell, Gilbert and O'Connor2006; Yoshiyama et al., Reference Yoshiyama, Namiki, Mita, Kataoka and Niwa2006; Rewitz et al., Reference Rewitz, Rybczynski, Warren and Gilbert2006a , Reference Rewitz, Rybczynski, Warren and Gilbert b ). More recently, accumulated evidence suggests that PG is not the only organ for ecdysteroid biosynthesis. Peripheral tissues such as MG and FB have been shown to convert the ketodiol into 20E (Brown et al., Reference Brown, Sieglaff and Rees2009). For example, CYP306A1 was also expressed in MG besides PG in the larvae of Spodoptera littoralis (Iga & Smagghe, Reference Iga and Smagghe2010). Our data showed that HarmCYP302A1, HarmCYP306A1 and HarmCYP314A1 transcripts were detected in many tissues of larvae (fig. 6). Real-time PCR results show that HarmCYP302A1 and HarmCYP315A1 were predominantly expressed in PG. However, this predominance was not observed for HarmCYP306A1 and CYP314A1. For example, the highest expression of HarmCYP314A1 was detected in FB of larvae (at day 2 in L5, day 3 in L6 (figs 7 and 8), similar to the pattern observed in S. littoralis (Iga & Smagghe, Reference Iga and Smagghe2010)). This work provides another case demonstrating the expression of Halloween P450 genes in non-endocrine tissues. Whether these tissues can serve as secondary sources of primary or secondary ecdysteroids deserves further investigation. High expression of HarmCYP314A1 in FB (figs 7 and 8) suggests that FB plays a crucial role in the last step of steroid synthesis, i.e. transforms E into the more active 20E.
Steroid hormones are involved in regulating biological processes such as germline development and innate immunity in the adult stage of the ecdysozoan animals, therefore steroid hormones must be synthesized in this stage (Niwa & Niwa, Reference Niwa and Niwa2014). In the case of H. armigera, the four P450s were all expressed in the ovaries, suggesting that the ovaries may be the site of ecdysteroid synthesis in female adults. This observation is in keeping with the generally accepted view that ecdysteroid production is taken over by the gonads in adults (Dubrovsky, Reference Dubrovsky2005). Interestingly, except HarmCYP315A1, the expressions of other three P450s were detectable in non-endocrine tissues (e.g. MGs and legs) besides the ovaries in adult females.
In summary, the sequences and expression profiles of four Halloween genes in the cotton bollworm H. armigera were described in this paper. All the four P450 genes are evolutionally conserved in Lepidoptera. Overall, these genes are predominantly expressed in PGs, and also detectable in non-endocrine tissues. High expression of HarmCYP314A1 in FB indicates that FB plays important roles in the conversion of E into 20E in larval–larval molt and in larva–pupal metamorhphosis. Further functional studies of these Halloween P450 orthologs are underway in our laboratory.
Supplementary Material
The supplementary material for this article can be found at http://dx.doi.org/10.1017/S0007485316000663
Acknowledgments
This work was supported by grants from National Basic Research Program of China (973 Program, Grant No. 2012CB114103) and National Natural Science Foundation of China (Grant No. 31471796) to X. Qiu.