Introduction
The bearded horse mussel Modiolus barbatus (Linnaeus, 1758) is a benthic marine bivalve, mainly distributed in the Mediterranean Sea and Moroccan and Iberian coasts of the eastern Atlantic Ocean (Poppe & Goto, Reference Poppe and Goto2000). On account of their physiological features, such as survival and growth rates, in combination with the existence of market demand, M. barbatus mussels constitute a promising candidate species for aquaculture (Peharda et al., Reference Peharda, Ezgeta-Balić, Davenport and Vrgoč2013). Horse mussel populations are indigenous in most of the Aegean Sea and are also products of high economic value for the Greek fisheries.
Evaluation of the genetic structure of an organism intended for farming is considered one of the major essential tools prior to the development of a new form of aquaculture. The capability of aquatic organisms to adapt towards environmental changes mainly depends on the intraspecific genetic variability of their populations. In order to prevent potentially harmful effects of inbreeding depression in the cultured populations, management strategies should take into consideration the preservation of genetic variability (Ye et al., Reference Ye, Li, Wu, Xu and Guo2012). Furthermore, historical evolutionary events may influence the genetic variability of distinct geographic populations by bottleneck effects, effective population size alterations or by creating barriers to gene flow (Geraghty et al., Reference Geraghty, Williamson, Macbeth, Wintner, Harry, Ovenden and Gillings2013). In this sense, phylogeographic relationships and comparison of estimated genetic variation among populations are key factors that provide necessary information for the evaluation of the evolutionary history of a species, with an eventual focus on the proper design of population management strategies.
Analysis of mitochondrial DNA (mtDNA) has been utilized in a wide range of aquatic taxa for the evaluation of molecular phylogeny, phylogeography and genetic diversity within and among geographic populations (Giantsis et al., Reference Giantsis, Abatzopoulos, Angelidis and Apostolidis2014a, Reference Giantsis, Kechagia and Apostolidis2015; Ye et al., Reference Ye, Wu and Li2015; Ladoukakis & Zouros, Reference Ladoukakis and Zouros2017). Widely accepted key factors for the utility of mtDNA markers include the relative lack of recombination, the selective neutrality, the fact that animal mitochondrial genes lack introns, the elevated mutation rate compared with nuclear DNA and the maternal inheritance in the majority of marine animal species (Ladoukakis & Zouros, Reference Ladoukakis and Zouros2017). Particularly, the analysis of a standardized fragment of cytochrome oxidase subunit I (COI) gene has been proven highly informative for providing the first genomic insights with regard to animal species for which there is limited information available concerning their genetic structure. This ~650 bp fragment serves as a DNA barcode for identifying animal species (Hebert et al., Reference Hebert, Cywinska, Ball and De Waard2003). As a consequence, it has been described for more animal species than any other DNA segment, being available in public databases, e.g. GenBank and BOLD (Barcode of Life Data Systems, http://www.boldsystems.org), and hence supporting an integrated phylogenetic reconstruction of the examined species. Although in most animal species mtDNA is inherited strictly through maternal lineage (Hassan et al., Reference Hassan, El Nahas, Kumar, Godithala and Roushdy2009), this feature meets some interesting idiomorphic exceptions in several bivalve molluscs (Ladoukakis & Zouros, Reference Ladoukakis and Zouros2017), including the horse mussels that belong to the species Modiolus modiolus (Robicheau et al., Reference Robicheau, Powell, Del Bel, Breton and Stewart2017). Briefly, according to this unusual pattern of inheritance, namely doubly uniparental inheritance (DUI), maternal mtDNA is transmitted to offspring of both sexes, whereas paternal mtDNA is transmitted exclusively to males (Ladoukakis & Zouros, Reference Ladoukakis and Zouros2017). As a result, two types of differentially evolved mtDNA co-occur, a fact that should receive special attention in population genetic studies of marine bivalves (Giantsis et al., Reference Giantsis, Abatzopoulos, Angelidis and Apostolidis2014a).
Larvae of marine molluscan species come up against a passive dispersal through sea currents, resulting in the absence of genetic differentiation among distant geographic populations (Larsson et al., Reference Larsson, Lönn, Lind, Świeżak, Smolarz and Grahn2016). Instead, oceanographic, temporal or environmental factors rather than geographic ones may form barriers to gene flow with an eventual effect on population sub-structure (Wu et al., Reference Wu, Bai, Liu, Jin, Yin and Li2016; Banci et al., Reference Banci, Mori, Oliveira, de Paganelli, Pereira and Pinheiro2017; Gutierrez et al., Reference Gutierrez, Turner, Gharbi, Talbot, Lowe, Peñaloza and Houston2017). Particularly in the Aegean Sea, following the intense water mass circulation (Olson et al., Reference Olson, Kourafalou, Johns, Samuels and Veneziani2007) that may favour gene flow among molluscan bivalve populations, absence of or small genetic differentiation has been revealed in the Mediterranean mussel populations through different genetic markers (Giantsis et al., Reference Giantsis, Abatzopoulos, Angelidis and Apostolidis2014a, Reference Giantsis, Mucci, Randi, Abatzopoulos and Apostolidis2014b). Regarding horse mussels, phylogeographic and population genetic relationships have been studied from various sampling localities throughout the distribution of M. modiolus (Halanych et al., Reference Halanych, Vodoti, Sundberg and Dahlgren2013), as well as by the inclusion of M. modiolus and M. barbatus COI haplotypes in a large phylogenetic analysis of molluscs (Barco et al., Reference Barco, Raupach, Laakmann, Neumann and Knebelsberger2016). Nevertheless, the genetic composition of horse mussels (M. barbatus) from any marine area in the Mediterranean is inadequately elucidated. In the context of this lack of knowledge, the main goal of the present study was the characterization of the population genetic structure of the horse mussels (M. barbatus) from the Aegean Sea, due to their ecological importance constituting an exported fishery product of high economic value. Moreover, due to their promising potential for aquaculture, our scope was also to compare genetic diversity indices among different geographic populations. Finally, the systematic status of M. barbatus from the eastern Mediterranean was re-stated, by constructing phylogeny upon previously published COI haplotypes of closely related species.
Materials and methods
Horse mussel collection and sampling sites
Adult Modiolus barbatus individuals were collected by professional scuba divers from five marine areas in the Aegean Sea, between April and September 2017. Approximately 40–50 specimens were collected from each locality, all of which originated from low depths (<15 m). All specimens were stored shelled in the freezer (−20°C) until further molecular analysis. Three out of the five sampling sites were located in Thermaikos Gulf, whereas the remaining two were located on the coast of Porto Lagos and in the western marine area of the island of Mytilene. All sampling sites were representative of the different microhabitats that favour high abundance (Figure 1).
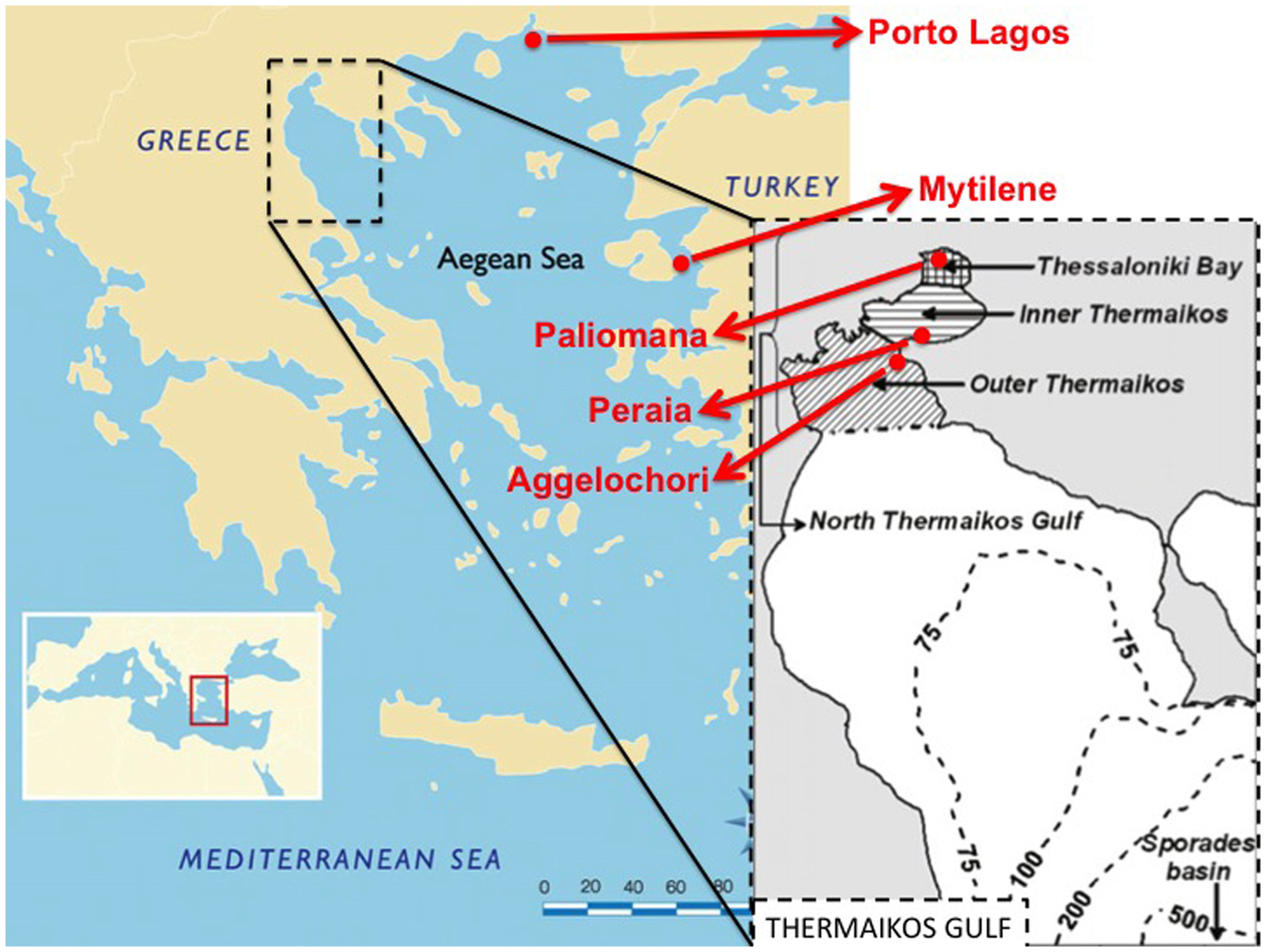
Fig. 1. Sampling sites of the five collected Modiolus barbatus populations in the Aegean Sea. Sub-division of Thermaikos Gulf (40.2893°N 23.0250°E) areas as defined by Krestenitis et al. (Reference Krestenitis, Kombiadou and Androulidakis2012).
Thermaikos Gulf is a semi-closed basin in northern Greece, plentiful of organic material due to the outflow of four rivers and therefore hosting various filter-feeding bivalve species. The three sampling sites in Thermaikos Gulf were representative of the different microhabitats of the gulf. Particularly, samples from Aggelochori were collected from the outer part of Thermaikos Gulf, which is affected by the higher salinity and clean waters of the north Aegean Sea (Krestenitis et al., Reference Krestenitis, Kombiadou and Androulidakis2012). The second sampling site, Peraia, is located in the inner Thermaikos Gulf, where the lower depths allow faster water temperature fluctuations, while the topography reduces the open sea impact (Krestenitis et al., Reference Krestenitis, Kombiadou and Androulidakis2012). Paliomana is located in inner Thessaloniki Bay, which is the second largest harbour and Thessaloniki is the second largest city in Greece, with ~1 million inhabitants. It should also be noted that the main Greek aquaculture area for the Mediterranean mussels (Mytilus galloprovincialis) is located in the western part of the Thermaikos Gulf. Fishery and trade of horse mussels originating from Thermaikos Gulf has been occasionally prohibited during the past years based on bio-accumulation of heavy metals in specific tissues (Katsikatsou et al., Reference Katsikatsou, Anestis, Pörtner, Kampouris and Michaelidis2011).
The marine area of Porto Lagos is located in the region of Thrace (north-eastern Greece, Figure 1), extended between two big cities, Xanthi and Komotini. Similarly to Thermaikos Gulf, Porto Lagos is affected by freshwater outflows due to the connection with the Vistonis Lake system, forming excellent conditions for marine bivalve populations.
The island of Mytilene is located in the Eastern Aegean Sea, only a few kilometres away from the coast of Turkey. Fisheries around the coasts of Mytilene are very well developed. Specifically, it should be noted that horse mussels exported from Greece, mainly originate from the island of Mytilene.
DNA extraction, PCR and sequencing
Approximately 20–30 mg of mantle tissue was cut with UV sterilized tools from each individual and subjected to DNA extraction. Genomic DNA was extracted using NucleoSpin Extraction Kit (Macherey-Nagel, Germany), following the manufacturer's protocol. The quantity and quality of the extracted DNA were estimated on a Biospec-nano spectrophotometer (Shimadzu, Japan).
A standardized fragment of the COI gene was amplified using the universal primers HCO2198 and LCO1490 (Folmer et al., Reference Folmer, Black, Hoeh, Lutz and Vrijenhoek1994). Amplifications were performed in total volumes of 25 µl, containing 0.6 µl of each primer (10 pmol µl−1), 12.5 µl MyTaqTM ready-to-use 2× mix (Bioline, UK), 50 ng of template DNA and ultrapure water up to 25 µl. Initially, 10 successfully amplified samples were purified using the NucleoSpin PCR Clean-up Kit (Macherey-Nagel, Germany) and were then sequenced in both directions using the PCR primers in an ABI Prism 3730XL capillary sequencer in Eurofins Genomics (Austria).
Male mitochondrial genome (M mitotype) evolves faster than the female (F) mitotype, whereas extreme sequence divergence has been noted between the two mitotypes in Modiolus modiolus (Robicheau et al., Reference Robicheau, Powell, Del Bel, Breton and Stewart2017). Keeping this in mind, in the present study the COI gene exclusively of the F mitotype was analysed since it is present in both males and females. Therefore, based on the 10 derived sequences as well as on six Modiolus haplotypes of both sexes obtained from the GenBank database (accession numbers: GQ480314, KC119352, KC119353, KC119349, AB076912, KX452400), a new internal primer was designed (5′- TATTGAGTTAGGTCGGCCCGGGAG-3′), specific for the female mitochondrial genome. A non-conserved region of high nucleotide divergence between the two mitotypes in M. modiolus was selected for the design of the primer, in order to exclude the possibility of hybridizing to the M mitotype. Our designed primer was utilized for sequencing of all the remaining amplified specimens using the purification kit and instruments described above.
Statistical analysis
Sequences were edited and aligned using the MUSCLE algorithm in the software MEGA version 6.05 (Tamura et al., Reference Tamura, Stecher, Peterson, Filipski and Kumar2013). Representative COI sequences of all available Modiolus species in the GenBank database including also a M. modiolus male haplotype, were utilized for the construction of the haplotype phylogeny dataset and one Mytilus galloprovincialis haplotype was also included to be defined as an outgroup in the phylogenetic analysis. Poorly aligned regions were excluded using the GBlocks program (Castresana, Reference Castresana2000) applying the default parameters. To examine potential saturation in any codon position, the uncorrected distances (p-distances) were plotted against corrected model distances (Maximum Likelihood) in the sense that a curved or plateau relationship would indicate evidence for saturation.
Phylogenetic relationships of the derived haplotypes against congeneric species were investigated by the Bayesian approach using the software BEAST 1.7.5 (Drummond et al., Reference Drummond, Suchard, Xie and Rambaut2012) and the Maximum Parsimony (MP) and the Maximum Likelihood (ML) approaches embedded in the software MEGA, applying 1000 bootstrap iterations. As evaluated by MEGA, the best-fit evolution model was the HKY + G + I substitution model, which was therefore used for the ML analysis. Since all Greek haplotypes were grouped together, only a bunch is presented in the constructed ML dendrogram, in an effort to provide a better depiction of the interspecific genetic relationships. An approximate Bayesian computation (ABC), in the software DIYABC (Cornuet et al., Reference Cornuet, Santos, Beaumont, Robert, Marin, Balding, Guillemaud and Estoup2008), was used to evaluate the possible scenarios of the horse mussel's introduction in the Eastern Mediterranean. For simplicity, apart from the Eastern Mediterranean haplotypes, congeneric haplotypes from north Europe and Asia-Pacific were included in the ABC and were considered as three populations. Relatedness among haplotypes was further evaluated by a median joining network generated in the software PopART (Leigh & Bryant, Reference Leigh and Bryant2015), using default goodness to fit parameters.
Genetic diversity indices such as the number of segregating sites, haplotype and nucleotide diversity were estimated considering all individuals as one population, as well as for each geographic population separately, using the software DnaSP version 6.10.04 (Rozas et al., Reference Rozas, Ferrer-Mata, Sánchez-Delbarrio, Guirao-Rico, Librado, Ramos-Onsins and Sánchez-Gracia2017). Selection neutrality was examined by Tajima's D index (Tajima, Reference Tajima1989) for each population separately and at the species level in the software MEGA. The software Arlequin version 3.11 (Excoffier et al., Reference Excoffier, Laval and Schneider2005) was finally used to perform pairwise comparisons for genetic differentiation (F st) and to estimate the levels of significance of these comparisons.
Results
In total 94 partial sequences of the COI gene were obtained from the F mitotype of the horse mussel (M. barbatus) from the sampled geographic populations within the research area (Figure 1). The final alignment dataset was 660 bp in length, with no insertions or deletions (indels) detected. From the 85 observed variable sites, 46 were parsimoniously informative, generating 49 different haplotypes, the distribution of which in the sampled populations and their accession numbers after deposition in GenBank are shown in Table S1. The relationship between corrected and model distances was linear (Figure 1S), indicating no evidence for saturation in any codon position.
Using the most shared haplotypes, a dendrogram was constructed depicting the phylogenetic relationships of M. barbatus from eastern Mediterranean with congeneric haplotypes from other marine areas of the world (Figure 2). The topology of the dendrograms of all three methods used (MP, ML and Bayesian) was identical and the bootstrap values and Bayesian posterior probabilities are indicated on each clade of the tree. Similarly to M. modiolus female haplotypes that were grouped together with high levels of genetic distance from the male mitotype, all novel M. barbatus haplotypes were monophyletic, providing evidence that all generated sequences belong to the female mitotype. With the exception of Eastern Mediterranean M. barbatus haplotypes that were unexpectedly not closely related with M. barbatus haplotypes from the North Sea, all the remaining groups of conspecific haplotypes were clustered together, supported by high bootstrap scores. The sequence JF496770 of Modiolula phaseolina (Philippi, 1844) originating from the Adriatic Sea was the most closely related haplotype to the M. barbatus haplotypes from the Aegean Sea. Based on these observations we evaluated the origin of horse mussels and their historical route into the Mediterranean. According to the ABC analysis, the most likely scenario of colonization suggests the introduction of M. barbatus from Asia-Pacific into the Eastern Mediterranean Sea (Figure 3).
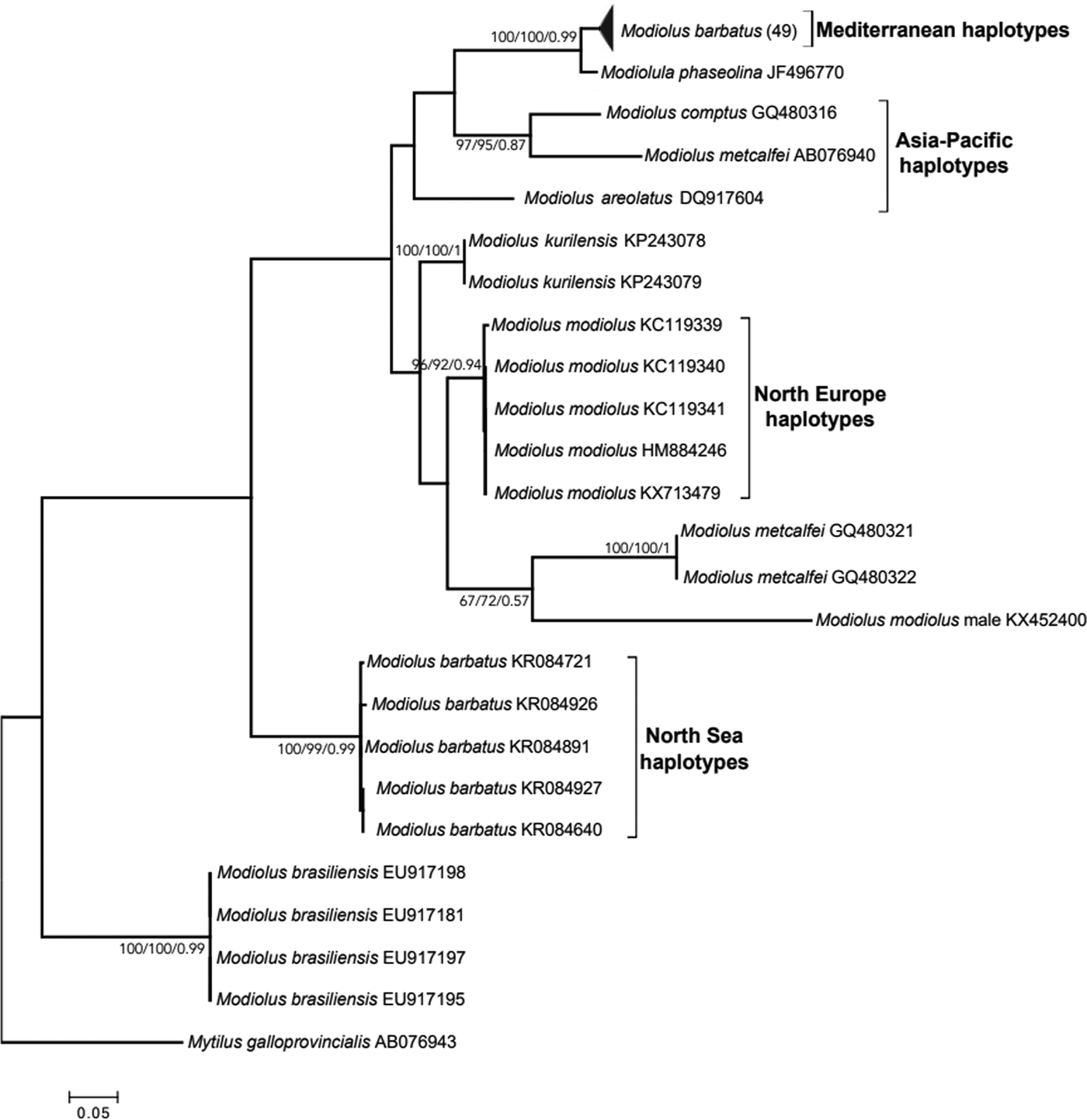
Fig. 2. Phylogeny of COI haplotypes originating from different Modiolus species based on three methods (Maximum Likelihood, Maximum Parsimony and Bayesian). Bootstrap values and posterior probabilities greater than 50% as analysed by 1000 iterations are shown for the main clades as follows: ML/MP/Bayesian.
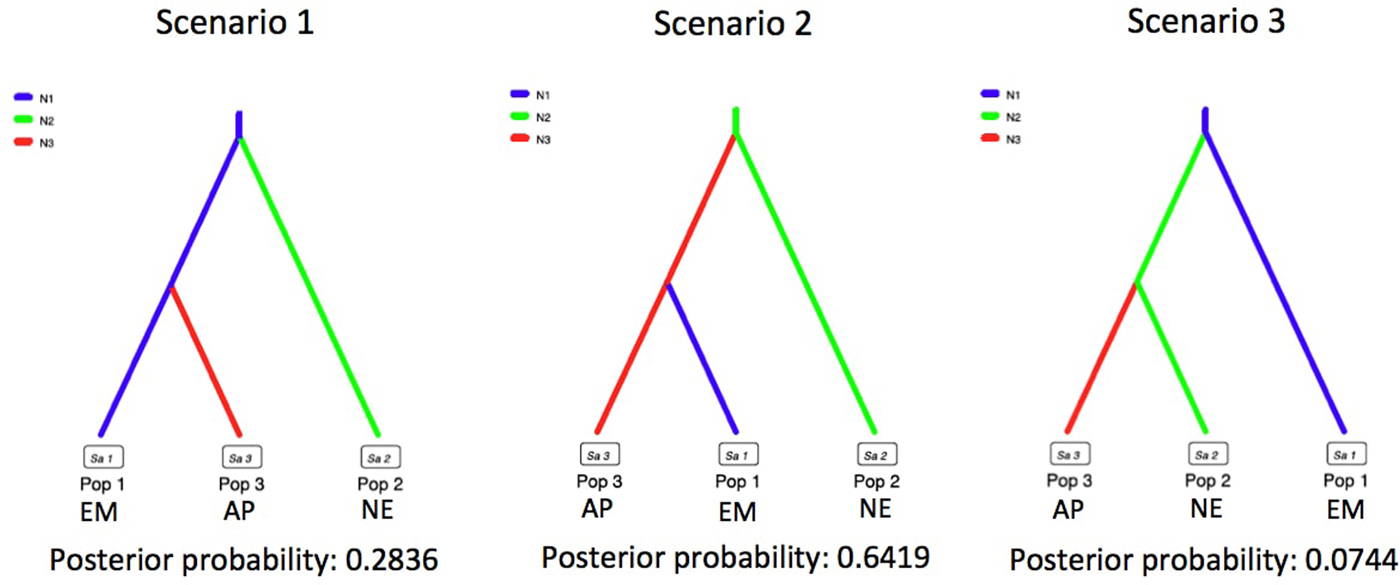
Fig. 3. Approximate Bayesian computation of potential colonization scenarios of horse mussels into the eastern Mediterranean basin. Scenario 1 indicates an independent introduction, whereas scenario 2 and 3 suggest introduction from Asian-Pacific and North Europe, respectively. The most probable scenario is scenario 2. EM, Eastern Mediterranean; AP, Asia-Pacific; NE, North Europe.
The genetic relationships and the mutational steps among the haplotypes are depicted in the network of Figure 4. The most widely distributed haplotype, which was found in individuals from all populations, is connected with several private haplotypes by one or two steps. This star-like pattern indicates that the Mb04 haplotype, holding a relatively central position, is probably the most ancestral one. Several pairs of haplotypes were separated by multiple mutational steps in line with their high nucleotide diversity (Table 1). Furthermore, no exclusive cluster was observed for a specific geographic region.
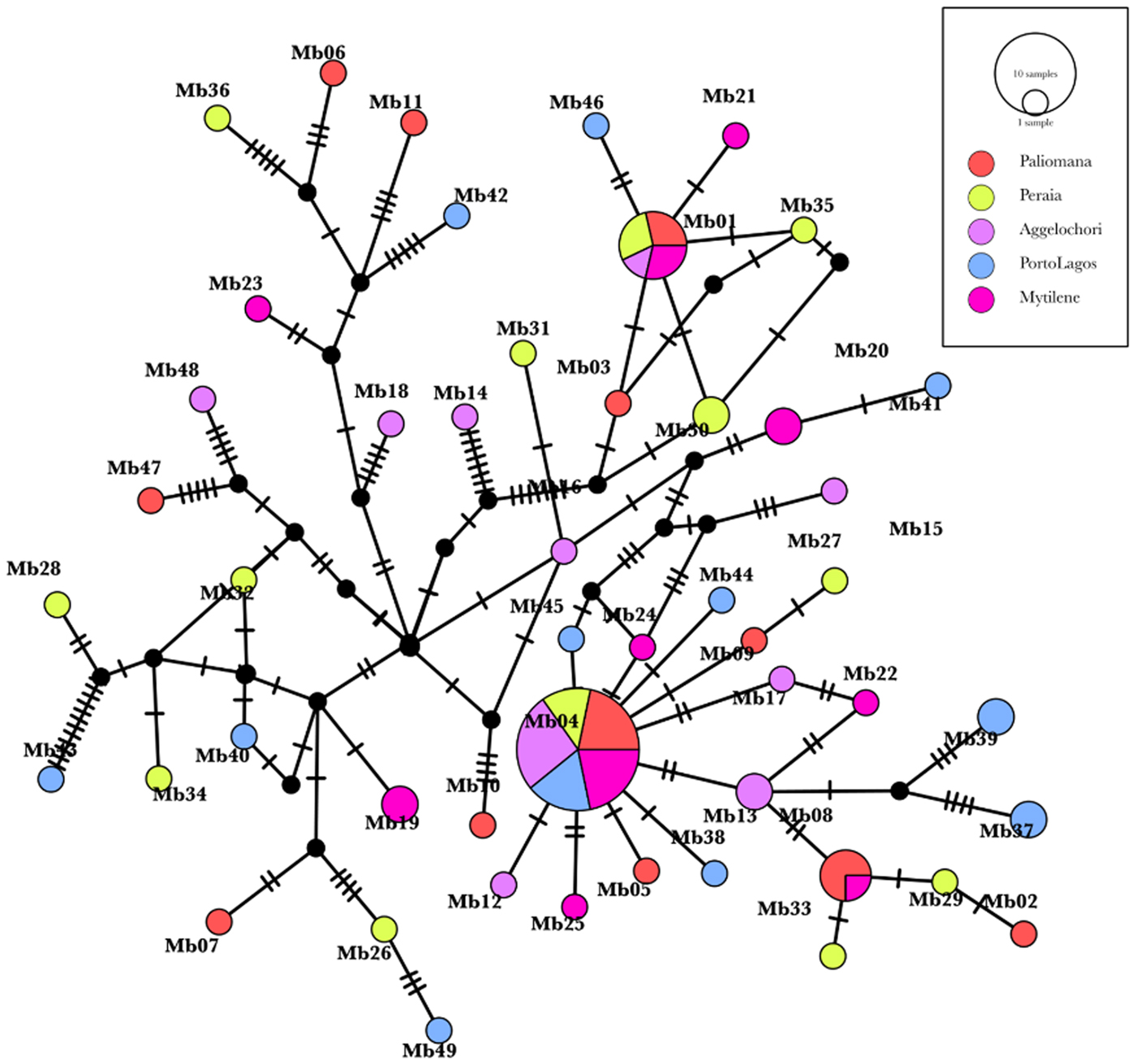
Fig. 4. Median-Joining network of M. barbatus haplotypes. Circle sizes are proportional of the number of times each haplotype occurred. Different colours indicate the different sampling locations. Hatch marks correspond to the number of mutational steps between two haplotypes. Black dots indicate potential missing haplotypes, not sampled in the present study.
Table 1. Genetic diversity parameters and Tajima's neutrality index for each population as well as at species level
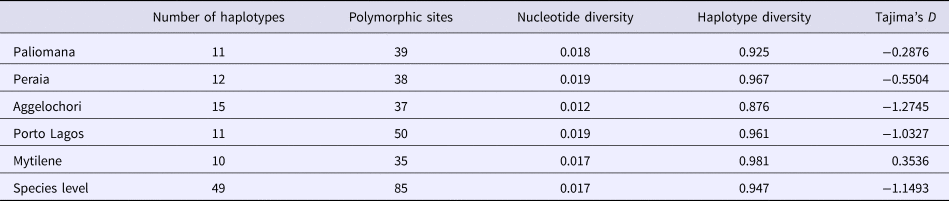
All Tajima's D estimates were non-significant at level P > 0.05.
Genetic diversity indices (haplotype and nucleotide diversity) for each population separately as well as at the species level are listed in Table 1. Haplotype diversity was at high levels in all examined populations, with the population from Aggelochori presenting the lowest genetic diversity. Although negative neutrality test values were found in a few populations, they were not significant (Table 1). These results, in combination with high haplotype diversities, indicate no departures from the assumption of neutrality, and absence of sudden demographic alterations such as population decreases or expansions after potential bottlenecks. Furthermore, generally low levels of population differentiation were observed, with F st values ranging from negative to 0.1812 (Table 2). Apart from differentiation of the population pairs Porto Lagos–Mytilene and Mytilene–Peraia, all comparisons were statistically non-significant.
Table 2. Pairwise genetic differentiation (F st) among the sampled populations

Significant values (P < 0.05) are shown with an asterisk (*).
Discussion
The present study provides the first insights towards the genetic relationships of eastern Mediterranean Modiolus barbatus populations, using sequence analysis of a COI mitochondrial gene segment. While DNA barcoding has evolved as a valuable tool for marine species identification, Barco et al. (Reference Barco, Raupach, Laakmann, Neumann and Knebelsberger2016) reported that more than 90% of estimated living marine species are molecularly undocumented in public databases such as GenBank. To the best of our knowledge, the newly described sequences deposited in the GenBank database under the accession numbers MH277125–MH277173, represent the first DNA barcodes of M. barbatus from the Mediterranean Sea biogeographic basin.
Phylogeography of the eastern Mediterranean horse mussel
Although M. barbatus is known to be distributed in the Mediterranean and neighbouring marine areas, such as the Black Sea and the Moroccan and Iberian Atlantic coasts, it has also been reported in west Ireland and the southern and western coasts of Great Britain (Lok et al., Reference Lok, Acarli, Serdar, Kose and Goulletquer2006). European populations of the congeneric bivalve M. modiolus are believed to span their range throughout the coastline of Europe, except from the Mediterranean and the Black Sea (Halanych et al., Reference Halanych, Vodoti, Sundberg and Dahlgren2013). In the current phylogenetic analysis, while all M. modiolus haplotypes are clustered in the same clade, M. barbatus haplotypes are separated by higher levels of genetic distance than those found for congeneric species. The first branch includes all Aegean haplotypes collected in the current sampling, whereas the second branch includes all previously described M. barbatus haplotypes originating from the North Sea (Figure 2). It is also worth noting that other Modiolus species from the Asian-Pacific region such as M. metcalfei, M. comptus and M. areolatus were found to be phylogenetically more closely related to the eastern Mediterranean haplotypes than to M. barbatus from the North Sea. These inferences indicate that the horse mussel populations from the eastern Mediterranean may not belong to the same species as those from the North Sea. A possible explanation for this puzzling situation could be the potential erroneous nomination of European horse mussel species. Examination of the distribution of M. modiolus as shown in the map of figure 1 in Halanych et al. (Reference Halanych, Vodoti, Sundberg and Dahlgren2013), as well as the reported distribution of M. barbatus in Lok et al. (Reference Lok, Acarli, Serdar, Kose and Goulletquer2006) and the geographic origin of both M. modiolus and M. barbatus haplotypes in the study of Barco et al. (Reference Barco, Raupach, Laakmann, Neumann and Knebelsberger2016), reveals that both species are sympatric in some marine areas of the North Sea. In this case there may be three Modiolus species in Europe, two of which co-occur in northern European coasts and the third in the eastern Mediterranean Sea. This third species, which inhabits the Eastern Mediterranean basin, seems to be phylogenetically more closely related to Modiolula phaseolina from the Adriatic. Alternatively, a potential explanation could be related with the unusual mitochondrial DNA inheritance of marine bivalves. Particularly, in marine bivalves with two mitochondrial genomes, such as Modiolus species (Robicheau et al., Reference Robicheau, Powell, Del Bel, Breton and Stewart2017), phylogeny reconstruction without distinguishing male from female mitochondrial haplotypes could lead to controversial inferences. Nevertheless, the inclusion of a male mitotype that was grouped with neither M. modiolus nor M. barbatus in the dendrogram of Figure 2 clearly rejects this hypothesis. Altogether, although more molecular data are probably needed to resolve the horse mussel's systematic status, COI results clearly indicate that their taxonomic status should be reconstructed.
Based on the depicted results of our phylogenetic analysis (Figure 2), as well as the comparisons of the newly described haplotypes as query sequences in the Basic Local Alignment Search Tool (BLAST) on the NCBI website, the horse mussel from the Aegean Sea is more closely related to the Asian-Pacific Modiolus species than to North European ones and very closely related to Modiolula phaseolina. Based on our results, M. phaseolina seems most likely to belong in the genus Modiolus and to have been misidentified as a different genus. In accordance with phylogenetic analysis, colonization analysis suggested that horse mussels were most probably introduced into the eastern Mediterranean from the Asian-Pacific coasts. However, these inferences may need further verification including more sampling locations, before conclusions can be drawn. A similar pattern has been observed in the southern hemisphere where Mytilus haplotypes were found closely related to the Mediterranean mussel M. galloprovincialis (Gérard et al., Reference Gérard, Bierne, Borsa, Chenuil and Féral2008), supporting an analogous lineage origination. Alternatively, the close relationship of the Mediterranean haplotypes with those from the Indo-Pacific, could be a consequence of migration through the Suez Canal that has to be examined in future studies by analysis of samples from the Red Sea.
However, questions still remain regarding the phylogeographic positioning of western Mediterranean horse mussel populations that would probably clarify the phylogeny and taxonomy of European Modiolus species. On account of the very few available genomic analyses of European horse mussel populations there is a basic lack of information regarding the potential existence of the Siculo-Tunisian genetic break, causing distinction between western and eastern Mediterranean populations in several marine species (Kaouèche et al., Reference Kaouèche, Bahri-Sfar, González-Wangüemert, Pérez-Ruzafa and Ben Hassine2011; Sanna et al., Reference Sanna, Cossu, Dedola, Scarpa, Maltagliati, Castelli and Casu2013; Giantsis et al., Reference Giantsis, Abatzopoulos, Angelidis and Apostolidis2014a). This lack of genomic data may be related to the difficulties in horse mussel sampling, which is only feasible by professional experienced divers and restricted to marine areas that can be reached by them. Thus, future studies should rely on comprehensive samplings from any possible marine area in the western Mediterranean and the Black Sea, in order to convincingly resolve taxonomic aspects of European Modiolus species and reconstruct their phylogeny.
Genetic diversity and population structure
The high levels of intraspecies and intrapopulation genetic diversity observed in M. barbatus from the Aegean Sea, in terms of haplotype and nucleotide diversity (Table 1), is a common feature of wild mytilid populations (Koehn et al., Reference Koehn, Milkman and Mitton1976; Koehn, Reference Koehn and Brussard1978; Giantsis et al., Reference Giantsis, Abatzopoulos, Angelidis and Apostolidis2014a). It is also worth mentioning the great number of 49 haplotypes found in the relatively restricted geographic marine area of the Aegean Sea, in comparison to the 52 derived haplotypes from the wider geographic marine area in the study of Halanych et al. (Reference Halanych, Vodoti, Sundberg and Dahlgren2013), which included samples from North Europe, the Pacific and the Atlantic. These inferences in combination with the high number of private haplotypes in all examined populations, suggest that the eastern Mediterranean marine area is likely the origin of M. barbatus. The high genetic diversity could also be attributed to the large effective population size (Ne), which although in mtDNA is one-quarter of the autosomal (Ladoukakis & Zouros, Reference Ladoukakis and Zouros2017), is still large in species of the family Mytilidae (Lallias et al., Reference Lallias, Stockdale, Boudry, Lapegue and Beaumont2009) and may even reach Ne > 30,000 (Kartavtsev & Nikiforov, Reference Kartavtsev and Nikiforov1993).
Despite the fact that farming of M. barbatus has not been developed yet in the Aegean, it is a promising candidate species for suspended aquaculture, similar to the long-line system of M. galloprovincialis (Lok et al., Reference Lok, Acarli, Serdar, Kose and Goulletquer2006; Peharda et al., Reference Peharda, Ezgeta-Balić, Davenport and Vrgoč2013). Aquaculture activities may occasionally cause harmful effects in farmed bivalve populations, owing to phenomena related to genetic drift or inbreeding (Ye et al., Reference Ye, Li, Wu, Xu and Guo2012). Nevertheless, due to the combination of (a) the lack of artificial breeding in suspended mussels, coupled with (b) the high genetic diversity observed, we do not foresee any negative impact of potential aquaculture activities on the genetic composition of horse mussel populations in the Aegean Sea.
Examination of the population structure of Aegean horse mussel populations revealed low levels of interpopulation genetic differentiation, most of which was statistically non-significant. The generally low levels of population structuring in eastern Mediterranean horse mussels are in accordance with previous studies of Modiolus species (De la Rosa-Velez et al., Reference De La Rosa-Velez, Farfan and Cervantes-Franco2000) and are mostly attributed to the long pelagic larval stage that can reach up to 6 months (Halanych et al., Reference Halanych, Vodoti, Sundberg and Dahlgren2013). During this period, larval translocation depends on waving and sea currents, promoting extensive gene flow. Thus, in contrast to the strong subdivision of populations often observed in bivalves with short larval stages (Ye et al., Reference Ye, Wu and Li2015), genetic homogeneity is an expected phenomenon for horse mussel populations.
Despite the non-significant results, values of genetic differentiation were comparable with, or occasionally even greater than those estimated between pairs of more distant North European populations (Halanych et al., Reference Halanych, Vodoti, Sundberg and Dahlgren2013). Although there was no Single Nucleotide Polymorphism (SNP) to determine a particular geographic location, the high numbers of unique haplotypes may provide an indication for designing the development of other types of molecular markers towards resolving traceability issues. The characterization of the first DNA barcodes from the eastern Mediterranean is of considerable importance in the context of traceability of horse mussels from the eastern Mediterranean basin or from other European coasts. Detection of specific geographic origin is of highlighted importance in horse mussels on account of local rules and policy. Particularly, fisheries and trade of horse mussels within the Aegean Sea are permitted when originating from islands such as Mytilene, while prohibited when originating from Thermaikos Gulf (I.A. Giantsis, pers. comm.), due to the high concentrations in heavy metals there (Katsikatsou et al., Reference Katsikatsou, Anestis, Pörtner, Kampouris and Michaelidis2011). In this sense, a ready-to-use molecular tool for tracing horse mussels’ origin would be extremely useful. To achieve this goal, sequencing of further genes, such as 16S or ITS ribosomal DNA, RADseq methodology or microsatellites would probably constitute promising molecular markers for such utility in mytilids (Larraín et al., Reference Larraín, Díaz, Lamas, Uribe and Araneda2014), in which extensive gene flow is usually observed in wild populations.
Supplementary material
The supplementary material for this article can be found at https://doi.org/10.1017/S0025315418001133.
Financial support
This research did not receive any specific grant from funding agencies in the public, commercial, or not-for-profit sectors.