1. Introduction
Trilobites are one of the most recognized and well-studied Palaeozoic groups. However, despite over 150 years of research and >22 000 described species, the relationships between higher-level groups within this iconic class of fossil arthropods remain unclear (Adrain, Reference Adrain and Zhang2011; Paterson, Reference Paterson2020). Advances in our understanding of the ontogeny of different trilobite groups will help to resolve these problems by providing crucial information for phylogenetic analyses (e.g. Edgecombe et al. Reference Edgecombe, Speyer and Chatterton1988; Fortey & Chatterton, Reference Fortey and Chatterton1988; Chatterton et al. Reference Chatterton, Siveter, Edgecombe and Hunt1990; Chatterton & Speyer, Reference Chatterton, Speyer and Kaesler1997; Hughes et al. Reference Hughes, Hong, Hou and Fusco2017). Determining the relationships between Cambrian trilobites in particular will help to illuminate not only the origins of the class (e.g. by providing information on character polarities; Paterson et al. Reference Paterson, Edgecombe and Lee2019), but also assist in resolving how some post-Cambrian clades are related to Cambrian taxa (the so-called ‘cryptogenesis problem’; see Paterson, Reference Paterson2020). Developmental information from early Cambrian trilobites considered to be ‘primitive’, such as those belonging to the Order Redlichiida, is therefore of high importance.
Within the Redlichiida (specifically the suborder Redlichiina sensu Adrain, Reference Adrain and Zhang2011), instances where multiple, well-preserved protaspides (the earliest biomineralized larval stage) are known is limited to less than 10 species. The most informative of these are phosphatized specimens of the estaingiid Ichangia ichangensis Zhang, Reference Zhang1957 and several indeterminate species described by Zhang & Pratt (Reference Zhang and Pratt1999) and Zhang & Clarkson (Reference Zhang and Clarkson2012). Protaspides from several other ellipsocephaloid species preserved in shale or mudstone are also well known, including the ellipsocephalid Ellipsostrenua granulosa (Ahlberg, Reference Ahlberg1983) (Laibl et al. Reference Laibl, Cederström and Ahlberg2018) and the estaingiid Estaingia sinensis (Zhang, Reference Zhang1953) (Dai & Zhang, Reference Dai and Zhang2012 a). Early ontogenetic information for the other major redlichiine superfamilies Redlichioidea and Paradoxidoidea is more limited, although Laibl et al. (Reference Laibl, Esteve and Fatka2017) described ‘giant’ protaspides of two paradoxidid species from the Miaolingian of the Czech Republic, and Dai & Zhang (Reference Dai and Zhang2012 b) presented well-preserved examples of the redlichiid Metaredlichia cylindrica (Zhang, Reference Zhang1953) from Cambrian Stage 3 of Hubei Province, China. Protaspides are either unknown from other examples of redlichiine ontogenies (e.g. Dai & Zhang, Reference Dai and Zhang2013), or represent relatively poorly preserved or isolated examples that do not provide detailed morphological information of protaspid stages (e.g. Westergård, Reference Westergård1936; Lu, Reference Lu1940; Kautsky, Reference Kautsky1945; Whittington, Reference Whittington1957; Šnajdr, Reference Šnajdr1958; Pocock, Reference Pocock1970; Öpik, Reference Öpik1975; Zhang et al. Reference Zhang, Lu, Zhu, Qian, Lin, Zhao, Zhang and Yuan1980; Pillola, Reference Pillola1991; Palmer & Rowell, Reference Palmer and Rowell1995; Hou et al. Reference Hou, Hughes, Yang, Lan, Zhang and Dominguez2017).
Here we describe a relatively complete post-embryonic ontogenetic series of the trilobite Redlichia cf. versabunda Öpik, Reference Öpik1970 from the Cambrian Series 2 (late Stage 4) Ramsay Limestone of Yorke Peninsula (South Australia), including some of the best-preserved redlichioid protaspides known to date.
2. Geological setting
The Ramsay Limestone on Yorke Peninsula, and its temporal equivalents the Wirrealpa and Aroona Creek limestones (Flinders Ranges), form part of the second major depositional sequence of the Cambrian succession in the Stansbury and Arrowie basins (as defined by Gravestock, Reference Gravestock, Drexel and Preiss1995; Gravestock & Shergold, Reference Gravestock, Shergold, Zhuravlev and Riding2001), representing relatively warm, shallow-marine environments during the last major Cambrian transgression in South Australia. These units are considered contemporaneous based on the co-occurrence of Daily’s (Reference Daily and Rodgers1956) ‘Faunal Assemblage 10’ (e.g. Horwitz & Daily, Reference Horwitz, Daily, Glaessner and Parkin1958; Daily, Reference Daily, Jago and Moore1990; Brock & Cooper, Reference Brock and Cooper1993).
The Ramsay Limestone is known from central Yorke Peninsula in the Stansbury Basin (Fig. 1a), where it crops out in a small area c. 7 km south of Curramulka as ‘dark, blue-grey, mottled, argillaceous limestone’ (Daily, Reference Daily, Jago and Moore1990, p. 223), as well as in the subsurface from a number of drill holes in the general vicinity (Fig. 1b). It has a maximum thickness of 85 m in the Stansbury West-1 well, within which Daily (Reference Daily, Jago and Moore1990) reported shelly fossils (including Redlichia) from oolitic, sandy and dolomitic limestones c. 15 m below the top of the formation. The Ramsay Limestone is 68.6 m thick in the Stansbury Town-1 well, although only a 3-m-section in the upper part of the formation was cored, consisting of interbedded grey, nodular, argillaceous limestone and black, pyritic, micaceous siltstone and/or mudstone containing Redlichia and other shelly fossils (B Daily, unpub. report, 1968; Daily, Reference Daily, Jago and Moore1990; Gravestock et al. Reference Gravestock, Alexander, Demidenko, Esakova, Holmer, Jago, Lin, Melnikova, Parkhaev, Rozanov, Ushatinskaya, Zang, Zhegallo and Zhuravlev2001). Lower portions of the Ramsay Limestone were also intersected by the Minlaton-1, Minlaton-2 and Cur-D1B drill holes, with the formation represented in these by dark- to light-grey, mottled, nodular limestones interbedded variously with shales, siltstones, planar stromatolites and evaporites (Ludbrook, Reference Ludbrook1965; Daily, Reference Daily, Jago and Moore1990; Gravestock et al. Reference Gravestock, Alexander, Demidenko, Esakova, Holmer, Jago, Lin, Melnikova, Parkhaev, Rozanov, Ushatinskaya, Zang, Zhegallo and Zhuravlev2001). Redlichia was reported by B Daily (unpub. report, 1957) from the basal c. 25 m of the formation in Minlaton-1. The Ramsay Limestone conformably overlies conglomerates, arkoses and evaporites of the Minlaton Formation and conformably underlies the shales, siltstones and calcareous sandstones of the Corrodgery Formation (Fig. 2) (Daily, Reference Daily, Jago and Moore1990; Gravestock et al. Reference Gravestock, Alexander, Demidenko, Esakova, Holmer, Jago, Lin, Melnikova, Parkhaev, Rozanov, Ushatinskaya, Zang, Zhegallo and Zhuravlev2001).

Fig. 1. (a) Map of southeastern South Australia showing the location of the Stansbury and Arrowie basins and the study area. (b) Map of central Yorke Peninsula showing the locality within the Ramsay Limestone where material considered in this study was collected (pentagon), as well as the locations of several drill holes that intersect the formation in the vicinity (stars).

Fig. 2. Correlation chart showing relationships between the Cambrian successions of the Stansbury Basin (Yorke Peninsula), Arrowie Basin and eastern Georgina Basin (Undilla Sub-basin). The dates in the Billy Creek Formation (1) and Hawker Group (2–4, all within the Mernmerna Formation) refer to 206U–238Pb chemical-abrasion thermal-ionization mass spectrometry (CA-TIMS) ages published by Betts et al. (Reference Betts, Paterson, Jacquet, Andrew, Hall, Jago, Jagodzinski, Preiss, Crowley, Brougham, Mathewson, García-Bellido, Topper, Skovsted and Brock2018). Correlations based mainly on those of Jago et al. (Reference Jago, Gehling, Paterson, Brock and Zang2012), Smith et al. (Reference Smith, Carr, Edwards, Hall, Kelman, Laurie, le Poidevin and Nicoll2013) and Jago & Kruse (Reference Jago and Kruse2020).
3. Occurrence of Redlichia in the Ramsay and Wirrealpa limestones
Trilobites within the upper part of the Cambrian succession in South Australia (i.e. above the first major depositional sequence of Gravestock, Reference Gravestock, Drexel and Preiss1995 and Gravestock & Shergold, Reference Gravestock, Shergold, Zhuravlev and Riding2001) are rare. Only three instances in three successive formations are currently recognized from the Arrowie Basin in the Flinders Ranges (Jago et al. Reference Jago, Zang, Sun, Brock, Paterson and Skovsted2006, Reference Jago, Gehling, Betts, Brock, Dalgarno, García-Bellido, Haslett, Jacquet, Kruse, Langsford, Mount and Paterson2020): the emuellid Balcoracania dailyi Pocock, Reference Pocock1970 from the Billy Creek Formation (Paterson & Edgecombe, Reference Paterson and Edgecombe2006; Paterson et al. Reference Paterson, Jago, Brock and Gehling2007); ‘Redlichia guizhouensis’ (re-assigned here to R. cf. versabunda) from the Wirrealpa Limestone and equivalent Aroona Creek Limestone (Daily, Reference Daily, Thomson, Daily, Coats and Forbes1976; Jell in Bengtson et al. Reference Bengtson, Conway Morris, Cooper, Jell and Runnegar1990; Jago & Zang, Reference Jago and Zang2006; Paterson & Brock, Reference Paterson and Brock2007); and Onaraspis rubra Jell in Bengtson et al. Reference Bengtson, Conway Morris, Cooper, Jell and Runnegar1990 from the Moodlatana Formation (Fig. 2). In the Stansbury Basin on Yorke Peninsula, Redlichia has been reported from the Ramsay and Stansbury limestones (e.g. Öpik, Reference Öpik1970; Daily, Reference Daily, Jago and Moore1990), and Pagetia cf. edura has been documented from the Coobowie Formation in the Port Julia-1A drill hole, the youngest trilobite known from the Stansbury and Arrowie basins and suggestive of a Wuliuan age (see Jago & Kruse, Reference Jago and Kruse2020).
Redlichia was first reported (as Olenellus sp.) in South Australia from the Wirrealpa Limestone by Etheridge (Reference Etheridge1905). Daily (Reference Daily and Rodgers1956) noted Redlichia aff. nobilis as part of his ‘Faunal Assemblage 10’ from the Wirrealpa and Aroona Creek limestones. Öpik (Reference Öpik1970, p. 7) reported ‘undescribed forms […] with delicate ornaments of lines and granules, reminiscent in this aspect of Redlichia versabunda’ from both the Wirrealpa and Ramsay limestones (he referred to the latter as the Wirrealpa Limestone), and Daily (Reference Daily1982, p. 60) reported a species ‘allied to R. versabunda’ from the Wirrealpa Limestone at the western end of Brachina Gorge in the Flinders Ranges. Jell in Bengtson et al. (Reference Bengtson, Conway Morris, Cooper, Jell and Runnegar1990) subsequently described Redlichia guizhouensis Zhao in Lu et al. Reference Lu, Zhang, Qian, Zhu, Lin, Zhou, Qian, Zhang and Yuan1974 from the Wirrealpa Limestone c. 7 km NW of the Wirrealpa homestead, and Paterson & Brock (Reference Paterson and Brock2007) described fragmentary silicified specimens of the same species from the Wirrealpa Limestone at Balcoracana Creek on the eastern side of the Bunkers Range. There have been various reports of Redlichia from core samples of the Ramsay Limestone (see above) and, in his brief description of the formation, Daily (Reference Daily, Jago and Moore1990) mentioned the presence of Redlichia aff. nobilis in outcrop of the Ramsay Limestone south of Curramulka on Yorke Peninsula. Given the morphological similarities between R. versabunda, R. nobilis and R. guizhouensis, it is almost certain that all of these reports refer to the same taxon (the latter two species are considered as possible synonyms: Jell in Zhang, Reference Zhang1985; Bengtson et al. Reference Bengtson, Conway Morris, Cooper, Jell and Runnegar1990; Zhang, Reference Zhang, Zhang, Chen and Palmer2003).
Previously, the presence of ‘R. guizhouensis’ in the Wirrealpa Limestone has allowed correlation with the R. nobilis (= R. guizhouensis) Zone of the Lungwangmiaoan Stage (= upper Duyunian) of China (Bengtson et al. Reference Bengtson, Conway Morris, Cooper, Jell and Runnegar1990; Brock & Cooper, Reference Brock and Cooper1993; Paterson & Brock, Reference Paterson and Brock2007). The reassignment of this species to R. cf. versabunda suggests correlation with the slightly older Redlichia chinensis Zone, a species it apparently co-occurs with in northern Australia (see discussion below).
4. Materials and methods
All specimens documented here are housed in the South Australian Museum, Adelaide (specimen number prefix SAM P). Most specimens from the Ramsay Limestone considered here (SAM P57716–57794) were collected and prepared by Mr Brent Bowman from a locality c. 7 km SSW of Curramulka (34° 45′ 25″ S, 137° 42′ 50″ E), from float at the edge of a farm track adjacent to poorly exposed Ramsay Limestone. Specimen SAM P57795 was collected by JD Holmes from float in a quarry c. 200 m WSW of the original locality (34° 45′ 27″ S, 137° 42′ 40″ E).
Specimens were prepared using a pneumatic percussive needle and then whitened with ammonium chloride sublimate using the method set out by Teichert (Reference Teichert1948). External moulds were cast in latex before whitening and photography. Large specimens were photographed using a Canon EOS 5DS digital SLR camera with a Canon MP-E 65 mm 1:2.8 macro lens, a Cognisys Stackshot 3X stacking system and the Canon EOS Utility software. Small specimens were photographed using an Olympus SZX7 binocular microscope with an Olympus SC50 camera attachment and the Olympus cellSens Standard v.1.17 software, and stacked using the HeliconFocus v.7.5.4 Pro software. Measurements of specimens were taken using ImageJ v.2.0.0 (Schneider et al. Reference Schneider, Rasband and Eliceiri2012).
Morphological terminology generally follows Whittington & Kelly (Reference Whittington, Kelly and Kaesler1997); for a discussion of ontogenetic terminology, see Section 6 below. For clarity, informal use of higher-level taxonomic names are as follows: ‘redlichiine’ for Suborder Redlichiina, ‘redlichioid’ for Superfamily Redlichioidea and ‘redlichiid’ for Family Redlichiidae.
5. Systematic palaeontology
Class Trilobita Walch, Reference Walch1771
Order Redlichiida Richter, Reference Richter, Dittler, Joos, Korschelt, Linek, Oltmanns and Schaum1932
Suborder Redlichiina Richter, Reference Richter, Dittler, Joos, Korschelt, Linek, Oltmanns and Schaum1932
Superfamily Redlichioidea Poulsen, Reference Poulsen1927
Family Redlichiidae Poulsen, Reference Poulsen1927
Genus Redlichia Cossmann, Reference Cossmann1902
Type species. Hoeferia noetlingi (Redlich, Reference Redlich1899) from lower Cambrian strata of the Salt Range, in what is now Pakistan.
Discussion. In two monographs, Öpik (Reference Öpik1958, Reference Öpik1970) reviewed the concept of this genus based largely on Redlichia forresti (Etheridge in Foord, Reference Foord1890) from the Ord Basin of Western Australia and the Northern Territory (Öpik, Reference Öpik1958), and addressed the taxonomy of Redlichia species in northern Australia (Öpik, Reference Öpik1970). In doing so, he recognized four previously described species – R. forresti, R. idonea Whitehouse, Reference Whitehouse1939, R. venulosa (Whitehouse, Reference Whitehouse1939) and R. chinensis Walcott, Reference Walcott1905 – and erected nine new species. Many of these new species were based on small numbers of incomplete specimens, and in some cases questionable interpretations; for example, R. amadeana was based partly on the presence of bacculae, but re-examination of material assigned to this taxon showed no evidence of these cranidial structures (Kruse, Reference Kruse1998; Laurie in Kruse et al. Reference Kruse, Laurie and Webby2004). As such, there is suspicion that Öpik (Reference Öpik1970) ‘oversplit’ Australian occurrences of this genus, and his specimens are in need of review (Laurie in Kruse et al. Reference Kruse, Laurie and Webby2004).
Öpik (Reference Öpik1970) recognized 10 species of Redlichia from the Thorntonia Limestone in western Queensland (including seven new species), with seven species being reported from a c. 2 m section of shale and siltstone at a single locality (M426); it is likely that a number of these are conspecific. Many of Öpik’s new species were diagnosed on minor differences between isolated cranidia, and it is now recognized that there can be considerable intraspecific variation within trilobite species, including those of Redlichia. For example, Laurie in Kruse et al. (Reference Kruse, Laurie and Webby2004) noted significant variation in the expression of the axial furrow and the continuity of the glabella with the fixigenae in specimens of R. forresti from the Ord Basin; see Laurie (Reference Laurie2016) for further discussion. This was originally considered a diagnostic character of Mesodema, which has since been synonymized with Redlichia (Öpik, Reference Öpik1958, Reference Öpik1970). Such variation also occurs among specimens preserved in limestone with minimal taphonomic distortion; for example, the cranidial width/length ratio of R. cf. versabunda from the Ramsay Limestone described here varies from 105 to 127% and is seemingly unrelated to ontogeny. Likewise, the recognition of two distinct types of ornament in the one species documented here (pustulose in smaller, and Bertillon in larger specimens) has not been previously described in other Redlichia occurrences from the one locality.
Redlichia cf. versabunda Öpik, Reference Öpik1970
Figs 3–6
1905 Olenellus sp. Etheridge, p. 247, pl. 25, fig. 1.
1919 Olenellus? sp. Etheridge, p. 382, pl. 39, fig. 1.
1956 Redlichia aff. nobilis Daily, p. 115, 121, 132, not figured.
cf. 1970 Redlichia versabunda Öpik, p. 27, pl. 9, figs 1–5.
cf. 1970 Redlichia lepta Öpik, p. 32, fig. 4, pl. 11, figs 3–5, pl. 12, figs 1–5.
1990 Redlichia guizhouensis Zhou; Jell in Bengtson et al., p. 267, fig. 179.
2007 Redlichia guizhouensis Zhou; Paterson & Brock, p. 124, fig. 6.
Material. 80 specimens, including 31 protaspides, 30 cranidia (mostly meraspides and small holaspides), two librigenae, eight hypostomes and nine pygidia.
Occurrence. Ramsay Limestone (Yorke Peninsula), Wirrealpa Limestone and Aroona Creek Limestone (Flinders Ranges), South Australia.
Description. The following description applies to larger holaspid specimens unless otherwise stated; for comparison with smaller specimens, see Section 6 below.
Cranidium highly convex; anterior cranidial width (tr.) averaging 113% of total length (sag.). Anterior margin moderately curved. Anterior branches of facial sutures (γ-β) sub-horizontal (tr.) and slightly bowed towards posterior; distal section (β-α) initially angling posterolaterally away from axis, curving sharply forwards to angle slightly adaxially at margin. Glabella tapering moderately towards anterior, highly convex (sag., tr.), approximately 70–75% as wide (tr.) across anterior of eye ridges as across occipital lobe. Frontal lobe well rounded, almost truncate in some specimens (e.g. Fig. 3e). Axial furrows generally straight, moderately well developed, extending to shallow preglabellar furrow. Glabellar furrows generally continuous, although often hard to see axially. S1 continuous, moderately curved towards posterior, less well developed axially than laterally, with deeper pits close to axial furrow. S2 gently curved posteriorly, poorly developed but usually discernible for about two-thirds distance from axial furrow to axis; continuous in well-preserved specimens but very poorly developed axially. S3 generally straight (tr.), very poorly developed, more obvious in certain specimens (e.g. Fig. 3d, e). Occipital furrow (SO) continuous, shallow and straight axially, well developed and angling slightly forwards laterally. Occipital ring large, sub-triangular, angling slightly upwards posteriorly and terminating in axial node. Frontal area length (sag.) approximately 20% that of cranidium. Very short (sag.) preglabellar field in the largest specimen (2% cranidial length: Fig. 3i), longer in smaller holaspides (c. 3–7% cranidial length: e.g. Fig. 3a, b). Distinct plectrum with medial depression; plectrum width (tr.) approximately half that of frontal glabellar lobe. Pre-ocular field moderately convex, steeply downsloping to narrow, well-developed anterior border furrow. Anterior border wide (sag., exsag.), usually moderately convex (sag., exsag.); width (sag.) 10–18% cranidial length in holaspides, slightly wider axially; border of the largest specimen is very wide (c. 18%) and highly convex (Fig. 3i). A line of pits along the posterior extremity of the border is clearly visible in this specimen (Fig. 3i); these pits are also present in smaller holaspides, but not as well developed (e.g. Fig. 3g). Palpebro-ocular ridge wide, crescentic, anterior margin meeting axial furrow adjacent to S3, posterior margin adjacent to rear half of occipital ring and very close to axial furrow. Palpebral area moderately convex, widest (tr.) at about level of L1 or S1, separated from palpebro-ocular ridge by wide, shallow palpebral furrow posteriorly, becoming narrower and deeper anteriorly. Maximum width (tr.) across palpebral lobes approximately equal to cranidial length (sag.). Posterolateral projections not known, but presumably small and narrow (exsag.) based on the librigenae.

Fig. 3. Redlichia cf. versabunda holaspid cranidia from the Ramsay Limestone (Yorke Peninsula, South Australia): (a) SAM P57723; (b) SAM P57720; (c) SAM P57724; (d) anterolateral oblique view of SAM P57722; (e) SAM P57722; (f) anterolateral oblique view of SAM P57717; (g) SAM P57712; (h) SAM P57795; and (i) SAM P57716. Specimens are arranged in order of size, from smallest (top left) to largest (bottom right). Note the transition from pustulose ornament in smaller specimens to Bertillon ornament in the largest two specimens. Scale bars: (a–g) 1 mm; (h, i) 3 mm.
Hypostome subquadrate, anterior margin not fully known, but appears to be gently curved; anterior wings not preserved in available material. The posterolateral margin bears a pair of large, laterally projecting posterior wings, and a more posterior pair of short spines. In the smallest specimen (Fig. 4g), the posterior wings are wider (tr.) and the posterior spines much longer (sag.). The lateral margin between anterior and posterior wings is gently concave (where known), as is that between posterior wings and spines. Posterior margin gently posteriorly curved axially. Middle body ovate, divided into anterior and posterior lobes by shallow middle furrow; anterior lobe ovate, highly convex (sag., tr.), c. 65% total length (sag.) of hypostome; posterior lobe sub-semicircular, smaller, c. 20% hypostomal length (sag.). Lateral and posterior borders wide near posterior wings/spines, narrower elsewhere. Maculae present as effaced areas in lateral sections of middle furrow (e.g. Fig. 4i). Large specimens covered in Bertillon ornament (except in the vicinity of middle furrow), transitioning to terrace lines on border (Fig. 4i); smaller specimens have a less obvious, pustulose ornament (Fig. 4h). Rostral plate not known.

Fig. 4. Redlichia cf. versabunda specimens from the Ramsay Limestone (Yorke Peninsula, South Australia): (a) left librigena (SAM P57792); (b) right librigena (SAM P57793); (c) large pygidium (SAM P57794); (d) small holaspid pygidium (SAM P57783); (e) very small holaspid(?) pygidium (SAM P57780); (f) small holaspid pygidium; arrow denotes the level where a faint furrow is present, delineating the second axial ring (SAM P57784); (g) mid-stage(?) meraspid hypostome with extended posterolateral spine (SAM P57772); (h) medium-sized hypostome (SAM P57774); and (i) large hypostome; note distinct Bertillon ornament in this large specimen compared with pustulose ornament in (h) (SAM P57775). Scale bars: (a–d, i) 1 mm; (e) 200 μm; (f–h) 400 μm.
The two known librigenae are quite small (Fig. 4a, b: 7–8 mm in length). Moderately curved lateral margin extending to long, curved, gently tapering, strongly advanced genal spine, c. 65% total librigenal length (exsag., including spine). High eye socle, separated from genal field by shallow eye socle furrow. Genal field crescentic, highly convex, widest at mid-point (exsag.), downsloping to broad, shallow lateral border furrow. Border relatively flat, widest (tr.) at midpoint of genal field (exsag.) near base of genal spine (c. 80–90% maximum genal field width). Genal field with close-packed, pustulose ornament extending on to border and morphing to terrace lines marginally.
Pygidium subquadrate, c. 75% as long (sag.) as wide (tr.) in large specimens (exc. articulating half-ring), c. 65% as long as wide in smaller specimens (Fig. 4c–f). Anterior margin straight to gently curved axially, becoming more curved at anterolateral corners. Lateral margins gently bowed outwards; posterior margin with slight medial embayment in large specimens (Fig. 4c). Axial region ovoid in shape, with two short (sag.) axial rings and a terminal axial piece. Anteriormost axial ring clearly defined, the second clearly visible laterally, less so axially; second ring poorly defined in smaller specimens. Terminal axial piece subtriangular and bilobate, as delineated by posteromedial crease. Axial rings and terminal axial piece separated by poorly developed axial ring furrows. Pleural area comprising one moderately well-defined anterior pleura with clear pleural furrow that wraps around axis to point posteriorly. Terminal area wide, c. 75% pygidial width (tr.), and very long, about one-third pygidial length (sag.).
Remarks. South Australian specimens assignable to this species have most recently been described as Redlichia guizhouensis from the Wirrealpa Limestone of the Flinders Ranges (see Jell in Bengtson et al. Reference Bengtson, Conway Morris, Cooper, Jell and Runnegar1990; Paterson & Brock, Reference Paterson and Brock2007), although their similarity to R. nobilis or R. versabunda has been noted by a number of authors (e.g. Daily, Reference Daily and Rodgers1956, Reference Daily1982; Öpik, Reference Öpik1968). Redlichia guizhouensis is a species originally described from China, based on a single cranidium, and no other material has since been referred to this taxon other than the abovementioned specimens from the Wirrealpa Limestone. It has been suggested previously that R. guizhouensis may be synonymous with R. nobilis (Jell in Zhang, Reference Zhang1985; Bengtson et al. Reference Bengtson, Conway Morris, Cooper, Jell and Runnegar1990; Zhang, Reference Zhang, Zhang, Chen and Palmer2003). Cranidia from the Ramsay and Wirrealpa limestones are similar to the type specimen of R. guizhouensis from China in some respects: they have an anterior cranidial width equal to c. 110% of the cranidial length, relatively wide palpebral lobes and a wide, convex anterior border. However, the single specimen of R. guizhouensis from China (see Lu et al. Reference Lu, Zhang, Qian, Zhu, Lin, Zhou, Qian, Zhang and Yuan1974, pl. 31, fig. 10) has a narrower, less conical glabella, and is much narrower across the palpebral area (tr. width c. 87% that of cranidial length, compared to subequal in cranidia from the Ramsay and Wirrealpa limestones). Given the probable synonymy of R. guizhouensis and R. nobilis, and the general lack of distinguishing features of the former, assignment of Australian material to R. guizhouensis is not supported by the available evidence.
Specimens from the Ramsay and Wirrealpa limestones resemble R. nobilis in having similar cranidial proportions (anterior cranidial width c. 110% of length), as well as being posteriorly curved and having strongly divergent anterior branches of the facial sutures. However, R. nobilis has a less conical glabella (similar to R. guizhouensis) and narrower palpebral lobes (see Zhang & Jell, Reference Zhang and Jell1987, pl. 5, figs 5, 6), and also differs with regard to pygidial morphology (as pointed out by Jell in Bengtson et al. Reference Bengtson, Conway Morris, Cooper, Jell and Runnegar1990); the pygidium of R. nobilis is more ovoid and shorter (sag.), with a much shorter terminal area ending in an arched posterior margin (see Zhang & Jell, Reference Zhang and Jell1987, pl. 7, fig. 4). Jell (in Bengtson et al. Reference Bengtson, Conway Morris, Cooper, Jell and Runnegar1990) cautioned that this may be a result of dimorphism, as noted by Öpik (Reference Öpik1958) for R. forresti; this is the only published example of such variation in Redlichia. Öpik (Reference Öpik1958) interpreted this variation as sexual dimorphism and rejected an ontogenetic explanation (for no apparent reason), despite the fact that the unusual ‘male’ pygidium occurred only in very small specimens. Moreover, neither of the two specimens he figured appear to have the full complement of 18 thoracic segments (Öpik, Reference Öpik1958, pl. 2, figs 1–3). In fact, the pygidia of these specimens appear similar to the transitional meraspid pygidia of certain other redlichiines, for example, those of Estaingia bilobata Pocock, Reference Pocock1964 from the Emu Bay Shale, South Australia (Holmes et al. Reference Holmes, Paterson and García-Bellido2020 b).
Redlichia versabunda was first described by Öpik (Reference Öpik1970) from two localities in western Queensland that he considered to be the Beetle Creek Formation – the ‘Yelvertoft Bed’ near Yelvertoft Homestead (M426) and a locality west of Mt Isa on May Downs Station (M262) – as well as from the Thorntonia Limestone at a locality further to the south (D41). All three localities are now considered to be within or equivalent to the ‘lower’ Thorntonia Limestone (sometimes referred to as the Hay River Formation; Smith et al. Reference Smith, Carr, Edwards, Hall, Kelman, Laurie, le Poidevin and Nicoll2013) (Fig. 2). Our comparison of the Ramsay Limestone specimens with R. versabunda from western Queensland is based on close similarities to the material originally assigned to that taxon by Öpik (Reference Öpik1970), as well as certain other co-occurring species he erected, in particular, Redlichia lepta. The specimens from the Ramsay Limestone conform to Öpik’s (Reference Öpik1970) diagnosis for R. versabunda in having: (1) an anterior cranidial width (tr.) of c. 110% of the cranidial length (sag.); (2) ‘slightly curved and strongly divergent’ anterior branches of the facial sutures (seemingly more divergent in the Ramsay specimens, although this is possibly due to differences in preservation); (3) an anterior border furrow with small pits in the lateral portions that is interrupted by a plectrum (or ‘swelling reminiscent of a plectrum’) in the axial region; (4) relatively wide palpebral lobes extending close to the axial furrow at the rear of the occipital ring; (5) Bertillon ornament on the glabella, with more pustulose ornament on the interocular cheeks and palpebral lobes (as seen in the largest two specimens from the Ramsay Limestone; Fig. 3h, i); and (6) a ‘trapezoidal’ or subtriangular occipital ring with a medial node (Öpik, Reference Öpik1970, p. 28). Small (but probable holaspid) specimens from the Ramsay Limestone (Fig. 3a–g) also display the above characteristics, except that they have dense pustulose ornament (weakly aligned in slightly larger specimens, e.g. Fig. 3d–g) in place of the Bertillon ornament of large specimens, and they have a longer (sag.) preglabellar field (and therefore plectrum) that relates to ontogeny (see Section 6 below). The Ramsay Limestone specimens are also very similar to figured specimens assigned to R. lepta by Öpik (Reference Öpik1970), a species he described from the Thorntonia Limestone (and co-occurring with R. versabunda and R. chinensis). Specimens assigned by Öpik (Reference Öpik1970) to R. versabunda have cranidial lengths of 12.8–14.0 mm, while those of R. lepta range over 3.6–8.0 mm. The morphological differences between these ‘species’ therefore correspond to the ontogenetic variation seen in specimens from the Ramsay Limestone. As such, we suggest that R. versabunda and R. lepta are conspecific, with R. versabunda being the senior synonym described first in Öpik’s (Reference Öpik1970) monograph. It is possible that other species co-occurring with R. versabunda in the Thorntonia Limestone are also conspecific. For example, Öpik (Reference Öpik1970) erected Redlichia mayalis from locality M262 (where it co-occurs with R. versabunda), based on two cranidia with bluntly rounded frontal glabellar lobes, similar to certain specimens from the Ramsay Limestone (e.g. Fig. 3e). Redlichia vertumnia (based on two cranidia from M262) is also similar. Given the uncertain validity of many Redlichia species from the Thorntonia Limestone (and Öpik’s Australian species in general), plus the absence of illustrated pygidia of R. versabunda or R. lepta from the Georgina Basin, we feel it is best to place the South Australian specimens under open nomenclature until a review of Öpik’s (Reference Öpik1958, Reference Öpik1970) material is completed and/or new specimens from the type localities are documented.
Previously, the Wirrealpa Limestone (Arrowie Basin) has been correlated with the Redlichia nobilis Zone of the (upper) Lungwangmiaoan Stage (= upper Duyunian) of China, based largely on the presence of ‘R. guizhouensis’ (Jell in Bengtson et al. Reference Bengtson, Conway Morris, Cooper, Jell and Runnegar1990; Paterson & Brock, Reference Paterson and Brock2007). Other Australian formations such as the Ramsay Limestone (Stansbury Basin), Tindall Limestone (Daly Basin) and Montejinni Limestone (Wiso Basin), as well as the Gum Ridge Formation, Thorntonia Limestone and Top Springs Limestone in the Georgina Basin, are also considered contemporaneous with the Wirrealpa Limestone, based on co-occurring shelly taxa such as the linguliformean brachiopods Kostjubella djagoran (Kruse, Reference Kruse1990) and Schizopholis napuru (Kruse, Reference Kruse1990) (Brock & Cooper, Reference Brock and Cooper1993; Gravestock et al. Reference Gravestock, Alexander, Demidenko, Esakova, Holmer, Jago, Lin, Melnikova, Parkhaev, Rozanov, Ushatinskaya, Zang, Zhegallo and Zhuravlev2001; Paterson & Brock, Reference Paterson and Brock2007; Percival & Kruse, Reference Percival and Kruse2014; Popov et al. Reference Popov, Holmer, Hughes, Ghobadi Pour and Myrow2015). The close comparison of Redlichia specimens from the Ramsay and Wirrealpa limestones with those of R. versabunda (and R. lepta) from the Georgina Basin would suggest correlation with the slightly older Redlichia chinensis Zone of China, equivalent to the mid-Lungwangmiaoan (upper Duyunian), based on the co-occurrence of R. versabunda and R. lepta with R. chinensis in the Thorntonia Limestone (Öpik, Reference Öpik1970). However, Öpik’s (Reference Öpik1970) identification of R. chinensis from Australian localities has been questioned by Kruse et al. (Reference Kruse, Laurie and Webby2004, p. 19), given the small number of illustrated specimens. As such, and given the uncertainty surrounding the specimens from the Ramsay and Wirrealpa limestones being conspecific with R. versabunda (and R. lepta) from northern Australia, this correlation remains tentative.
6. Post-embryonic development of Redlichia cf. versabunda
6.a. Ontogenetic terminology
As with holaspid morphology, ontogenetic terms generally follow Whittington & Kelly (Reference Whittington, Kelly and Kaesler1997), except we use ‘trunk’ instead of ‘protopygidium’ for protaspides (after Hughes et al. Reference Hughes, Minelli and Fusco2006). We use ‘intergenal spine separation’ in the sense of Laibl et al. (Reference Laibl, Cederström and Ahlberg2018, after Webster, Reference Webster2007) to refer to the distance between the posterior fixigenal (or ‘intergenal’) spines of protaspides.
The protaspid period has previously been subdivided into substages (e.g. anaprotaspis, metaprotaspis) based on the appearance of a distinct trunk and a furrow separating this from the cephalon. We follow the recommendation of Chatterton & Speyer (Reference Chatterton, Speyer and Kaesler1997) and Edgecombe et al. (Reference Edgecombe, Speyer and Chatterton1988) in avoiding these terms, as they likely do not represent homologous stages across all trilobites. It should be noted that this argument may also apply to the broader protaspid, meraspid and holaspid periods defined above; however, these are widely used and useful for discussion and descriptive purposes.
6.b. Protaspid period
Two stages can be recognized in protaspides of Redlichia cf. versabunda from the Ramsay Limestone, namely, an early stage with no visible trunk and a later stage with a distinct trunk; these stages form two distinct size clusters (Fig. 7). In early protaspides (Fig. 5a–g), the exoskeleton is ovoid and moderately convex, ranging over 0.56–0.62 mm in length and 0.63–0.72 mm in width (averaging 117% as wide as long). The axis is composed of five segments: the occipital ring, three glabellar lobes (L1–L3) and the frontal lobe (LA). The glabella is raised slightly above the surrounding exoskeleton in profile view, gently expanding anteriorly from SO to S3 with an inflated, truncate, slightly bilobed frontal lobe almost reaching the anterior margin, approximately twice the width of the occipital lobe. A shallow, narrow medial furrow divides glabellar lobes L1–LA. The axial furrows are wide, shallow and slightly bowed outwards, separated from a very shallow preglabellar/border furrow (= change in slope) by a wide (sag., tr.), relatively deep fossula. The glabellar furrows are wide (sag.) and continuous (tr.), as is the occipital furrow. The anterior border is narrow and not well defined. The palpebro-ocular ridge is crescentic and gently convex, extending laterally from the posterior of the frontal lobe, then curving towards the posterior, reaching a level just anterior of S1. The fixigenal area is large, approximately the same width as the glabella at its widest point (about level with L2); it is semicircular, highly convex, downsloping, and bounded laterally by a broad palpebral furrow (= change of slope), which extends rearwards to a less obvious posterior border furrow. There appears to be slight expression of the segmental boundaries defined by the glabellar furrows in the fixigenal area (Fig. 5a–d). Bacculae are present as small protrusions laterally adjacent to the anterior of the occipital ring, separated from the glabella by the axial furrows. A small, laterally directed anterior fixigenal spine is present just behind the posterior branch of the facial suture (Fig. 5a, e, f). The posterior margin bears two large, broad (tr.) fixigenal (‘intergenal’) spines or protrusions, of length (sag.) approximately equal to that of the occipital lobe in the longest specimens (Fig. 5f, g). The posterolateral margin between the anterior and posterior fixigenal spines is gently rounded and appears to bear at least one additional fixigenal spine (Fig. 5f); the border in this region widens towards the posterior. The posterior border behind the occipital ring is very short (sag.), with the margin slightly protruding posteromedially. Librigenae are not known, but appear to have been small, crescentic and laterally orientated.

Fig. 5. Redlichia cf. versabunda protaspides from the Ramsay Limestone (Yorke Peninsula, South Australia). (a–g) Early-stage protaspides with no discernible trunk and (h–n) late-stage protaspides with distinct trunk: (a) SAM P57752; (b) SAM P57753; (c) SAM P57754; (d) SAM P57756; (e) anterolateral oblique view of SAM P57752 showing anterior fixigenal spine (arrow); (f) posterior detail of SAM P57752; note anterior and posterolateral fixigenal spines (arrows); (g) posterior view of SAM P57751, showing details of posterior fixigenal spines; (h) SAM P57765; (i) SAM P57741; (j) SAM P57768; (k) SAM P57746; (l) SAM P57750; (m) posterior view of SAM P57750, showing details of trunk and posterior fixigenal spines; and (n) anterolateral view of SAM P57750. All scale bars: 200 μm.
Later-stage protaspides (Fig. 5h–n) range over 0.66–0.78 mm in length and 0.68–0.83 mm in width (averaging 115% as wide as long), and are similar to early-stage examples, with the following exceptions: the exoskeleton is less convex and the glabella narrower (tr.) along its length, with the axial furrows close to parallel and more developed, and the medial furrow less obvious; the fixigenal area is wider (tr.), bacculae are less obvious and the palpebral lobes are longer (exsag.), reaching to about the level of S1 or L1; a very short (sag.) preglabellar field is present and the anterior border is more clearly defined; the posterior fixigenal (intergenal) spines are much longer and more pointed, and the intergenal spine separation wider (Figs 5l, m, 7), resulting in the posterior part of the exoskeleton becoming less rounded; and a distinct trunk is present, the clearest examples of which appear to display two axial segments and a pair of pleural spines, separated from the cephalon by a narrow, well-defined furrow, but without a distinct articulation (i.e. the anterolateral margins of the trunk appear ‘fused’ to the cephalon) (Fig. 5m).
6.c. Meraspid period
Meraspid cranidia range from 0.86 mm to c. 4 mm in length (Fig. 6). Due to the disarticulated nature of the specimens, it is difficult to determine the exact point of the meraspid/holaspid transition. Nevertheless, cranidia larger than c. 4 mm in length (sag.) show very minimal allometric change, suggesting that these probably belong to holaspides or very late-stage meraspides. The morphology of Redlichia cf. versabunda meraspides from the Ramsay Limestone spans a continuum of variation between that of the protaspides and holaspides described above; the major changes across this period are summarized below.

Fig. 6. Redlichia cf. versabunda meraspides from the Ramsay Limestone (Yorke Peninsula, South Australia). Specimens are arranged in order of size, from smallest ((a), top left) to largest ((l), bottom right): (a) SAM P57730; (b) SAM P57739; (c) SAM P57734; (d) SAM P57727; (e) SAM P57733; (f) SAM P57738; (g) SAM P57735; (h) SAM P57736; (i) SAM P57740; (j) SAM P57726; (k) SAM P57728; and (l) SAM P57731. Note the initial retraction of the glabella and development of a distinct preglabellar field with plectrum in smaller meraspides, followed by the extension of the glabella and reduction of the preglabellar field in later stages. Across the meraspid period the glabella also changes shape from clavate to conical. Scale bars: (a–f) 200 μm; (g–l) 500 μm.
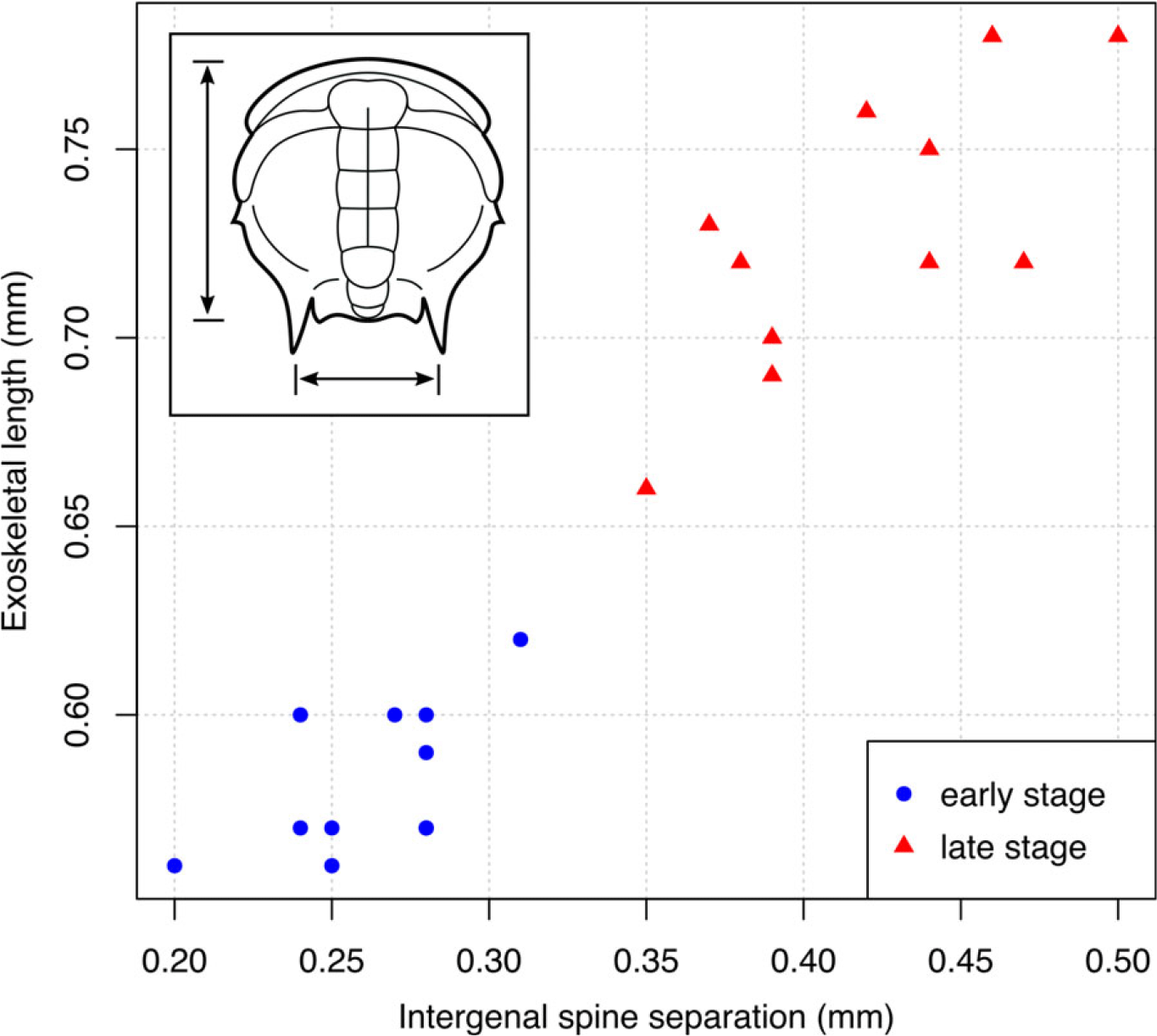
Fig. 7. Plot of axial exoskeletal length against intergenal spine separation for Redlichia cf. versabunda protaspides from the Ramsay Limestone. Intergenal spine separation represents the distance between posterior fixigenal spine bases that will become the intergenal spines/angles. Blue circles represent early-stage protaspides with no visible trunk, and red triangles represent late-stage protaspides with a distinct trunk; these groups are clearly separated based on these size measures. See inset for visual of measurement definitions.
Early meraspid cranidia are sub-quadrate with later stages becoming more ovoid. In the smallest meraspides, the glabella tapers slightly from LO to L3, with an inflated frontal lobe that is slightly wider than the occipital lobe (Figs 6a, 8c); the frontal lobe has a subtle medial depression that in slightly later stages develops into a distinctly bilobed frontal lobe (Figs 6c, d, 8d), which gradually disappears about midway through the meraspid period as the glabella shortens and a distinct preglabellar field with plectrum develops (Figs 6e–h, 8e). In later-stage meraspides, the glabella widens considerably and gradually lengthens again, becoming more tapered and conical in shape (Figs 6i–l, 8f, g). The preglabellar field decreases in length, becoming very short in holaspides (Figs 3, 8h). In early meraspides, the glabellar furrows are continuous but weakly impressed medially (Fig. 6a–f); the occipital furrow is continuous and well developed; these gradually become less well developed during ontogeny (e.g. Fig. 6g–l). A distinct medial node is present on the occipital ring in well-preserved specimens from the early meraspid period onwards.

Fig. 8. Reconstructions of various ontogenetic stages of Redlichia cf. versabunda from the Ramsay Limestone (Yorke Peninsula, South Australia): (a) early-stage protaspis; (b) late-stage protaspis; (c–g) meraspid stages in order of increasing size; and (h) holaspis. Dashed lines represent uncertain morphology.
In early meraspides, the palpebro-ocular ridge extends laterally from the posterior of LA (with which it is partly continuous, separated by a weakly impressed axial furrow), curving towards the posterior, extending to about the level of S1 or L1 (exsag.); across the meraspid period, this becomes more isolated from the glabella at the anterior, and extends further towards the posterior of the cranidium and curves adaxially, with the posterior extremity being close to the axial furrow near the occipital ring in holaspides. Across the same period, the fixigenal area gradually narrows (tr.), the anterior border widens (sag., exsag.) and the anterior branches of the facial sutures gradually lengthen (tr.). The posterior fixigenal (intergenal) spines present in protaspides shorten dramatically in the earliest meraspides and presumably disappear, and the intergenal spine separation widens considerably.
The smallest known hypostome from the Ramsay Limestone is 1.36 mm long, and therefore likely belongs to a middle- to late-stage meraspis of R. cf. versabunda based on size comparisons with the cranidia. It is similar in morphology to adult hypostomes, except the posterior wings are wider and the single posterior spine preserved is much longer (Fig. 4g).
The smallest pygidium is 0.38 mm long, and exhibits a single obvious axial ring and a bilobed terminal piece (Fig. 4e); morphology and size suggest this is possibly from a very early holaspis. The terminal area is much shorter (sag.) than in late holaspides, with a shallow but obvious medial embayment. Pygidia likely belonging to slightly larger holaspides (e.g. Fig. 4d, f) have terminal areas intermediate in length between this and those of late holaspid pygidia (Fig. 4c). Holmes et al. (Reference Holmes, Paterson and García-Bellido2020 a) showed that holaspides of Redlichia takooensis Lu, Reference Lu1950 from the Cambrian Series 2 (Stage 4) Emu Bay Shale of South Australia have a pygidium with two axial rings and bilobed terminal piece, pointing out that the second ring is often faint and difficult to discern. This also seems to be the case in R. cf. versabunda.
7. Discussion
7.a. Morphological comparisons with other redlichiine protaspides
In most known cases, protaspides of redlichiine trilobites have similar morphologies overall. Early-stage protaspides are generally circular to ovoid and display an anteriorly expanding axis composed of five segments (LO–LA), with the anterior lobe being distinctly wider than L3 and often bilobed. The eye ridges extend laterally from the posterior of the frontal lobe (with which they appear partly continuous, separated by a weakly developed axial furrow) before curving towards the posterior, with the palpebral lobe reaching about exoskeletal mid-length (exsag.). The fixigenae are semicircular and convex, with the posterior border furrow curving anterolaterally from near the occipital ring to meet the posterior of the palpebral lobe. In well-preserved specimens, bacculae can be discerned at the posterior of the fixigenae, at about the level of the occipital furrow. Distinct pits (or fossulae) are present in the angle created by the junction of the eye ridge and the frontal lobe. There is a distinct gap between the glabellar frontal lobe and the anterior cephalic margin that Laibl et al. (Reference Laibl, Cederström and Ahlberg2018) called a ‘preglabellar field’ in Ellipsostrenua granulosa. In reality, this gap most likely represents a poorly defined anterior border (although there is a short but distinct preglabellar field in later protaspides of R. cf. versabunda). The librigenae appear to have been small, very narrow (tr.) and laterally (or anterolaterally) orientated. In most cases, a pair of small, laterally directed fixigenal spines are present just behind the posterior facial suture, with a second pair of ventrally orientated posterior fixigenal (or ‘intergenal’) spines present along the posterior margin. Many of these similarities were noted by Laibl et al. (Reference Laibl, Cederström and Ahlberg2018) in comparing early protaspides of the ellipsocephaloids Ichangia ichangensis, Estaingia bilobata and E. granulosa; however, this general description also applies to redlichioid protaspides such as Metaredlichia cylindrica (Dai & Zhang, Reference Dai and Zhang2012 b). Protaspides of the paradoxidid Acadoparadoxides pinus are also similar (Westergård, Reference Westergård1936; Whittington, Reference Whittington1957); however, related species such as Eccaparadoxides pusillus and Hydrocephalus carens (despite displaying similar features in general) have very large protaspides with greatly enlarged glabellas that were interpreted as possible instances of lecithotrophy by Laibl et al. (Reference Laibl, Esteve and Fatka2017). Excluding minor allometric changes, later-stage redlichiine protaspides are comparable to early stages, the major difference being the appearance of a trunk region with at least one pair of pleural spines.
There are currently very few redlichioid trilobites for which protaspid morphology is known in detail. Some of the best examples are those of Metaredlichia cylindrica figured by Dai & Zhang (Reference Dai and Zhang2012 b) from the Shuijingtuo Formation in the Hubei Province of China, who described a relatively complete post-embryonic ontogeny of this species based on protaspides and isolated cranidia. They also suggested that the superbly phosphatized specimens of ‘genus and species indeterminate 1’ of Zhang & Pratt (Reference Zhang and Pratt1999) from the Shuigoukou Formation (Henan Province, China) may be conspecific with M. cylindrica. This suggestion is strengthened by the identification of additional specimens of ‘genus and species indeterminate 1’ of Zhang & Pratt (Reference Zhang and Pratt1999) in the Shuijingtuo Formation of Shaanxi Province (China) by Zhang & Clarkson (Reference Zhang and Clarkson2012).
Protaspides of R. cf. versabunda from the Ramsay Limestone closely resemble those of M. cylindrica (and the possibly conspecific ‘genus and species indeterminate 1’ of Zhang & Pratt, Reference Zhang and Pratt1999). These species exhibit the standard morphology discussed above, although they differ in minor ways. The glabella of M. cylindrica has more divergent axial furrows and potentially longer posterior fixigenal spines in early protaspides compared with R. cf. versabunda, although these spines may be incomplete in available specimens of the latter species. The overall shape of the exoskeleton is also slightly different, with M. cylindrica appearing to be slightly longer (sag.) relative to width (tr.), but this may be a result of preservation in shale compared with limestone (see discussion below about overall size).
These redlichiid species also share several additional characteristics that are not shared across all redlichiine groups. Firstly, they exhibit a longitudinal medial glabellar furrow that subdivides the anterior glabellar lobes (L1–LA). Such a feature is shared with other redlichioids (where known), such as Dolerolenus zoppii (Meneghini, Reference Meneghini1882) (Pillola, Reference Pillola1991), and is also present in paradoxidoids (Westergård, Reference Westergård1936; Whittington, Reference Whittington1957; Šnajdr, Reference Šnajdr1958), but is apparently absent in ellipsocephaloids (e.g. Zhang & Pratt, Reference Zhang and Pratt1999; Laibl et al. Reference Laibl, Cederström and Ahlberg2018).
Secondly, these redlichiid protaspides bear additional paired fixigenal spines, situated between the anterior and posterior pairs, and orientated posterolaterally. Dai & Zhang (Reference Dai and Zhang2012 b) identified one such pair in M. cylindrica, while Zhang & Clarkson (Reference Zhang and Clarkson2012) figured phosphatized specimens of ‘genus and species indeterminate 1’ (Zhang & Pratt, Reference Zhang and Pratt1999; tentatively assigned to M. cylindrica by Dai & Zhang, Reference Dai and Zhang2012 b) that clearly show a second additional pair close to the posterior fixigenal spines. It appears at least one additional pair of spines is present in R. cf. versabunda from the Ramsay Limestone (although these are not very clear; Figs 5e, f, 8a), probably corresponding to those identified in M. cylindrica (Dai & Zhang, Reference Dai and Zhang2012 b, figs 1.10, 1.11, 4.1–4.3) and the more anterior pair in ‘genus and species indeterminate 1’ (Zhang & Clarkson, Reference Zhang and Clarkson2012, pl. 19, figs 4–7). These additional spines are also apparently absent in ellipsocephaloids (e.g. Zhang & Pratt, Reference Zhang and Pratt1999; Laibl et al. Reference Laibl, Cederström and Ahlberg2018).
Finally, in R. cf. versabunda protaspides, the segmental boundaries of the cranidium indicated by the glabellar furrows appear to extend into the fixigenal area, a trait shared with other redlichioids and emuelloids (and also observed in olenelline meraspides), but lacking in paradoxidoids (including xystridurids) and ellipsocephaloids (see Paterson & Edgecombe, Reference Paterson and Edgecombe2006).
7.b. Size comparisons with other redlichioid protaspides
Dai & Zhang (Reference Dai and Zhang2012 b) noted two size clusters of early-stage protaspides lacking a trunk in Metaredlichia cylindrica (their ‘anaprotaspides’, ranging over both 0.50–0.54 and 0.70–0.81 mm in length), as well as two size clusters of later stage protaspides (their ‘metaprotaspides’, ranging over both 0.73–0.78 and 0.82–0.96 mm in length), which they interpreted as having one and two trunk segments, respectively. In this instance, the larger ‘anaprotaspid’ cluster overlapped substantially with the smaller ‘metaprotaspid’ cluster. Zhang & Pratt (Reference Zhang and Pratt1999) recognized three stages of early protaspides of their ‘genus and species indeterminate 1’ (all lacking a visible trunk): their ‘P0a’ (c. 0.35 mm in length), ‘P0b’ (c. 0.38 mm in length) and ‘P0c’ (c. 0.57 mm in length) stages, as well as a later-stage protaspis with two axial rings in the trunk (their ‘P2’ stage: 0.62–0.75 mm in length). Additional specimens assigned to this taxon by Zhang & Clarkson (Reference Zhang and Clarkson2012) identified an additional late-stage protaspis with a single axial ring (their ‘P1’ stage), although no measurements were listed and only non-dorsal images provided. The size discrepancy between the different stage clusters of these taxa suggest that they may belong to different species, contra Dai & Zhang (Reference Dai and Zhang2012 b). This is not surprising given the apparent overall similarity between redlichioid protaspides, although in this case the size difference discussed above may be partly related to compression in mudstone compared with three-dimensional phosphatization.
Both Dai & Zhang (Reference Dai and Zhang2012 b) and Zhang & Pratt (Reference Zhang and Pratt1999) suggested that in the earliest protaspid stages of M. cylindrica and ‘genus and species indeterminate 1’, the ‘mid-fixigenal’ spines (i.e. those between the anterior and posterior spines) were absent, although this may be related to preservation. Based on size and morphology, it appears that the two protaspid stages of R. cf. versabunda recognized from the Ramsay Limestone most closely resemble the ‘P0c’ and ‘P2’ stages of ‘genus and species indeterminate 1’ of Zhang & Pratt (Reference Zhang and Pratt1999).
7.c. Allometric change and throughout ontogeny
During the course of ontogeny, cranidia of Redlichia cf. versabunda from the Ramsay Limestone underwent a significant amount of allometric change (Fig. 8), particularly in relation to the glabella. In protaspides, particularly in the early stage, the glabella is long, reaching close to the anterior margin, and expanding towards the anterior. Throughout the early meraspid period, the glabella shortens relative to the length of the cranidium and develops a conical shape, tapering towards the anterior. At the same time, a distinct preglabellar field with a medial plectrum develops. In the later meraspid period, the glabella extends again but maintains a conical shape, and the preglabellar field reduces until it is extremely short in holaspides. This pattern of glabella retraction and subsequent extension across ontogeny is common across a number of Cambrian trilobite groups, including olenellines, redlichiids, xystridurids and paradoxidids (see McNamara, Reference McNamara1986). Interestingly, Metaredlichia cylindrica does not follow this pattern; rather, the glabella retains its clavate shape throughout ontogeny, with a short preglabellar field developing in holaspides; there is no retraction and subsequent extension of the glabella during the meraspid period. This is likely a paedomorphic trait, with ancestral juvenile glabellar morphology retained throughout development.
7.d. Phylogenetic implications
Redlichiid protaspides such as those of Redlichia cf. versabunda and Metaredlichia cylindrica are characterized by a longitudinal medial glabellar furrow, three or four pairs of fixigenal spines, and the degree of dorsal cephalic segmentation evident in the glabellar furrows continuing onto the fixigenal area. This last trait is also present in certain olenelloid meraspides (e.g. Nephrolenellus geniculatus; Webster, Reference Webster2007), as well as many other redlichiine protaspides such as the gigantopygid Zhangshania typica (Li et al. Reference Li, Kang and Zhang1990), the emuellids Balcoracania dailyi and Emuella polymera (Paterson & Edgecombe, Reference Paterson and Edgecombe2006) and several redlichioid taxa (see Paterson et al. Reference Paterson, Edgecombe and Lee2019). This trait is likely to be plesiomorphic for Trilobita, being present in a wide range of early Cambrian taxa, but is apparently lost in the more derived ellipsocephaloids and paradoxidoids (Paterson et al. Reference Paterson, Edgecombe and Lee2019). The presence of several fixigenal spines in redlichiid protaspides may also be plesiomorphic, as this morphology is possibly associated with the expression of dorsal segmentation in the cephalon, as indicated by the presence of transverse furrows in the fixigenal area (noted above). Unfortunately, detailed information on protaspides from more basal groups (e.g. emuellids) is lacking, so the polarity of this character cannot be easily established; however, it is important to note that the protaspides of more derived ellipsocephaloids display a reduced number of fixigenal spines (e.g. Zhang & Pratt, Reference Zhang and Pratt1999; Dai & Zhang, Reference Dai and Zhang2012 a; Laibl et al. Reference Laibl, Cederström and Ahlberg2018; Holmes et al. Reference Holmes, Paterson and García-Bellido2020 b). The longitudinal medial glabellar furrow is present in some redlichioid and paradoxidoid protaspides, but is absent in ellipsocephaloids, emuellids and many other Cambrian taxa, suggesting this trait is homoplasious (Paterson et al. Reference Paterson, Edgecombe and Lee2019). The retraction and subsequent extension of the glabella observed throughout the ontogeny of R. cf. versabunda is probably a plesiomorphic trait, being present in many early Cambrian trilobites, including some of the earliest known taxa (e.g. Fallotaspis: McNamara, Reference McNamara1986). Non-retraction of the glabella, or retraction without subsequent re-extension, has been suggested to reflect derived paedomorphic states (e.g. Hupé, Reference Hupé1953; McNamara, Reference McNamara1986).
Acknowledgements
We thank Brent Bowman for collection and preparation of the specimens described in this contribution. We are grateful to Lukáš Laibl and an anonymous referee for their helpful reviews. This research was supported by an Australian Government Research Training Scheme (RTS) scholarship and the Constance Fraser Supplementary Scholarship from the University of Adelaide to JDH.
Declaration of interest
None.