Introduction
Salinity stress is considered one of the most significant abiotic stresses, affecting various aspects of metabolism and physiology. To understand how algae respond and adapt to salinity changes, biochemical and physiological responses have been studied (Kirst, Reference Kirst1990). Information about the effects of salinity stress on induction of oxidative stress is limited in algae (Jahnke & White, Reference Jahnke and White2003; Liu et al., Reference Liu, Au, Anderson, Lam and Wu2007). Adapting to salinity in various ways, algae are divided into two groups according to their tolerance as halophytic (requiring salt for optimum growth) and halo-tolerant (having response mechanisms allowing them to live in saline environments) (Rao et al., Reference Rao, Dayananda, Sarada, Shamala and Ravishankar2007). As a unicellular photoautotroph halo-tolerant green algae, Dunaliella lacks a rigid cell wall. Furthermore, it can store many important organic compounds such as β-carotene, glycerol and others (Ghetti et al., Reference Ghetti, Herrmann, Häder and Seidlitz1999). Additionally, Dunaliella responds to salt stress by high accumulation of glycerol and certain proteins as well as enhanced elimination of Na+ ions (Pick, Reference Pick, Läuchli and Lüttge2002). However, the function and physiological role of glycerol may be different in each Dunaliella species. Moreover, it seems to function as an osmotic regulator such that cells without walls should maintain iso-osmotic conditions in their environment (Hadi et al., Reference Hadi, Shariati and Afsharzadeh2008). Following a change of external salinity, a basic biochemical response is observed at the internal glycerol level, changing the external salinity directly (Ben-Amotz, Reference Ben-Amotz1975, Chitlaru & Pick, Reference Chitlaru and Pick1991). Carotenoids (astaxanthin and lutein) act as complementary pigments in the harvesting complex and as protective agents against the active oxygen products (AOS) formed from photo-oxidation. They are also an integral part of the photosynthetic apparatus in the algae. These oxygen radicals can react to macromolecules and lead to cellular damage (Malanga et al., Reference Malanga, Calmanovici and Puntarulo1997). The algae have developed defensive systems against photo-oxidative damage and can remove these highly reactive oxygen species by antioxidative mechanisms. These antioxidant defensive systems consist of hydrophobic (carotenoids and α-tocopherol) and hydrophilic antioxidants (ascorbic acid and glutathione) and antioxidant enzymes such as superoxide dismutase (SOD), catalase (CAT), glutathione reductase (GR), ascorbic peroxidase (APX) and peroxidase (POD) (Malanga et al., Reference Malanga, Calmanovici and Puntarulo1997; Rijstenbil, Reference Rijstenbil2002; Rao et al., Reference Rao, Dayananda, Sarada, Shamala and Ravishankar2007). In previous reports, it was mentioned that D. salina elevated the activities of the antioxidant enzymes and accumulated a large amount of carotenoids, α-tocopherol and ascorbic acid when grown in a culture medium containing high salt concentration and/or limiting nitrogen (El-Baz et al., Reference El-Baz, Aboul-Enein, El-Baroty, Youssef and Abd El-Baky2002).
Due to interest in a homogenous genetic background, some studies conducted in recent decades compared polyploids and their diploid relatives for tolerance to environmental stressors. Polyploidy is caused by doubling chromosomes of a single species (autopolyploidy) or the hybrids between two species (allopolyploidy). Polyploids clearly show higher resistance to drought, heat, cold, salt, viruses, fungi and pest stressors compared with their diploid relatives (Zhang et al., Reference Zhang, Hu and Yao2010). Therefore, polyploidy has been considered an effective way to increase the resistance to environmental stresses in plants, playing important roles in agriculture and forestry (Xiong et al., Reference Xiong, Li and Zhang2006). Polyploids also show variation in physiological functions or gene expressions. These alterations might affect stress responses and phenotypess (Wang et al., Reference Wang, Wang, Liu and Meng2013). Ectocarpus siliculosus has an alternation of haploid gametophytes and diploid heterozygous sporophyte, showing a moderate tendency toward heteromorphism (Muller, Reference Muller1975). Differences in responses to salt stress between gametophytes and sporophytes originating from the same parent plants were confirmed. The sporophytes are osmotically more robust than gametophytes, having higher salt tolerance than their corresponding gametophytes. These differences are the result of differing physiological tolerance to environmental conditions between diploid and haploid plants. There is some evidence that the variation in the salt tolerances between E. siliculosus gametophytes and sporophytes may not result from obvious differences in ploidy level alone (Thomas & Kirst, Reference Thomas and Kirst1991).
Comparative cytochemical studies of untreated and colchicine-treated cells of algae (Chlamydomonas) illustrated an enhancement in the content of starch, protein and lipid materials. The data also suggest that the enlarged walls of treated cells are composed of a complex polysaccharide (Walne, Reference Walne1966). The aim of this work was to study the effect of polyploidy on many important metabolites, increasing of carotenoids under polyploidy and also the antioxidant enzymatic system of D. salina when grown in a medium containing different levels of NaCl.
Materials and methods
Growth conditions
Dunaliella salina was isolated (Sharma et al., Reference Sharma, Agarwal, Mohan, Kachhwaha and Kothari2012) from the Salt River of Shahdad (30°24′16.164′′N 57°40′57.828′′E) in Kerman, Iran and identified based on physiological and morphological descriptions in the references cited in Massyuk (Reference Massyuk1973c) and Borowitzka & Siva (Reference Borowitzka and Siva2007). After transferring the samples to the laboratory, EC (33.5 ds cm−1) and pH (7.75) of water were measured. Algae were cultured on the agar plate. After 2–3 weeks, each colony was transferred to 20 ml liquid growth medium (Artificial Seawater, ASW) with NaCl added to obtain the required salinity medium at 2 M (pH 7.5) (Raja et al., Reference Raja, Iswarya, Balasubramanyam and Rengasamy2007). The cultures were incubated in a growth chamber under 16/8 h light-dark provided by cool white fluorescent lamps at an intensity of 49 µmol photons m−2 s−1 at 25 ± 2°C, and were shaken manually twice a day to ensure a uniform illumination of the cells. The stock cultures were sub-cultured at least once per month.
Polyploidy induction by colchicine
Haploid vegetative cells of D. salina were exposed to concentrations of 0.1 and 0.5% colchicine (Sigma-Aldrich), made in culture solution for 36 h. After passing these times, algae cells were washed completely two or three times with culture solution to free algae from alkaloid traces (Sarma, Reference Sarma1957) and then were finally transferred to a sterile fresh medium for 21 days. Afterward, the treated cells (0.5 × 103 cells per μl) with colchicine were counted by cell counter. The fresh cultures containing 1, 2, 3 and 4 molar NaCl with 3 replications per treatment were provided and inoculated with 10 ml (5 × 106 cells) stock cultures of every colchicine treatment (0, 0.1 and 0.5%). The various treated samples were allowed to grow under the same conditions as control samples. At the end of 3 weeks, all samples were centrifuged and the pellets obtained were then frozen and stored at −70°C prior to analysis.
Flow cytometry analysis
Ploidy level of the cells of D. salina treated with different colchicine concentrations at different time of exposure was determined by BD FACS Calibur cell sorting system flow cytometry (FCM) (USA) equipped with two lasers. First, the cells were harvested by centrifuging at 3000 rpm for 5 min. After discarding the supernatant, the obtained pellet was resuspended in a specific lysis buffer (300 µl) of kit connected to a flow cytometry device with high resolution DNA analysis of nuclei optimal for some plant species and microorganisms. This kit has two steps: disaggregation and propidium iodide staining. Nuclear suspensions were filtered through a 50 µm nylon filter and 5 µl of propidium idoid 0.1 mg ml−1 (PI, Sigma, USA) added to each sample. A maximum cell concentration of 500,000 cells ml−1 was accepted for each culture for staining. All processes must operate on ice and samples must also be maintained on ice until analysis by FCM. Histograms were analysed using the internal software of FCM (BD FAC Station data processing system), determining peak position and the relative ploidy index of the samples (Galbraith et al., Reference Galbraith, Harkins and Maddox1983).
Analytical methods
Biomass was determined by filtering 20 ml of algal culture through a pre-weighed Whatman GF/C filter. The filter with algae was dried overnight at 60°C in a hot air oven and weighed again to estimate the final dry weight. To obtain fresh weight, the Whatman filter was wetted with culture medium then weighed. After filtering 20 ml algal culture by vacuum pump, the wetted filter with fresh biomass was weighed again.
The amounts of chlorophyll a, chlorophyll b, total chlorophyll and carotenoids were measured spectrophotometrically as described by Lichtenthaler (Reference Lichtenthaler1987).
Total soluble carbohydrates and starch were analysed according to the methods employed by Roe (Reference Roe1955) and Thayumanavan & Sadasivam (Reference Thayumanavan and Sadasivam1984). Proteins were extracted according to Bradford (Reference Bradford1976) spectrophotometrically.
To determine glycerol content, 4 ml of the growth culture of D. salina was centrifuged at 1500 rpm for 10 min at room temperature, and then the precipitated pellets were washed twice in a solution of 1.5 M NaCl and 5 mM phosphate buffer at pH 7.5. One ml of periodate reagent and 2.5 ml of acetyl acetone reagent was added to 200 µl of precipitated pellets. The mixture was incubated at 45°C for 20 min. After that, optical density was determined at 410 nm. Results were compared to the prepared standard curve using the known amount of glycerol (Chitlaru & Pick, Reference Chitlaru and Pick1991).
The level of lipid peroxidation was determined by estimating malondialdehyde (MDA) and other aldehydes content using thiobarbituric acid (TBA) as the reactive material following the method employed by Heath & Packer (Reference Heath and Packer1968).
Enzyme assay
Enzymes assayed in this work were extracted by grinding 0.15 g of fresh algae in a porcelain mortar contain 1.5 ml phosphate buffer containing 50 mM (pH 7.5) ethylene diamine tetraacetic acid (EDTA), 1 mM phenyl methanesulfonyl fluoride (PMSF) and polyvinylpyrrolidone (PVP) 1%. The extract was centrifuged for 15 min at 4°C at 14,000g and the supernatant was assayed for enzymatic activity and quantification of protein by the Bradford method (1976). All operations were performed at 4°C. The activity of superoxide dismutase (SOD) (EC 1.15.1.1), catalase (CAT) (EC 1.11.1.6) and Guaiacol peroxidase (GPX) (EC 1.11.1.7) was determined according to the methods employed by Giannopolitis & Ries (Reference Giannopolitis and Ries1977), Azevedo et al. (Reference Azevedo, Alas, Smith and Lea1998) and Urbanek et al. (Reference Urbanek, Kuzniak-Gebarowska and Herka1991), respectively.
Statistical analysis
The experiment was arranged in a completely randomized design with three replicates. SPSS software was employed for statistical analysis, and graphs were plotted by Excel software. Means were compared using Duncan's multiple range tests at P < 0.05.
Results
In Figure 1, in histogram (a) related to control cells (without colchicine treatment), the peak in channel 160 indicates the haploid cells. In the two other histograms, channel 320 indicates diploid cells and both histograms (histogram (b), treated cells with 0.1% colchicine and histogram (c), 0.5% colchicine) also show that there are still haploid cells, but with increase of colchicine concentration, the percentage of haploid cells is lower.

Fig. 1. Histograms of relative DNA content of D. salina. (A) Histogram of relative fluorescence intensity of nuclei isolated from the control cells, (B) the treated cells with 0.1% colchicine and (C) the treated cells with 0.5% colchicine.
First, it should be mentioned that according to our previous results (unpublished), the cultures treated with 0.1 and 0.5% colchicine contained 58 and 74% polyploid cells, respectively.
The results related to algal growth indicated that the highest fresh and dry weight of D. salina was observed at concentration of 3 M NaCl (Table 1). Algae exposed to 4 M NaCl contained the least amount of fresh and dry weight. In concentrations ranging from 2 to 4 M, it was found that 0.1% colchicine had a positive effect on fresh and dry weight, respectively in the treated algae. Algae exposed to 0.5% colchicine only showed an increase in dry weight in 3 M NaCl.
Table 1. Effect of different concentrations of colchicine on the growth expressed as fresh and dry weight and photosynthetic pigments of D. salina growing in media containing a range of salt concentrations (1 to 4 M NaCl)
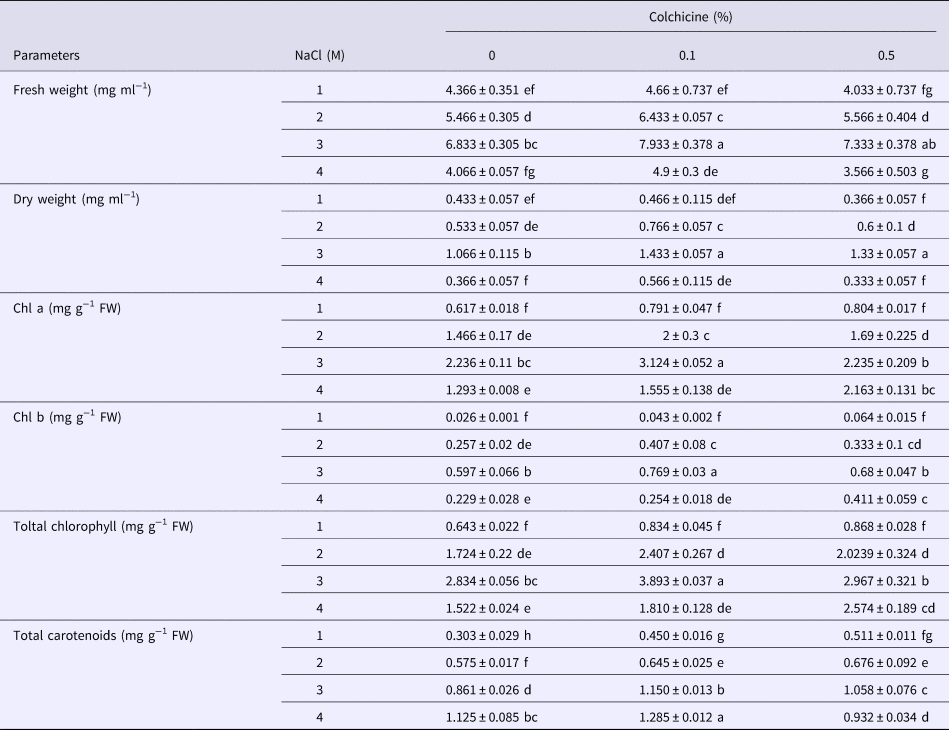
Means of three replications ± SD. Means followed by different letters in a column are significantly different (P < 0.05) according to Duncanʼs test.
Table 1 demonstrates the impact of colchicine and salinity on chlorophyll and carotenoid content in Dunaliella. At higher and lower concentrations of 3 M NaCl, a significant reduction in the amount of chlorophyll was observed. The lowest amount of chlorophyll was observed in 1 M salinity. In this respect, chlorophyll b was more sensitive than chlorophyll a. A concentration of 0.1% colchicine caused an enhancement in chlorophyll a and b content compared with control samples (without colchicine) at 2 and 3 M NaCl, and treatment of 0.5% colchicine had no significant change compared with controls to salinity 3 M, but at 4 M NaCl caused an increase in content of chlorophyll a and b.
Carotenoid content was enhanced with increasing of salinity in culture. The highest cellular carotenoid level was recorded at 4 M NaCl. Algae exposed to 0.1% colchicine showed enhancement of carotenoids accumulation at all salinities (1–4 M) (Table 1). Treatment of 0.5% colchicine increased the carotenoid content in the concentration range of 1–3 M NaCl.
The decrease in carbohydrate content (Figure 2A) was observed under 1 and 4 M salinity. Treatment of 0.1% colchicine positively affected soluble sugar content under 3 and 4 M salinity. In addition, a significant increase in sugar content was noticed in the algae treated with 0.5% colchicine and 3 M NaCl.

Fig. 2. Effect of different salinity concentrations on (A) soluble carbohydrate, (B) glycerol synthesis, (C) total protein and starch content (D) of D. salina. Cultures were grown in medium containing a wide range of salinity (1 to 4.0 M NaCl). The vertical bars represent the standard deviation; different letters above the bars indicate significance level at P < 0.05.
After exposure to different salinity levels, a considerable change in the glycerol concentrations was evident in untreated-colchicine samples (Figure 2B). The maximum production of glycerol value was achieved in 4 M salinity. Induced, polyploidy created by 0.1 and 0.5% colchicine considerably affected increasing of glycerol content at optimum salinity level (3 M NaCl).
Ranges of 1–3 M NaCl stimulated protein accumulation (Figure 2C). However, algae exposed to 4 M NaCl had the least amount of protein. The algae treated by 0.1% colchicine showed an increase in protein content at all salinity levels. The highest increase was observed in the algae treated with 0.1% colchicine and 3 M NaCl. Higher concentration of colchicine caused an increase in protein content under 1 and 4 M salinity.
The amount of starch was higher in the algae grown with 3 and 4 M NaCl (Figure 2D). The algae treated by both concentrations of colchicine had more starch content at all salinity levels in comparison to untreated-colchicine algae. The highest increase was observed at 3 M NaCl with ~1.5-fold of untreated cells at this concentration (Figure 2D).
The present results showed a correlation between increasing of salinity level and that of MDA and other aldehydes content (Figure 3). Algal cultures exposed to 0.1 and 0.5% colchicine had less MDA and other aldehydes content under 2, 3 and 4 M NaCl. In this regard, concentration of 0.1% colchicine was more effective. Furthermore, the results showed that lipid peroxidation was stimulated in the algae treated by 0.5% colchicine under 1 M salinity.

Fig. 3. Changes in the content of (A) MDA and other aldehydes (B) in D. salina in response to different concentrations of colchicine under salinity. Different letters above the vertical bars indicate significance level at P < 0.05.
Salinity significantly affected the activity of ROS scavenging enzymes (Figure 4A–C). Catalase activity in the algae grown under optimum salinity (1, 2 and 4 M) increased in comparison to cultures containing 3 M NaCl (Figure 4A). Induced polyploidy by 0.1 and 0.5% colchicine stimulated catalase activity at cultures containing 3 and 4 M NaCl. Polyploidy had no significant changes at two other salinity concentrations in catalase activity.

Fig. 4. The activities of (A), CAT (Catalase), (B), GPX (Guaiacol peroxidase), (C), SOD (superoxide dismutase) in D. salina in response to different salinities. Letters above vertical bars indicate significance level at P < 0.05.
The results revealed that the activity of peroxidase (PX) increased ~2-fold in the cultures treated by 4 M NaCl in comparison to other salinity treatments (1, 2 and 3 M NaCl) (Figure 4B). Specific peroxidase activity of treated algae by colchicine in salinities 3 and 4 M was lower than that of their controls. Like catalase, there was no significant change in the PX between control and colchicine treatments in the concentrations of 1 and 2 M NaCl.
The specific activity of SOD was almost doubled at 4 M NaCl in D. salina. Samples containing polyploidy cells showed a remarkable increase compared with the control at the same salinity concentration (Figure 4C).
Discussion
Optimal concentration of NaCl for growth of D. salina was 3 M, therefore the maximum fresh and dry weight content was gained at this concentration, but the fresh weight decreased with higher and lower concentrations of salinity. A negative trend in the growth rate of the microalga Chlorella sp. was observed when the NaCl concentration was further increased. This decrement in growth is due to accumulation of reactive oxygen species (Kalita et al., Reference Kalita, Baruah, Goswami, Talukdar and Kalita2011). Cultures with 58% polyploidy level (0.1% colchicine) had more fresh and dry weight at most salinity levels. However, higher percentage of polyploidy (74%) induced by 0.5% colchicine treatment increased dry weight only in the optimum condition (3 M NaCl). This suggests that the presence of an equal percentage from haploid and polyploid cells had an improvement effect on the algae growth. In one experiment on diatoms, a correlation between 4N polyploidy class (with 21% percentage of ploidy) and increased growth rate was shown. Vonshak & Richmond (Reference Vonshak and Richmond1981) provided evidence that increase in the cellular DNA content in blue-green algae occurred in association with an increase in growth rate, indicating an increase in the number of genomes in the cell. D'Amato & Durante (Reference D'Amato and Durante2003) reported that the increased availability of nuclear templates was reflected in the growth of protoplast and its functional activity. He also indicated the correlation between the rate of RNA synthesis and the cell ploidy level. Thus, increased availability of RNA templates allows for higher level of protein translation and ultimately higher production of structural and functional proteins increasing the growth rate. Higher growth rate is desirable for commercialization as it allows more biomass to be produced within a shorter period of time. Bagheri & Mansouri (Reference Bagheri and Mansouri2014) reported that mixoploidy Cannabis sativa induced by colchicine treatment showed better growth compared with tetraploid plants. Despite the importance of polyploidy, there are few studies on induction of polyploidy or its effect on algae, but in plants, it is well known that polyploidy leads to an increase in organ size, which may be caused by changes in activities of cell division and expansion as the result of the duplication of gene loci and increase in nuclear DNA content (Sugiyama, Reference Sugiyama2005). Furthermore, in other studies, plants with higher yield were observed by colchicine treatment compared with untreated plants (Mensah et al., Reference Mensah, Obadoni, Akomeah, Ikhajiagbe and Ajibolu2007). Some varieties of potato responded significantly and positively in plant height, number of leaves and fresh weight of plant after colchicine treatment (Alam et al., Reference Alam, Rezaul Karim, Abdul Aziz, Monzur Hossain, Ahmed and Mandal2011).
The results showed a significant reduction in the amount of chlorophyll at higher and lower concentrations of 3 M NaCl, but in the colchicine treated cultures of D. salina, the amount of chlorophyll a and b significantly increased (especially in 0.1% colchicine). The total chlorophyll content of Sesame indicum L. treated with colchicine was enhanced at higher concentrations. Contrary to these findings, with increasing ploidy level in Juncus effusus, chlorophyll content decreased significantly (Xu et al., Reference Xu, Najeeb, Naeem, Daud, Cao, Gong, Shen and Zhou2010).
There is a direct relationship between carotenoids content and increased levels of salinity. High salinity caused an increment in carotenoids content and inversely low salinity caused a decrease in carotenoids content. The photosynthetic apparatus does not use light energy sufficiently, and the excess energy leads to formation of free radicals rather than active oxygen molecules (singlet oxygen) under high salinity. These radicals are responsible for peroxidation reactions destroying diverse compounds of photosynthesis apparatus. Thus, the algae like Dunaliella and Chlorella accumulate large amounts of pigments to scavenge or omit and reduce the radicals (El-Baz et al., Reference El-Baz, Aboul-Enein, El-Baroty, Youssef and Abd El-Baky2002). Induction of polyploidy in D. salina increased the carotenoids content, especially in 0.1% colchicine in all salinities. Comparative results of chlorophyll a, chlorophyll b, total chlorophyll and carotenoids between tetraploid and diploid plants of cannabis demonstrated no significant changes (Bagheri & Mansouri, Reference Bagheri and Mansouri2014).
The amounts of protein and sugar in Dunaliella increased to 3 M NaCl, but in high salinity, their contents declined. However, Rao et al. (Reference Rao, Dayananda, Sarada, Shamala and Ravishankar2007) reported a rise in carbohydrate content when the algae were cultured at different salinities. However, polyploidy raised protein and sugar content in some salt concentrations compared with their controls. Levine et al. (Reference Levine, Garland, Olive, Amici, Clement, Lenz, Ahn, Shaltiel and Stadtman1990) in accordance with the present result, reported that salt stress inhibited growth and development, decline in photosynthesis, respiration and protein synthesis, and disturbed nucleic acid metabolism. Bagheri & Mansouri (Reference Bagheri and Mansouri2014) reported that tetraploid cannabis had higher values of total proteins compared with control plants (Bagheri & Mansouri, Reference Bagheri and Mansouri2014). In the mentioned study, the highest amount of protein and sugar was found in mixoploid plants. Jaskani et al. (Reference Jaskani, Kwon and Kim2005) reported that the total sugar content was comparable in diploid and tetraploid fruits of watermelon line. Like our results, Grange et al. (Reference Grange, Leskovar, Pike and Cobb2003) observed higher total sugar content in triploid than in diploid fruit of watermelon, but in tetraploid cannabis, a reduction in carbohydrate content was observed due to decrease of CO2 fixation (Bagheri & Mansouri, Reference Bagheri and Mansouri2014). It can be illustrated that in polyploid cells with higher DNA content, protein and sugar biosynthesis pathways were stimulated.
With increasing of salinity, the content of glycerol in this alga rose and reached its maximum in 4 M NaCl. The main role of glycerol in Dunaliella seems to be as an osmotic regulator so that it is a compatible solute at high concentrations of salt, protecting enzyme activity. According to Avron & Ben-Amotz (Reference Avron and Ben-Amotz1992), glycerol is an important compound for osmotic regulation in Dunaliella. Polyploidy increased the amount of glycerol only in the optimum growth condition. These results showed that in spite of an increase in DNA content, the genes related to glycerol biosynthesis at a high salinity level (4 M) were inactive.
In this study, the amount of starch increased at 3 and 4 M NaCl, but polyploidy induced starch accumulation at all concentrations of salinity. For D. parva, with higher concentrations of salt in the medium a higher rate of glycerol synthesis was observed whereas this relation does not exist between starch content and the medium salinity (Gimmler & Molle, Reference Gimmler and Molle1981). In polyploid races of Atriplex confertifolia, content of DNA, enzyme activity, photosynthesis per cell and cell volume increased (Warner & Edwards, Reference Warner and Edwards1989). Higher photosynthetic rate in polyploidy samples can be explained by inducing starch accumulation. The leaves of mixoploid and tetraploid plants of Cannabis sativa L. compared with control plants contained higher amounts of starch (Bagheri & Mansouri, Reference Bagheri and Mansouri2014).
Raja et al. (Reference Raja, Iswarya, Balasubramanyam and Rengasamy2007) mentioned that part of the main physiological responses was caused by stress conditions, including the flux of carbon between starch production in the chloroplast, synthesis of glycerol in the cytoplasm and accumulation of carotenoids. Goyal (Reference Goyal2007) also observed that increased salt stress caused increments in the contribution of products of starch breakdown to glycerol synthesis. However, in the present study, we did not find a special relationship between these metabolites.
Results show that salinity significantly increased MDA and other aldehydes content in controls. However, in treated samples with 0.1% colchicines, the amount of MDA declined considerably. MDA is an important indicator of the damage caused by salt stress (Verslues et al., Reference Verslues, Agarwal, Katiyar-Agarwal, Zhu and Zhu2006). Lower lipid peroxidation in polyploid algae can be attributed to the effect of non-enzymatic antioxidant compounds (such as carotenoids) protecting the photosynthetic apparatus from damage by quenching triplet chlorophyll and singlet oxygen, in addition to the different strategies in the enzymatic system (Young & Frank, Reference Young and Frank1996). Salinity increased O2− and H2O2 content in both diploid (2X) and tetraploid (4X) of Robinia pseudoacacia. However, 2X plants showed higher O2− and H2O2 content compared with 4X plants at the end of 10 days of experiment. Additionally, MDA content increased in 2X and 4X after salt treatment, and 2X showed much higher levels than those of 4X, indicating more damage to membranes in 2X plants (Wang et al., Reference Wang, Wang, Liu and Meng2013).
Gossett et al. (Reference Gossett, Banks, Millhollon and Luceas1996) suggested that protection from oxidative damage was induced under salt stress by more active ascorbate-glutathione cycles and a higher level of antioxidant enzymes such as CAT, SOD and POD. Accordingly, D. salina exposed to the high level of salinity (4 M NaCl) showed a significant increase in SOD, CAT and GPX activity, indicating that these enzymes were utilized for enzymatic scavenging of AOS. Polyploidy had no remarkable impact on increase of antioxidant enzymes activity, except its impact on the SOD activity in the algae treated by 4 M NaCl.
Interestingly, the activities of the antioxidant enzymes (POD and APX) in 4X plants increased under salt stress. Similar to the present results, tetraploid Robinia pseudoacacia showed higher SOD activity than that of diploid plants, indicating that 4X had a more efficient enzymatic antioxidant system against salt stress and dealing with ROS compared with 2X plants (Wang et al., Reference Wang, Wang, Liu and Meng2013).
In conclusion, we investigated the existence of different percentages of polyploidy cells in D. salina cultures and its response to salinity. As expected, all analysed parameters showed variation in each of the three ploid populations. In general, mixoploid cultures showed better characterization than haploid cultures, especially under optimum condition (3 M NaCl). Thus, populations with equal percentages of haploid and polyploid cells (0.1% colchicine treatment) had a higher function. We argue that polyploidy can be used to increase algal productivity.
Acknowledgements
This work was carried out at Shahid Bahonar University of Kerman, Iran. We also thank the Research Support Fund of Shahid Bahonar University.