Introduction
Bytízite is a new Cu-Sb selenide. It occurs in a complex selenide assemblage at the abandoned complex uranium and base-metal ore district of Příbram, Czech Republic, during an extensive research program focused on selenide mineralization of the Bohemian Massif (Sejkora et al., Reference Sejkora, Makovicky, Topa, Putz, Zagler and Plášil2011, Reference Sejkora, Plášil, Litochleb, Škácha and Pavlíček2012, Reference Sejkora, Macek, Škácha, Pauliš, Plášil and Toegel2014, Reference Sejkora, Škácha, Kopecký, Kopecký, Pauliš, Malíková and Velebil2016; Škácha, Reference Škácha2015; Škácha et al., Reference Škácha, Buixaderas, Plášil, Sejkora, Goliáš and Vlček2014, Reference Škácha, Plášil, Sejkora and Goliáš2015, Reference Škácha, Palatinus, Sejkora, Plášil, Macek and Goliáš2016, Reference Škácha, Sejkora and Plášil2017; Sejkora and Škácha, Reference Sejkora and Škácha2015a,Reference Sejkora and Škáchab).
Bytízite is named after its type locality, the Bytíz deposit, near the village of Bytíz. The Bytíz deposit is the most important within the Příbram uranium and base-metal district; it produced more than 52% of uranium from the whole district. The Příbram uranium and base-metal district is the largest vein-type uranium deposit in the Czech Republic (Litochleb et al., Reference Litochleb, Černý, Litochlebová, Sejkora and Šreinová2003).
The new mineral and the name were approved by the Commission on New Minerals, Nomenclature and Classification of the International Mineralogical Association (IMA2016-044). The cotype material (two polished sections) is deposited in the Mineralogical collection of the Department of Mineralogy and Petrology of the National Museum, Prague, Czech Republic (catalogue number P1P 11/2016) and in the mineralogical collection of the Mining Museum Příbram, Příbram, Czech Republic, under the catalogue number 2/2016.
Occurrence
The Příbram ore area (central Bohemia, Czech Republic) is known for the deposits of base-metals as well as for uranium ores. It could be divided into two main ore districts: the base-metal Březové Hory ore district and the complex U and base-metal Příbram district. The latter represents the largest accumulation of vein-type hydrothermal U ores in the Czech Republic and is comparable to world-class deposits of this type. The hydrothermal U mineralization of late Variscan age is related to a 1–2 km wide and almost 25 km long zone formed by a strongly tectonized series of Upper Proterozoic rocks along the contact with granitoids of the Permo-carboniferous Central Bohemian Plutonic Complex. The Příbram uranium and base-metal district can be sub-divided into several ore deposits (also called ore nodes) – among them the most important were Bytíz, Háje and Brod (Ettler et al., Reference Ettler, Sejkora, Drahota, Litochleb, Pauliš, Zeman, Novák and Pašava2010).
In this ore district, there are four main mineralization stages: (1) siderite–sulfides; (2) calcite; (3) calcite–uraninite; and (4) calcite–sulfides. Selenide mineralization occurs in close association with uraninite of the calcite–uraninite mineralization, but selenides are always younger than uraninite. It is uncertain whether the selenides at Příbram formed at the end of the calcite–uraninite stage, or at the beginning of the following calcite–sulfides stage. The age of the uranium mineralization obtained by U–Pb radiometric age determination of two uraninite samples is middle Permian, 275 ± 4 and 278 ± 4 Ma (Anderson, Reference Anderson1987).
The first selenide found there – clausthalite – was mentioned by Růžička (Reference Růžička1986); subsequently, eight selenides were found during a study of the National museum (Prague) specimen by Litochleb et al. (Reference Litochleb, Sejkora and Šrein2004). Since 2005, the first author (PŠ) has found many specimens of a selenide-containing gangue in the dump material of shafts #16, #11A and #9. The collected material contains a very rich selenide assemblage (Škácha, Reference Škácha, Plášil, Sejkora and Goliáš2015; Škácha and Sejkora, Reference Škácha and Sejkora2007, Škácha et al., Reference Škácha, Sejkora, Litochleb and Hofman2009; Reference Škácha, Vlček, Sejkora, Plášil and Goliáš2010, Reference Škácha, Buixaderas, Plášil, Sejkora, Goliáš and Vlček2014, Reference Škácha, Plášil, Sejkora and Goliáš2015, Reference Škácha, Palatinus, Sejkora, Plášil, Macek and Goliáš2016), including a new mineral, here described as bytízite.
One hand-sized sample with bytízite was found in mine dump material of shaft #16 (49°40′33.7″N, 14°03′30.5″E), located in the Háje deposit. Shaft #16 exploited mainly the middle and deeper parts of the vein system of the deposits Háje, Bytíz and Jerusalem (from 500 m down to a depth of 1800 m below the surface). The majority of the selenide-bearing material probably came from the ore deposit Bytíz, which provided 52% of the net U production of the entire district. The Se–U mineralization is of low-temperature hydrothermal origin and is confined to calcite veins with a thickness varying from tens of centimetres to several metres. The main ore is represented by uraninite, while younger pyrobitumen predominates in deeper parts of the deposit.
Macroscopically, bytízite forms a substantial part of up to 1 mm large steel-grey grains in a calcite vein several millimetres thick. This younger carbonate vein cuts perpendicularly through an older calcite vein containing common umangite and uraninite, so umangite is unequivocally older than other selenides in the association. Bytízite forms anhedral grains enclosing other coexisting selenides which involves chaméanite (Cu,Fe)4As(Se,S)4, bukovite Tl2(Cu,Fe)4Se4, příbramite CuSbSe2, eskebornite, crookesite and giraudite (Cu6[Cu4(Fe,Zn)2]As4Se13. Therefore, bytízite appears to be the youngest selenide in the association. Hakite and tetrahedrite also occur, probably as relatively late phases in the association.
Physical and optical properties
Bytízite forms anhedral grains 10–40 µm in diameter (Fig. 1, 2), growing together in aggregates up to 300 µm across. The mineral is steel grey in colour and is opaque in transmitted light; it has a metallic lustre. No cleavage and fracture were observed. The calculated density (Z = 4) for the empirical formula is 6.324 g cm–3. Mohs hardness is assumed at 2–3 by analogy with wittichenite and skinnerite. In reflected light, bytízite is grey with a yellowish hue and weak bireflectance. Pleochroism is weak. Anisotropy under crossed polarisers is strong (Fig. 1), with grey to brownish rotation tints. Internal reflections were not observed. Reflectance percentages for the four Commision on Ore Mineralogy wavelengths (R min and R max) for bytízite from Příbram are: 36.1/40.2 (470 nm), 36.1/39.3 (546 nm), 35.5/38.3 (589 nm) and 34.7/37.0 (650 nm); the full reflectance dataset are given in Table 1 and Fig. 3.
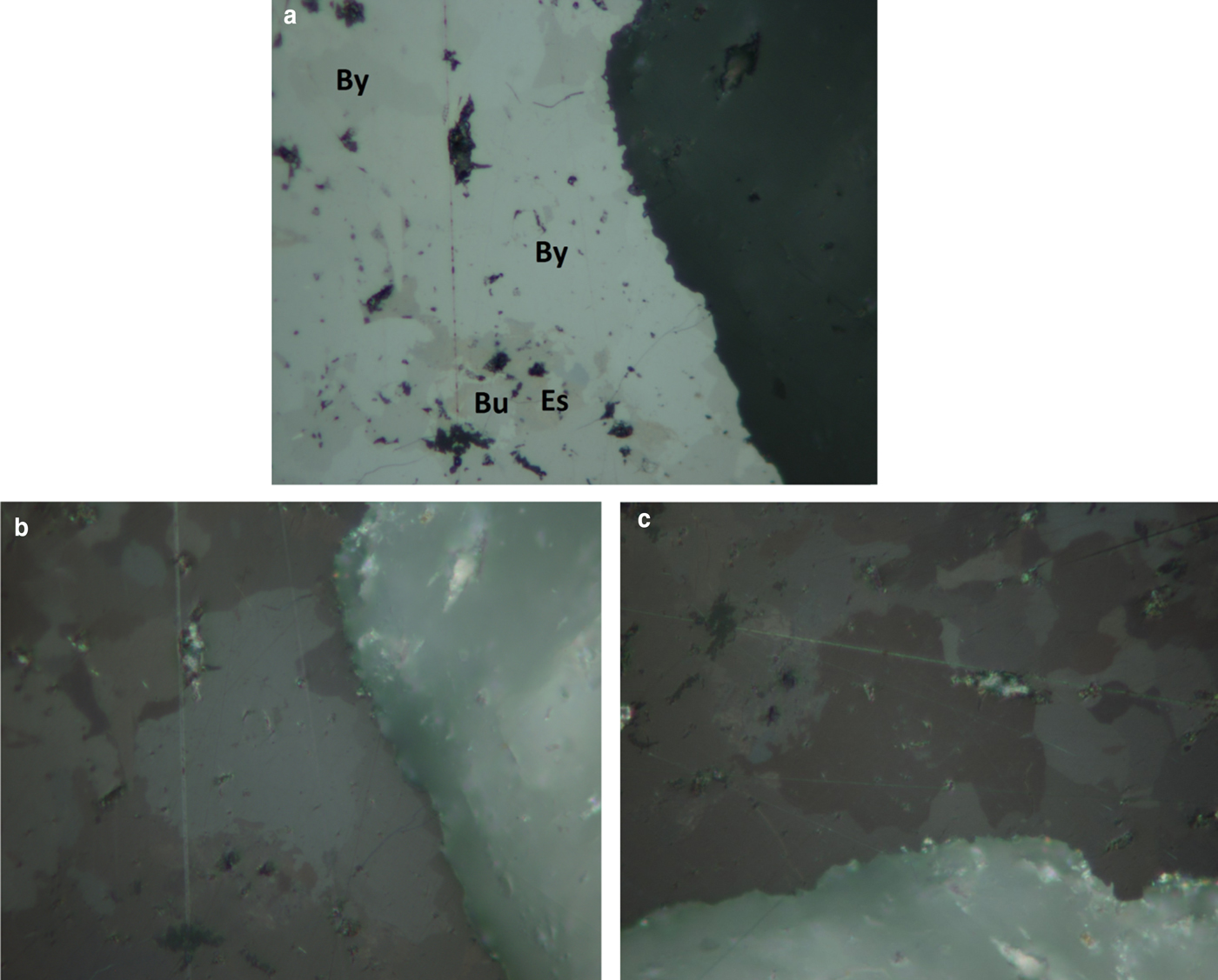
Fig. 1. Reflected light image of an aggregate (Field of view ≈ 100 µm) of bytízite (By), bukovite (Bu) and eskebornite (Es) with one nicol; (b and c) reflected light image, partly crossed polarisers (different extinction); with crossed polars, bytízite is strong anisotropic with grey to brownish rotation tints; photos: P. Škácha and J. Sejkora.
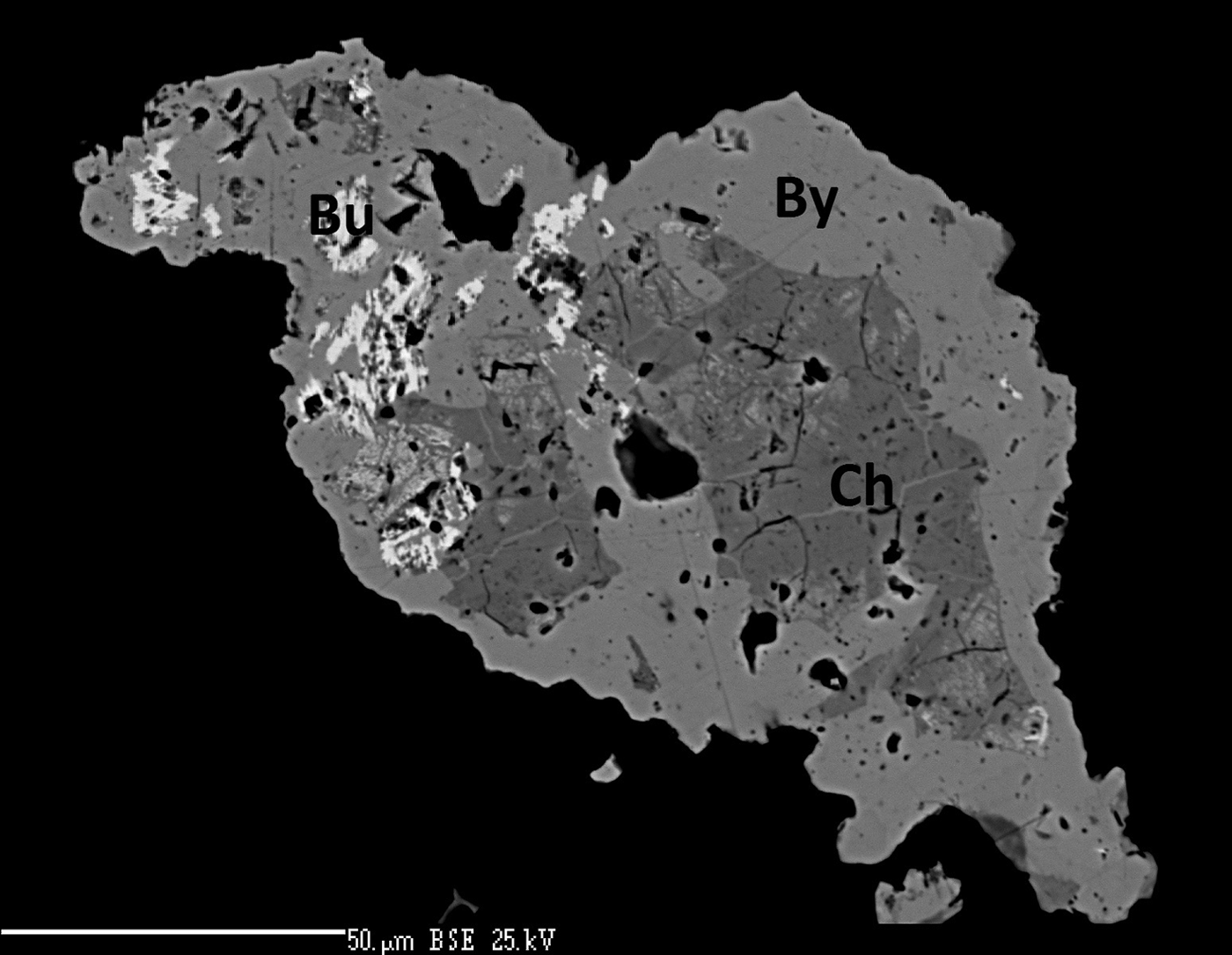
Fig. 2. Back-scatter electron image of a bytízite aggregate (By) enclosed anhedral grains of bukovite (Bu) and chaméanite (Ch). Photo: J. Sejkora and P. Škácha.
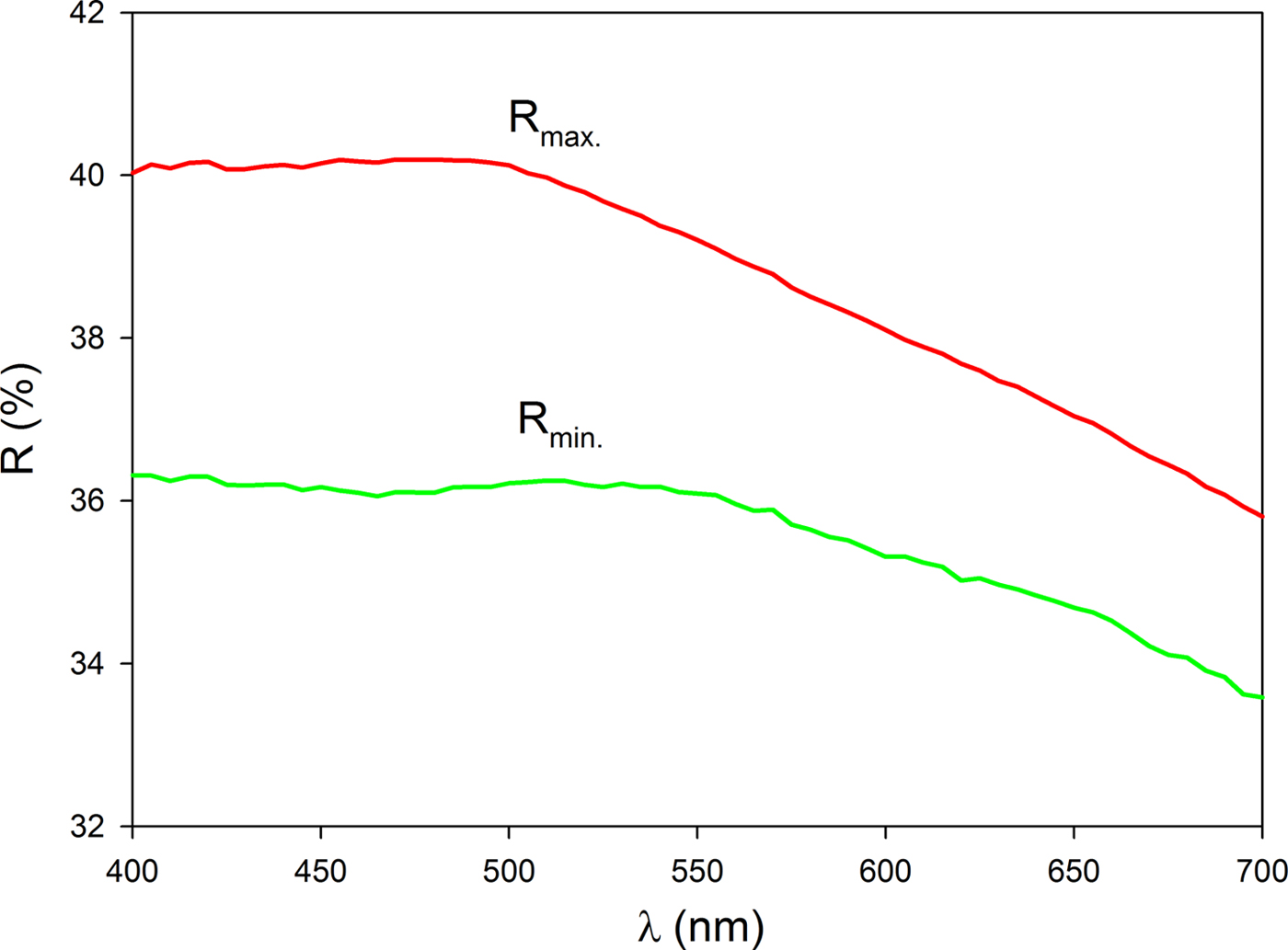
Fig. 3. Reflectivity curves for bytízite.
Table 1. Reflectance values (WTiC standard in air, Zeiss 370; spectrophotometer MSP400 TIDAS with Leica microscope, objective 50x). Data are plotted in Fig. 1.

Note: The values required by the Commission on Ore Mineralogy are given in bold.
Chemical composition
Chemical analyses (n = 68) were performed using a Cameca SX100 electron microprobe (National Museum, Prague) operating in wavelength-dispersive mode (25 kV, 20 nA and 2 µm wide beam). Analytical data are given in Table 2 and representative analyses are given in Table 3. The following standards and X-ray lines were used to minimize line overlaps: Ag (AgLα), Au (AuMα), Bi (BiMβ), CdTe (CdLα), Co (CoKα), chalcopyrite (CuKα), FeS2 (FeKα, SKα), HgTe (HgMα), NiAs (NiKα, AsLα), PbS (PbMα), PbSe (SeLα), PbTe (TeLα), Sb2S3 (SbLα), Tl(BrI) (TlLα) and ZnS (ZnKα). Peak counting times were 20 s for all elements, and one half of the peak time for each background. Other elements, such as Au, Bi, Cd, Co, Ni, Pb, Te, Tl and Zn were found to be below the detection limits (0.02–0.05 wt.%). Raw intensities were converted to the concentrations of elements using the automatic ‘PAP’ (Pouchou and Pichoir, Reference Pouchou, Pichoir and Armstrong1985) matrix-correction software.
Table 2. Compositional data (wt.%) for bytízite (n = 68).

SD – Standard deviation
Table 3. Representative analyses (wt.%) for bytízite*.

*1–15 representative analyses of bytízite from Příbram; coefficients of the empirical formula were calculated on the basis of 7 atoms per formula unit.
The chemical compositions of individual grains of bytízite are very similar, and correspond very well with the formula Cu3SbSe3 derived from the crystal structure study. Only minor Sb–As and S–Se substitutions were observed; a similar trend was found for other Cu-Sb selenides from Příbram, i.e. permingeatite (Škácha et al., Reference Škácha, Buixaderas, Plášil, Sejkora, Goliáš and Vlček2014) and příbramite (Škácha et al., Reference Škácha, Sejkora and Plášil2017). The empirical formula for bytízite, based on electron-microprobe analyses, is (Cu3.00Fe0.01Ag0.01)3.02(Sb0.97As0.06)1.03Se2.94. The ideal formula is Cu3SbSe3, which requires Cu 34.71, Sb 22.16 and Se 43.13, total 100.00 wt.%.
X-ray diffraction data
The powder X-ray study was carried out using a Rigaku (Oxford Diffraction) SuperNova single-crystal diffractometer with Atlas S2 CCD detector utilizing MoKα radiation provided by the micro-focus X-ray tube and monochromatized by primary mirror optics. A Gandolfi-like motion on the ϕ and ω axes was used to randomize the sample and observed d spacings and intensities were derived using CrysAlis software (Rigaku, Reference Rigaku2016). The powder data presented in Table 4 show good agreement with the pattern calculated from the structure determination. Unit-cell parameters refined from the powder data using Celref (Laugier and Bochu, Reference Laugier and Bochu2003) are: a = 7.94(1), b = 10.55(3), c = 6.84(1) Å and V = 573(1) Å3.
Table 4. Powder X-ray diffraction data (d in Å) for bytízite*.

*The calculated diffraction pattern was obtained with the atom coordinates reported in Table 6 (only reflections with I rel. ≥ 4 are listed).
A 0.02 mm × 0.01 mm × 0.01 mm large crystal of bytízite, extracted from the polished section, was examined using a Rigaku (Oxford Diffraction) SuperNova single-crystal diffractometer with Atlas S2 CCD detector utilizing MoKα radiation provided by the micro-focus X-ray tube and monochromatized by primary mirror optics. The ω rotational scans (frame width of 1.0° and counting time of 250 s) were adopted for the acquisition of the three dimensional intensity data. From the total of 8231 reflections, 809 were independent and 590 classified as unique observed with I >3σ(I). Corrections for background, Lorentz effects and polarization were applied during data-reduction in the CrysAlis software, a correction for absorption, using Gaussian integration (μ = 34.44 mm−1) were applied in Jana2006 (Petříček et al., Reference Petříček, Dušek and Palatinus2014) to the data, with R int of 0.117. The structure of bytízite was refined from diffraction data based on the structure model for synthetic orthorhombic Cu3SbSe3 (Pfitzner, Reference Pfitzner1995) by the full-matrix least-squares algorithm of the Jana2006 program. All atoms were refined with anisotropic atomic displacement parameters. Refinement for 37 parameters converged smoothly to the final R = 0.0437, wR = 0.0898 for 530 observed reflections with Goof = 1.30. Details of data collection, crystallographic data and refinement are given in Table 5. Atom coordinates and displacement parameters are listed in Table 6; selected interatomic distances and bond-valence sums are provided in Table 7. The bond-valence parameters were taken from Shields et al. (Reference Shields, Raithby, Allen and Motherwell2000) for Cu1+–Se and from Brown (pers. comm.) for Sb3+–Se.
Table 5. Summary of data collection conditions and refinement parameters for bytízite.

Table 6. Atom positions and displacement parameters (Å2) for bytízite.

Table 7. Interatomic distances (in Å) in the structure of bytízite and bond-valence sums (in valence units).

Symmetry codes: (i) –x + 3/2, –y + 1, z–1/2; (ii) –x + 3/2, y–1/2, z–1/2; (iii) x, –y + 1/2, z; (iv) x–1/2, –y + 1/2, –z + 3/2; (v) x + 1/2, y, –z + 3/2.
On the basis of the current X-ray study we conclude bytízite is orthorhombic, which is in line with the studies on synthetic Cu3SbSe3 prepared by Pfitzner (Reference Pfitzner1995). The structure of bytízite (Fig. 4) contains two Cu sites, one Sb site and two Se sites, all sites were assumed to be fully occupied. The Cu1 site is 3 + 1 coordinated by three Se atoms at a distance of ~2.4 Å and one longer Cu–Se bond, ~2.88 Å (Table 7; Fig. 5). The Cu2 site is regularly coordinated by four Se atoms to form a tetrahedron. The Sb site is coordinated by three Se atoms as a regular trigonal pyramid (Fig. 5). There is no departure from the structure of synthetic orthorhombic Cu3SbSe3 (Pfitzner, Reference Pfitzner1995). In the structure of both synthetic Cu3SbSe3 and bytízite there are groups of three cis-edge-sharing tetrahedra [Cu3Se8], which are interlinked to a 3D framework by SbSe3 groups (Fig. 4). Wittichenite, Cu3BiS3 (Kocman and Nuffield, Reference Kocman and Nuffield1973) possesses a similar structure to bytízite, synthetic Cu3SbSe3 phases (Pfitzner, Reference Pfitzner1995) and skinnerite (Karup-Møller and Makovicky, Reference Karup-Møller and Makovicky1974; Makovicky and Balič-Žunič, Reference Makovicky and Balič-Žunič1995); however, there are substantial differences among these structures. While in the case of bytízite, synthetic Cu3SbSe3 and skinnerite, one of the Cu sites is tetrahedrally coordinated, in wittichenite all Cu atoms are in a three-fold planar coordination. As reported by Makovicky and Balič-Žunič (Reference Makovicky and Balič-Žunič1995), in wittichenite, the Cu atoms are cis-oriented around octahedral voids, while in the rest of the above mentioned phases, two distinct types of configuration occur: cis- and trans- patterns alternate in layers, as a consequence of the smaller size of SbS3 (as well as SbSe3) compared to BiS3 pyramids.
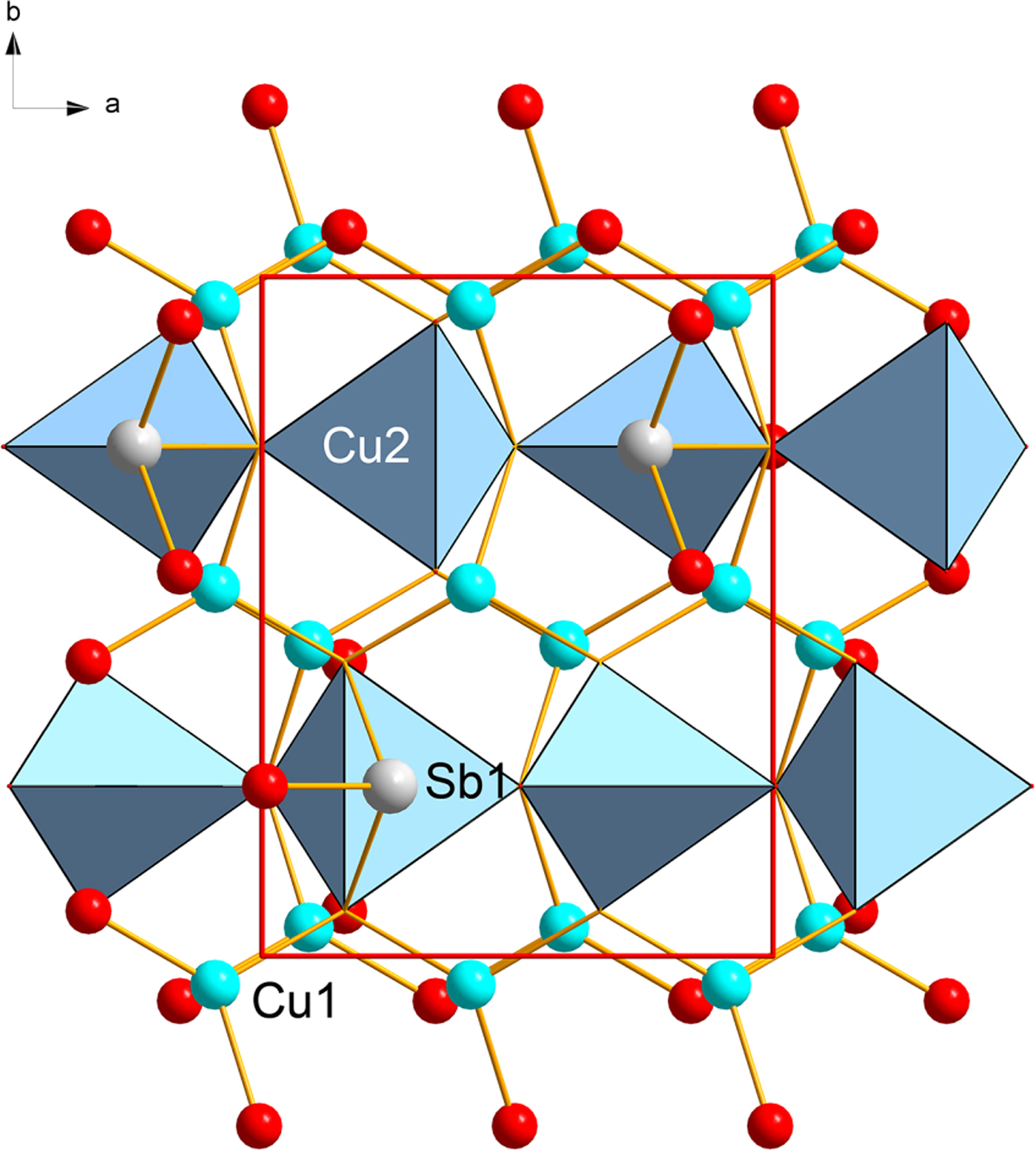
Fig. 4. Crystal structure of bytízite: Cu1 atoms (blue trigonal base) occur in 3 + 1 coordination, the Cu2 atom is coordinated as regular tetrahedron and the Sb1 atom (grey) is in trigonal pyramidal coordination. Unit-cell edges are outlined in red solid lines, the viewing direction is along [001].

Fig. 5. Coordinations of cations found in the bytízite crystal structure. The symbols of symmetry operations are related to those in Table 7.
Relationship to the known species
As mentioned previously, bytízite (Strunz class 2/E.03-40, Dana class 3.4.8.4) is related structurally to the high temperature (>121°C) orthorhombic modification of natural monoclinic skinnerite Cu3SbS3 (Karup-Møller and Makovicky, Reference Karup-Møller and Makovicky1974; Pfitzner, Reference Pfitzner1994; Makovicky and Balič-Žunič, Reference Makovicky and Balič-Žunič1995). The synthetic analogue of bytízite has been prepared by Pfitzner (Reference Pfitzner1995) in a sealed tube containing Cu, Sb and Se at 350°C for 3 weeks. Comparative data for bytízite and relevant natural and synthetic phases are given in Table 8.
Table 8. Comparative data for the relevant minerals.

* Calculated from the structure data.
Origin of the selenium mineralization in Příbram
Several selenide assemblages have been found in Příbram, where 24 selenides have been identified so far (Škácha et al., Reference Škácha, Sejkora and Plášil2017). One of the main reasons for the species diversity of the studied Se mineralization is the crystallization of a part of selenides from late hydrothermal solutions containing remobilized Sb, As, Cu, Hg, Ag and Fe.
According to observation of >120 polished sections, crystallization of first selenides started after formation of colloform uraninite (pitchblende), with Pb and Hg selenides (clausthalite, tiemannite) and eucairite, followed by a Cu–Se mineralization represented by berzelianite, umangite, klockmannite, athabascaite and bellidoite. Minerals of the tetrahedrite group (hakite and giraudite) are younger than Cu selenides in most cases. Bytízite is the result of the youngest mobilization processes together with antimonselite, hakite, příbramite, chaméanite and other selenides. The temperature of this hydrothermal solution was ~100°C, according to the presence of umangite in the association (Simon and Essene, Reference Simon and Essene1996). It is in accordance with the homogenization temperatures of the fluid inclusions determined in the Příbram uranium district (Žák and Dobeš, Reference Žák and Dobeš1991).
The abundance of calcite in gangue indicates a neutral to weakly alkaline environment (Dymkov, Reference Dymkov1985; Kvaček, Reference Kvaček1987). Selenides are formed from hydrothermal fluids at conditions of ${f}\!_{O_2} $ above the hematite-magnetite buffer, probably in the range of 5.8 log units (hematite-ferroselite univariant reaction) to 7 log units (anglesite-galena buffer), above the hematite-magnetite buffer (Simon et al., Reference Simon, Kesler and Essene1997). Such high oxygen fugacity values result in geochemical separation of selenium from sulfur in the hydrothermal fluids, a high Se2/S2 fugacity ratio, and in the deposition of various selenide minerals. The quantity and variability of selenide association, which formed under oxidizing conditions, is a function of Se2 fugacity and reflects the concentration of available Se in solutions (Simon et al., Reference Simon, Kesler and Essene1997). The selenide mineralization in the Příbram uranium district shows variations in Se2 and S2 fugacities (–12 to –18 in the case of Se and –17 to –24 of S, respectively), which are similar to those published in detail (Simon et al., Reference Simon, Kesler and Essene1997) for “selenide-bearing unconformity-related uranium deposits”. Considering the absence of kruťaite from the association despite its stability at 100°C and sufficiently high
${f}_{{\rm Se}_2} $ (Simon et al., Reference Simon, Kesler and Essene1997), we believe the Se mineralization to have occurred at Se fugacities too low for kruťaite stability, log
${f}_{{\rm Se}_2} $ < –12 (Škácha et al., Reference Škácha, Sejkora and Plášil2017).
Acknowledgements
The authors appreciate the comments of two anonymous reviewers, Pete Leverett and the Principal Editor Peter Williams. The research was financially supported by the project GA14-27006S of the Czech Science Foundation for PŠ a JS. Single-crystal X-ray diffraction experiments were done using instruments of the ASTRA lab established within the Operation program Prague Competitiveness – project CZ.2.16/3.1.00/24510.