Introduction
Bronchopulmonary verminosis in wild ruminants is caused by nematodes that inhabit bronchi, bronchioles and pulmonary alveoli. After ingestion, the third-stage larvae migrate from the digestive tract to the lungs, where they moult to the fourth stage in the bronchi and bronchioles and develop into adults (Panuska, Reference Panuska2006). Embryonated eggs are coughed up, swallowed and first-stage larvae (L1) develop in the small intestine and are released to the environment via faeces (Levine, Reference Levine1985). It is well known that bronchopulmonary nematodes in wild, farmed and domestic ruminants are causal agents of respiratory disease with potentially serious consequences for the health of the host (Carreno & Hoberg, Reference Carreno and Hoberg1999).
Lungworms are common parasites in wild ruminants (Panadero et al., Reference Panadero, Carrillo, López, Dı́ez-Baños, Dı́ez-Baños and Morrondo2001; Marreros et al., Reference Marreros, Frey, Willisch, Signer and Ryser-Degiorgis2012). Some Protostrongylus and Elaphostrongylus spp. have been mentioned among the most important causes of disease in wild ruminants (Vicente et al., Reference Vicente, Fierro and Gortázar2005; Alasaad et al., Reference Alasaad, Morrondo, Dacal-Rivas, Soriguer, Granados, Serrano, Zhu, Rossi and Pérez2009). Although most infections are subclinical, the co-infection with other microparasites or stress conditions can lead to the development of respiratory disorders such as pneumonia (Jenkins, Reference Jenkins2001) and other diseases (Mansfield et al., Reference Mansfield, Gamble, Baker and Lichtenfels1993). Lungworms can thus exert a negative impact on the individual health and population fitness of wild ruminants, inducing loss of weight, diminished offspring, abortions, neonatal deaths and increased mortality (Berrag & Urquhart, Reference Berrag and Urquhart1996; Lavín et al., Reference Lavín, Marco, Rossi, Meneguz and Viñas1997; Gunn & Irvine, Reference Gunn and Irvine2003).
The Baermann–Wetzel technique, based on the migration and movement of L1, is the most widely used diagnostic tool for bronchopulmonary nematodes (Rode & Jørgensen, Reference Rode and Jørgensen1989; Eysker, Reference Eysker1997). There are discrepancies in the diagnostic performance of the Baermann–Wetzel technique using different sample types (lung parenchyma vs. faecal), with a higher sensitivity for the pulmonary ones (Díez-Baños & Hidalgo-Argüello, Reference Díez-Baños and Hidalgo-Argüello2006). However, faecal samples offer several advantages with respect to the pulmonary tissue: (1) they are easily obtainable in a non-invasive and cost-effective manner; (2) no animals are removed, so their obtention does not impact small or otherwise vulnerable populations; and (3) they enable repeated sampling and monitoring of populations with marked or confined individuals in fenced areas, which makes it possible to follow the evolution of the infection or to study the phenology of these parasites, improving the knowledge of the temporal and spatial dynamics of the lungworm at population level (Cassini et al., Reference Cassini, Párraga, Signorini, Frangipane di Regalbono, Sturaro, Rossi and Ramanzin2015; Kafle et al., Reference Kafle, Lejeune, Verocai, Hoberg and Kutz2015). While faecal samples are routinely used for diagnosing livestock, the diagnosis of wild ruminants relies on the lung parenchyma of hunted or already dead individuals (Panadero et al., Reference Panadero, Carrillo, López, Dı́ez-Baños, Dı́ez-Baños and Morrondo2001; Viña et al., Reference Viña, Panadero, Díaz, Fernández, Pérez, Díez-Baños, Morrondo and López2013).
Proper sampling and determination of wildlife helminths can be an outright challenge, although in the case of bronchopulmonary nematodes it is feasible through the use of faecal samples (Kafle et al., Reference Kafle, Lejeune, Verocai, Hoberg and Kutz2015). Faecal samples collected in the field have the potential to answer many ecological questions that may otherwise remain out of reach (Putman, Reference Putman1984). Lungworms also present the advantage of being thermostable for cryopreservation as well as drought-tolerant in faeces (Rose, Reference Rose1957; Andermatt-Mettler et al., Reference Andermatt-Mettler, Eckert, Ramp and Gottstein1987), easing fieldwork and allowing for long-term sample conservation, which can be key to carrying out comparative or retrospective studies. The evaluation of samples that bring together the greatest possible number of advantages to detect and identify bronchopulmonary nematodes at population and individual levels in wild ruminants is key to correct management, especially for endangered host species and in wild–domestic interface areas, where there is a potential for parasite spillover (Bienioschek et al., Reference Bienioschek, Rehbein and Ribbeck1996; Panuska, Reference Panuska2006; Kelly et al., Reference Kelly, Paterson, Townsend, Poulin and Tompkins2009; Sleeman et al., Reference Sleeman, Richgels, White and Stephen2019). For this reason, this study aimed to evaluate the agreement between the results obtained from lungworm monitoring in faeces and lung tissue from wild ruminants using the Baermann–Wetzel technique by comparing (1) the total number of larvae recovered from each type of sample; (2) the sensitivity of the technique according to the type of sample used; and (3) the agreement between both sample sources to address a possible interchangeability in their use.
Material and methods
Type of samples, collection and storage
A total of 500 samples (n = 250 pulmonary samples; n = 250 faecal samples) was obtained from four different host species of free-ranging wild ruminants hunted at the Sierras de Cazorla, Segura y Las Villas Natural Park, Jaen, Andalusia (south-east Spain): mouflon (Ovis aries musimon), red deer (Cervus elaphus), fallow deer (Dama dama) and Iberian ibex (Capra pyrenaica). The respiratory tract, including lungs and trachea, and rectal faecal samples were recovered from each animal during the field necropsy. Faecal and pulmonary samples were kept in pairs but in separate storage bags for one-to-one comparison and stored at −20°C until diagnostic analyses were performed.
Identification of parasites
The Baermann–Wetzel method was used to extract bronchopulmonary L1 as described by Forrester & Lankester (Reference Forrester and Lankester1997). To process the respiratory system of each animal, after opening the tracheobronchial tree, 25 g of trachea, bronchi and bronchioles were cut into pieces of 0.5 cm; subsequently, 0.5-cm-diameter pieces were taken from different parts of the parenchyma of both lungs, evenly distributed, until a total of 25 g was collected. Each of these pooled samples was immersed into a separate beaker filled with tap water. Likewise, 25 g of faeces was processed as mentioned above. The samples were kept for 12 h in the Baermann apparatus, after which 10 ml of the fluid from the bottom of the migration system were collected, centrifuged for 5 min (800 g) and the sediment was saved. Larvae were counted under the microscope with a Favatti chamber and then deposited on slides with the addition of a drop of Lugol solution to fix the L1; this semi-permanent preparation allowed the morphological identification of the larvae to genus according to Pyziel (Reference Pyziel2014) and van Wyk et al. (Reference van Wyk, Cabaret and Michael2004). Up to 100 individual larvae per sample were determined. In the case of samples with a lower number of lungworms, all specimens were identified. Results were expressed as the number of L1 per gram (LPG) of examined sample.
Statistical analysis
The relative performance of the Baermann–Wetzel technique applied to two different sample categories was assessed with several statistical indicators.
First, the sensitivity of the two approaches was calculated as the true positive rate of positive samples (capacity of the test to correctly classify animals with lungworm infection). Specificity was assumed to be 100% for both diagnostic approaches, as the method allows a perfect identification of the parasites. In other words, the percentage of false-positive results is equal to zero.
Afterwards, to determine the agreement between LPG results obtained from lung tissues and faeces, a comparison of the average differences of LPG was performed using the Bland–Altman test (Bland & Altman, Reference Bland and Altman1986). The method considers the two sample types to be in agreement if their results fall within the so-called Limit of Agreement (LoA) interval. This interval was calculated using the mean difference ± 1.96 standard deviation (SD) of the LPG obtained using both samples. LoA was calculated for all the larvae together and then split by parasite genus.
Finally, correlation analysis using the Person correlation test and a correlation chart was implemented as a third indicator of agreement between samples. High correlation values between the results obtained on pulmonary tissue and faecal samples were considered an indicator of a good match between the two sampling approaches.
All analyses were carried out using RStudio software version 1.2.5033 (RStudio Team, 2015).
Results
Overall bronchopulmonary larval frequency and intensity
From the overall analysed samples, 39.3% (n = 98) were positive when using pulmonary tissue and 38.0% (n = 95) by faeces. Nonetheless, 45.2% (n = 113) of the analysed animals turned out positive with at least one method. These numbers suggest a similar sensitivity for both methods (86.7% for pulmonary tissue and 84.1% for faeces). On average, the parasite load (parasite intensity) was 61.4 ± 306.1 LPG, with different values according to the sample used: 47.3 ± 256.8 LPG on pulmonary tissue, and 14.3 ± 66.2 on faeces. The LPG values obtained on the two types of sample are represented in fig. 1a.

Fig. 1. (A) Graphical representation of overall bronchopulmonary larval frequency; (B) Bland–Altman results for the evaluation of agreement between pulmonary and faecal samples using the Baermann–Wetzel technique; (C) correlation between the values of both sample types was calculated and showed significantly high results (R = 0.54; P < 0.001). P values lower than 0.05 were considered statistically significant: *P < 0.05, **P < 0.01, ***P < 0.0001. LPG, larvae per gram.
Evaluation of the agreement for total LPG
The Bland–Altman test was used to evaluate the agreement between both samples using the Baermann–Wetzel technique. As represented in fig. 1b, samples ranging from zero to 500 mean ± 1.96 SD LPG do not exceed the LoA, showing that the two samples are in agreement and may be used interchangeably. Nonetheless, for samples exceeding 500 LPG (n = 3), the two methods were not in agreement, and the real LPG value was under or overestimated, depending on the sample. Interestingly, samples for which there is a lack of agreement were those with very high parasite intensity (above 500 LPG). Finally, the overall correlation between the values obtained using both sample types was quite high and significant (R = 0.54; P < 0.001; fig. 1c).
Evaluation of the agreement for each genera LPG
From the positive samples (n = 113), seven different bronchopulmonary nematode genera were identified: Muellerius spp. (61/113; 53.9%), Cystocaulus spp. (54/113; 46.9%), Neostrongylus spp. (39/113; 34.5%), Elaphostrongylus cervi (34/113; 30.0%), Protostrongylus spp. (22/113; 19.4%), Varestrongylus spp. (14/113; 12.3%) and Dyctiocaulus spp. (11/113; 9.7%). As presented in fig. 2, we performed the Bland–Altman agreement test for the one-to-one comparison of each parasite genus following the same principle described above.
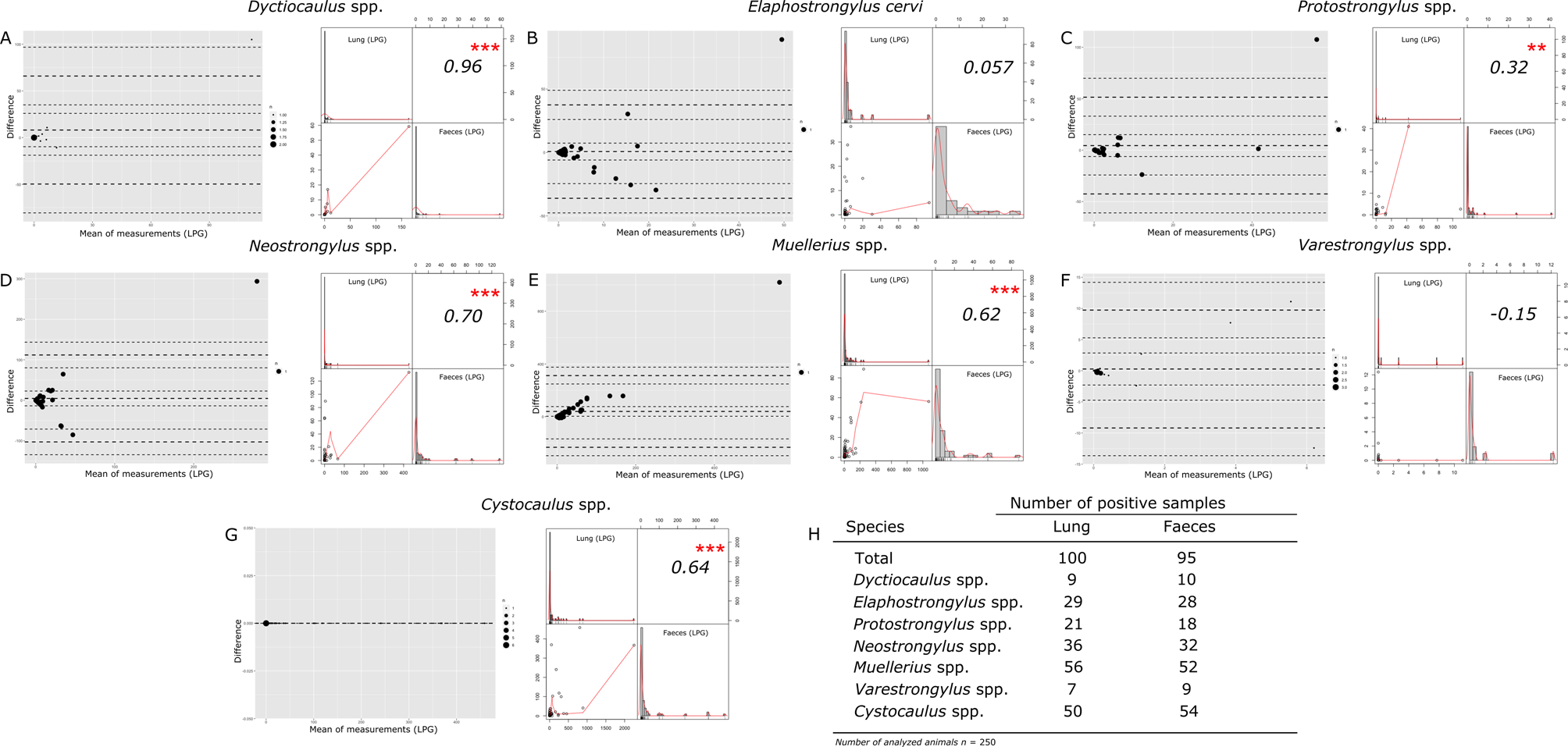
Fig. 2. (A–G) Bland–Altman representation of the agreement test and graphical correlations of all identified genera for one-to-one comparison of each parasite genus between pulmonary and faecal samples using the Baermann–Wetzel technique; (H) positive samples for each nematode genus. P values lower than 0.05 were considered statistically significant: *P < 0.05, **P < 0.01, ***P < 0.0001. LPG, larvae per gram.
Considering the LPG values obtained in each of the four nematode genera globally (Dyctiocaulus spp., Protostrongylus spp., Neostrongylus spp. and Muellerius spp.) and in E. cervi, only one sample exceeded the LoA LPG. This could be considered as an outlier present in this study (fig. 2a–e). The Bland–Altman test showed a perfect agreement for Dyctiocaulus spp., E. cervi and Protostrongylus spp. in the range of 0–40 LPG (LoA not exceeded) (fig. 2a–c). Neostrongylus spp. and Muellerius spp. showed agreement up to 200 LPG (fig. 2d, e). Finally, all LPG values from both sample types positive to Varestrongylus spp. and Cystocaulus spp. did not exceed the LoA (fig. 2f, g). In general, for most of the genera there was a significant correlation between the LPG values detected with the two types of sample, with an R-value ranging between 0.32 (Protostrongylus spp.) and 0.96 (Dyctiocaulus spp.) (fig. 2a, c–g). No significant correlation was detected for E. cervi and Varestrongylus spp. (fig. 2b, f). The number of positive samples is summarized in fig. 2h.
Discussion
This study presents the evaluation and performance comparison of the Baermann–Wetzel technique applied to two types of sample (pulmonary tissue and faeces). Bronchopulmonary nematodes have been widely reported in wild ruminants (Meana et al., Reference Meana, Luzón-Peña, Santiago-Moreno, De Bulnes and Gómez-Bautista1996; Panadero et al., Reference Panadero, Carrillo, López, Dı́ez-Baños, Dı́ez-Baños and Morrondo2001; Alasaad et al., Reference Alasaad, Morrondo, Dacal-Rivas, Soriguer, Granados, Serrano, Zhu, Rossi and Pérez2009), and many studies are based on results obtained using the Baermann–Wetzel technique on lung tissue samples (Panadero et al., Reference Panadero, Carrillo, López, Dı́ez-Baños, Dı́ez-Baños and Morrondo2001; Pyziel, Reference Pyziel2014). In contrast, the use of faecal samples for the diagnosis of lungworm infection in livestock is usually carried out using faecal samples (Viña et al., Reference Viña, Panadero, Díaz, Fernández, Pérez, Díez-Baños, Morrondo and López2013). Our results allow us to evaluate the accuracy and agreement of the results obtained with the two types of sample. Since both kinds of sample come from the same animal, we can confidently apply our results as correction factors to the estimation of prevalence of lungworm and LPG values obtained from different biological samples, which will undoubtedly serve as a reference for future studies to be conducted only with the analysis of wild ruminant faeces. Our results are helpful in understanding when and under which circumstances the Baermann–Wetzel technique can be applied in non-invasive sampling schemes to evaluate the status and dynamics of lungworms in free-roaming ruminant populations.
The Baermann–Wetzel method is considered the gold standard for the detection of bronchopulmonary infections (MAFF, 1986), as described by different authors who have demonstrated its high sensitivity in detecting L1 (Eysker, Reference Eysker1997; Viña et al., Reference Viña, Panadero, Díaz, Fernández, Pérez, Díez-Baños, Morrondo and López2013). Sensitivity of the diagnostic test seems to reach up to 90% (Willard et al., Reference Willard, Roberts, Allison, Grieve and Escher1988; Traversa et al., Reference Traversa, Iorio and Otranto2008) and this finding has been confirmed by our results. In fact, using both kinds of sample, the sensitivity is close to 90%. However, in terms of LPG evaluation, the Baermann–Wetzel technique applied to faecal samples always detected a lower number of L1 in comparison with lung tissue samples. The variability between faeces and bronchopulmonary tissue might be due to extrinsic factors (i.e. period of sampling) as described for the chamois (Rupicapra rupicapra) by Diez-Baños et al. (Reference Diez-Baños, Diez-Baños, Morrondo-Pelayo and Campillo1990), or simply because of the lower concentration of L1 in the faeces than in the pulmonary system due to the location of the fertile adult nematodes.
Despite the different LPG detected, our results show a significant agreement between faecal and pulmonary sample LPG values, as highlighted by the Bland–Altmann test (Bland & Altman, Reference Bland and Altman1986). This tool is considered the most appropriate approach to evaluate the agreement between diagnostic techniques, as opposed to the more widely used correlation analysis, which can be misleading and has been used only as an additional agreement indicator in our work. Many published methodological studies about diagnostic techniques for bronchopulmonary nematodes support their conclusions only on the lack of correlation between L1 found in faeces and pulmonary tissue as a way of comparison (Díez-Baños & Hidalgo-Argüello, Reference Díez-Baños and Hidalgo-Argüello2006), underestimating the importance of faecal material as a sensitive and non-invasive monitoring approach. Thus, we aimed, for the first time, to measure the agreement using a different and more accurate approach, to better investigate and evaluate the importance and potential use of faecal samples in the study of lungworm dynamics in wild ruminant populations, endangered host species and zoo animals (Nocture et al., Reference Nocture, Cabaret and Hugonnet-Chapelle1998; Cassini et al., Reference Cassini, Párraga, Signorini, Frangipane di Regalbono, Sturaro, Rossi and Ramanzin2015).
In general, our study highlighted a consistent agreement in the diagnostic results obtained with the two kinds of samples. In particular, the consistent agreement highlighted for two lungworm genera – Neostrongylus spp. and Muellerius spp. – is noteworthy, suggesting that for these two genera in particular it might be possible to use both pulmonary and faecal samples for proper sanitary surveillance. These two nematode genera had the highest LPG values compared to the other genera of lungworm isolated. Similar results have already been described for the chamois by Diez-Baños et al. (Reference Diez-Baños, Diez-Baños, Morrondo-Pelayo and Campillo1990), who also compared pulmonary versus faecal samples and described an association of the aforementioned nematode genera, thus indicating a possible relation between animals presenting co-infection and high LPG. Hence, parasitic infection with high prevalence and LPG values shows an important agreement between LPG values from both kind of samples.
Our results show that the sensitivity of the Baermann–Wetzel technique using faeces is comparable to that obtained when using pulmonary tissues – that is, our study confirms that epidemiological or diagnostic studies of wild ruminant lungworms can be perfectly performed with faeces, maintaining the same level of accuracy as obtained using lung tissue, but with the advantage that faeces are a non-invasive and easy to obtain sample. This should be taken into account by field researchers in understanding the value of faecal collection for the coprological measurement of L1 when necropsies are not possible or inconvenient (Marreros et al., Reference Marreros, Frey, Willisch, Signer and Ryser-Degiorgis2012). Our findings also revealed that for highly infected animals the agreement between the two sampling approach is progressively lost. However, considering that in our study a lack of agreement has been observed in less than 1% of the examined animals, the problem can be considered negligible and would not influence the results of large-scale monitoring.
A correct and precise diagnosis of lungworm genera is key for the accurate sanitary surveillance, long-term monitoring and treatment of affected animals, and as a basis for population management. Our work highlights the agreement of pulmonary versus faecal samples for one of the most commonly used diagnostic methods for lungworm infection, indicating that both types of samples can be used without losing sensitivity and accuracy. These results support the use and value of faeces as a non-invasive and cost-effective sampling technique for long-term studies, as well as for the preservation and conservation of threatened wild ruminant populations.
Acknowledgements
The authors thank the staff and guards of Sierras de Cazorla, Segura y Las Villas Natural Park, Spain, for their help in carrying out this study.
Financial support
This study was funded by the Spanish Ministry of Science and Technology (project number AGL2002–02916).
Conflicts of interest
None.
Ethical approval
The Ethical Committee for Animal Experimentation of the University of Murcia reports that, following the basic rules applicable for the protection of animals used in experimentation and other scientific purposes (described in RD 53/2013), procedures in this study are considered to be out of the scope of application of said RD since we do not use live animals, but carcasses donated from authorized hunts in the study area.
Author contributions
T.C. and C.M.-C. contributed equally to this study.