1. Introduction
The Hettangian was a crucial period in the evolution of Dinosauria, corresponding to the end of the Late Triassic – Early Jurassic evolutionary radiation of the clade (Brusatte et al. Reference Brusatte, Benton, Ruta and Lloyd2008). However, Hettangian-aged geological units showing a co-occurrence of dinosaur remains (e.g. Larsonneur & Lapparent, Reference Larsonneur and Lapparent1966; Delsate & Ezcurra, Reference Delsate and Ezcurra2014) and associated faunas or floras are rare in Europe. In this context, deposits containing dinosaur tracks and associated fossils constitute an excellent opportunity to reconstruct lowermost Jurassic ecosystems hosting dinosaurs and which are still poorly documented.
The Causses Basin in southern France is known for its abundant Jurassic archosaur footprints (Demathieu et al. Reference Demathieu, Gand, Sciau and Freytet2002; Gand et al. Reference Gand, Demathieu and Montenat2007). The Hettangian Dolomitic Formation from this area yielded abundant vertebrate tracks mainly ascribed to theropod dinosaurs and more rarely to crocodylomorphs (Ellenberger, Reference Ellenberger1988; Demathieu, Reference Demathieu1990; Demathieu & Sciau, Reference Demathieu and Sciau1992, Reference Demathieu and Sciau1999; Demathieu et al. Reference Demathieu, Gand, Sciau and Freytet2002; Sciau, Reference Sciau2003; Moreau et al. Reference Moreau, Baret, Trincal and André2012 a, Reference Moreau, Trincal, Gand, Néraudeau, Bessière and Bourel2014, Reference Moreau, Fara, Néraudeau and Gand2019 a). Dozens of tracksites were reported from the French departments of Aveyron, Gard and Lozère. However, because fossils are extremely rare in the Dolomitic Formation, our knowledge of Early Jurassic ecosystems inhabited by dinosaur communities is limited in this area.
A new palaeontological site showing the co-occurrence of rich plant-bearing beds and dinosaur tracks was discovered from the Dolomitic Formation of the Bleymard Strait (Lozère). Although palaeofloras were previously reported from Hettangian–Sinemurian deposits of several localities in the Causses Basin (e.g. Saporta, Reference Saporta1873, Reference Saporta1884; Ressouche, Reference Ressouche1910; Roquefort, Reference Roquefort1934; Barale et al. Reference Barale, Philippe and Thévenard1991; Thévenard, Reference Thévenard1992, Reference Thévenard1993; Moreau & Thévenard, Reference Moreau and Thévenard2018; Moreau et al. Reference Moreau, Philippe and Thévenard2019 b), they were rarely reported and undescribed associated with dinosaur tracksites (Sciau, Reference Sciau1992). The discovery presented here consists of the first Hettangian dinosaur tracksite yielding plant-rich beds in the Bleymard Strait.
The main objective of this research was to reconstruct Hettangian dinosaur ecosystems from Bleymard using a multiproxy approach. Sedimentology, petrology and mineralogy analyses are combined to characterize the lithofacies and to understand the evolution of the depositional environments. Palaeoichnological analyses based on vertebrate tracks and palaeobotanical analyses based on cuticles, wood and pollen are used to reconstruct the ichnofaunas and palaeofloras.
2. Geographical and geological setting
The fossil plants and dinosaur tracks described here have been discovered in a small quarry in the Bleymard Strait area, in the northeastern extremity of the Causses Basin (Occitanie, southern France). The quarry is located in the Lozère department, 25 km to the east of Mende (Fig. 1). In the Causses Basin, Hettangian deposits are divided into two formations: the Detrital Sandstones – Variegated Mudstones Formation and the Dolomitic Formation (Brouder et al. Reference Brouder, Gèze, Macquar and Paloc1977; Briand et al. Reference Briand, Couturié, Geffroy and Gèze1979; Gèze et al. Reference Gèze, Pellet, Paloc, Bambier, Roux and Senaud1980).
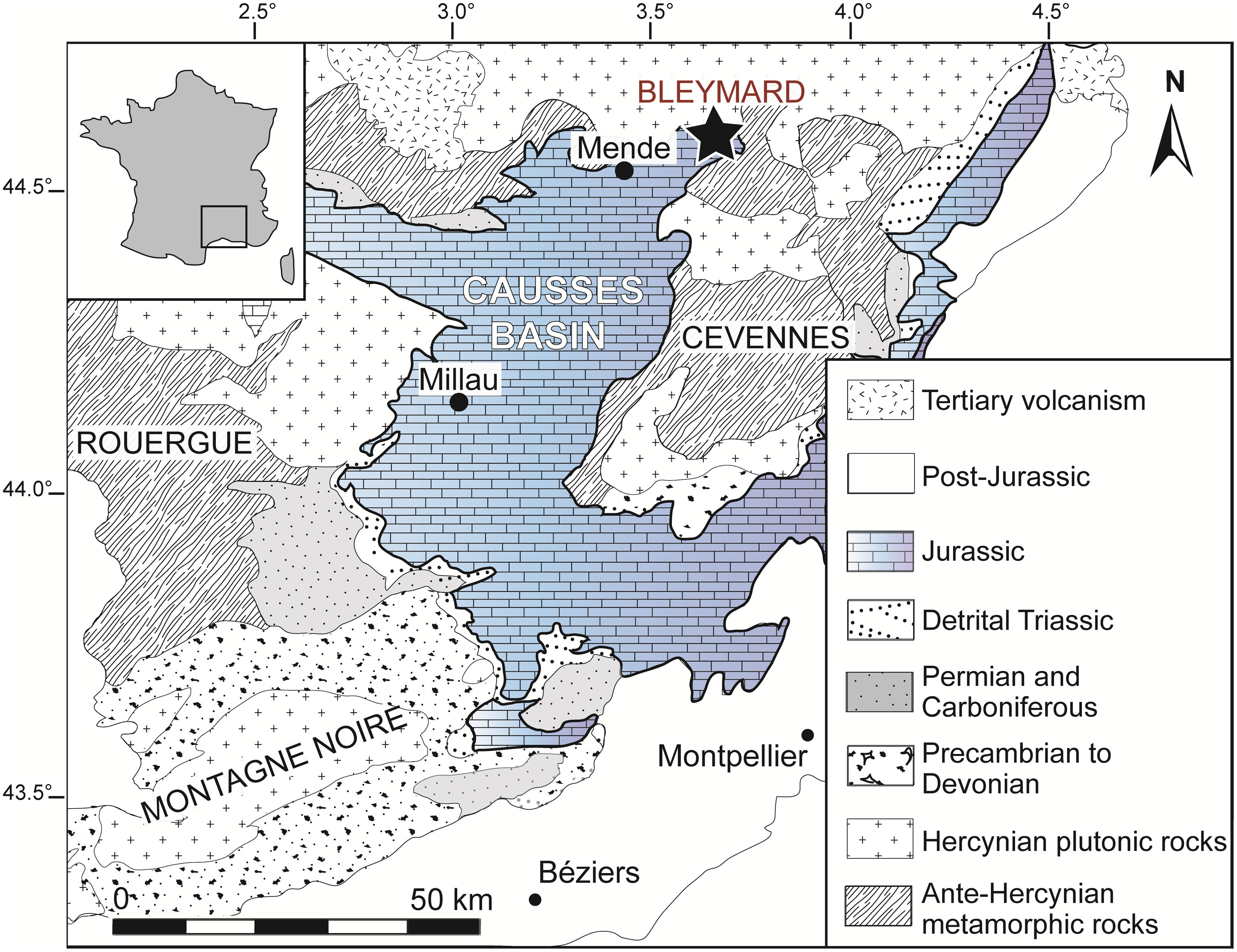
Fig. 1. Location and geological context of the Causses Basin with the geographical position of the Bleymard Strait.
The Detrital Sandstones – Variegated Mudstones Formation consists of rubefied lenticular and channelized sandstones that alternate with variegated argillite or marl (Simon-Coinçon, Reference Simon-Coinçon1989). Near Bleymard, the Detrital Sandstones – Variegated Mudstones Formation is poorly developed (c. 0–30 m thick; Briand et al. Reference Briand, Combémorel, Couturié, Bérard and Vaurelle1993) and is largely dominated by sandstone and conglomerate. According to Briand et al. (Reference Briand, Combémorel, Couturié, Bérard and Vaurelle1993), this formation does not yield any fossils near Bleymard. However, the Detrital Sandstones – Variegated Mudstones Formation yields rare plant impressions (e.g. Malafosse, Reference Malafosse1873; Saporta, Reference Saporta1891; Moreau & Thévenard, Reference Moreau and Thévenard2018) as well as dinosaur tracks (Moreau et al. Reference Moreau, Gand, Fara and Michelin2012 b) in the northern part of the Causses Basin. Plant remains consist of Bennettitalean vegetative structures ascribed to Otozamites latior Saporta and Bennettitalean fructification such as Weltrichia fabrei Saporta emend. Moreau & Thévenard (Saporta, Reference Saporta1891; Moreau & Thévenard, Reference Moreau and Thévenard2018).
Near Bleymard, the lower part of the Dolomitic Formation consists of dolomite as well as sublithographic limestone organized in massive layers (0–20 m thick), whereas the upper part of the formation consists of thin layers of yellow-coloured limestone alternating with green to blue argillite (20–40 m thick; Briand et al. Reference Briand, Combémorel, Couturié, Bérard and Vaurelle1993). The fossiliferous beds exposed in the Bleymard quarry are located in the lower part of the Dolomitic Formation. The rare ammonites (e.g. Psiloceras planorbis Sowerby; Brouder et al. Reference Brouder, Gèze, Macquar and Paloc1977) which were described from the Dolomitic Formation of Lozère confirm the Hettangian age of this formation.
3. Material and methods
3.a. Sedimentology and mineralogy
Detailed sedimentological, petrographic and mineralogical analyses were conducted along a 13 m thick stratigraphic section that could be accessed in the Bleymard quarry (Fig. 2). Fourteen lithostratigraphic levels were distinguished (Fig. 2). Each of them was sampled (BL.S1–BL.S14) in order to both conduct optical microscopy on standard polished thin-sections and to perform mineralogical analyses on ground samples. Based on field observations as well as microfacies and mineralogical analyses, we have characterized the evolution of the depositional environments along the section. X-ray fluorescence spectrometry (XRF) and X-ray diffraction (XRD) measurements were performed at the Civil and Environmental Engineering Department, LGCgE of IMT Lille–Douai, following the methods described in Moreau et al. (Reference Moreau, Trincal, André, Baret, Jacquet and Wienin2018) and Trincal et al. (Reference Trincal, Thiéry, Mamindy-Pajany and Hillier2018).

Fig. 2. Stratigraphic section of the Bleymard quarry showing the location of the dinosaur tracks and plant remains. Thi., thickness (m); Fac., facies; Sa., location of samples taken; Lev., levels; Lith., lithology.
XRF analyses were performed using a Bruker S4 Pioneer spectrometer and a 4 kW wavelength dispersive X-ray fluorescence spectrometer equipped with a rhodium anode. Measurements were taken at 60 keV and 40 mA on powdered rock-compressed tablets. XRD diffractograms were obtained with a Bruker D8 Advance diffractometer system using Co–Kα radiation equipped with a fast LynxEye position sensitive detector (WL = 1.74). The diffractometer was operated at 35 kV and 40 mA. Scans were run from 5° to 60–80°2θ, with a step interval of 0.02°2θ and a time acquisition of 96–192 s per step. Two protocols were used: the first to quantify the bulk rock minerals; the second for the clay fraction (particles < 2 μm) only.
Bulk rock minerals were identified from powders (thoroughly dried and micronized by grinding with an agate mortar and pestle) using the Bruker-AXS DiffracPlus EVA software and the International Centre for Diffraction Data (ICDD) Powder Diffraction File 2015 database. Quantification was achieved using the Rietveld method (e.g. Rietveld, Reference Rietveld1969; Snyder & Bish, Reference Snyder and Bish1989; Bish & Post, Reference Bish and Post1993) with the DIFFRACplus TOPAS software, version 4.2 (Bruker-AXS), and the crystal structure data were taken from the ICDD PDF and Bruker Structure Database. For more information about the Rietveld method and estimates of accuracy, refer to Taylor & Hinczak (Reference Taylor and Hinczak2006) and Trincal et al. (Reference Trincal, Charpentier, Buatier, Grobety, Lacroix, Labaume and Sizun2014).
Clay mineral extraction was carried out by sedimentation after a treatment with a weak-concentration hydrochloric acid. At least three XRD runs were performed on the oriented mounts following air-drying, ethylene-glycol solvation and heating at 550 °C for 2 h (Moore & Reynolds, Reference Moore and Reynolds1997; Thiry et al. Reference Thiry, Carrillo, Franke and Martineau2013). Clay minerals were identified according to the position of the (00l) series of basal reflections on the three XRD diagrams; qualitative quantification using the RIR method (e.g. Klug & Alexander, Reference Klug and Alexander1974; Hillier, Reference Hillier2000, Reference Hillier, Worden and Morad2003; Trincal et al. Reference Trincal, Thiéry, Mamindy-Pajany and Hillier2018). The intensity peaks of the illite 001 (10 Å) and kaolinite 001 (7 Å) were used with RIR ratios of 1 and 2.4, respectively (Hillier, Reference Hillier, Worden and Morad2003). The relative content between kaolinite and chlorite was evaluated using the intensities of the 002 peak (3.58 Å) for kaolinite and the 004 peak (3.54 Å) for chlorite (Biscaye, Reference Biscaye1964).
3.b. Palaeobotany
Plant beds in the Bleymard quarry were first observed by one of us (J.-D.M.) in 2009. Four levels yielded plant meso-remains: levels 1, 4, 7 and 12 (Fig. 2). In levels 1, 4 and 12, plants are rare and mainly preserved as tiny impressions (i.e. external casts < 1 cm) of wood fragments or isolated cuticles. However, level 7 yields abundant compressions preserving cuticles (Fig. 3a–b), and most of the specimens studied here were collected from this level. Plant remains vary greatly in size and consist of micro- to macro-remains. Wood remains consist of millimetric to pluricentimetric fragments. The collection includes hundreds of well-preserved specimens, and these consist of leafy axes, isolated leaves, cones, wood and pollen grains of conifers. Specimens are housed in the collections of the Musée du Gévaudan (Mende, Lozère, France).

Fig. 3. Facies and microfacies. (a–b) Dolomitic slabs from the main fossiliferous bed (facies F4) showing accumulations of conifer remains preserved as compressed cuticles; C., cones; L., leafy axes; specimens, (a) M486_2020.4_9, (b) M486_2020.4_1. (c–d) Sandy dolomite showing quartz grains (c) and dolomite crystals (d) (facies F1). (e) Dolomudstone showing some plant cuticles (facies F2). (f) Plant-bearing dolomite showing abundant plant cuticles (facies F4). Scale bars: (a–b) = 20 mm; (c, e–f) = 1 mm; (d) = 100 μm.
Cuticle analysis. Sediment was soaked in a solution of hydrogen peroxide (12 %) and water for a few days. The disaggregated sediment was then washed with tap water through a column of sieves (1.0 mm and 0.5 mm meshes). Fossils were picked out by naked eye or under a stereomicroscope. SEM micrographs were taken using a Hitachi TM-1000 scanning electron microscope at Biogéosciences, Univ. Bourgogne Franche-Comté (Dijon) and a JEOL JSM5600 scanning electron microscope in Femto laboratory, Univ. Bourgogne Franche-Comté (Besançon).
Wood analysis. Wood samples superficially look like fusain, as they are black, brittle and glossy. Microscopic examination, however, revealed that tracheid walls retained fibrous structure. The fossil wood is rather an evolved lignite, which was investigated by pyroxylin microcasting. Parlodion was dissolved in amylacetate to a thick paste-like consistency, and applied in a 1 mm-thick layer on an area selected under the microscope as featuring a well-preserved radial breaking plan. This was allowed to dry for 24 hours and then carefully lifted. This translucent peel was mounted on glass and examined under a microscope. This inexpensive, quick and easy method is not suitable for the preparation of photographs, as the peel surface is too irregular, but it is efficient for the observation of the characteristic wood features.
Pollen analysis. We used the following standard procedure: (1) immersion of 2 g of sediment in HCl (33 %, 1 h) to remove the carbonates; (2) digestion in HF (48 %, 12 h); (3) immersion in HCl (33 %, 4 h) to remove the fluorosilicates produced in the previous step; and (4) immersion in KOH (20 %, 10 h) in a heated bath to remove the organic matter. Next, the sample was sieved at 150 µm, and then we used heavy liquid (sodium polytungstate) at a specific gravity 2.2 kg m−3 to separate the pollen in the <150 µm fraction.
3.c. Ichnology
A single bed (level 14) yields tridactyl dinosaur tracks (Fig. 2). The track-bearing surface was discovered in 2015. The descriptive terminology and biometric parameters used are derived from Leonardi (Reference Leonardi1987) and Marty (Reference Marty2008). We used the following standard abbreviations: length of the trace, ‘L’; width of the trace, ‘W’; and divarication angle between digit II and digit IV, ‘II–IV’.
4. Results
4.a. Stratigraphy, petrography and mineralogy
Sedimentological, petrographic and mineralogical analyses distinguish five lithofacies, F1 to F5 (Table 1). The base of the stratigraphic section (from 0 to 2 m) displays plurimetric dunes showing large megaripple marks and oblique stratification (Fig. 2). These dunes correspond to F1 which consists of yellow to grey mudstone–packstone sandy dolomite locally yielding breccia and coquina lenses (Fig. 3c–d; Table 1). F1 is mainly composed of dolomite (weight percentage of 90 to 97 wt %) and quartz (1.5 to 6 wt %), with traces of clay confirmed by the presence of Al and Fe using XRF. Almost 80 wt % of illite and 20 wt % of kaolinite were identified among these clays (Figs 4–5; Fig. S1 and Tables S1–S3 in the Supplementary Material available online at https://doi.org/10.1017/S001675682100039X). Kaolinite peaks are very narrow, indicating that this mineral is very well crystallized. Quartz is also present in the clay fraction with an unusual high-intensity ratio between the (100) peak at 4.26 Å and the (101) peak at 3.33 Å.
Table 1. Microfacies. M, mudstone; W, wackestone; P, packstone; G, grainstone.


Fig. 4. Bulk rock XRD analyses of the Bleymard samples. The stratigraphic locations of samples BL.S1–BL.S14 are reported in Figure 2.

Fig. 5. Chemical, mineralogical and clay composition of the diverse levels from the Bleymard quarry. (a) Chemical composition obtained using XRF analyses. (b) Mineralogical composition obtained using XRD analyses. (c) Clay distribution in the <2 µm decarbonated fraction. The stratigraphic locations of samples BL.S1–BL.S14 are reported in Figure 2.
F2 corresponds to a brown to grey dolomudstone organized in planar pluridecimetric to metric beds (Fig. 2). F2 yields some tiny impressions (i.e. external casts) and cuticles of foliar plant remains, as well as wood fragments (Fig. 3e; Table 1). This facies is mainly composed of carbonates (85 to 98 wt % of dolomite and 0 to 10 wt % of calcite), as well as quartz and clay (mainly represented by illite) to a lesser extent (sum up to 5 wt %; Figs 4–5; S1–S3 in the Supplementary Material available online at https://doi.org/10.1017/S001675682100039X).
F3 consists of green-blue to black clay (mudstone) organized in up to 20 cm thick lenses and which is locally lignitic (Table 1). This facies displays a high content of clay (more than 72 wt %) and quartz (almost 17 wt %; Figs 3–4; Tables S1–S3 in the Supplementary Material available online at https://doi.org/10.1017/S001675682100039X). Compared with other facies, carbonates are scarce (less than 9 wt %) whereas titanium oxide is particularly abundant (almost 2 wt %; Supplementary Table S2). In all of the other facies, rutile is almost absent, as confirmed by XRF analyses where titanium is detected in trace amounts (i.e. ≤0.1%; Supplementary Table S1). The clay fraction is essentially composed of poorly crystallized illite showing a high 001/002 ratio suggesting an iron-rich composition of this mineral (Figs 4–5; Supplementary Fig. S1; Supplementary Tables S1–S3).
F4 is characterized by grey to blue, clayey dolomite (mudstone–packstone texture) showing lenticular, and dark, thin (millimetric to centimetric) lignitic layers bearing abundant plant micro- to macro-remains (Fig. 3a–b, f; Table 1). F4 characterizes the main fossiliferous bed (level 7) which is a 50 cm thick lens that pinches out laterally. Chemically and mineralogically, F4 is close to F1, but shows a little more clay which this time is more concentrated in the illite (Figs 4–5; Tables S1–S3 in the Supplementary Material available online at https://doi.org/10.1017/S001675682100039X).
Finally, F5 consists of grey-blue to yellow dolomite locally organized in millimetric laminites in the transverse section (Table 1). Bedding plane surfaces of laminites bear mud cracks. The microbial laminae are parallel to the bedding planes and are wavy to planar. They show alternating dark microbial laminae and more transparent laminae composed of dolomitic mudstone. The cryptalgal laminites mainly exhibit a porous fabric with bioturbations. At the top of the stratigraphic section, F5 bears dinosaur footprints (level 14). Microfossils are absent from F5. The chemistry and mineralogy of F5 display a strong similarity with F1 and F4 which consist of almost 90 wt % Mg-rich carbonates and 10 wt % silicates and phyllosilicates. Unlike all of the other facies containing kaolinite and sometimes chlorite, only illite was observed in the F5 decarbonated clay fraction (Figs 4–5; Tables S1–S3 in the Supplementary Material available online at https://doi.org/10.1017/S001675682100039X).
4.b. Systematic palaeontology
4.b.1. Plants
Order – Coniferales
Family – Cheirolepidiaceae
Leafy axes
Pagiophyllum peregrinum (Lindley & Hutton) A. Schenk emend. Kendall
Material. M486_2020.4_1a; M486_2020.4_2 to M486_2020.4_8; M486_2020.4_13 to M486_2020.4_17.
Description. The leafy axes are up to twice branched and straight to slightly curved (Fig. 6). The longest specimen is 12 cm long. The shoots are narrow and up to 7 mm in diameter. The leaves are persistent, helically arranged (phyllotaxy 2/5 and 3/8), appressed and imbricated (Fig. 6). The leaves are scale-like, triangular to rhomboidal, ‘longer than wide’ up to ‘as long as wide’, and keeled on the abaxial side (Figs 6–7). They are 2.0–4.1 mm long, 1.6–2.9 mm wide (based on 13 measured specimens). Apically, the leaves display a short free part which is 0.3–1.2 mm long. The abaxial surface of leaves is convex, whereas the adaxial surface is concave. The leaf margin is entire. The shape of the leaf apex is obtuse to slightly acute. The surface of leaves locally shows the outlines of epidermal cells, mainly near the margin. The adaxial cuticle of the leaves is slightly thicker than the abaxial cuticle; they are 3–11 μm and 3–7 μm thick, respectively. The leaves are amphistomatic. On the abaxial surface, the stomatal apparatuses are arranged in longitudinal rows that converge toward the leaf apex or are more or less randomly distributed (Fig. 8a–b). On the adaxial surface, they are more dispersed. The stomatal apparatuses of the abaxial surface are slightly larger than those of the adaxial surface. They are 44–66 μm long and 32–46 μm wide, and 37–52 μm long and 27–41 μm wide, respectively (Fig. 8c–d). The stomatal rows are not sunken, but the guard cells of individual stomata are sunken in a pit. The pit apertures are circular to oval, measuring 6–16 μm in diameter. Their orientation is not consistent. The pit apertures are oriented obliquely, longitudinally or transversally relative to the leaf margin. The subsidiary cells form a thick and well-marked rim around the pit apertures. The guard cells are not visible from the external surface of the cuticle. The ordinary epidermal cells are square, rectangular or polygonal, and are 9–53 μm long and 7–31 μm wide. They form longitudinal rows that are oriented parallel to the leaf axis (Fig. 8e–f). The anticlinal walls of ordinary epidermal cells are straight and are up to 2–6 μm thick. The outer periclinal walls of the epidermal cells are more or less convex but do not form individual papillae.

Fig. 6. Leafy axes of Pagiophyllum peregrinum from the Hettangian of Bleymard (level 7), stereomicroscopic images. (a–h) Narrow shoots showing persistent, helically arranged, highly appressed, imbricated and triangular to rhomboidal leaves. Specimens: (a) M486_2020.4_1a, (b) M486_2020.4_2, (c) M486_2020.4_3, (d) M486_2020.4_4, (e) M486_2020.4_8, (f) M486_2020.4_5, (g) M486_2020.4_6, (h) M486_2020.4_7. All scale bars = 2 mm.
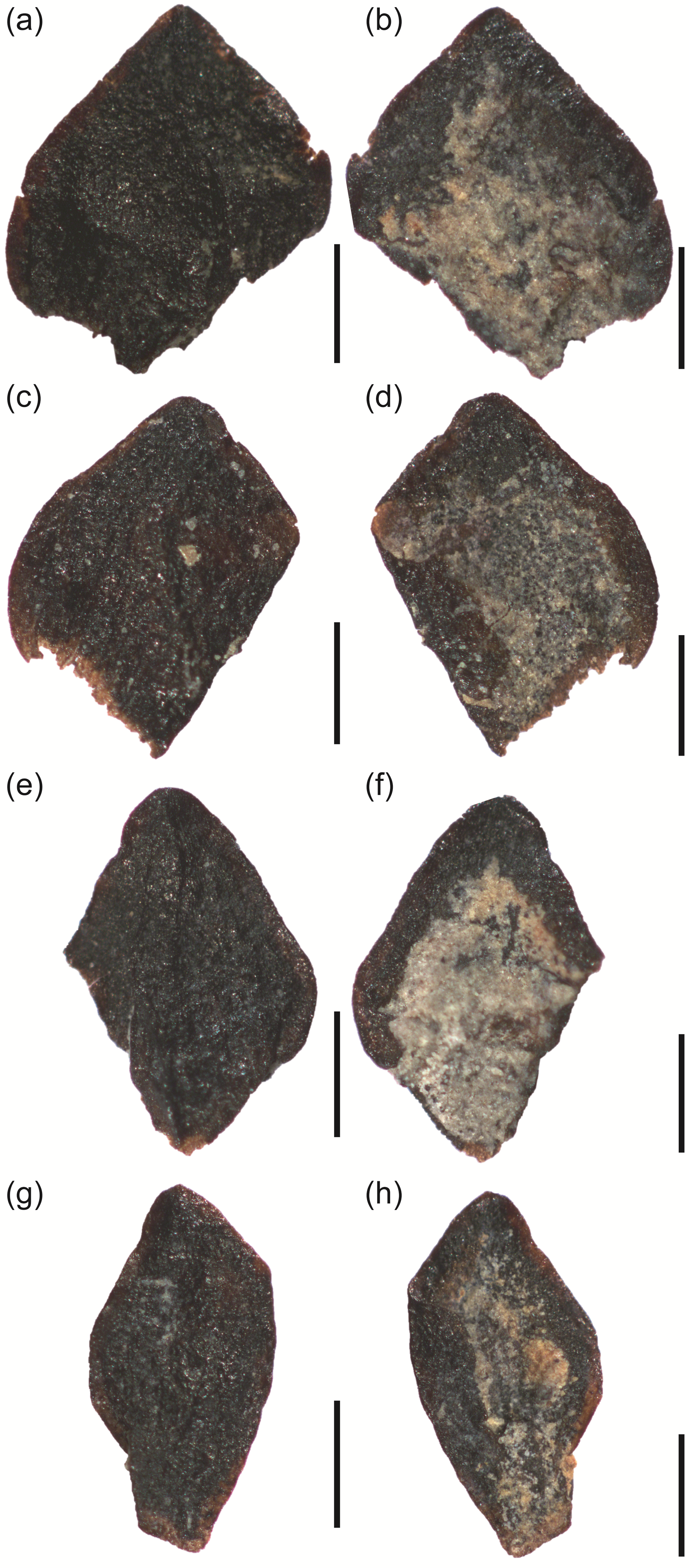
Fig. 7. Triangular to rhomboidal leaves of Pagiophyllum peregrinum from the Hettangian of Bleymard, stereomicroscopic images. The abaxial surface of the leaves (a, c, e, g) is convex, whereas the adaxial surface (b, d, f, h) is concave. Specimens: (a) M486_2020.4_13, (b) M486_2020.4_14, (c) M486_2020.4_15, (d) M486_2020.4_16. All scale bars = 1 mm.

Fig. 8. Pagiophyllum peregrinum, SEM microphotographs of the leaf cuticles. (a–b) Abaxial view showing the alignment of the stomatal apparatuses. (c–d) Stomatal apparatuses with a thick and well-marked rim around the pit apertures, adaxial surface. (e) Outer surface of the cuticle showing longitudinal parallel rows of rectangular, polygonal to square ordinary epidermal cell outlines, adaxial view. (f) Inner surface of the cuticle showing polygonal to square ordinary epidermal cells, adaxial view. Specimen: M486_2020.4_17. Scale bars: (a) = 1 mm; (b) = 250 μm; (c–f) = 25 μm.
Remarks. Although SEM micrographs provided information about the outer surface of the cuticle remains, their inner surface was commonly not well-preserved and therefore it was difficult to observe. The stomatal apparatuses were not well-preserved from the inner surface of the cuticle.
Pagiophyllum peregrinum (Lindley & Hutton) A. Schenk emend. Kendall and Brachyphyllum paparelii Saporta are the most common plant remains from the Hettangian–Sinemurian floras of the Causses Basin (Thévenard, Reference Thévenard1992, Reference Thévenard1993; Moreau et al. Reference Moreau, Philippe and Thévenard2019 b). However, although the gross morphology of P. peregrinum is highly variable (Thévenard & Barbacka, Reference Thévenard and Barbacka1999), it shares many similarities with B. paparelii (Thévenard, Reference Thévenard1993). As explained by Thévenard (Reference Thévenard1992), it is difficult to distinguish between both taxa when considering only the gross morphology of the leafy axes and leaves. The leaves of P. peregrinum can be as long as broad with a rounded apex, as well as longer than wide with an acute apex. Brachyphyllum paparelii and P. peregrinum can be almost exclusively distinguished by its cuticular features, mainly from stomatal apparatuses (Thévenard, Reference Thévenard1993). Both show haplocheilic stomatal apparatuses with typical subsidiary cells displaying rim-shaped thickening. However, in B. paparelii, this rim-shaped thickening bears clearly individualized papillae in the top part. These papillae are not present in P. peregrinum. We may note that some species of Brachyphyllum such as B. cyclophorum Reymanovna from the Liassic of Poland, B. ardenicum T. M. Harris from the Middle Jurassic of Yorkshire (Harris, Reference Harris1979), and B. trautii Barale & Contini from the Middle Jurassic of France (Barale, Reference Barale1981) also show an absence of papillae on stomatal apparatuses. Brachyphyllum cyclophorum differs from P. peregrinum in its irregular arrangement of the stomata (Thévenard, Reference Thévenard1993). Brachyphyllum ardenicum mainly differs in that it has a thicker cuticle and by the absence of stomatal apparatuses on the adaxial surface of the leaves (Harris, Reference Harris1979). Brachyphyllum trautii differs in that it shows scattered stomatal apparatuses that are never organized in longitudinal rows converging toward the leaf apex.
Pagiophyllum peregrinum was reported from several Hettangian–Sinemurian localities of France, with the most famous being from Ardennes, Aveyron, Hérault, Lozère and Vendée (Thévenard, Reference Thévenard1992; Thévenard et al. Reference Thévenard, Philippe and Barale1995, Reference Thévenard, Deschamps, Guignard and Gomez2003). The size of the leaves from Bleymard is slightly smaller than that of specimens commonly observed in the Causses Basin and Ardennes (Thévenard, Reference Thévenard1992; Thévenard et al. Reference Thévenard, Philippe and Barale1995). We can note that Pagiophyllum ?kurrii from the Hettangian of Aveyron (southern France; Thévenard, Reference Thévenard1992) and P. araucarinum (Pomel) Saporta from the Hettangian of Vendée (western France; Thévenard et al. Reference Thévenard, Deschamps, Guignard and Gomez2003) differ from P. peregrinum in the presence of well-developed papillae on the stomatal apparatuses.
Male cones
Classostrobus sp. Alvin, Spicer & J. Watson
Material. M486_2020.4_1b; M486_2020.4_9 to M486_2020.4_12.
Description. The five cones are spherical to ovoid, 7.0–15.5 mm long and 8.0–11.5 mm wide and bear helically arranged microsporophylls (Fig. 9a–d). The length/width ratio of the cones is 1.5–1.7. The microsporophylls are flattened to convex, triangular in shape and are longer than they are wide. They show a large base and an acute apex. The abaxial surface is slightly keeled along the total length of the microsporophyll. The microsporophylls are up to 4.8 mm long and up to 2.5 mm wide. The microsporophyll margin is entire.

Fig. 9. Classostrobus sp., light microscope microphotographs. (a–d) Spherical to ovoid cones showing helically arranged microsporophylls; the microsporophylls are flattened to greatly convex, triangular in shape, longer than wide, and they show a large base and acuminate apex. (e–g) Pollen grains of Classopollis classoides in the proximal polar view (e), distal polar view (f) and equatorial view (g). Specimens: (a) M486_2020.4_9, (b) M486_2020.4_10, (c) M486_2020.4_11, (d) M486_2020.4_12. Scale bars: (a–d) = 0.5 cm; (e–g) = 10 µm.
Remarks. SEM analyses reveal pollen grains along broken microsporophylls; however, it is not possible to determine the number and arrangement of the pollen sacs. The gross morphology and size of the grains (c. 20–25 µm) suggest an affinity with Classopollis sp. It can be noted that Classostrobus Alvin, Spicer & J. Watson species from several Jurassic localities yielded pollen grains ascribed to Classopollis H. D. Pflug or Corollina Malyavkina (Barale, Reference Barale1987; Van Konijnenburg-Van Cittert, Reference Van Konijnenburg-Van Cittert1987; Thévenard, Reference Thévenard1993).
In France, the male cone Classostrobus was rarely reported from the Hettangian–Sinemurian deposits (Barale, Reference Barale1987; Thévenard, Reference Thévenard1993). In the Causses Basin, only two Hettangian–Sinemurian localities yield specimens of the genus (Thévenard, Reference Thévenard1992). Based on seven specimens from Chaldecoste (Mende), Thévenard (Reference Thévenard1993) proposed a new species, C. lozerianus Thévenard. Although Classostrobus specimens from Bleymard are clearly bigger than those from Chaldecoste (see Thévenard, Reference Thévenard1993), male cones from the two localities show a similar gross morphology.
Wood
Brachyoxylon cf. voisinii Thévenard, Philippe & Barale
Material. M486_2020.4_18.
Description. The wood is a tracheidoxyl, with marked growth-rings and narrow late woods, limited to a few cell layers. No resin channels or axial parenchyma were observed. The tracheids often have a rounded cross-section, and their radial pits are found at the bottom of an oblique furrow (Fig. 10a), which suggests a compression wood. The tracheids are narrow, measuring roughly 10 µm in diameter. Tracheid pitting occurs on the radial wall only, with pits that are either round and spaced or contiguous and slightly compressed (Fig. 10a). As estimated from the limited possible observations, the two types of radial pitting are equally frequent and distributed randomly through the growth-rings. Rays are 2 to 9 cells high (mean = 3.6; n = 37), homogeneous and slightly heterocellular. The marginal ray cells are broader than inner ray cells, but of variable width, and their outer limit draws a wavy line (Fig. 10b). Ray cells are short, usually crossing no more than three tracheids. Their walls are thin and unpitted, except for the cross-fields. These have two to six cupressoid oculipores disposed in an araucarioid way (Fig. 10c).

Fig. 10. Schematic illustrations of wood. (a) Radial view, pitting on the radial wall of the tracheids, from early wood (right) to late wood (left); all pit pores are open at the bottom of an oblique furrow, suggesting wood compression or alteration. (b) Radial view, cellular detail of a wood ray; note the short ray cells and the wavy margins. (c) Radial view, four non-contiguous cross-fields; note the araucarioid disposition of the oculipores and the cracks resulting from alteration. Scale bars: (a–b) = 50 µm; (c) = 25 µm.
Remarks. Although we can confidently assign this specimen to Brachyoxylon Hollick & Jeffrey (Philippe & Bamford, Reference Philippe and Bamford2008), the limited nature of the observations, in particular of the radial pitting of the tracheids, means that we are less sure of the species identifications; we feel it most likely belongs to Brachyoxylon voisinii Thévenard, Philippe & Barale, though this is not certain. Brachyoxylon liebermannii Philippe has a more abietinean radial pitting of the tracheids and more pits per cross-field, while Brachyoxylon trautii (Barale) Philippe has a more araucarian radial pitting of the tracheids, where the abietinean pits are almost limited to late wood, and also with more pits per cross-field (Philippe, Reference Philippe1995). A well-preserved and very similar material from a nearby locality, Mende, was identified as B. voisinii (Moreau et al. Reference Moreau, Philippe and Thévenard2019 b).
Pollen grains
Classopollis classoides Pflug
Material. M486_2020.4_19.
Description. The pollen grains are oblate or isodiametric, and oval to circular in polar and equatorial view (Fig. 9e–g). The polar axis is 20–25 μm long and the equatorial axis is 15–20 µm long. The proximal pole shows a tetrad mark. The exine is tectate with infratectal structures and an equatorial rimula (infratectal striate band). The equatorial band is 5–7 µm wide. The exine is 1–2 µm thick.
Remarks. The specimens (n = 237) were counted under a light microscope and are all ascribed to Classopollis classoides H. D. Pflug. Distal cryptopores are not observed. This pollen was previously described as Corollina torosus (Reissinger) Klaus in the Hettangian deposits from the Causses Basin (Thévenard, Reference Thévenard1992, Reference Thévenard1993). As explained in Jersey & McKellar (Reference Jersey and McKellar2013), ‘Traverse (Reference Traverse2004) proposed conservation of the name Classopollis against Corollina and Circulina. This proposal, recommended by the Committee for Fossil Plants (Skog, Reference Skog2005), was adopted by the International Botanical Congress, Vienna, 2005 (McNeill et al. Reference McNeill, Barrie, Burdet, Demoulin, Hawksworth, Marhold, Nicolson, Prado, Silva, Skog, Wiersema and Turland2006)’. Traverse (Reference Traverse2004) stated: ‘Couper (Reference Couper1958) formally transferred Pollenites torosus to Classopollis torosus, and this is often cited as the correct name for Classopollis classoides. Reissinger’s (Reference Reissinger1950) Pollenites torosus was not validly published because it lacks a description or diagnosis, as required by the ICBN [International Code of Botanical Nomenclature]’. In this context we follow the suggestions of Traverse (Reference Traverse2004) and Jersey & McKellar (Reference Jersey and McKellar2013), and prefer to use Classopollis classoides rather than Corollina torosus.
4.b.2. Dinosaur tracks
The observed tracks consist of three tridactyl footprints comprising traces of digits II, III and IV. They are weakly marked and consist of concave epireliefs. The footprints may obtain a score of 1 to 2 as per the morphological preservation scale of Marchetti et al. (Reference Marchetti, Belvedere, Voigt, Klein, Castanera, Díaz-Martínez, Marty, Xing, Feola, Melchor and Farlow2019). The dinosaur footprints are all isolated and no trackway was observed. Two morphotypes can be distinguished.
Morphotype 1
Description. The first morphotype consists of an isolated footprint (Bl_T1; Fig. 11a–b). The track is longer than wide (L/W = 1.3), 29.0 cm long and 21.5 cm wide. The divarication angle II–IV is 33°. Impressions of the digits are quite well-defined and elongated. The traces of digits III and II are the longest and the shortest imprints, respectively. The position of the digito-metatarsal pad of digit IV is more proximal than for digit II. There is no plantar impression.

Fig. 11. Tridactyl dinosaur footprints from the Hettangian of the Bleymard quarry. (a–b) Morphotype 1: photograph (a) and interpretative sketch (b). (c–g) Morphotype 2: photographs (c, e), interpretative sketches (d, f) and digital elevation model (g). Specimens: (a–b) = Bl_T1; (c–d) = Bl_T2; (e–g) = Bl_T3. All scale bars = 10 cm.
Remarks. These tracks share similarities with Grallator Hitchcock from the Early Jurassic of the Causses Basin: they are longer than wide and have a long free part of digit III, a small divarication angle II–IV and slender digit impressions. In the Hettangian–Sinemurian deposits of the Causses Basin, four ichnospecies of Grallator were previously reported, G. lescurei Demathieu, G. minusculus Hitchcock, G. sauclierensis Demathieu & Sciau, and G. variabilis de Lapparent & Montenat (Demathieu et al. Reference Demathieu, Gand, Sciau and Freytet2002). The dimensions of the track included in Morphotype 1 are close to those of G. lescurei and G. minusculus (L = 12.5–36.0 cm and 24–35 cm, respectively; Demathieu et al. Reference Demathieu, Gand, Sciau and Freytet2002). However, based on a single and poorly preserved footprint, this material cannot be conclusively ascribed to an ichnospecies.
Morphotype 2
Description. The second morphotype is represented by two isolated footprints (Bl_T2 and Bl_T3; Fig. 11c–d, e–f). The tracks are as long as they are wide (L/W = 1.0–1.1), 22.0 cm long and 15.5–22.0 cm wide. The divarication angle II–IV varies from 51° to 57°. The digit impressions are moderately elongated and show claw marks. On Bl_T2, the imprints of the pads are preserved and are circular to oval. The impression of the proximal plantar surface is marked on Bl_T2 (Fig. 11c–d).
Remarks. These tracks differ from Morphotype 1 because the footprints are as long as they are wide, and they show a larger divarication angle II–IV. These features are similar to those of tracks previously ascribed to Dilophosauripus williamsi Welles and which were reported from several Hettangian–Sinemurian tracksites in France (e.g., Demathieu & Sciau, Reference Demathieu and Sciau1992; Demathieu, Reference Demathieu1993; Demathieu et al. Reference Demathieu, Gand, Sciau and Freytet2002; Demathieu & Gand, Reference Demathieu and Gand2003; Sciau, Reference Sciau2003; Gand et al. Reference Gand, Demathieu and Montenat2007; Moreau et al. Reference Moreau, Trincal, Gand, Néraudeau, Bessière and Bourel2014). Dilophosauripus williamsi was established based on tracks from the Early Jurassic of the United States of America (Welles, Reference Welles1971). Given that the type material is poorly preserved, the validity of this ichnotaxon was strongly debated (Lockley & Hunt, Reference Lockley and Hunt1995; Lucas et al. Reference Lucas, Klein, Lockley, Spielmann, Gierliński, Hunt and Tanner2006; Lockley et al. Reference Lockley, Gierliński and Lucas2011; Gand et al. Reference Gand, Fara, Durlet, Caravaca, Moreau, Baret, André, Lefillatre, Passet, Wiénin and Gély2018). It is interesting to note that some authors consider Dilophosauripus as a junior synonym of Eubrontes Hitchcock (see references in Lucas et al. Reference Lucas, Klein, Lockley, Spielmann, Gierliński, Hunt and Tanner2006; Lockley et al. Reference Lockley, Gierliński and Lucas2011). Recently, based on morphometric similarities, Gand et al. (Reference Gand, Fara, Durlet, Caravaca, Moreau, Baret, André, Lefillatre, Passet, Wiénin and Gély2018) recommended using Kayentapus rather than Dilophosauripus for the lowermost Jurassic tracks of the Causses Basin.
5. Discussion
5.a. Depositional palaeoenvironments
Carbonate platform sediments of the Hettangian Dolomitic Formation were deposited in the context of the earliest Jurassic Tethyan marine transgression (Hamon, Reference Hamon2004). The lithological characteristics as well as the co-occurrence of marine and terrestrial organisms suggest that the sediments from the Bleymard quarry were deposited in a panel of marginal-littoral palaeoenvironments (Table 1). Based on field observations, together with microfacies and mineralogical analyses, we identified various depositional environments (Table 1).
At the base of the stratigraphic section, the sandy dolomite displaying megaripple marks with oblique stratifications and coquinas (F1) was deposited in shoreface environments regularly impacted by storm currents (Swift et al. Reference Swift, Figueiredo, Freeland and Oertel1983). Laterally, the local breccia lenses bearing limestone lithoclasts correspond to very proximal and high-energy foreshore domains (beach?). The alternation between dolomudstone organized in planar beds and yielding plants (F2) with lenses of lignitic clay (F3) marks the installation of lower-energy, restricted and shallow water environments. The abundance of leafy axes, cones, wood and pollen of conifers in level 7 (F4) indicates strong terrestrial inputs. The absence of marine organisms in the plant-bearing levels suggests that the environment was probably not open to the sea and that it was locally paralic. The preservation of long conifer twigs and the absence of morphological sorting indicate that when the plant debris was transported, it was not over a long period of time, over a long distance or under low hydrodynamism. It suggests the para-autochthony of the remains composing the taphocoenosis. In the upper part of the section, the cryptalgal laminites of the F5 facies are characteristic of regularly flooded intertidal and supratidal zones (e.g. Alsharhan & Kendall, Reference Alsharhan and Kendall2003; Hamon, Reference Hamon2004; Matysik, Reference Matysik2016). The lack of bioclasts and coarse sediment suggests limited storm-generated transport. All the elements of this facies may reflect a relative distance to the subtidal zone. At the top of the section, the surfaces bearing mud cracks, ripple-marks and dinosaur footprints indicate that sediments were deposited in environments with a thin layer of water which were periodically emerged (tidal flat). The absence of invertebrate skeletons suggests restricted life conditions, probably related to high salinity. At Mende, 25 km west of the Bleymard quarry, the Dolomitic Formation yielded halite pseudomorphs that attest to local evaporitic conditions (Moreau, Reference Moreau2011).
5.b. Origin and significance of clay minerals
Two types of clay assemblages are identified, either a mixture of illite and kaolinite in dolomitic facies or a clay fraction composed entirely of illite in marly layers (Fig. 5c). As shown by Daoudi & Deconinck (Reference Daoudi and Deconinck1994), the very good crystallinity of kaolinite in a porous dolomitic facies suggests an authigenic origin. In the Hettangian Dolomitic Formation from the Escalette section (50 km south of the Bleymard quarry; Marza et al. Reference Marza, Séguret and Moussine-Pouchkine1998), the pervasive authigenic development of kaolinite in the porosity of the dolomites has been observed by SEM (JF Deconinck, unpublished data). However, SEM observations of several samples from the Bleymard quarry did not show clay particles, probably because the total amount of clay mineral in these facies is too small. Therefore, our data cannot be used to definitively conclude the authigenic origin of the kaolinite, but this origin is highly suspected based on the XRD profiles. The unusual high-intensity ratio of the diffraction peaks of the quartz at 4.26 and 3.33 Å occurring in the clay fraction of the dolomitic facies (Figs 4–5; Fig. S1 and Tables S1–S3 in the Supplementary Material available online at https://doi.org/10.1017/S001675682100039X) suggests that the tiny quartz grains are authigenic in origin, which is common in evaporitic environments (Chen et al. Reference Chen, Chafetz, Andreasen and Lapen2016).
In the Mesozoic sediments, clay fractions composed of pure illite are very uncommon, since the erosion of continental areas leads to mixtures of minerals including chlorite, illite, smectite and kaolinite for the most encountered clay minerals. A monomineral clay fraction composed of pure illite is difficult to explain in terms of detrital inputs since illite usually indicates the deep erosion of crystalline rocks (Chamley, Reference Chamley1989). Such intense erosion is not consistent with the sedimentological context of the study area corresponding to a carbonate platform. Therefore, a detrital origin of illite can be discarded.
Similarly, pure, poorly crystallized illitic clay fractions were first described in Purbeckian sediments (Jurassic/Cretaceous transition) outcropping in the Jura Mountains (Deconinck & Strasser, Reference Deconinck and Strasser1987; Deconinck et al. Reference Deconinck, Strasser and Debrabant1988, Reference Deconinck, Gillot, Steinberg and Strasser2001) where illitic minerals originated from the illitization of smectite at surface temperature in shallow supratidal environments subjected to wetting and drying cycles. This process was subsequently described in Cenozoic formations on the Isle of Wight (southern England; Huggett & Cuadros, Reference Huggett and Cuadros2005, Reference Huggett and Cuadros2010). The depositional environments of the Bleymard samples are quite similar to that described for the Purbeckian environments. Therefore, we suggest that the illite from the marly layers interstratified in the dolomitic succession have the same origin, i.e. an illitization of smectite at surface temperature in a carbonate platform environment submitted to repeated wetting (by seawater) and subsequent drying cycles. These conditions clearly indicate that a warm and probably seasonally humid climate prevailed during the Hettangian in southern France.
5.c. Implications for the dinosaur ecosystem and palaeoecophysiological insights
In the Causses Basin, theropod footprints were reported in several layers that correspond to deposits ranging from the Hettangian to the Sinemurian (Demathieu et al. Reference Demathieu, Gand, Sciau and Freytet2002). However, bone remains of dinosaurs are still unknown in the Early Jurassic formations from this area. As shown by well-preserved tracks from the Dolomitic Formation (Demathieu et al. Reference Demathieu, Gand, Sciau and Freytet2002), Grallator and Kayentapus are tridactyl footprints showing a similar phalangeal formula (type 3, 4 and 5 for toes II, III and IV). This deduced osteological architecture corresponds to that of theropod dinosaurs (Baird, Reference Baird1957; Olsen et al. Reference Olsen, Smith and McDonald1998; Demathieu et al. Reference Demathieu, Gand, Sciau and Freytet2002). Body fossils of earliest Jurassic theropods were ascribed to Coelophysidea and Ceratosausoria and reported in Africa, Antarctica, China and the USA (Weishampel et al. Reference Weishampel, Dodson and Osmolska2004; Smith et al. Reference Smith, Makovicky, Hammer and Currie2007; Xing et al. Reference Xing, Bell, Rothschild, Ran, Zhang, Dong, Zhang and Currie2013). In Europe, rare theropod body fossils were reported in Hettangian deposits of England, France, Italy and Luxembourg (Larsonneur & Lapparent, Reference Larsonneur and Lapparent1966; Carrano & Sampson, Reference Carrano and Sampson2004; Delsate & Ezcurra, Reference Delsate and Ezcurra2014; Dal Sasso et al. Reference Dal Sasso, Maganuco and Cau2018). In France, there is a unique occurrence of theropod bones in the Moon-Airel Formation (Normandie; Larsonneur & Lapparent, Reference Larsonneur and Lapparent1966). This material was ascribed to the Coelophysidae Liliensternus airelensis by Cuny & Galton (Reference Cuny and Galton1993) and later assigned to Lophostropheus airelensis (Ezcurra & Cuny, Reference Ezcurra and Cuny2007).
In Early Jurassic deposits from France, plant remains are rarely found associated with dinosaur footprints (Sciau, Reference Sciau1992). Similarly to the Veillon tracksite (in western France) which shows a co-occurrence of dinosaur tracks and abundant conifer cuticles (Lapparent & Montenat, Reference Lapparent and Montenat1967; Thévenard et al. Reference Thévenard, Deschamps, Guignard and Gomez2003), the Bleymard outcrop is an opportunity to better understand the ecosystems in which dinosaurs roamed. Although plant remains are abundant in level 7, they are clearly weakly diversified as they are all related to Cheirolepidiaceae. Similarly, other studies have reported the co-occurrence of Pagiophyllum, Classostrobus, Brachyoxylon and Classopollis (Thévenard, Reference Thévenard1993). It is tempting to hypothesize that twigs, cones, wood and pollen grains from Bleymard belong to the same conifer. However, since Classopollis is also known to co-occur with other cone and twig genera (e.g. Frenelopsis; Kvaček, Reference Kvaček2000; see also discussion in Van Konijnenburg-Van Cittert, Reference Van Konijnenburg-Van Cittert1987) this hypothesis cannot be verified. In other Hettangian–Sinemurian floras from the Causses Basin, Barale et al. (Reference Barale, Philippe and Thévenard1991) and Thévenard (Reference Thévenard1992) estimated that conifers constituted ~98 % of foliar remains (mainly Pagiophyllum peregrinum and Brachyphyllum paparelii) and Pteridospermales (Thinnfeldia obtusa A. Schenk and Thinnfeldia rhomboidalis Ettingsh.) made up the remaining ~2 %. In addition, rare Cycadales (Ctenozamites Nathort emend. T. M. Harris), Filicales (Clathropteris platyphylla Brongn.) and Ginkgoales (Eretmophyllum caussenense Thévenard; see Saporta, Reference Saporta1873; Ressouche, Reference Ressouche1910; Thévenard, Reference Thévenard1992; Moreau et al. Reference Moreau, Philippe and Thévenard2019 b) were reported from other Hettangian–Sinemurian plant-yielding localities from the Causses Basin (e.g. Mende in Lozère). Similarly to data obtained from the Bleymard material, xylological analyses ascribed wood from the Causses Basin to conifers alone (Thévenard, Reference Thévenard1993; Moreau et al. Reference Moreau, Philippe and Thévenard2019 b).
Although the paucity of the plant assemblage contained in level 7 (Fig. 2) needs to be carefully interpreted, the taphonomy suggests that the taphocoenosis probably reflected the composition of the local flora. Garcia et al. (Reference Garcia, Philippe and Gaumet1998) stated that Brachyoxylon-dominated wood assemblages usually originate from nearby carbonate platforms. Such a poorly diversified conifer-dominated plant assemblage is common in the Laurasian littoral floras during the Hettangian (e.g. Thévenard, Reference Thévenard1992, Reference Thévenard1993; Thévenard et al. Reference Thévenard, Deschamps, Guignard and Gomez2003). From an ecophysiological point of view, some xerophytic characteristics of conifers such as Brachyphyllum and Pagiophyllum clearly show that the flora of the Causses Basin and the Bleymard Strait was adapted to withstand intense sunlight and coastal environments exposed to desiccant conditions coupled with salty sea spray, and dry conditions: fleshy shoots; adapted leaf morphology (short and small leaf pressed against the axis); thick cuticles; sunken stomata apparatuses with subsidiary cells forming a thick rim and sometime bearing papillae limiting the water loss; and narrow tracheids, even in the early wood. These features are those of a conifer-dominated flora in a tropical to subtropical climate with contrasted seasons cyclically dry (Thévenard et al. Reference Thévenard, Deschamps, Guignard and Gomez2003; Karakitsios et al. Reference Karakitsios, Kvaček and Mantzouka2015). Similar Hettangian Pagiophyllum-dominated floras were reported from several plant beds deposited in littoral context (western France; Thévenard et al. Reference Thévenard, Deschamps, Guignard and Gomez2003). This suggests that the optimal habitat for P. peregrinum was a marginal-littoral environment that probably had strong marine inputs. In Europe, during the Jurassic, the wood genus Brachyoxylon had the southernmost distribution of a set of six wood genera (Philippe et al. Reference Philippe, Puijalon, Suan, Mousset, Thévenard and Mattioli2017), and can probably be considered as indicative of a warm subtropical climate. Brachyoxylon was also hypothesized as characteristic of a tropophilous climate, with a marked alternation between a wet and a dry season (Oh et al. Reference Oh, Legrand, Kim, Philippe and Paik2011). Here, palynology also supports this hypothesis, with Classopollis being mainly reported from sediments deposited in a littoral context and under a warm tropophilous climate (Srivastava, Reference Srivastava1976; Vakhrameev, Reference Vakhrameev1981, Reference Vakhrameev1987).
Some lowermost Jurassic archosaur tracksites from the Causses Basin also yield rare trackways ascribed to Batrachopus and which attest to the presence of crocodylomorphs in a theropod-rich ecosystem (Demathieu & Sciau, Reference Demathieu and Sciau1992; Demathieu et al. Reference Demathieu, Gand, Sciau and Freytet2002; Moreau et al. Reference Moreau, Fara, Néraudeau and Gand2019 a). Moreover, rare footprints from the Hettangian dolomite of Aveyron and Lozère were tentatively ascribed to ornithopods (Demathieu et al. Reference Demathieu, Gand, Sciau and Freytet2002; Moreau, Reference Moreau2011). Considering the local presence of woodlands along the Hettangian–Sinemurian coasts, we could suppose that this vegetation was probably an attractive and important source of food for megaherbivorous dinosaurs. However, data collected from more than 60 Hettangian–Sinemurian tracksites of the Causses Basin (e.g. Demathieu et al. Reference Demathieu, Gand, Sciau and Freytet2002; Moreau et al. Reference Moreau, Trincal, Gand, Néraudeau, Bessière and Bourel2014, Reference Moreau, Trincal, André, Baret, Jacquet and Wienin2018), as well as traces from the Bleymard Strait, suggest that lowermost Jurassic dinosaur communities from these areas were mainly composed of theropods. Since Hettangian sauropod tracks were abundantly reported in marginal-marine paralic facies from other areas of Laurasia (Gierliński et al. Reference Gierliński, Pieńkowski and Niedźwiedzki2004), the absence of their trackways in the littoral environments of the Causses Basin and the Bleymard Strait raises questions.
6. Conclusions
-
The quarry studied here constitutes the first dinosaur tracksite reported from the Bleymard Strait. It represents one of the rare Hettangian archosaur tracksites from France yielding plant-rich beds. The two morphotypes of tridactyl dinosaur tracks share similarities with Grallator and Kayentapus. The plant assemblage exclusively yields remains of Cheirolepidiaceae conifers (Pagiophyllum peregrinum, Classostrobus sp., Brachyoxylon sp., Classopollis classoides).
-
Sedimentological, petrological and mineralogical analyses demonstrated that, in the Dolomitic Formation from Bleymard, the palaeoenvironment progressively evolved from shoreface/foreshore domain (facies F1) to a restricted shallow environment that was only partially/occasionally open to the sea (facies F2 to F4), then an intertidal to supratidal zone (tidal flat; facies F5).
-
This study supports that theropods were abundant and particularly adapted to tidal flats that were periodically emerged and bordering paralic environments inhabited by littoral Cheirolepidiaceae-dominated forests.
-
The xerophytic characteristics of the flora as well as the clay mineral analyses suggest tropical to subtropical climate with contrasting seasons, cyclically dry then humid.
Supplementary material
To view supplementary material for this article, please visit https://doi.org/10.1017/S001675682100039X
Acknowledgements
We would like to express our gratitude to the Society Llorens and particularly Guillaume Llorens who authorized the access to the quarry. We thank Lara Sciscio, Johanna HA van Konijnenburg-van Cittert and the anonymous reviewer for their constructive and thoughtful reviews of the manuscript. We thank Emilie Steimetz for her technical contribution during SEM investigations. This research did not receive any specific grants from any funding agencies, commercial or not-for-profit sectors.
Declaration of interest
The authors have no known conflict of interest.