Introduction
Amoebozoa, including species of Entamoeba (mainly Entamoeba coli, Entamoeba histolytica/dispar and Entamoeba hartmanni), Endolimax nana and Iodamoeba bütschlii are among the most prevalent single-celled parasites colonizing the gut of Tunisian healthy individuals (Siala et al., Reference Siala, Toumi, Bettaieb, Boulehmi, Zallega, Aoun and Bouratbine2015). Unlike E. histolytica, none of these protists are considered pathogens, but rather thought of as commensals and not taken into consideration in the clinical-diagnostic setting (Fotedar et al., Reference Fotedar, Stark, Beebe, Marriott, Ellis and Harkness2007). Nevertheless, recent data indicate remarkable genetic diversity among these genera (Stensvold et al., Reference Stensvold, Lebbad, Victory, Verweij, Tannich, Alfellani, Legarraga and Clark2011b, Reference Stensvold, Lebbad and Clark2012; Poulsen and Stensvold, Reference Poulsen and Stensvold2016), the clinical and public health importance of which remains to be explored. Moreover, recent studies on gut microbiota have increased the knowledge of the influence of gut microbes on human health and disease, highlighting several associations between single-celled parasites and bacteria as potential indicators of clinical phenotypes (Berrilli et al., Reference Berrilli, Di Cave, Cavallero and D'Amelio2012; Andersen et al., Reference Andersen, Vedel Nielsen and Stensvold2013; Morton et al., Reference Morton, Lynch, Froment, Lafosse, Heyer, Przeworski, Blekhman and Segurel2015; Andersen and Stensvold, Reference Andersen and Stensvold2016; Iebba et al., Reference Iebba, Santangelo, Totino, Pantanella, Monsia, Di Cristanziano, Di Cave, Schippa, Berrilli and D'Alfonso2016; O'Brien Andersen et al., Reference O'Brien Andersen, Karim, Roager, Vigsnaes, Krogfelt, Licht and Stensvold2016; Beghini et al., Reference Beghini, Pasolli, Truong, Putignani, Caccio and Segata2017; Stensvold and van der Giezen, Reference Stensvold and van der Giezen2018). Hence, studies of commensal amoeba colonizing the human gut of both healthy and diseased individuals have gained novel significance.
While microscopy is still the cornerstone of diagnostic testing for intestinal protozoa, particularly amoeba of the digestive system, this approach is labour-intensive and requires a high level of skill for accurate identification (McHardy et al., Reference McHardy, Wu, Shimizu-Cohen, Couturier and Humphries2014). Its diagnostic yield is also significantly impacted by the number of stool specimens collected and submitted for testing (Bouratbine et al., Reference Bouratbine, Aoun, Siala, Chahed, Ben Hassine and Meherzi2000). In fact, many intestinal protozoa are irregularly shed, and at least three sequential stool specimens are necessary for reliable detection of intestinal parasites in routine laboratory examinations (Nazer et al., Reference Nazer, Greer, Donnelly, Mohamed, Yaish, Kagalwalla and Pavillard1993). In contrast, the enhanced sensitivity of molecular detection methods may require only one specimen for testing to achieve sensitivity equal to, if not greater than, microscopy (Bruijnesteijn van Coppenraet et al., Reference Bruijnesteijn van Coppenraet, Wallinga, Ruijs, Bruins and Verweij2009). Furthermore, polymerase chain reaction (PCR)-based methods also enable species differentiation in case of protozoa with similar morphological features such as the pathogenic E. histolytica and the commensal E. dispar and Entamoeba moshkovskii (Ben Ayed et al., Reference Ben Ayed, Aoun, Maamouri, Ben Abdallah and Bouratbine2008a, Reference Ben Ayed, Ben, Mousli, Aoun, Thellier and Bouratbine2008b).
Over the past 20 years, there has been a remarkable development in molecular biology based diagnostic procedures to detect E. histolytica, to the point where, today, such DNA-based methods are the preferred approach (Tanyuksel and Petri, Reference Tanyuksel and Petri2003). In this context, Department of Parasitology, Pasteur Institute of Tunis has offered since 2009 two standardized real-time quantitative PCRs (qPCRs) for detection and identification of E. histolytica and E. dispar. These are routinely used for accurate identification of E. histolytica and E. dispar in food handlers with amoeba infection initially diagnosed by microscopy and patients with symptoms suggestive of intestinal amoebiasis (Bouratbine et al., Reference Bouratbine, Maamouri, Ben Abdallah, Aoun, Haouet, Boubaker, Ben Jilani, Ghorbel, Najjar and Ben Ammar2003; Ben Ayed et al., Reference Ben Ayed, Ben, Mousli, Aoun, Thellier and Bouratbine2008b). These two PCRs were designed and evaluated on a series of clinical stool samples analysed by microscopy and corresponding conventional PCR (data not shown). In contrast, data on the molecular identification of E. hartmanni, E. coli, E. polecki, E. nana and I. bütschlii has so far been very limited (Clark and Diamond, Reference Clark and Diamond1997; Silberman et al., Reference Silberman, Clark, Diamond and Sogin1999; Santos et al., Reference Santos, Bandea, Martins, de Macedo, Peralta, Peralta, Ndubuisi and da Silva2010; Stensvold et al., Reference Stensvold, Lebbad and Verweij2011a, Reference Stensvold, Lebbad, Victory, Verweij, Tannich, Alfellani, Legarraga and Clark2011b, Reference Stensvold, Lebbad and Clark2012, Reference Stensvold, Winiecka-Krusnell, Lier and Lebbad2018; Gomes Tdos et al., Reference Gomes Tdos, Garcia, de Souza Cunha, Werneck de Macedo, Peralta and Peralta2014; Poulsen and Stensvold, Reference Poulsen and Stensvold2016).
The aim of this study was to develop and evaluate a panel of specific PCRs targeting ‘non-pathogenic’ amoebic species of epidemiological importance in Tunisia, namely E. hartmanni, E. coli, E. nana and Iodamoeba RL1 and Iodamoeba RL2, which altogether represent about 50% of intestinal single-celled parasites colonizing Tunisian healthy individuals (Siala et al., Reference Siala, Toumi, Bettaieb, Boulehmi, Zallega, Aoun and Bouratbine2015). Although, the presence of E. polecki cysts have never been reported in Tunisia, their morphological features which may be confused with those of other Entamoeba species have justified their research by a recently available molecular tool enabling the identification of all four subtypes (ST1–ST4) of E. polecki (Stensvold et al., Reference Stensvold, Winiecka-Krusnell, Lier and Lebbad2018).
Materials and methods
Faecal specimens
Thirty-six faecal samples for which the presence of parasites had been documented by microscopy were used for the study. Eighteen samples contained at least one amoeba species whereas the 18 other specimens were amoeba-negative (Table 1). All specimens were provided by the Laboratory of Parasitology and Mycology, Pasteur Institute of Tunis, and reflected anonymous samples and were received in the setting of routine diagnosis for stool parasite testing and screened using the conventional ‘ova and parasites’ examination. Faecal specimens used in this study had been preserved in S.T.A.R. buffer (Roche Diagnostics, Indianapolis, IN, USA) at −20 °C prior to DNA extraction.
Table 1. Comparison of microscopy and PCR results on the 36 samples included in the study

a No nucleotide sequences available.
DNA extraction
Total genomic DNA was extracted from the stool samples using the QIAamp DNA Stool MiniKit (Qiagen, Inc., Valencia, CA, USA) with bead beating prior to DNA extraction. Briefly, 250 µL of stool specimen was added to 1.4 mL of the stool lysis buffer (ASL) provided by the Qiagen kit, the suspension was mixed with Glass beads (500 mg; diameter, 0.5 mm; Sigma), centrifuged at 700 g for 3 min and incubated for 5 min at 70 °C prior to DNA extraction according to the manufacturer's recommendations. All DNA samples were stored at −20 °C until use.
Real-time qPCRs identifying E. histolytica and E. dispar
The 36 DNA extracts were subjected to PCR-based screening for E. histolytica and E. dispar. Two in-house real-time qPCR assays routinely used in the Laboratory of Parasitology and Mycology at the Pasteur Institute of Tunis for E. histolytica and E. dispar identification were applied in this study. These two qPCRs are based on the amplification of the small subunit (SSU) rRNA genes of E. histolytica and E. dispar and use two species-specific forward primers Eh 196F: AAATGGCCAATTCATTCAATGA and Ed 185F: GTATTAGTACAAAGTGGCCAATTTATGT and the same reverse primer Ehd294R: CATTGGTTACTTGTTAAACACTGGTG and 6-FAM/MGB-labelled probe (FAM-AGGATGCCACGACAA-NFQ) that are complementary to a region of homology between the two species. Real-time qPCRs were performed in a final volume of 20 µL using a TaqMan universal master mixture (Applied Biosystems, USA) containing 300 nm of each primer, 200 nm of probe and 5 µL of DNA extract. The DNA was amplified in an Applied Biosystems® PCR machine (Applied Biosystems, Foster City, CA) using 40 cycles at 95 and 60 °C.
Conventional PCRs identifying E. coli, E. hartmanni, E. polecki, E. nana and I. bütschlii
The 36 DNA extracts were subjected to PCR-based screening for E. coli, E. hartmanni, E. polecki, E. nana and Iodamoeba RL1 and Iodamoeba RL2. Published primers targeting E. coli and E. polecki 18S SSU rRNA genes were used for E. coli and E. polecki species identifications (Stensvold et al., Reference Stensvold, Lebbad, Victory, Verweij, Tannich, Alfellani, Legarraga and Clark2011b, Reference Stensvold, Winiecka-Krusnell, Lier and Lebbad2018) (Table S1). Moreover, four sets of primers were designed for species identification of E. hartmanni, E. nana, Iodamoeba RL1 and Iodamoeba RL2, respectively. Primers were designed using Primer/Blast software (http://ncbi.nlm.nih.gov/tools/primer-blast) based on SSU rRNA gene sequences available in GenBank: E. hartmanni AF149907 (1960 bp), E. nana AF149916 (2589 bp), Iodamoeba RL1 JN635741.1 (2376 bp) and Iodamoeba RL2 JN635740.1 (2187 bp). Selected primers (Table S1) were checked for sequence specificity using Nucleotide Basic Local Alignment Search Tool (BLASTN) (https://blast.ncbi.nlm.nih.gov). DNA amplification was performed in a total volume of 25 µL with 1× reaction buffer (10 mm Tris-HCl [pH 8.3], 50 mm KCl), 2.5 mm MgCl2, 200 mm (each) deoxynucleotide triphosphate, 15 pm (of each) primer, 0.25 U of Taq polymerase (ATGC, Noisy le Grand, France) and 5 µL of template DNA or water (negative control). For E. polecki PCR, E. polecki ST4 (UNE25) DNA kindly provided by the Public Health Agency of Sweden was used as positive control. Thermal cycle reactions were set to (i) an initial denaturing step at 95 °C for 7 min followed by (ii) 40 cycles of a denaturing step at 95 °C for 30 s, an annealing step at (55° for 30 s for E. polecki, 58 °C for 30 s for E. nana and E. hartmanni, 60 °C for 30 s for Iodamoeba RL1 and RL2 and 62 °C for 30 s for E. coli) and an extension step at 72 °C for 30 s and (iii) a final extension step at 72 °C for 7 min. Tubes were kept at 4 °C until removal from the thermocycler (T100™ Thermal Cycler, Bio-Rad, California, USA). PCR products were separated by electrophoresis on a 2% agarose gel in Tris/acetate/EDTA (pH 8.0) buffer, stained with Midori Green Advance DNA Stain (Nippon Genetics Europe GmbH, Germany) and visualized on an UV trans-illuminator. PCR products were purified using a NucleoSpin® extract II kit (Macherey-Nagel GmbH & Co. KG, Germany) and cycle-sequenced using an ABI Prism® Big Dye™ Terminator, Cycle Sequencing Ready Reaction Kit and AB1 3130 sequencing system (ABI, PE Applied Biosystems), with the same primers as used for PCR. DNA sequences from both strands were edited and assembled into one contig using the Staden software package (http://staden.sourceforge.net/). MEGA version 7 software (www.megasoftware.net) was used to perform multiple sequence alignment (ClustalW option) and for phylogenetic analysis. For each species, relationships between specimens and reference isolates were inferred based on genetic distances using the neighbour joining (NJ) method with the Kimura 2 parameters model. Statistical support for tree distances was evaluated using bootstrapping (1000 replicates).
Accession numbers
Nucleotides sequences were deposited in the GenBank database (http://www.ncbi.nlm.nih.gov/) under accession numbers MG925061–MG925076.
Results
Overall results
The 18 specimens amoeba-positive by microscopy were identified at the species/ribosomal lineage level by PCR-based methods. Molecular species identification was 100% concordant with microscopic results (Table 1). Moreover, the PCR assays identified amoebae in several microscopy negative samples and revealed more samples with a mixture of amoeba species than microscopy (22 vs 18 and 7 vs 1, respectively) (Table 1).
Moreover, species specific PCR-based methods enabled the species differentiation between E. dispar and E. histolytica (Table 1) and confirmed the absence of E. polecki in all study specimens.
Entamoeba coli-specific PCR
Entamoeba coli-specific PCR was positive for seven samples. Six of these samples had been observed to contain cysts of E. coli, and in the remaining sample, only cysts of Giardia had been observed (Table 1). The E. coli in the seven specimens reflected four different SSU rDNA sequences (GenBank acc. nos., MG925061–MG925064). Phylogenetic analyses of these DNA sequences using E. histolytica/dispar/moshkovskii as out-group positioned the seven specimens in the same cluster as sequences of E. coli collected by other authors (Fig. 1A). Furthermore, DNA sequencing allowed the identification of E. coli subtypes and classified samples as ST1 (n = 5) and ST2 (n = 2) (Fig. 1A).
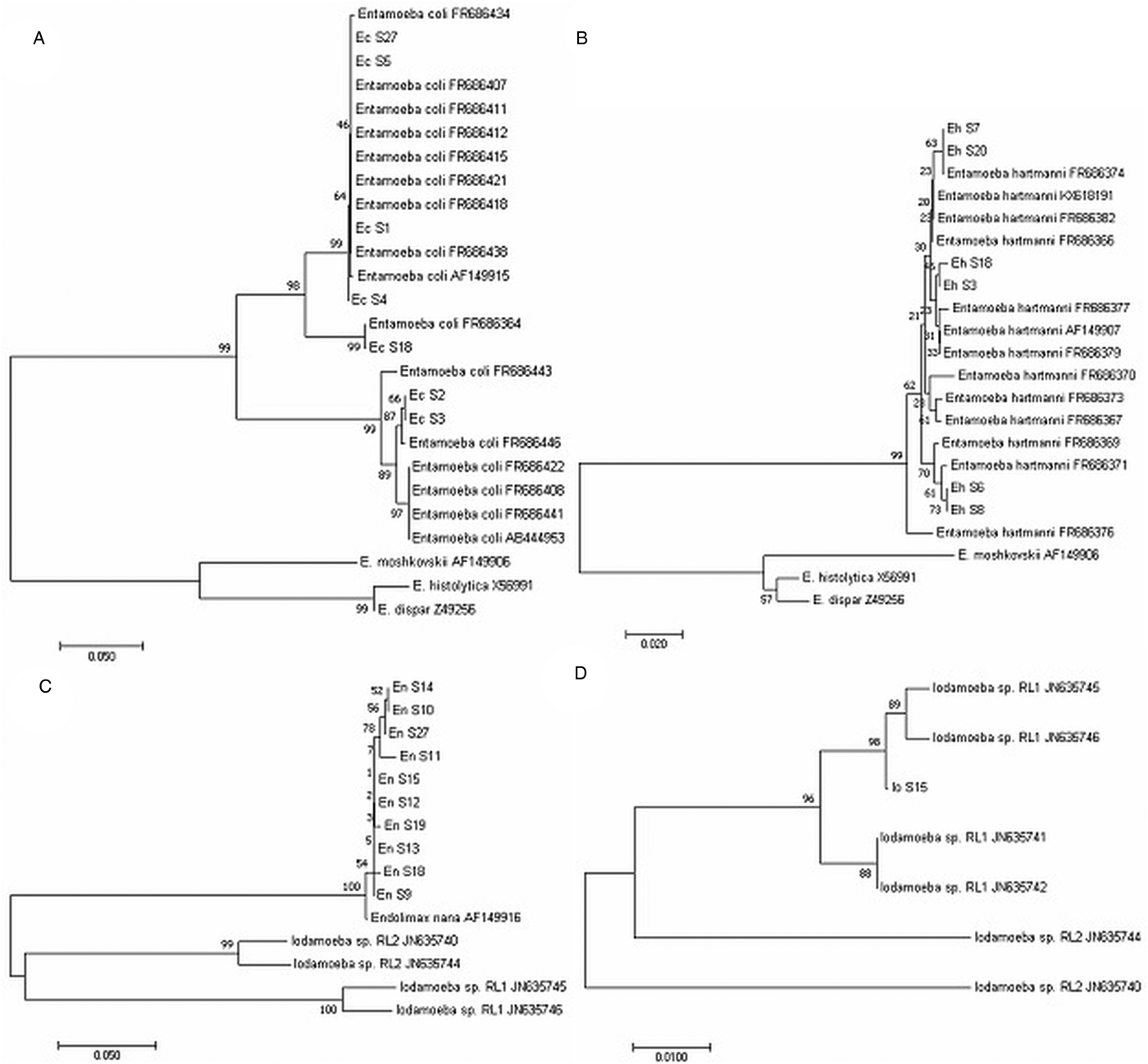
Fig. 1. Phylogenetic trees showing intra-specific variations in intestinal amoeba species. The consensus tree was based on the distance NJ using 1000 replicates samples. (A) Entamoeba coli across the 500 bp sequence, (B) Entamoeba hartmanni across the 300 bp sequence. Sequences from Entamoeba histolytica, Entamoeba dispar and Entamoeba moshkovskii were used as outgroup for both species. (C) Endolimax nana across the size between 400 and 700 bp sequence. Sequences from Iodamoeba RL1 and RL2 were used as outgroup. (D) Iodamoeba across the 460 bp sequence. Sequence from E. nana was used as outgroup.
Entamoeba hartmanni-specific PCR
Entamoeba hartmanni-specific PCR was positive for eight samples (Fig. 2A). Three of these samples were microscopy positive for E. hartmanni cysts, three for cysts of E. nana and E. coli and two for Blastocystis only (Table 1). DNA sequencing was possible only for six samples out eight (Table 1) that corresponded to four different sequences identified by sequence alignment and visual inspection of the sequence traces and deposited in the GenBank under accession numbers MG925065–MG925068. Phylogenetic analyses of rDNA sequences with E. histolytica/dispar/moshkovskii as out-group positioned the six specimens in the same cluster as sequences from E. hartmanni collected by other authors (Fig. 1B).

Fig. 2. Stained agarose gel electrophoresis with products amplified by specific amoeba species SSU rDNA PCRs. (A) Entamoeba hartmanni PCR: S7, S6, S12, S20, S18, S3 positive samples showing a band at expected size (300 pb); S16, S17, S2, S9, S25 negative samples. (B) E. nana PCR: S9, S10, S19, S18 positive samples showing a band at expected size (740 pb); S1, S3, S31: negative samples. (C) Iodamoeba RL1 and RL2 PCRs: S15 positive sample by Iodamoeba RL1 PCR showing a band at expected size (590 pb); S9, S17, S2: negative samples. M: 100-bp ladder; NC: negative control.
Endolimax nana-specific PCR
Endolimax nana-specific PCR was positive for 11 samples (Fig. 2B). Seven samples were positive for Endolimax cysts by microscopy, one for cysts of I. bütschlii, and three were known to contain other protists, namely Blastocystis and Giardia (Table 1). DNA sequencing was possible only for ten samples out of 11 (Table 1) that corresponded to six different sequences (GenBank acc. nos., MG925069–MG925075). Phylogenetic analyses of the DNA sequences using Iodamoeba as out-group positioned the ten specimens in the same cluster as the single E. nana reference sequence AF149916 available in the GenBank (Fig. 1C). Phylogenetic tree also indicated that Endolimax from this study may constitute a phylogenetically distinct group from the Endolimax reported in the GenBank. However, because the low bootstrap values, genetic variation in the SSU rRNA gene among Endolimax could not be considered (Fig. 1C).
Iodamoeba specific PCRs
For Iodamoeba, two PCRs were applied in order to identify the two lineages RL1 and RL2. RL1 specific PCR was positive for one sample (Fig. 2C) that contained Iodamoeba cysts while RL2 specific PCR was negative for all samples (Table 1). Nucleotide sequence alignment was deposited in the GenBank under accession number MG925076. Phylogenetic analyses of rDNA sequences with Endolimax as out-group positioned the specimens in the same cluster as sequences from Iodamoeba of RL1 lineage characterized by other authors (Fig. 1D).
Discussion
Based on a series of morphological key differences, microscopy allows the differentiation between the three genera of Amoebozoa infecting the human gut. In fact, mature cysts of Endolimax contain four nuclei and those of Iodamoeba contain only one, but these nuclei differ markedly in appearance from their counterparts in Entamoeba. Within the genus Entamoeba, several morphological differences including, cyst size, number of nuclei, appearance of the peripheral chromatin, size of the karyosome, presence/absence and appearance of chromatoid bars, presence/absence of inclusion bodies are often used for Entamoeba species differentiation. For instance, E. coli which forms large cysts containing eight nuclei can easily be distinguished from other Entamoeba species. However, cysts of genetically different E. coli strains may differ in size and smaller immature forms may be mistaken for E. histolytica/E. dispar (Ben Ayed et al., Reference Ben Ayed, Ben, Mousli, Aoun, Thellier and Bouratbine2008b). Similarly, E. hartmanni exhibits morphological structures similar to the features of E. histolytica/dispar complex which could lead to misdiagnosis. However, E. hartmanni cysts are generally smaller than those of the E. histolytica/dispar complex (Burrows, Reference Burrows1959). Thus, for microscopic differentiation an appropriate morphometric analysis is generally required. In the other hand, immature cysts of E. histolytica/dispar may have only one nucleus and it may be almost impossible to differentiate such cysts from those of E. polecki, a uninucleated species of Entamoeba, the cysts of which are similar in size to those of E. histolytica and E. dispar (Stensvold et al., Reference Stensvold, Winiecka-Krusnell, Lier and Lebbad2018). Hence, morphological diagnosis of Entamoeba species is still a renowned challenge in clinical microbiology laboratories and lack of data on several Entamoeba species such as E. polecki, may be related to the possibility that they are confused with other Entamoebas (Stensvold et al., Reference Stensvold, Winiecka-Krusnell, Lier and Lebbad2018). Moreover, E. histolytica cysts are morphologically indistinguishable from those of E. dispar and E. moshkovskii, which is why they are often reported at a complex level (Fotedar et al., Reference Fotedar, Stark, Beebe, Marriott, Ellis and Harkness2007; Ben Ayed et al., Reference Ben Ayed, Aoun, Maamouri, Ben Abdallah and Bouratbine2008a, Reference Ben Ayed, Ben, Mousli, Aoun, Thellier and Bouratbine2008b).
The target gene of many diagnostic PCRs for intestinal parasites is the SSU rRNA gene which is present in all eukaryotes, often even in high copy numbers (Verweij and Stensvold, Reference Verweij and Stensvold2014). SSU rDNA sequence data are available for the vast majority of intestinal parasites and offer good resolution in terms of detecting and differentiating species and subtypes of Amoebozoa found in the human intestinal tract. These data were exploited to design oligos used in PCRs targeting E. histolytica, E. dispar, E. coli, E. hartmanni, E. polecki, E. nana and I. bütschlii. Each of the developed PCR was species-/lineage-specific and at least as or even more sensitive than microscopic examination, which highlights the diagnostic and epidemiological relevance of applying DNA-based methods for detecting and differentiating amoebae in faecal samples.
Genetic diversity within the genus Entamoeba has been subject to substantial investigation (Clark and Diamond, Reference Clark and Diamond1997; Silberman et al., Reference Silberman, Clark, Diamond and Sogin1999; Stensvold et al., Reference Stensvold, Lebbad, Victory, Verweij, Tannich, Alfellani, Legarraga and Clark2011b). Riboprinting supported the division into groups based on the number of nuclei present in the mature cyst (Clark and Diamond, Reference Clark and Diamond1997). Most phylogenetic reconstructions supported monophyly of the Entamoebidae and were largely in accord with riboprinting results. Thus, species of Entamoeba producing cysts with the same number of nuclei formed monophyletic groups. The most basal Entamoeba species are those producing cysts with eight nuclei, while the group producing four-nucleated cysts was most recently derived (Silberman et al., Reference Silberman, Clark, Diamond and Sogin1999). Among Entamoeba species, E. coli exhibits the highest intragenetic diversity (more than 15% polymorphic sites across the SSU rRNA gene) (Stensvold et al., Reference Stensvold, Lebbad and Verweij2011a). It comprises at least two major subtypes, ST1 and ST2, with no absolute host specificity (Stensvold et al., Reference Stensvold, Lebbad, Victory, Verweij, Tannich, Alfellani, Legarraga and Clark2011b). In our study, using published primers, we identified both of these two subtypes. ST1 however, was more prevalent than ST2. On the other hand, primers for detecting E. hartmanni were published and used by Suzuki et al. (Reference Suzuki, Kobayashi, Murata, Tajima, Hashizaki, Yanagawa and Takeuchi2008). However, recent accumulation of information on intra-specific sequence variation of E. hartmanni species allowed a re-evaluation of these primers (Stensvold et al., Reference Stensvold, Lebbad, Victory, Verweij, Tannich, Alfellani, Legarraga and Clark2011b). In fact, in light of recent E. hartmanni sequencings, it appeared that some of the E. hartmanni SSU rDNA sequence variants might not have been amplifiable using those primers as sequence variation exists in both of the primer binding regions, raising questions about the prevalence data generated based on those primers (Stensvold et al., Reference Stensvold, Lebbad, Victory, Verweij, Tannich, Alfellani, Legarraga and Clark2011b). Taking into account the more recently published E. hartmanni sequences, we designed two specific primers for PCR-based detection. These primers turned out to be both specific and sensitive. All six sequences fell into a single clade in the same cluster as sequences from E. hartmanni collected by other authors. Multiple sequence alignment confirmed the low intra-specific variation within this species (Clark and Diamond, Reference Clark and Diamond1997; Stensvold et al., Reference Stensvold, Lebbad, Victory, Verweij, Tannich, Alfellani, Legarraga and Clark2011b).
Of the so-called non-pathogenic intestinal protozoa, Endolimax and Iodamoeba belong to the least well-described amoeba infecting human gut. Most data on these parasite protists have emerged from general surveys of intestinal parasites, and the two genera remain largely unexplored in terms of genetic diversity, host specificity and molecular epidemiology (Stensvold et al., Reference Stensvold, Lebbad and Clark2012; Poulsen and Stensvold, Reference Poulsen and Stensvold2016). In their phylogenetic analysis, Silberman et al. placed Endolimax as a sister taxon to the Entamoeba assemblage (Silberman et al., Reference Silberman, Clark, Diamond and Sogin1999). Sequencing the SSU rRNA gene of Iodamoeba, Stensvold et al. (Reference Stensvold, Lebbad and Clark2012) found that Endolimax and Iodamoeba grouped together. Recently, however, and mostly due to availability of more sequences from related organisms, Zadrobílková et al. were able to obtain monophyly for both Endolimax and Iodamoeba (Zadrobilkova et al., Reference Zadrobilkova, Walker and Cepicka2015). In 2016, Poulsen and Stensvold (Reference Poulsen and Stensvold2016) developed specific primers based on the single complete SSU rRNA gene sequence of Endolimax in the GenBank. However, these later primers have proved partially effective for diagnosing E. nana in genomic DNA extracted from fresh stool. In-house primers developed in this study let to successful amplification in all samples positive for Endolimax by microscopy. DNA sequencing grouped all human specimens together and suggested two phylogenetically distinct groups within Endolimax: one group from human origin and the second from non-human primate. In fact, the only available complete SSU rRNA gene sequence of Endolimax is from grey cheeked mangabey, Cercocebus albigena (National Zoological Park, Washington, DC, 1991). In all cases, designing genus-specific primers based on a single DNA sequence remains problematic and more sequence data is needed to better evaluate primer design and intra-genetic variability (Poulsen and Stensvold, Reference Poulsen and Stensvold2016). Concerning Iodamoeba, taking into account its remarkable degree of genetic diversity and the presence of two ribosomal lineages (RL1 and RL2), two RL-specific PCRs were designed. However, given its relative rarity, only one sample containing Iodamoeba cyst was available for PCR analysis. This sample was positive by the RL1-specific PCR and negative by the RL2-specific PCR. DNA sequencing confirmed its ribosomal lineage (RL1).
There can be little doubt that in the next decades, screening for intestinal parasites by PCR will become more and more common, and might replace microscopy to a large extent. Real-time PCR-based methods are nowadays increasingly used for intestinal protozoa screening including E. histolytica (ten Hove et al., Reference ten Hove, van Esbroeck, Vervoort, van den Ende, van Lieshout and Verweij2009; Stensvold and Nielsen, Reference Stensvold and Nielsen2012; Cimino et al., Reference Cimino, Jeun, Juarez, Cajal, Vargas, Echazu, Bryan, Nasser, Krolewiecki and Mejia2015; Efunshile et al., Reference Efunshile, Ngwu, Kurtzhals, Sahar, Konig and Stensvold2015; Won et al., Reference Won, Kim, Kee, Shin, Suh, Chai, Ryang and Shin2016; Abu-Madi et al., Reference Abu-Madi, Boughattas, Behnke, Sharma and Ismail2017; Incani et al., Reference Incani, Ferrer, Hoek, Ramak, Roelfsema, Mughini-Gras, Kortbeek and Pinelli2017). Compared with conventional PCR, it has the advantages of increased specificity because of the role of the probe, reduced risk of contamination and the capacity of multiplexing different targets and quantification (Verweij and Stensvold, Reference Verweij and Stensvold2014). However, in the interpretation of PCR results, one should keep in mind that because of the specificity of the primers and probe, the only sequences detected will be those from which they were designed. While in the future, amplicon-based approaches coupled to next generation sequencing (NGS) may prove useful and represent a viable method for detecting and differentiating amoebae in faecal samples, a major limitation to completing our ability to detect and differentiate amoebae in stool samples is the lack of data on diversity within the Amoebozoa infecting man and other host species in publicly available databases and complete ribosomal genes are necessary for phylogeny and epidemiology (host specificity). NGS/shotgun on faecal genomic DNA will only give us fragments. Nevertheless, NGS-based technology may reveal intra-specific variation within species that to some extent might prove useful for differentiation between the various subtypes and lineages.
Supplementary material
The supplementary material for this article can be found at https://doi.org/10.1017/S0031182018002196.
Author ORCIDs
Christen R. Stensvold 0000-0002-1417-7048
Acknowledgements
The authors thank Najette Zallaga and Nada Boulehmi from the Department of Clinical Parasitology, Institut Pasteur de Tunis for their kind contribution to the technical aspect of this work. They also thank Soumaya Ben Ayed and Emmanuelle Delabesse who contributed to the standardization and evaluation of the two real-time qPCRs used for detection and identification of E. histolytica and E. Dispar and Marianne Lebbad from Public Health Agency Sweden for providing E. Polecki ST4 DNA.
Financial support
This study was supported by the Ministry of Higher Education and Scientific Research, Tunisia in the setting of the Research Laboratory LR 16-IPT-06.
Conflict of interest
None.
Ethical standards
Not applicable.