Introduction
Approximately one in eight couples suffer from subfertility (Sharlip et al., Reference Sharlip, Jarow, Belker, Lipshultz, Sigman, Thomas, Schlegel, Howards, Nehra, Damewood, Overstreet and Sadovsky2002; Kumar and Singh, Reference Kumar and Singh2015), defined as the failure to conceive in the fertile phase of the menstrual cycles after 1 year of unprotected intercourse (Evers, Reference Evers2002; Gnoth et al., Reference Gnoth, Godehardt, Frank-Herrmann, Friol, Tigges and Freundl2005). About half of the cases can be ascribed to male factors (de Kretser, Reference de Kretser1997; Evers, Reference Evers2002). Cryptorchidism, a urogenital birth defect, is a common male factor likely to contribute to subfertility (Lee et al., Reference Lee, Bellinger, Songer, O’Leary, Fishbough and LaPorte1993). Cryptorchidism is characterized by the absence of one (unilateral) or two (bilateral) testes in the scrotum, i.e. without the testis descending from the abdominal cavity to the scrotum through the inguinal canal. It has been known that the temperature in the scrotum is usually 2–8°C lower than that in the abdominal cavity (Ivell, Reference Ivell2007), and is crucial to spermatogenesis. Indeed, previous studies have shown that raised temperature by surgical induction of cryptorchidism impairs spermatogenesis, devastates spermatogenic cells and facilitates the generation of multinucleated giant cells, further expediting the process of germ cell removal (Clegg, Reference Clegg1963; Liu et al., Reference Liu, Huang, Xu, Qian and Qiu2012). Nevertheless, the underlying mechanism for this type of germ cell death has been poorly studied.
Cryptorchidism has been reported to induce germ cell apoptosis in mice (Ogi et al., Reference Ogi, Tanji, Yokoyama, Takeuchi and Terada1998) and rats (Henriksen et al., Reference Henriksen, Hakovirta and Parvinen1995). Kocak and colleagues (Kocak et al., Reference Kocak, Dundar, Hekimgil and Okyay2002) considered apoptosis rather than atrophy or necrosis as a principal reason for germ cell death in the cryptorchid testis. Apoptosis, also called type I programmed cell death (PCD), is an energy-dependent active biological process of cellular decomposition characterized by pronounced morphological transformations. Apoptosis can alternatively depend on caspase, and caspase-dependent apoptosis is mainly triggered by the intrinsic mitochondrial pathway and the extrinsic death-receptor pathway (Elmore, Reference Elmore2007; Peterson et al., Reference Peterson, Timmons, Mondragon and McCall2015; Xu et al., Reference Xu, Dong and Yang2016). Previous studies have demonstrated that the intrinsic mitochondrial pathway (Absalan et al., Reference Absalan, Movahedin and Mowla2010; Jung et al., Reference Jung, Yon, Lin, Jung, Lee, Baek, Lee, Yun and Nam2015), the Fas system (Ogi et al., Reference Ogi, Tanji, Yokoyama, Takeuchi and Terada1998) and the p53 signalling pathway (Yin et al., Reference Yin, DeWolf and Morgentaler1998; Absalan et al., Reference Absalan, Movahedin and Mowla2010) are involved in germ cell apoptosis in the cryptorchid testis. Yet, it remains indefinite whether both intrinsic and extrinsic apoptotic pathways are implicated in this process. In addition, it is unclear when cell apoptosis is initiated and which types of spermatogenic cells undergo apoptosis in the cryptorchid testis.
Unlike apoptosis, autophagy is an evolutionarily conserved intracellular catabolic process. By forming a peculiar double-membrane structure called autophagosome, a portion of the cytoplasm and organelles are engulfed and delivered to the lysosome for degradation (Nakatogawa et al., Reference Nakatogawa, Suzuki, Kamada and Ohsumi2009; Liu et al., Reference Liu, Chen, Gao, Gu, Yang, Peng, Xu, Wang, Yang, Liu, Fan, Chen, Zhou, Sun, Ruan, Cheng, Komatsu, White, Li, Ji, Finley and Hu2014; Ohsumi, Reference Ohsumi2014; Kliosnky et al., Reference Kliosnky2016). Autophagy can consistently occur in all cells at a basal level and increase under various stress conditions such as the presence of intracellular pathogens or inflammation (Deretic, Reference Deretic2006; Chen and Klionsky, Reference Chen and Klionsky2011), oxidative stress (Coto-Montes et al., Reference Coto-Montes, Boga, Rosales-Corral, Fuentes-Broto, Tan and Reiter2012), growth factor withdrawn (Song et al., Reference Song, Yoon, Kim, Sim, Kim, Cha, Choi, Min, Lee, Huh, Lee, Kim, Koo, Choo, Kim, Kim and Chang2012) or heat stress (Zhang et al., Reference Zhang, Jiang, Bi, Zhu, Zhou and Sha2012). The induction of autophagy and subsequent germ cell death by heat stress suggests that autophagy may also occur in germ cells of the cryptorchid testis that, however, has not been probed before.
In this present study, by using an artificial cryptorchid mouse model, we aimed to increase knowledge about the mechanisms for cryptorchidism-induced male infertility. To be more specific, we aimed to answer the following questions: (1) What are the exact effects of cryptorchidism on spermatogenic cells and seminiferous epithelial cycles? (2) Are apoptosis and autophagy both involved in germ cell death in the cryptorchid testis? (3) If so, what is their crosstalk?
Materials and methods
Animals
Adult (aged 8–9 weeks) Kunming male mice, weighing 34±2 g were obtained from the Fourth Military Medical University (Xi’an, China). All mice were used and maintained under a controlled environment of 25±5°C, 12 h/12 h light/dark cycles, and 30–70% humidity, with food and water ad libitum.
Unilateral surgery-induced cryptorchidism
Surgery was performed under avertin (Sigma-Aldrich, 20 µl/g) anaesthesia. A midline abdominal incision was made, and the right testis was pulled into the abdominal cavity and sutured to the abdominal wall, while the left testis was maintained in the scrotum. The right testis of control mice was subjected to a sham surgery.
Testicular tissue collection and treatment
Overall, 72 mice were used for three independent experiments (n=3). Specifically, in each independent experiment, 21 mice were subjected to surgery-induced cryptorchidism, whereas three mice subjected to a sham surgery were used as controls. The surgery-induced cryptorchid mice were sacrificed on 0.5 day, 1 day, 2 days, 3 days, 4 days, 5 days and 7 days after cryptorchid treatment (three mice for each time point), respectively. Testicular tissue was collected, and then fixed in Bouin’s solution (for histological analysis) or 2.5% glutaraldehyde (for ultrastructural analysis). A portion of testicular tissue was frozen in liquid nitrogen (for western blot analysis).
Histological analysis
Testicular tissue from control and surgery-induced cryptorchid mice was fixed in Bouin’s solution and embedded in paraffin. Testis sections were sliced at a thickness of 7 µm, deparaffinized and rehydrated. Testis sections were stained with haematoxylin and eosin (HE). After dehydration and embedding, sections were visualized under a Nikon i90 microscope (Nikon, Tokyo, Japan).
Transmission electron microscopy
For ultrastructural analysis, testicular tissue from control and surgery-induced cryptorchid mice was perfused with 2.5% glutaraldehyde. Later, testicular tissue was washed with phosphate-buffered saline (PBS), fixed in 1% OsO4 for 2 h at 4°C, and embedded in Araldite. Semi-thin sections were stained with toluidine blue and visualized under a light microscope. Ultrathin sections were stained with uranyl acetate and lead citrate, and visualized under a transmission electron microscope (JEM.1010, JEOL, Tokyo, Japan).
TUNEL assay
Terminal deoxynucleotidyl transferase dUTP nick end labelling (TUNEL) assay was performed using a colorimetric TUNEL apoptosis assay kit (Beyotime Institute of Biotechnology, Jiangsu, China), following the manufacturer’s instructions. In brief, testis sections were deparaffinized and rehydrated, and then incubated with 20 μg/ml proteinase K (Tiangen, Beijing, China) at room temperature (RT) for 15 min, followed by blocking with 3% H2O2 at RT for 10 min to deactivate endogenous peroxidase. The sections were incubated with the TUNEL reaction mix (2 μl terminal deoxynucleotidyl transferase and 48 μl biotin–dUTP) at 37°C for 1 h. The negative TUNEL control was prepared by omitting the enzyme. After washing, the sections were incubated with horseradish peroxidase-conjugated streptavidin solution at RT for 30 min, followed by staining with diaminobenzidine (DAB) and counterstaining with haematoxylin. The slides were later dehydrated and mounted, and visualized under a Nikon i90 microscope (Nikon, Tokyo, Japan).
Western blot
Testicular tissue was ground with liquid nitrogen in a porcelain mortar and lysed in RIPA buffer supplemented with PMSF (Beyotime, Jiangsu, China). Denatured protein samples were electrophoresed on a 12% sodium dodecyl sulfate (SDS) polyacrylamide gel, then transferred to a polyvinylidene fluoride (PVDF) membrane (Millipore, Billerica, USA). The membrane was blocked with 5% non-fat dried milk in Tris-buffered saline with 0.05% Tween-20 (TBST) for 2 h, and then incubated at 4°C overnight with the following primary antibodies: rabbit anti-Bcl-2 (Abcam, 1:1000), rabbit anti-Bax (Cell Signaling Technology, 1:1000), rabbit anti-caspase 8 (Cell Signaling Technology, 1:1000), rabbit anti-caspase 3 (Cell Signaling Technology, 1:1000), rabbit anti-PARP-1 (Cell Signaling Technology, 1:1000), rabbit anti-LC3B (Abcam, 1:1000) and mouse anti-β-actin (Comwin, 1:1000). After washing the next day, the membrane was incubated with horseradish peroxidase-conjugated anti-rabbit or anti-mouse secondary antibody (Abcam, Cambridge, UK, 1:5000) at RT for 2 h. The protein bands were visualized with a Bio-Rad Chemidoc XRS and a Western Bright ECL Kit (Comwin, Beijing, China).
Statistics
Statistical analyses were conducted using SPSS v17.0 software (SPSS, USA). Multiple comparisons were performed using one-way analysis of variance (ANOVA) followed by a least significant difference (LSD) test. Data were presented as the mean±standard error of the mean (SEM). A P-value <0.05 was considered to be statistically significant.
Results
Surgery-induced cryptorchidism leads to a reduced testicular weight
We first administered unilateral cryptorchid treatment on adult mice. In brief, the right testis was pulled into the abdominal cavity and sutured to the abdominal wall, while the left testis was maintained in the scrotum. The right testis of mice subjected to a sham surgery was used as a control. The testicular weight experienced a steady decrease from day 4 after cryptorchid treatment and reached the bottom on day 7 (Fig. 1).

Figure 1 Effects of surgery-induced cryptorchidism on the testicular weight. Data are presented as the mean±standard error of the mean (SEM), n=3. *P<0.05.
Surgery-induced cryptorchidism leads to aberrant seminiferous epithelial cycles and impaired spermatogenesis
Next, we performed histological analysis on testis sections from control and surgery-induced cryptorchid mice. Compared with the control, there was no significant morphological difference on day 0.5 after surgery-induced cryptorchid treatment. Nevertheless, aberrant seminiferous epithelial cycles and impaired spermatogenesis were observed later. On day 1, some pachytene spermatocytes at stages VII–VIII (Fig. 2e) and I–IV (Fig. 2g), as well as some dividing spermatocytes at stage XII (Fig. 2f) showed pyknotic nuclei. Round spermatids at stages VII–VIII (Fig. 2e) and I–IV (Fig. 2g) disappeared from the seminiferous epithelium. On day 3, some pachytene spermatocytes at stages V–VI and IX–X showed pyknotic nuclei. Some round spermatids at the stage VIII (Fig. 2i) displayed typical ring-like nuclei. At stages V–VI and IX–X, spermatids were absent in the seminiferous epithelium. On day 5, seminiferous tubules with rare or no round spermatids were discerned, and pachytene and onward spermatocytes disappeared from the seminiferous epithelium. On day 7, most spermatocytes degenerated and showed pyknotic (Fig. 2q) or even ring-like nuclei (Fig. 2r, t, left arrows). At this time point, it was difficult to recognize some seminiferous epithelial cycles. Moreover, we observed multinucleated giant cells (Fig 2r–t, right arrows) in almost all seminiferous tubules of the cryptorchid testis. These multinucleated giant cells contained aggregates of degenerating spermatocytes and round spermatids.
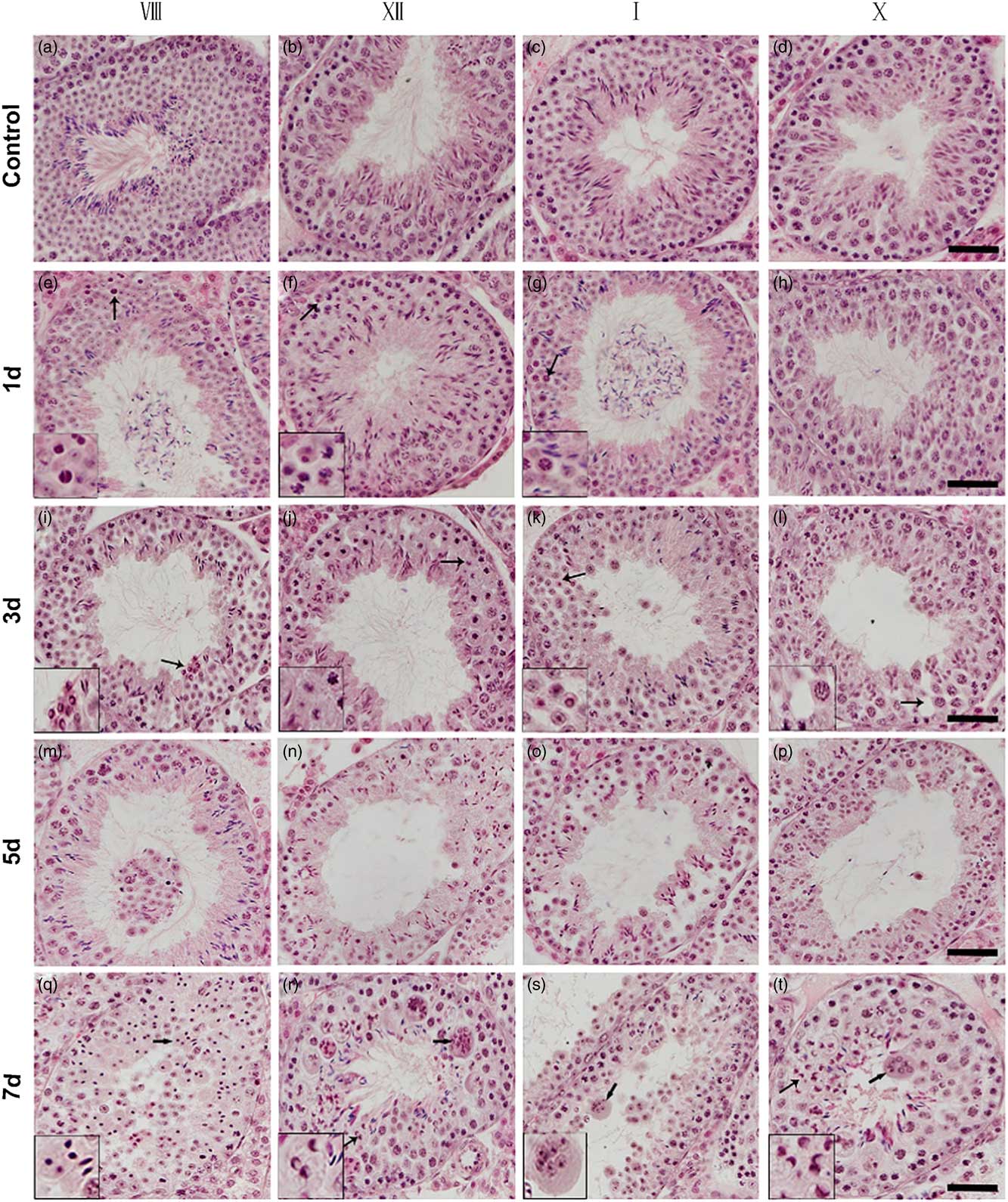
Figure 2 Histological analysis of testis sections from control and surgery-induced cryptorchid mice. (a–d) Representative images of testis sections from control mice showing normal spermatogenesis. (e–t) Representative images of testis sections on day 1 (e–h), day 3 (i–l), day 5 (m–p) and day 7 (q–t) after surgery-induced cryptorchid treatment. Insets are the magnification of indicated areas. Bar represents 100 µm.
Surgery-induced cryptorchidism induces apoptosis of spermatogenic cells
To demonstrate the occurrence of cell apoptosis in the cryptorchid testis, we first performed a transmission electron microscopy study. We observed that apoptosis was initiated on day 3 after cryptorchid treatment, characterized by the formation of pyknotic chromatin and concrete nucleoli (Fig. 3g–i, arrows). On day 7, concentrated organelles (Fig, 3j, arrow) were observed. Moreover, apoptotic bodies (Fig. 3k, arrows), which contain cellular components such as organelles and fragments of nuclear chromatin, became discernible, indicative of progressive cell apoptosis.
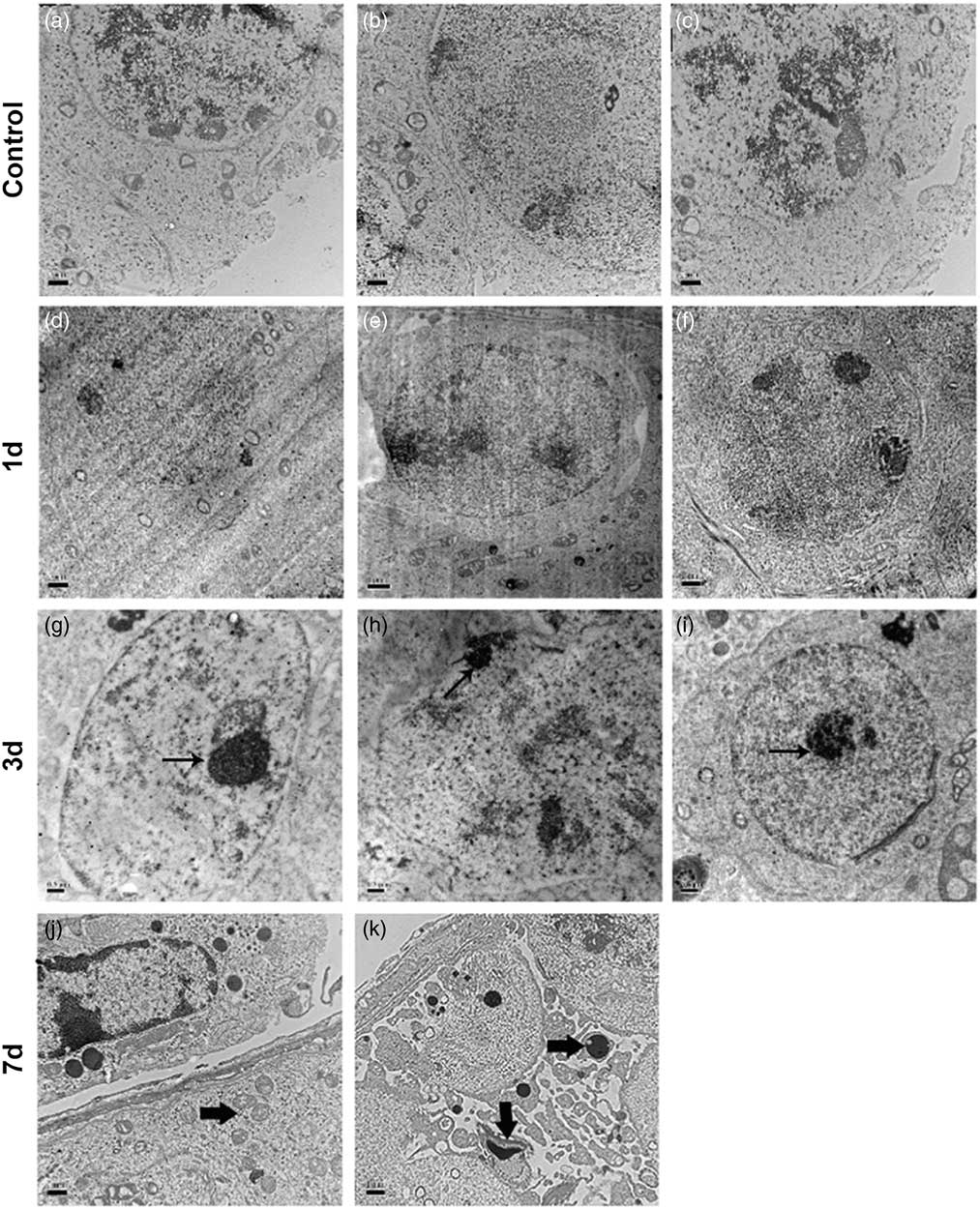
Figure 3 Representative transmission electron micrographs of testis sections from control and surgery-induced cryptorchid mice. (a–f) Representative micrographs of testis sections from control (a–c) and cryptorchid mice 1 day after treatment (d–f) showing normal cell morphology. (g–k) Representative micrographs of testis sections on day 3 (g–i) and day 7 (j, k) after surgery-induced cryptorchid treatment. Bar represents 1 µm.
Next, we performed a TUNEL assay on testis sections from control and surgery-induced cryptorchid mice. We observed that the majority of TUNEL+ apoptotic cells occurred at day 4 after treatment (Fig. 4g–i, arrows). TUNEL+ apoptotic cells mainly consisted of pachytene spermatocytes, a few round spermatids and rare spermatogonia. Some multinucleated giant cells were also positive for TUNEL signalling (Fig. 4i, arrow). To assess germ cell apoptosis in the cryptorchid testis, we quantified the percentage of seminiferous tubules harbouring TUNEL+ cells (Fig. 4j), the average number of total TUNEL+ cells (Fig. 4k) and TUNEL+ multinucleated giant cells (Fig. 4l) per seminiferous tubule, as well as the percentage of TUNEL+ multinucleated giant cells in all TUNEL+ cells (Fig. 4m). Compared with the control, the percentage of seminiferous tubules harbouring TUNEL+ cells (Fig. 4j) and the number of total TUNEL+ cells (Fig. 4k) gradually increased from day 4 after cryptorchid treatment and reached the peak on day 7. Surgery-induced cryptorchidism also facilitated the formation of TUNEL+ multinucleated giant cells (Fig. 4l, m).

Figure 4 TUNEL assay of testis sections from control and surgery-induced cryptorchid mice. (a) Negative TUNEL control. (b) TUNEL assay of testis sections from control mice. (c–i) TUNEL assay of testis sections on day 0.5 (c), day 1 (d), day 2 (e), day 3 (f), day 4 (g), day 5 (h) and day 7 (i) after surgery-induced cryptorchid treatment. Insets are the magnification of indicated areas. Bar represents 100 µm. (j) Percentages of seminiferous tubules harbouring TUNEL+ cells. (k) Average number of total TUNEL+ cells per seminiferous tubule. (l) Average number of TUNEL+ multinucleated giant cells per seminiferous tubule. (m) Percentages of TUNEL+ multinucleated giant cells in all TUNEL+ cells. Data are presented as the mean±standard error of the mean (SEM), n=3. *P<0.05. In each independent experiment, overall 100 seminiferous tubules were examined.
Next, we performed a western blot assay to detect the expression of apoptosis-related proteins in the control and cryptorchid mouse testis. Bcl-2 (the anti-apoptosis protein) and Bax (the pro-apoptosis protein) are two important members of the Bcl-2 family regulating the intrinsic mitochondrial apoptotic pathway (Kaur and Bansal, Reference Kaur and Bansal2015). Consistently, the expression of Bcl-2 gradually deceased (Fig. 5a,b), whereas that of Bax showed an upward trend after day 1 after surgery-induced cryptorchid treatment (Fig. 5a,c). Previous reports have shown that caspase-8 and caspase-3 are cleaved and activated in the extrinsic death-receptor apoptotic pathway (Fan et al., Reference Fan, Huang, Boucher, Shang, Zuo, Brinks, Lau, Zhang, Chuprun and Gao2013). In our present study, with time, surgery-induced cryptorchidism caused the increasing cleaved caspase-8 (Fig. 5a, d), caspase-3 (Fig. 5a, e) as well as PARP-1 (Fig. 5a, f). These overall results suggest that both intrinsic and extrinsic pathways are involved in the germ cell apoptosis of cryptorchid mice.

Figure 5 Western blot analysis of apoptosis-related protein levels in the control and surgery-induced cryptorchid mouse testis. (a) Representative images of immunoblotting. β-actin is used as a loading control. The band intensity of Bcl-2 (b), Bax (c), cleaved caspase-8 (d), cleaved caspase-3 (e) and cleaved PARP-1 (f) is divided by that of β-actin, respectively. Data are presented as the mean±standard error of the mean (SEM), n=3. *P <0.05.
Surgery-induced cryptorchidism induces autophagy of spermatogenic cells
Finally, we investigated whether autophagy occurs in germ cells of the cryptorchid mouse testis. Autophagy can be characterized by the formation of double-membrane structures called autophagosome (Kliosnky et al., Reference Kliosnky2016). To this end, we performed an additional transmission electron microscopy study, and observed typical autophagosome in germ cells from day 3 after cryptorchid treatment (Fig. 6a–f).
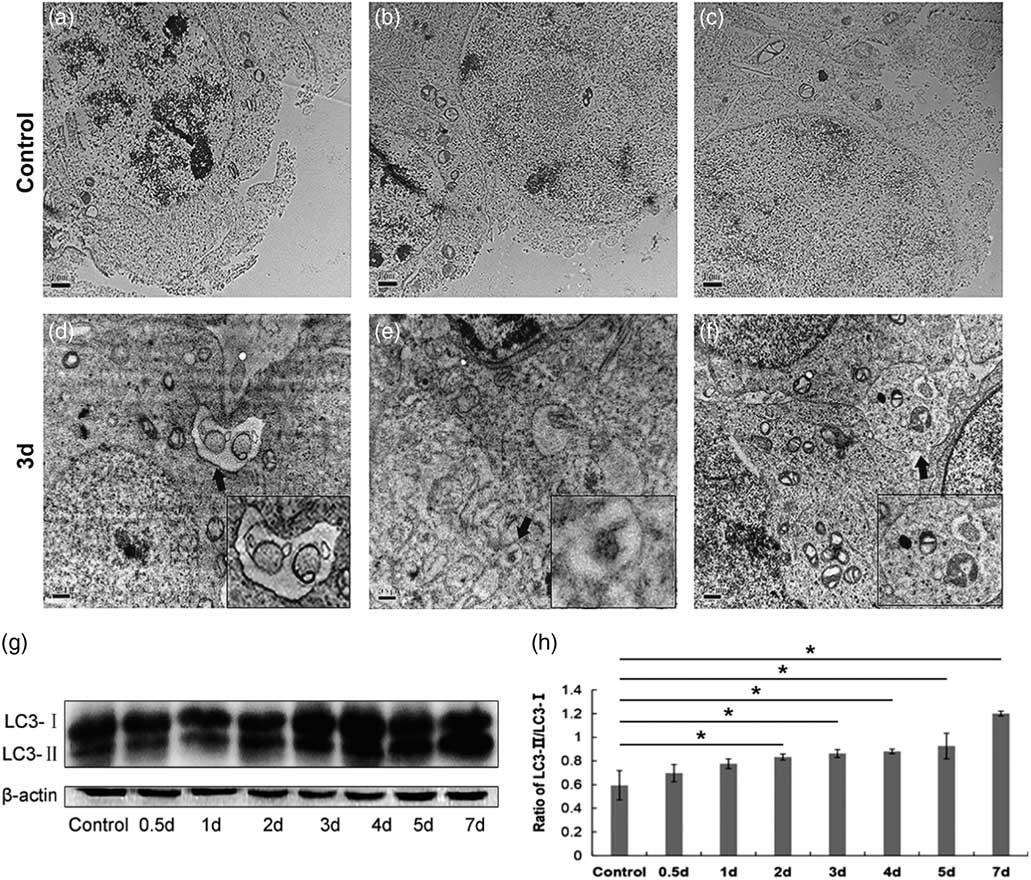
Figure 6 Surgery-induced cryptorchidism induces autophagy of spermatogenic cells. (a–f) Representative transmission electron micrographs of testis sections from control (a–c) and cryptorchid mice on day 3 after cryptorchid treatment (d–f). Arrows show the typical autophagosome. Insets are the magnification of indicated areas. Bar represents 1 µm. (g) Western blot analysis of LC3-I and LC3-II levels in the control and surgery-induced cryptorchid mouse testis. β-Actin is used as a loading control. (h) The ratio of the band intensity of LC3-II/LC3-I. Data are presented as the mean±standard error of the mean (SEM), n=3. *P<0.05.
LC3 is a mammalian homologue of the yeast Apg8p protein. There are two forms of LC3 (LC3-I and LC3-II), and the conversion of LC3-I to LC3-II serves as a marker for autophagy (Kabeya et al., Reference Kabeya, Mizushima, Ueno, Yamamoto, Kirisako, Noda, Kominami, Ohsumi and Yoshimori2000). Therefore we performed a western blot assay to detect the expression of LC3-I and LC3-II in the control and cryptorchid mouse testis. Indeed, the ratio of LC3-II/LC3-I increased with time and reached a peak on day 7 after cryptorchid treatment (Fig. 6g, h). Collectively, the results indicated that surgery-induced cryptorchidism induces autophagy of spermatogenic cells.
Discussion
Cryptorchidism, characterized by the presence of one (unilateral) or both (bilateral) undescended testes, is a common male urogenital defect. Cryptorchidism can lead to male infertility (Lee et al., Reference Lee, Bellinger, Songer, O’Leary, Fishbough and LaPorte1993), testicular cancer being the most extreme clinical symptom (Pettersson et al., Reference Pettersson, Richiardi, Nordenskjold, Kaijser and Akre2007), as well as psychological issues of the inflicted individual (Xi et al., Reference Xi, Cheng, Wan and Hua2015). Despite this, knowledge about the aetiology of cryptorchidism and the mechanism for cryptorchidism-induced male infertility remain limited. Here, we took advantage of an artificial cryptorchid mouse model to systematically investigate the effect of surgery-induced cryptorchidism on spermatogenic cells and seminiferous epithelial cycles. We found that surgery-induced cryptorchidism led to a reduced testicular weight, aberrant seminiferous epithelial cycles and impaired spermatogenesis characterized by degenerating spermatogenic cells. Moreover, we identified that both apoptosis and autophagy were involved in germ cell death of the cryptorchid testis. Overall, our results provide novel insights into the cryptorchidism-induced male infertility, thereby contributing to the development of male contraceptive strategies and, more importantly, treatment options for male infertility caused by cryptorchidism.
Spermatogenesis is a highly efficient, intricate and precise process in testes by which lifelong male fertility is maintained. Crucial to this process is the appropriate temperature in the scrotum, which is usually 2–8°C lower than that in the abdominal cavity (Ivell, Reference Ivell2007). Indeed, higher temperatures often leads to disrupted spermatogenesis (Schulz and Miura, Reference Schulz and Miura2002). In our study, testicular weight experienced a sharp decrease from day 4 after surgery-induced cryptorchid treatment, in accordance with a previous report (Yin et al., Reference Yin, Hawkins, DeWolf and Morgentaler1997) and a study that involved intra-scrotal heat stress in mice (Lin et al., Reference Lin, Shin, Park, Chu, Gwon, Lee, Yon, Baek and Nam2015). The underlying reason for the reduced testicular weight is likely to be the death and later large-scale elimination of spermatogenic cells.
Raised temperature by surgical induction of cryptorchidism can impair spermatogenesis, devastate spermatogenic cells and disrupt seminiferous epithelial cycles (Clegg, Reference Clegg1963). In our present study, we observed the initiation of morphological abnormality in pachytene spermatocytes at stages VII–VIII and I–IV, and in dividing spermatocytes at stage XII on day 1 after cryptorchid treatment, in line with a previous report (Henriksen et al., Reference Henriksen, Hakovirta and Parvinen1995). Chowdhury and Steinberger (Reference Chowdhury and Steinberger1970) reported the initiation of morphological abnormality in pachytene spermatocytes at stages X–XII, in dividing spermatocytes at stages XIII–XIV and in round spermatids at the stage I, and that the morphological alterations could be observed in as early as 1 hour following exposure of rat testes to 43°C for 15 min. The inconsistent outcome might be ascribed to distinct treatment (surgery-induced cryptorchidism versus intra-scrotal heat stress) and species (mice versus rats).
We observed multinucleated giant cells in seminiferous tubules on day 7 after cryptorchid treatment. Multiple reports have demonstrated the emergence of multinucleated giant cells in cryptorchid testes (Clegg, Reference Clegg1963; Kerr et al., Reference Kerr, Rich and de Kretser1979; Yin et al., Reference Yin, DeWolf and Morgentaler1998; Kumar et al., Reference Kumar, Misro and Datta2012). Multinucleated giant cells are therefore regarded as a marker for the cryptorchid seminiferous epithelium. Nantel and co-workers (Nantel et al., Reference Nantel, Monaco, Foulkes, Masquilier, LeMeur, Henriksen, Dierich, Parvinen and Sassone-Corsi1996) reported that multinucleated giant cells were associated with cell apoptosis. Indeed, the formation of multinucleated giant cells can be perceived as a response to cryptorchidism, and it is these cells that further expedite the process of germ cell removal. A decline in intra-testicular testosterone may act as a catalyst for the formation of these cells (Shikone et al., Reference Shikone, Billig and Hsueh1994; Barqawi et al., Reference Barqawi, Trummer and Meacham2004).
In normal rat testes, apoptosis is mainly observed in spermatogonia (Allan et al., Reference Allan, Harmon and Roberts1992). Nonetheless, previous studies have shown that in mice, primary spermatocytes and round spermatids are most vulnerable to heat stress and prone to undergo apoptosis (Lue et al., Reference Lue, Hikim, Swerdloff, Im, Taing, Bui, Leung and Wang1999; Yin et al., Reference Yin, Hawkins, DeWolf and Morgentaler1997; Zhang et al., Reference Zhang, Jiang, Bi, Zhu, Zhou and Sha2012). In our present study, we found that TUNEL+ apoptotic cells were mainly comprised of pachytene spermatocytes, a few round spermatids and rare spermatogonia, indicating that, in surgery-induced cryptorchid mouse testes, pachytene spermatocytes are the predominant cell type undergoing apoptosis. Notably, not all multinucleated giant cells were positive for the TUNEL signalling, suggesting that non-apoptotic multinucleated giant cells may also contribute to germ cell removal in the cryptorchid testis. Apoptotic multinucleated giant cells seem to be unique to testes, and the adjacent Sertoli cells may play a role in the formation of these multinucleated giant cells (Yin et al., Reference Yin, Hawkins, DeWolf and Morgentaler1997; Chaki et al., Reference Chaki, Misro, Ghosh, Gautam and Srinivas2005).
Apoptosis mostly depends on caspase, and caspase-dependent apoptosis is mainly triggered by the intrinsic mitochondrial pathway and the extrinsic death-receptor pathway. The intrinsic pathway is mainly regulated by the Bcl-2 protein family (Kaur and Bansal, Reference Kaur and Bansal2015). Bcl-2, as well as its structural homologue, the long form of Bcl-x (Bcl-xL), facilitate cell survival by inhibiting apoptosis (Boise et al., Reference Boise, Gonzalez-Garcia, Postema, Ding, Lindsten, Turka, Mao, Nunez and Thompson1993; Absalan et al., Reference Absalan, Movahedin and Mowla2010), while other members of the Bcl-2 protein family, such as Bax, Bak and Bad, promote cell apoptosis (Oltvai et al., Reference Oltvai, Milliman and Korsmeyer1993; Yang et al., Reference Yang, Zha, Jockel, Boise, Thompson and Korsmeyer1995; Kheradmand et al., Reference Kheradmand, Dezfoulian and Tarrahi2011). In the extrinsic pathway, caspase-8 and caspase-3 are successively cleaved and activated. Yet, regardless of the intrinsic (which involves activation of caspase-9) or extrinsic pathway (which involves activation of caspase-8), the cleavage of the downstream executioner caspase-3 ensues, eventually resulting in apoptosis (Hikim et al., Reference Hikim, Lue, Yamamoto, Vera, Rodriguez, Yen, Soeng, Wang and Swerdloff2003). In our present study, the expression of Bcl-2 gradually deceased, whereas that of Bax, cleaved caspase-8, caspase-3 as well as PARP-1 showed an upward trend from day 1 after surgery-induced cryptorchid treatment, suggesting that both intrinsic and extrinsic pathways are involved in germ cell apoptosis of cryptorchid mice. In addition, by transmission electron microscopy, we observed that apoptosis was initiated on day 3 after cryptorchid treatment, characterized by the formation of pyknotic chromatin and concrete nucleoli. These results provided new insights for studies on germ cell apoptosis in the cryptorchid testis.
Autophagy can consistently occur in all cells at a basal level and increase under various stress conditions such as the presence of intracellular pathogens or inflammation (Deretic, Reference Deretic2006; Chen and Klionsky, Reference Chen and Klionsky2011), oxidative stress (Coto-Montes et al., Reference Coto-Montes, Boga, Rosales-Corral, Fuentes-Broto, Tan and Reiter2012), growth factor withdrawn (Song et al., Reference Song, Yoon, Kim, Sim, Kim, Cha, Choi, Min, Lee, Huh, Lee, Kim, Koo, Choo, Kim, Kim and Chang2012) or heat stress (Zhang et al., Reference Zhang, Jiang, Bi, Zhu, Zhou and Sha2012). Here we suggest that, like heat stress, cryptorchidism can induce autophagy of spermatogenic cells. Interestingly, by transmission electron microscopy, we observed typical autophagosome in germ cells from day 3 after cryptorchid treatment, when apoptosis was also initiated. Both apoptosis and autophagy fall within the scope of PCD. Nonetheless, their crosstalk remains elusive in most cell types under variable conditions. First, autophagy can occur prior to apoptosis and then promote apoptosis. Second, autophagy and apoptosis can antagonize each other thereby promoting cell survival/death. Third, autophagy and apoptosis can interconvert to each other under certain conditions, eventually safeguarding cell death (Gozuacik and Kimchi, Reference Gozuacik and Kimchi2007; Maiuri et al., Reference Maiuri, Zalckvar, Kimchi and Kroemer2007; Eisenberg-Lerner et al., Reference Eisenberg-Lerner, Bialik, Simon and Kimchi2009; Gump and Thorburn, Reference Gump and Thorburn2011). Our study showed the synchronous and progressive apoptosis and autophagy after cryptorchid treatment, suggestive of their synergetic roles in promoting germ cell death. Future studies on mechanisms regulating apoptosis and autophagy of germ cells in the cryptorchid testis may provide insight for resolving male fertility issues, e.g. sterility and failure to concieve.
Financial support
This study was supported in part by National Key R&D Programme of China (2018YFD0501000), the National Natural Science Foundation of China (Grant No. 31572401) to WZ; Young Talent fund of University Association for Science and Technology in Shaanxi, China (Grant No. 20180204), China Postdoctoral Science Foundation (Grant No. 2018M641032) and a startup fund from Northwest A&F University (Grant No. Z109021803) to YZ.
Conflicts of interest
The authors declare no conflicts of interest.
Ethical standards
All experimental procedures involving mice were approved by the Institutional Animal Care and Use Committee of the Northwest A&F University.