Introduction
The technique of somatic cell nuclear transfer (SCNT) involves the removal of the nuclear DNA of a metaphase II stage oocyte (enucleation), the insertion of a single cell into the perivitelline space of the enucleated oocyte (donor cell injection), followed by electrofusion, chemical activation and in vitro culture of embryos. Somatic cell NT has been successfully applied in Bos taurus and Bos indicus cattle using homologous oocytes, resulting in the establishment of pregnancies and live offspring (Cibelli et al., Reference Cibelli, Stice, Golueke, Kane, Jerry, Blackwell, Leon and Robl1998; Shiga et al., Reference Shiga, Fujita, Hirose, Sasae and Nagai1999; Wells et al., Reference Wells, Misica and Tervit1999; Hill et al., Reference Hill, Winger, Long, Looney, Thompson and Westhusin2000; Enright et al., Reference Enright, Taneja, Schreiber, Riesen, Tian, Fortune and Yang2002; Savage et al., Reference Savage, Maull, Tian, Taneja, Jatz, Darre. and Yang2003). However, the current low efficiencies of the SCNT procedure, in addition to the high perinatal mortality, require that numerous attempts are needed to produce live offspring (Wells et al., Reference Wells, Misica and Tervit1999; Colman et al., Reference Colman2000). In exotic or endangered species, the limited availability of both oocytes and recipients precludes the use of traditional SCNT, and an approach such as interspecies SCNT (iSCNT) may be the only alternative to produce embryos and offspring. In addition, the iSCNT model could have applications in the production of homologous blastocysts for the isolation and establishment of embryonic stem cell lines (Cibelli et al., Reference Cibelli, Kiessling, Cunniff, Richards, Lanza and West2001).
One of the early attempts of iSCNT using the enucleated bovine oocyte as recipient cytoplast was reported by Dominko et al. (Reference Dominko, Mitalipova, Haley, Beyhan, Memili, McKusick and First1999). In this study, karyoplasts from monkey, sheep, pig and rat somatic cells were injected into bovine cytoplasts, resulting in various degrees of early in vitro development. Although no pregnancies were reported, this study demonstrated that the bovine oocyte is capable of supporting mitotic divisions after the introduction of a differentiated nucleus. Further iSCNT attempts using the bovine cytoplast and the karyoplasts from other species include somatic cells from pigs (Yoon et al., Reference Yoon, Choi, Han, Shim and Roh2001), saolas (Bui et al., Reference Bui, Vignon, Campion, Laloy, Lavergne, Ty, Nguyen and Renard2002), horses (Li et al., Reference Li, Siedel and Squires2002; Sansinena et al., Reference Sansinena, Reggio, Denniston and Godke2002; Tecirlioglu et al., Reference Tecirlioglu, Guo and Trounson2006), bears (Ty et al., Reference Ty, Hanh, Uoc, Duc, Thanh, Bui, Huu and Nguyen2003), humans (Cibelli et al., Reference Cibelli, Kiessling, Cunniff, Richards, Lanza and West2001), desert Big Horn sheep (Williams et al., Reference Williams, Shin, Liu, Flores-Foxworth, Romano, Blue-McClendon, Kraemer and Westhusin2006), yaks (Li et al., Reference Li, Dai, Du, Zhao, Wang, Wang, Liu, Li and Li2007), Siberian tiger (Song et al., Reference Song, Hua, Song and Zhang2007), elands (Damiani et al., Reference Damiani, Wirtu, Miller, Cole, Pope, Godke and Dresser2003; Nel-Themaat et al., Reference Nel-Themaat, Gómez, Pope, Lopez, Wirtu, Jenkins, Cole, Dresser, Bondioli and Godke2008), red panda (Tao et al., Reference Tao, Liu, Zhang, Zhang, Fang, Han, Zhang, Liu, Ding and Zhang2009) and Sei whale (Lee et al., Reference Lee, Bhuiyan, Watanabe, Matsuoka, Fujise, Ishikawa and Fukui2009). The first iSCNT live offspring was reported for the gaur by Lanza et al. (Reference Lanza, Cibelli, Diaz, Moraes, Farin, Farin, Hammer, West and Damiani2000) but died within the first 48 h. Using domestic sheep (Ovis aries) as recipient cytoplasts, two pregnancies were reported after iSCNT using an exotic argali (Ovis ammon) as donor karyoplasts, but both of these pregnancies were lost by 59 days of gestation (White et al., Reference White, Bunch, Mitalipov and Reed1999). Subsequently, domestic sheep (Ovis aries) oocytes used as recipients for mouflon (Ovis orientalis musimon) cells resulted in one live offspring (Loi et al., Reference Loi, Ptak, Barboni, Fulka, Cappai and Clinton2001). In addition, our laboratory reported banteng (Bos javanicus) and Riverine rabbit (Sansinena et al., Reference Sansinena, Owiny, Denniston, Salamone and Barry2007) pregnancies using iSCNT.
An in vitro developmental block has been described by several authors in iSCNT when the species of donor karyoplasts and recipient cytoplasts are far apart in their taxonomic classification. An 8- to 16-cell stage in vitro developmental block has been reported using iSCNT in the pig (Yoon et al., Reference Yoon, Choi, Han, Shim and Roh2001), horse (Li et al., Reference Li, Siedel and Squires2002; Sansinena et al., Reference Sansinena, Reggio, Denniston and Godke2002), llama (Sansinena et al., Reference Sansinena, Taylor, Taylor, Denniston and Godke2003a,Reference Sansinena, Taylor, Taylor, Denniston and Godkeb), whale (Bhuiyan et al., 2009) and monkey (Song et al., Reference Song, Lee, Kim, Kim, Park, Kim, Han, Lee, Lee and Koo2009) when using bovine recipient cytoplasts. Although progression through the early stages of embryogenesis is regulated by maternally inherited gene products derived from the cytoplasm, the embryonic development beyond the early stages becomes dependent on embryonic gene activation at a species-specific developmental stage (Telford et al., Reference Telford, Watson and Schultz1990). It has been suggested that although the bovine cytoplast seems capable of supporting a few mitotic divisions, heteroplasmy or mitochondrial incompatibilities may affect nuclear-ooplasm events occurring at the time of genomic activation (Sansinena et al., Reference Sansinena, Reggio, Denniston and Godke2002).
Ooplasm donation, a technique developed in the human infertility field for improving embryo development in cases of persistent developmental failure and to address the problem of age-related infertility in women (Cohen et al., Reference Cohen, Scott, Schimmel, Levron and Willadsen1997, Reference Cohen, Scott, Alikani, Schimmel, Munné, Levron, Wu, Brenner, Warner and Willadsen1998; Ferreira et al., Reference Ferreira, Burgstaller, Perecin, Garcia, Chiaratti, Méo, Muller, Smith, Meirelles and Steinborn2010) involves the injection of a small volume of the ooplasm from a healthy donor oocyte into a recipient oocyte prior to fertilization by intracytoplasmic sperm injection (ICSI). The mechanisms by which ooplasm transplantation can restore growth and viability of developmentally compromised embryos still remain unclear. Cellular components that might benefit development include the pool of stored mRNAs and proteins that might regulate oocyte function and embryo development, as well as mitochondria and other organelles.
To date, there has been no report of ooplasm transfer (OT) used in combination with iSCNT in an attempt to overcome the previously observed in vitro block. To our knowledge, no report of heteroplasmy after ooplasm transfer or patterns of migration of foreign mitochondria is available in animal studies. Therefore, the objectives of this study were: (1) to evaluate the combination of the OT procedure with SCNT in the cow model; (2) to determine the presence and pattern of migration of foreign mitochondria after OT procedure; and (3) to evaluate the effect of the transfer of caprine ooplasm into bovine oocytes used as recipients for iSCNT in the goat.
Materials and methods
Preparation of bovine and caprine donor cells
Adult fibroblasts were isolated from 6-mm skin biopsies taken from a mature, cycling, mixed breed beef cow (body condition score = 7; 1 = thin to 9 =obese) and a mature, cycling, Spanish-type crossbred doe (body condition score = 6; 1 = thin to 9 = obese). Primary cultures were obtained by mincing dermal tissue using sterile curved scissors in a 35 × 10-mm Falcon™ Petri dish (Becton Dickinson). The culture medium used consisted of TCM-199 (Gibco) with 10% fetal bovine serum (FBS, HyClone) and 0.1% gentamicin (Gibco). Tissue samples were washed by swirling the tissue suspension in a 15-ml centrifuge tube and allowing the tissue to settle in the bottom. Supernatant was discarded and then 3 ml of fresh culture medium was added to the tube. This process was repeated three times, after which tissue was transferred to a 35-mm Petri dish. All but 500 to 700 μ1 of excess medium was aspirated to allow oxygen tension over the tissue. All cultures were conducted at 38.5 °C and 5% CO2 in humidified atmosphere.
Once a fibroblast cell layer was established (7 to 10 days from initiation of culture), cells were grown in 25-cm2 tissue culture flasks (Costar™) until they reached 95% confluency. Cells were then trypsinized (Sigma), counted using a Neubaur hemocytometer and re-seeded at a density of 100,000 cells in 25-cm2 tissue culture flasks. After two subpassages, cultured cells were exposed to a cryoprotectant solution consisting of TCM-199, 20% calf serum, 5% dimethylsulfoxide (DMSO) and frozen in aliquots of 500,000 cells/ml of cryoprotectant in CryoTubes™ (Nunc, Fisher Scientific). Frozen/thawed adult cow fibroblasts were used as donor cells in the passage three of culture. One week prior to NT procedure cells were thawed, allowed 2 days of proliferation in serum-rich medium (TCM-199 containing 10% FBS and 0.1% gentamicin in 25-cm2 tissue culture flasks), then serum starved 3–5 days to increase the proportion of cells in G0/G1 stage (Wilmut et al., Reference Wilmut and Campbell1998).
Experiment 1: combination of NT and OT procedures in the bovine model
Oocyte preparation
Abattoir-derived bovine oocytes were harvested by follicular puncture (Ovagenix), matured overnight while being shipped by air courier. At 18 to 22 h of maturation, cumulus–oocyte complexes were vortexed for 2 min in TCM-199 containing 1.2 mg/ml of hyaluronidase (Sigma) to remove cumulus investments. Mature, metaphase II oocytes were selected based on the visualization of the first polar body and then randomly assigned to treatment groups. Treatment groups consisted of OT followed by SCNT in the cow (OT-SCNT, treatment A), SCNT followed by OT in the cow (SCNT-OT, treatment B) and parthenogenetically activated cow oocytes served as laboratory control (treatment C).
Ooplasm transfer procedure
Oocytes were manipulated in modified TL-HEPES (mTLH; TL-HEPES supplemented with 10% FBS and 0.1% gentamicin) in two elongated, parallel 120-μl droplets overlaid with warm, embryo-tested mineral oil, one containing the oocytes to be used as ooplasm donors and one containing the oocytes to be used as ooplasm recipients. Micromanipulations were conducted on the heated stage (38 °C) of an inverted microscope (Nikon Diaphot) equipped with Hoffman Modulation Contrast objectives, Narishige micromanipulators and epifluorescent illumination. A 120-μm diameter (o.d.) borosilicate pipette was used to hold the oocytes by negative pressure during the procedure. Ooplasm from the donor oocyte was aspirated into a 7 μm (o.d.) glass pipette until the oolemma was ruptured. Ooplasm was taken from the pole opposite to the polar body to avoid the metaphase spindle of the oocyte and the ooplasm was aspirated until it filled about 200 μm of the micropipette. Ooplasm was then carefully injected into the recipient oocyte. The oolemma of the recipient oocyte was also ruptured by partial aspiration into the micropipette, and ooplasm of the donor and/or the recipient were deposited into the oocyte pole opposite to the metaphase spindle.
Somatic cell nuclear transfer procedure
Nuclear DNA was labelled by passive diffusion with 3 μg/ml of Hoechst 33342 (Sigma) for 3 min. Oocytes were then washed in mTL-HEPES and placed in groups of 25 oocytes in an elongated 150-μl micromanipulation droplet containing 6 μg/ml of cytochalasin D (Sigma) overlaid with warm, embryo-tested mineral oil. All micromanipulations were conducted on the stage of an inverted microscope (Nikon Diaphot) equipped with Hoffman Modulation Contrast objectives, Narishige micromanipulators, Uniblitz VMM-D1 shutter system (Vincent Associates) and epifluorescent illumination. A 120-μm diameter (o.d.) pipette was used to hold the oocytes by negative pressure. After a brief exposure (<5 s) to ultraviolet light to visualize nuclear DNA, the metaphase spindle and polar body with a small amount of surrounding ooplasm were removed by negative pressure applied to an enucleation pipette (20 μm o.d., 35° bevel, spiked). Once enucleated, the cytoplasts were held in modified TCM (mTCM; TCM-199 supplemented with 10% FBS and 0.1% gentamicin) at 38.5 °C and 5% CO2 in humidified air.
Cow adult fibroblasts were used as nuclear donor cells at passage three of culture. Donor cells were serum starved (0.5% FBS) for 3 to 5 days prior to NT procedures. Donor cell injection was performed in groups of 25 cytoplasts in a 200-μl elongated droplet of mTLH containing 6 μg/ml of cytochalasin D overlaid with warmed, embryo-tested mineral oil. A single adult cow fibroblast (15 to 20 μm in diameter) was inserted into the perivitelline space of an enucleated oocyte. Fusion of karyoplast-cytoplast couplets was performed in a microslide fusion chamber with stainless steel electrodes separated by a 1-mm gap and the chamber was filled with fusion buffer (0.3 M mannitol, 0.1 mM MgSO4.7H2O, 0.05 mM CaCl2, 0.5 mM TL-HEPES and 4 mg/ml of BSA (Fraction V, Sigma). The couplets were then fused by two consecutive DC pulses (1.9 kV/cm for 25 μs) delivered by a BTX Electrocell Manipulator 200 (Gentronics).
Couplets were evaluated for fusion by microscopic examination within a 1-h period. At that time, ova randomly allocated to parthenogenetic activation treatment group (treatment C) were placed in the microslide fusion chamber and pulsed once (1.9 kV/cm for 25 μs) to initiate activation. Chemical activation of all treatment groups was performed by a 4-min exposure to 5 μM of ionomycin (Sigma) followed by 5 min in TL-HEPES with 30 mg/ml of BSA (Fraction V, Sigma) and 2 h incubation in 10 μg/ml of cycloheximide (Sigma) with 6 μg/ml of cytochalasin D prepared in CR1aa medium (Rosenkrans et al., Reference Rosenkrans, Zeng, McNamara, Schoff and First1993).
Embryos were cultured in 35-μ1 droplets of CR1aa medium in a modular incubator chamber (Billups-Rothenberg) at 38.5 °C and 5% O2, 5% CO2 and 90% N2 humidified atmosphere. Embryos were examined for cleavage after 3 days of in vitro culture, the culture medium was then replaced with CR1aa with 5% FBS and blastocyst production was recorded at days 7 and d 9 (fusion = day 0) of in vitro culture.
Statistical analysis
Mean cleavage rates and embryo development rates were compared across treatment groups by chi-squared analysis (SAS Institute, 1985). In this study, mean values between treatment groups were considered statistically significant at the p < 0.05 level.
Experiment 2: staining of foreign mitochondria introduced by the OT procedure
Bovine oocytes to be used as ooplasm donors were stained with 200 nM of MitoTracker® Green FM (M-7514, Molecular Probes, Inc.) for 45 min. Stained oocytes were washed for 30 min in modified TCM-199 (mTCM; TCM-199 with 10% fetal bovine serum and 0.1% gentamicin) and were then placed in micromanipulation droplets of mTLH for ooplasm transfer. Bovine ooplasm transfer and SCNT procedures were conducted as described in Experiment 1. Couplets were cultured in 35-μ1 droplets of CR1aa medium in a modular incubator chamber (Billups-Rothenberg) at 38.5 °C and 5% O2, 5% CO2 and 90% N2 humidified atmosphere and imaged for presence and distribution of labelled mitochondria at 12 h post-fusion (precleavage), days 3 and days 7 of in vitro culture. Fluorescence was assessed using a Leica TCS SP2 laser scanning confocal microscope using a ×20 objective at ex = 490 nm and em > 516 nm.
Experiment 3: iOT (goat ooplasm injected into bovine oocytes) followed by iSCNT (goat somatic cell injected into iOT-derived bovine oocyte)
Goat oocyte collections
Mature does were implanted subcutaneously (day 1) on the dorsal side of the ear with a 3-mg norgestomet implant (Synchro-Mate B, Rhone Merieux) and then subjected (day 6) to ovarian superstimulation by twice daily administration of pFSH (Sioux Biochemical) (i.m.) in decreasing doses (10, 7.5, 5, 2.5 mg), with a total dose of 50 units/female. Oocytes were surgically aspirated from does 24 h after final pFSH injection, with the norgestomet implant removed just prior to surgery. All doe treatment and management procedures were approved by the Institutional Animal Care and Use Committee of the Louisiana State University Agricultural Center, USA.
Cumulus–oocyte complexes (COC) were harvested as previously described (Reggio et al. Reference Reggio, James, Green, Gavin, Behboodi, Echelard and Godke2001) and placed in groups of 10 to 15 oocytes in 35 μl droplets of maturation medium overlaid with warmed, embryo-tested mineral oil. The oocyte maturation medium (TCM) consisted of TCM-199 supplemented with 10% goat serum (Sigma), 10 μg/ml of LH, 5 μg/ml of FSH and 1 μg/ml of estradiol-17β and the COC were allowed to mature for 18 to 22 h. Cumulus–oocyte complexes were vortexed for 1.5 min in TCM containing 0.6 mg/ml of hyaluronidase (Sigma) to aid in the removal of the cumulus investments and then metaphase II oocytes were selected based on the visualization of a first polar body and maintained in mTCM at 38.5 °C and 5% CO2 in humidified air until their use as ooplasm donors.
Bovine oocyte preparation
Abattoir-derived bovine oocytes were prepared as described in Experiment 1. Metaphase II bovine oocytes were selected and randomly assigned to treatment groups. Treatment groups consisted of transfer of goat ooplasm (iOT) into bovine oocytes followed by iSCNT using bovine oocytes as recipient cytoplast and goat adult fibroblasts as karyoplast donors (iOT-iSCNT, treatment A), iSCNT only (iSCNT, treatment B) and parthenogenetically activated bovine (treatment C) and caprine oocytes (treatment D) served as laboratory controls.
Transfer of goat ooplasm into bovine oocytes was conducted as described in Experiment 1. Nuclear transfer was performed, as described in Experiment 1, but in this case goat adult fibroblasts were used as nuclear donors at passage three of culture (Fig. 1). In vitro culture of reconstructed embryos was conducted as described in Experiment 1.
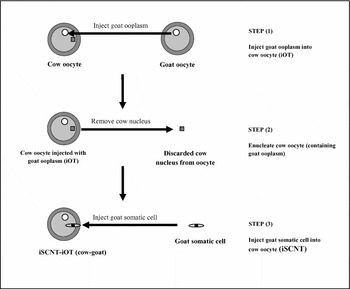
Figure 1 Procedures of iOT and iSCNT conducted for Experiment 3.
Results
Experiment 1: combination of NT and OT procedures in the bovine model
Developmental rates for bovine embryos and parthenogenetically activated bovine oocyte controls are summarized in Table 1. Of a total of 48 reconstructed couplets in treatment A (OT–SCNT), 65% fused following two electrical pulses. Of fused couplets, 87% of the embryos cleaved, 48% reached the blastocyst stage in 168 h and 32% hatched after 216 h of in vitro culture. Of a total of 45 reconstructed couplets in treatment B (SCNT–OT), 73% fused after two electrical pulses. Of fused couplets, 85% of the embryos cleaved, 39% reached blastocyst stage in 168 h and 27% hatched after 216 h of in vitro culture. Of a total of 55 parthenogenetically activated bovine oocytes in treatment C, 80% of the embryos cleaved, 36% reached the blastocyst stage in 168 h and 25% hatched after 216 h of in vitro culture. Presence of nuclei was confirmed in all blastocysts produced by Hoechst 33342 staining and examination with epifluorescence microscopy. There were no significant differences in the number of fused couplets between treatment groups (treatments A and B). Also, no significant differences were detected in embryo cleavage, blastocyst and hatching rates among all treatments.
Table 1 Development of bovine embryos after somatic cell nuclear transfer (SCNT) and ooplasm transfer (OT).

aMII = metaphase II spindle.
bBLST = blastocysts.
cHBLST = hatched blastocysts.
dParthenogenetic control consisted of chemically activated bovine oocytes.
eOocytes were exposed to fusion buffer and electrical pulse (not fused) prior to chemical activation.
Experiment 2: staining of foreign mitochondria introduced by the OT procedure
Results from reconstructed bovine embryos imaged with confocal laser microscopy at 12, 72 and 144 h are summarized in Table 2. Localized clusters on foreign mitochondria were detected in 83% of the 12 h post-activation (precleavage) reconstructed couplets. No foreign mitochondria were observed in the remaining 17% of the 12 h post-activation reconstructed couplets. At 72 h post-activation, a clustered pattern of foreign mitochondria was observed in 75% of cleaved embryos. A dispersed pattern of foreign mitochondria was found in 17% of the cleaved embryos at 72 h post-activation and no mitochondria were observed in 8%. Of cleaved embryos imaged at 144 h post-activation, clusters of foreign mitochondria were detected in 33%, dispersed foreign mitochondria were observed in 33%, and no foreign mitochondria were found in the remaining 33%. A typical localized mitochondria cluster is illustrated in Fig. 2.
Table 2 Laser confocal results of bovine OT-derived mitochondria stained with MitoTracker® Green FM in OT-SCNT bovine embryos imaged after 12, 72 or 144 h of in vitro culture (IVC).

aPrecleavage confocal microscopy evaluation, thus embryo quality grades were not available.

Figure 2 Confocal image of the localization of OT-derived mitochondrial cluster (arrow) in an 8-cell, grade-1 embryo fixed after 72 h of in vitro culture.
All embryos imaged with confocal microscopy were evaluated and assigned a quality grade score before they were mounted onto microslides. The embryos were graded on a 1 to 4 scale according to IETS guidelines, with quality grade-1 embryos corresponding to excellent quality embryo with evenly-shaped blastomeres and no cytoplasmic fragments and a quality grade-4 embryos corresponding to a poor quality embryo with uneven blastomeres and cytoplasmic fragments. Embryo quality grades were not assigned during the confocal evaluations at 12 h of in vitro culture (precleavage). Of the embryos imaged at 72 and 144 h of in vitro culture, quality grade-1 and quality grade-2 embryos consistently displayed a cluster pattern of foreign mitochondria; whereas, the quality grade-3 embryos were found to display a dispersed pattern of mitochondria. In contrast, quality-4 embryos did not have a detectable signal for foreign mitochondria.
Experiment 3: iOT (caprine ooplasm injected into bovine oocytes) followed by iSCNT (caprine somatic cell injected into iOT-derived bovine oocytes)
A total of three replicates were conducted in this experiment. The developmental rates for embryos and parthenogenetically activated bovine and caprine oocyte controls are summarized in Table 3. Of a total of 60 reconstructed couplets in treatment A (iOT-iSCNT), 52% fused following two electrical pulses. Of fused couplets, 55% cleaved and then all embryos arrested at the 8- to 16-cell stage. Of a total of 55 reconstructed couplets in treatment B (iSCNT only), 82% fused after two electrical pulses. Of fused couplets, 78% of the embryos cleaved but none of the embryos progressed through the 8- to 16-cell stage. Of a total of 40 parthenogenetically activated bovine oocytes in treatment C, 85% of the embryos cleaved, 40% reached the blastocyst stage in 168 h and 22% hatched after 216 h of in vitro culture. Of a total of 25 parthenogenetically activated caprine oocytes in treatment D, 88% of the embryos cleaved, 32% reached the blastocyst stage in 168 h and 12% hatched after 216 h of in vitro culture.
Table 3 Development of embryos from transfer of caprine ooplasm into bovine recipient oocytes of caprine interspecies SCNT (iOT-iSCNT) or interspecies SCNT only (iSCNT only).

a ,b Mean values with different superscripts in the same column are significantly different (chi-squared test; p < 0.05).
1MII = metaphase II spindle.
2BLST = blastocysts.
3HBLST = hatched blastocysts.
4Oocytes were exposed to fusion buffer and electrical pulse (not fused) prior to chemical activation.
Couplets from iSCNT followed by goat iOT (treatment A) had a significantly lower fusion rate (p < 0.05) than couplets from iSCNT alone (treatment B). In addition, the number of cleaved embryos from iSCNT followed by goat iOT (treatment A) was significantly lower (p < 0.05) than the number of embryos cleaving from iSCNT alone (treatment B).
Discussion
Early studies in ooplasm and oocyte micromanipulation in laboratory animals subsequently led to the development and implementation of the OT technique in humans. Levron et al., (Reference Levron, Willadsen, Bertoli and Cohen1996) were among many that provided important basic information for the first OT in humans by obtaining mouse zygotes after fusion with synchronous and asynchronous cytoplasts. Since the birth of a child was reported after OT (Cohen et al., Reference Cohen, Scott, Schimmel, Levron and Willadsen1997) the procedure in humans has become subjected to controversy; the application of OT to overcome fertilization failures in older women has become a matter of concern due to the possibility of heteroplasmy of mitochondrial DNA. However, the technique remains a valuable tool to study early nuclear and cytoplasmic events and embryo development.
Meirelles et al. (Reference Meirelles and Smith1998) reported the production of heteroplasmic mice after transfer of cytoplasts followed by electrofusion and demonstrated that mitochondrial genotypes were segregated during the preimplantation period. More recently, Barritt et al. (Reference Barritt, Brenner, Malter and Cohen2001) attempted to determine if active mitochondria were transferred from human donor oocytes to recipient oocytes, and if these mitochondria could persist in preimplantation embryos. Observations after 48 h of in vitro culture showed segregation of labelled mitochondria to some, but not to all, blastomeres and demonstrated the recipient oocyte contained a heteroplasmic population of mitochondria after ooplasm transfer. Heteroplasmy was identified in two out of 15 ooplasm transfer children using the polymerase chain reaction (Brenner et al., Reference Brenner, Barritt, Willadsen and Cohen2000).
To our knowledge, the present study is the first report of OT used in combination with the SCNT procedure. In the first experiment, we demonstrated the two procedures can be successfully combined without any further detrimental effect on in vitro embryo development. Furthermore, the sequence of events (OT followed by SCNT or vice versa) had no effect on subsequent embryonic cleavage, blastocyst development or hatching rates. As none of these embryos were transferred to recipients in the present study, speculation can not be made about their subsequent viability. However, the presence of nuclei indicated that these embryos underwent apparently normal karyokinesis in conjunction with cytokinesis.
Our second experiment confirmed mitochondria are incorporated into the bovine recipient oocyte at the time of OT. This finding is in agreement with other studies conducted in mice (Meirelles et al., Reference Meirelles and Smith1998) and humans (Barritt et al., Reference Barritt, Brenner, Malter and Cohen2001). The present study also found that the majority of the foreign mitochondria remain in a distinct cluster pattern after 72 h of in vitro culture. However, the pattern of distribution after genomic activation was more difficult to predict. Although some embryos still showed ooplasmic clustering of foreign mitochondria, 33% exhibited a dispersed pattern indicating that foreign mitochondria could have relocated to other blastomeres. Constant levels of mtDNA have been detected during development from mature oocytes to blastocyst stage, leading to the proposal that mitochondria do not replicate their genomes during this early period of development (Pikó & Taylor, Reference Pikó and Taylor1987; Ebert et al., Reference Ebert, Liem and Hecht1988).
In the present study, MitoTracker® Green FM had no detrimental effect of subsequent embryo development. Because this probe preferentially accumulates in mitochondria regardless of its membrane potential (Molecular Probes' Product Information), it was chosen to identify the presence of foreign mitochondria introduced by the OT procedure, as well as a means to track the redistribution of foreign mitochondria during in vitro culture of the reconstructed embryo. The use of this probe, however, does not give information on the level of activity of the organelles. It has long been known that the movement and redistribution of mitochondria is an important part of normal cell function. Subcellular organelle dynamics are strongly influenced by interactions with cytoskeletal filaments and their associated motor proteins (Knowles et al., Reference Knowles, Guenza, Capaldi and Marcus2002). It is possible that the redistribution of organelles within the cytoskeleton is not only a function of their association with cytoskeletal components, but also of their level of activity and membrane potential. Certainly, further studies are needed in this area.
In our study, the higher quality-grade embryos (embryo quality grades 1 and 2) displayed a pattern of transferred mitochondria clustered in a small portion of the cytoplasm. However, as embryos were on a microslide and covered with a coverslip for confocal imaging, we could not verify this mitochondrial cluster was isolated to one single blastomere.
The use of alternative SCNT techniques, such as iSCNT, is an exciting possibility for species with limited availability of oocytes and recipients. However, an 8- to 16-cell in vitro developmental block has been previously reported using this procedure (Yoon et al., Reference Yoon, Choi, Han, Shim and Roh2001; Li et al., Reference Li, Siedel and Squires2002; Sansinena et al., Reference Sansinena, Reggio, Denniston and Godke2002, Reference Sansinena, Taylor, Taylor, Denniston and Godke2003, Reference Sansinena, Hylan, Hebert, Denniston and Godke2005), particularly when the species of donor karyoplasts and recipient cytoplasts are far apart in their taxonomic classification. Results from our third experiment indicated the transfer of ooplasm from the same species of the donor karyoplast into the bovine oocytes used for iSCNT had no effect in overcoming the in vitro block. However, the increased levels of heteroplasmy introduced after iOT may explain the decreased cleavage rates in this experiment. Previously, Nagao et al. (Reference Nagao, Totsuka, Atomi, Kaneda, Lindahl, Imai and Yonekawa1998a,Reference Nagao, Totsuka, Atomi, Yonekawa and Imaib) reported decreased physical performance in congenic mice with a mismatch between nuclear and mitochondrial genome. It is possible that although the bovine cytoplast appears capable of supporting a few mitotic divisions after iOT-iSCNT, heteroplasmy or mitochondrial incompatibilities may affect nuclear-cytoplasmic events occurring at the time of genomic activation.
In conclusion, the present study demonstrates that foreign mitochondria can be introduced with OT and that these mitochondria tend to remain as a distinct cluster without relocation after a few mitotic divisions. To our knowledge, this is the first scientific report of OT used in combination with iSCNT in an attempt to overcome the in vitro developmental block. Further work is needed to determine the characteristics of foreign mitochondrial dynamics as well as the replication of foreign mitochondria introduced into NT embryos.
Acknowledgements
The authors would like to thank Hollie Donze for her assistance in confocal imaging. Approved for publication by the Director of the Louisiana Agricultural Experiment Station as Manuscript No. 05–18–0178. This research was funded, in part, by the Louisiana Agricultural Experiment Station and the Federal Multistate Project W-171. This research was also made possible by NIH Grant Number P20 RR16456 from the BRIN Program of the National Center for Research Resources. The manuscript's contents are solely the responsibility of the authors and do not necessarily represent the official views of NIH.