Introduction
Mammalian ovaries consist of follicles with oocyte surrounded by granulosa cells (GCs) and thecal cells (TCs). These follicles develop through primordial, primary, and secondary stages before acquiring an antral cavity (antral follicle) (Pangas et al., Reference Pangas, Rajkovic, Vinci and Carpi2015). Most follicles are lost by atresia and only a few follicles reach the preovulatory stage (Graafian follicle) and ovulate (Krysko et al., Reference Krysko, Diez-Fraile, Criel, Svistunov, Vandenabeele and D’Herde2008). Ovarian follicles undergo atresia mainly through GC death by apoptosis (Matsuda-Minehata et al., Reference Matsuda-Minehata, Inoue, Goto and Manabe2006). Pituitary gonadotropins (follicle-stimulating hormone – FSH, and luteinizing hormone – LH) promote follicular development and prevention of atresia. FSH regulates gene expression and post-translational modification of proteins that inhibit apoptosis, stimulate proliferation, trigger steroidogenesis and promote cell differentiation, allowing GCs to respond appropriately to the preovulatory gonadotropin surge. Next, LH prevents atresia of preovulatory follicles and promotes ovulation (Erickson and Danforth, Reference Erickson and Danforth1995).
In addition to the gonadotropins, many growth factors and intraovarian regulators such as Bcl-2 (Wang et al., Reference Wang, Wu, Tan and Bin Zeng2012), insulin-like growth factor (Hu et al., Reference Hu, Cowan, Harman and Quirk2004) and members of the TGF-β superfamily (inhibins and activins) (Hillier, Reference Hillier2009) also act on follicular development and the survival of ovarian follicles. Apoptosis of GCs occurs through extrinsic and intrinsic signalling pathways (Hussein, Reference Hussein2005; Matsuda et al., Reference Matsuda, Inoue, Manabe and Ohkura2012). Ligand binding to cell-surface receptors mediates the extrinsic pathway, which activates caspase-8, while the intrinsic pathway is triggered by the mitochondrial release of cytochrome c initiated by caspase-9. These initiator caspases activate executioner caspases, such as caspase 3, that degrade cellular components producing morphological changes observed in apoptosis (Johnson and Bridgham, Reference Johnson and Bridgham2002).
Previous studies have established a model to investigate the mechanisms of atresia in preovulatory follicles after stimulation of immature rats with equine chorionic gonadotropin (eCG) (Braw and Tsafriri, Reference Braw and Tsafriri1980; Choi et al., Reference Choi, Jo, Lee, Yoon and Choi2010; Dhanasekaran and Moudgal, Reference Dhanasekaran and Moudgal1989; Hughes and Gorospe, Reference Hughes and Gorospe1991). This model is obtained through recruitment of a follicular pool by a single injection of eCG, which displays FSH-like effects. After 48 h, the ovaries present many preovulatory follicles. Considering LH inhibits apoptosis in preovulatory follicles and promotes ovulation in cycling rats, the absence of a preovulatory LH surge in immature rats will induce atresia of preovulatory follicles. Therefore, the aim of the present study was to investigate the occurrence of ovulation and provide some morphological features of GC apoptosis of atretic follicles from this model of atresia in preovulatory follicles.
Materials and methods
Immature female Wistar rats (25 days old, five animals per group) were obtained from CEBIO/Federal University of Minas Gerais (Belo Horizonte, Brazil). The animals were maintained in a room with controlled temperature (22±2°C) and a 12 h light: 12 h dark cycle, with free access to tap water and standard rat chow (Nuvilab, Brazil). The animals received a single subcutaneous injection of 20 IU eCG (NHPP, Torrance, CA, USA) to induce follicular development and atresia (Braw and Tsafriri, Reference Braw and Tsafriri1980; Choi et al., Reference Choi, Jo, Lee, Yoon and Choi2010; Dhanasekaran and Moudgal, Reference Dhanasekaran and Moudgal1989; Hughes and Gorospe, Reference Hughes and Gorospe1991). Animals were anaesthetised with ketamine–xylazine and euthanized at 0, 48, 72 or 120 h after gonadotropin stimulation. The ovaries were immediately removed and weighed. The right ovaries were frozen in liquid nitrogen for the dosage of ovarian steroids. Fragments of the left ovaries were fixed in phosphate-buffered formaldehyde for histology/immunohistochemistry. Next, they were serially dehydrated in graded ethanol baths, embedded in paraffin (Histotec Pastilles—Merck, Darmstadt, Germany), sectioned at 5 µm and stained with haematoxylin and eosin (H&E). Images were acquired using an Olympus B53 microscope.
Immunodetection of cleaved caspase-3, an executioner caspase that promotes morphological changes observed in apoptosis, was performed by the avidin–biotin–peroxidase method using the Vectastain Universal Elite ABC Kit (Vector Laboratories, Burlingame, CA, USA), as described previously (Gonzaga et al., Reference Gonzaga, Campolina-Silva, Werneck-Gomes and Oliveira2017), using anti-cleaved caspase-3 antibody (Cell Signaling Technology-Massachusetts) diluted 1:1000 dilution as primary antibody. Ovarian sections with 5-µm thick were dewaxed in xylene, hydrated through a series of descending concentrations of alcohol to water and endogenous peroxidase activity was blocked in 0.6% hydrogen peroxide in methanol for 30 min. After microwaving for antigen retrieval in 0.1 M citrate buffer pH 6.0, non-specific antibody binding was blocked with normal goat serum (10%). Sections with primary antibodies were incubated overnight at 4°C. Sections without primary antibodies were used as negative controls. Next, sections were incubated with biotinylated goat anti-rabbit secondary antibody (Dako-Carpinteria), diluted 1:100 for 30 min and with the avidin–biotin complex (1:100 dilution) for 30 min. The immunoreaction was visualized using 0.05% (p/v) diaminobenzidine containing 0.01% (v/v) hydrogen peroxide in 0.05 M Tris–HCl buffer, pH 7.6. Immunostaining was performed in duplicate to confirm the results.
For transmission electron microscopy (TEM), ovarian fragments were fixed in Karnovsky’s solution (2.5% glutaraldehyde and 2% paraformaldehyde) in 0.1 M cacodylate buffer pH 7.4 overnight at 4°C. Fragments were postfixed in 2% (w/v) osmium tetroxide and 1.5% (w/v) potassium ferrocyanide for 2 h at room temperature. They were washed in distilled water and kept in 2% uranyl acetate (en bloc staining) overnight. Then, they were dehydrated and embedded in Epon 812 (EMS, Hatfield, PA, USA). Specimens were sectioned in 50 nm ultrathin sections, stained with Reynolds lead citrate and analyzed in a Tecnai G2-12 Spirit electron microscope (FEI Company, Hillsboro, Oregon, USA) at 80 kV.
Ovarian steroids extraction were performed as previously described (Pereira et al., Reference Pereira, Honorato-Sampaio, Martins, Reis and Reis2014), and testosterone (T), 17β-estradiol (E2) and progesterone (P4) levels were determined by enzyme-linked immunosorbent assay (ELISA) using commercial kits (DRG Diagnostics, Marburg, Germany). The assays were performed according to the manufacturers’ instructions and all samples were measured in duplicate.
Data were expressed as the mean±standard error of the mean (SEM), and differences were considered significant if P-values were<0.05. Data exhibited normal distribution by Kolmogorov–Smirnov test and were analyzed using one-way analysis of variance (ANOVA) followed by Tukey’s post-hoc test.
Results and Discussion
In the present study, we observed ovulation after the induction of follicular development in an well established model of atresia in immature rats treated with eCG (Braw and Tsafriri, Reference Braw and Tsafriri1980; Choi et al., Reference Choi, Jo, Lee, Yoon and Choi2010; Dhanasekaran and Moudgal, Reference Dhanasekaran and Moudgal1989; Hughes and Gorospe, Reference Hughes and Gorospe1991). Untreated animals (0 h) showed primordial follicles (Pr) surrounded by a single layer of flat follicular cells, primary follicles with a monolayer of cubic follicular cells and secondary follicles, which possessed more than one layer of follicular cells without visible follicle fluid (antrum) (Fig. 1A, B ). Ovaries of untreated animals also exhibited antral follicles with oocytes surrounded by differentiated GCs and TCs, and an accumulation of liquid forming the antral cavity (Fig. 1C ). After 48 h of stimulation with eCG, we observed large antral follicles (Fig. 1D ). Preovulatory follicles were observed 72 h after eCG stimulation ( Fig 1E ), and two out of five rats displayed cumulus–oocyte complexes (COCs) in the oviducts (Fig. 1F ). All animals exhibited corpora lutea after 120 h (Fig. 1G ). According to the literature, this treatment induces atresia in preovulatory follicles due to gonadotropin surge deprivation (Choi et al., Reference Choi, Jo, Lee, Yoon and Choi2010; Hughes and Gorospe, Reference Hughes and Gorospe1991). So, the presence of corpora lutea (on days 4 and 5) after eCG treatment was rare (5%) in experimental animals (Kim et al., Reference Kim, Yoon and Tsang1999). In the present study, all animals had been ovulated 120 h after eCG stimulation, suggesting an endogenous gonadotropin surge had happened. The induction of atresia after eCG injection resembles the widely used protocols of ovulation based on exogenous gonadotropins stimulation (Luo and Zhu, Reference Luo and Zhu2000). When prepubertal rats are injected with eCG, a follicular pool recruitment will take place. After 48–50 h, if the rats are injected with human chorionic gonadotropin (hCG), which simulates endogenous LH surge, the animals will ovulate (Luo and Zhu, Reference Luo and Zhu2000).

Figure 1 Histological sections (H&E) of rat ovaries showing follicular development 0 h (A–C), 48 h (D), 72 h (E) and 120 h (G) after stimulation with equine chorionic gonadotropin (eCG). (B) High magnification of selected area from (A) showing primordial follicles (Pr) surrounded by a single layer of flat follicular cells, primary follicle (PI) with a single layer of cubic follicular cells and secondary follicles (PII) with a multiple layers of follicular cells. (C) High magnification of a second selected area from (A) showing antral follicle (AF) with differentiated granulosa cells (GCs) and theca cells (TCs) surrounding oocyte (Oo) and beginning of antral cavity (AC) formation. (D) Antral follicle (AF). (E) GF: Graafian/preovulatory follicle (GF). (F) Cumulus–oocyte complex (arrowhead) in the oviducts 72 h after eCG stimulation, indicating that ovulation happened. (G) Corpus luteum (CL). Scale bars represent 200 µm (A, B, E–G) and 40 µm (C, D).
We also measured T, E2, and P4 from ovaries to evaluate the ovarian steroidogenic profile. T and E2 reached to higher levels after 48 h of eCG treatment (Fig. 2A, B ) and P4 levels increased 7.8-fold at 120 h compared with 0 h (Fig. 2C ). The increased E2 levels 48 h after eCG treatment might contribute to promoting a positive feedback in the hypothalamus–pituitary axis, culminating in endogenous gonadotropin-releasing hormone (GnRH) and LH surges that triggered ovulation (Levine, Reference Levine2015). The high level of E2 exhibits a preovulatory positive feedback in the hypothalamus, as well documented in the literature (Richards et al., Reference Richards, Liu and Shimada2015). This positive effect of E2 on LH surge was first reported in 1971 with adult ovariectomized rats treated with exogenous E2 (Caligaris et al., Reference Caligaris, Astrada and Taleisnik1971).

Figure 2 Ovarian testosterone (T; A), 17β-estradiol (E2; B) and progesterone (P4; C) 0 h, 48 h, 72 h and 120 h after eCG stimulation. Values represent means±standard error of the mean (SEM) (n=5/group). Common lowercase letters indicate that means do not differ significantly (P<0.05).
We also observed higher P4 levels 120 h after eCG stimulation, indicating the presence of functional corpora lutea. If ovulation occurs, the remaining GCs and TCs will become a corpus luteum that is associated with cellular hypertrophy and increased P4 synthesis and release. LH stimulates expression of P-450 cholesterol side chain cleavage (SCC), which converts cholesterol to pregnenolone, and 3β-hydroxysteroid dehydrogenase (3β-HSD), that converts pregnenolone to P4 (Hunzicker-Dunn and Mayo, Reference Hunzicker-Dunn and Mayo2015).
In spite of the ovulation confirmed by COCs in the oviducts, hormonal dosage and corpus luteum in ovarian sections, we observed atresia in early follicles. We considered atresia in any follicle showing pyknotic granulosa cell nuclei (Braw and Tsafriri, Reference Braw and Tsafriri1980). Pyknotic bodies were randomly distributed throughout atretic follicles in the granulosa layer of follicles (Fig. 3A ). We observed atretic follicles with fragmented oocytes showing loss of contacts between the oocytes and GCs (Fig. 3B ). Macrophages were commonly found in atretic follicles. They were recognized by acidophilic cytoplasm and vesicular nucleus in the antral cavity or in the granulosa layer (Fig. 3A, B ). Previous studies have shown the presence of macrophages in any phase of follicular development (Katabuchi et al., Reference Katabuchi, Fukumatsu, Araki, Suenaga, Ohtake and Okamura1996; Wu et al., Reference Wu, Van der Hoek, Ryan, Norman and Robker2004). In atretic follicles, GCs in apoptosis might stimulate a recruitment of macrophages as reported in atretic follicles induced by nanosilver particles (Mirzaei et al., Reference Mirzaei, Razi and Sadrkhanlou2017).
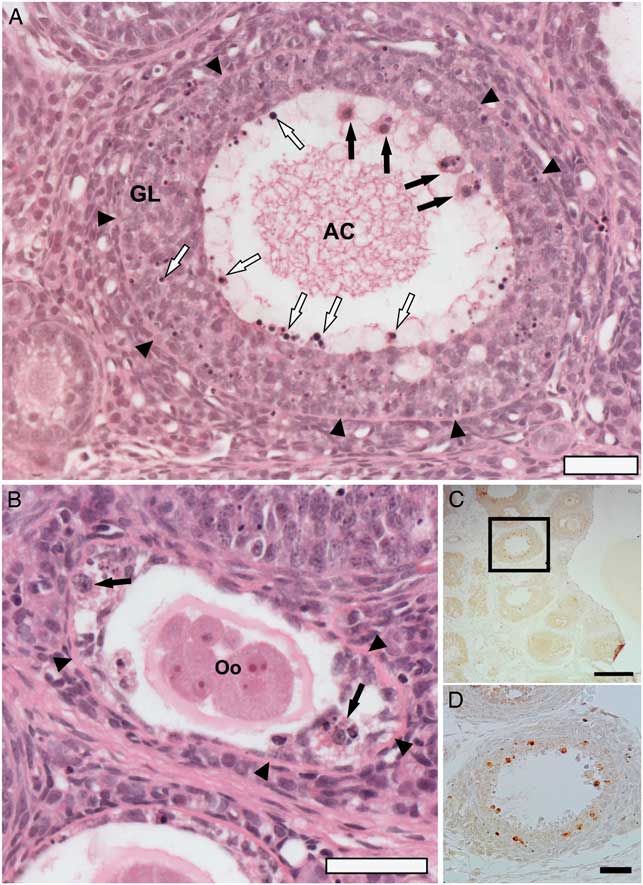
Figure 3 Apoptosis of granulosa cells (GCs) of atretic follicles from rat ovaries after eCG stimulation observed in ovarian sections stained by H&E (A, B) and immune labelling for cleaved caspase-3 by avidin–biotin–peroxidase method (C, D). (A) Antral follicle with pyknotic nuclei of GCs (white arrows) in antral cavity (AC) and in granulosa layer (GL), and the presence of macrophages (black arrows). (B) Secondary follicle showing fragmentation of the oocyte (Oo) and macrophages (black arrows). (C, D) Immunolocalization of cleaved caspase-3 in granulosa cells (brown staining). Arrowheads: basement membrane between theca and granulosa cells. Scale bars represent 200 µm (C) and 40 µm (A, B, D).
To confirm apoptosis in GCs, we used immunohistochemistry for cleaved caspase 3 and TEM, which is a standard method of detecting apoptosis of the cell. The GCs from atretic follicles showed positive staining for cleaved caspase-3, an effective marker of apoptosis (Fig. 3C, D ), which is activated in both apoptotic extrinsic and intrinsic pathways (Johnson and Bridgham, Reference Johnson and Bridgham2002; Hussein, Reference Hussein2005), corroborating the hypothesis that apoptosis of GCs plays a pivotal role in atresia.
Many studies used terminal deoxynucleotidyl transferase dUTP nick end labelling (TUNEL) to investigate the follicular atresia in immature rats treated with eCG (Asselin et al., Reference Asselin, Wang and Tsang2001; Boone et al., Reference Boone, Carnegie, Rippstein and Tsang1997; Kim et al., Reference Kim, Yoon and Tsang1999; Zwain and Amato, Reference Zwain and Amato2000). This assay is widely used to identify and quantify apoptotic cells through detection of DNA fragmentation (Gavrieli et al., Reference Gavrieli, Sherman and Ben-Sasson1992). However, TUNEL labels any cell with DNA cleavage, such as in autolysis and necrosis (Grasl-Kraupp et al., Reference Grasl-Kraupp, Ruttkay-Nedecky, Koudelka, Bukowska, Bursch and Schulte-Hermann1995). Therefore, identification of apoptosis stills requires the demonstration of morphological and ultrastructural features. Ultrastructure of follicles revealed GCs with developed rough endoplasmic reticulum, Golgi apparatus, mitochondria and contacts such as tight junctions coupled adjacent cells (Fig. 4A, B ). Atretic follicles exhibited GCs with a compacted chromatin aggregated against the nuclear envelope, nuclear fragmentation, cell shrinkage and fragmentation similar to apoptotic bodies (Fig. 4C, D ). These cells with apoptotic characteristics were observed in secondary and antral stages any time after eCG treatment. No preovulatory follicle showed signs of chorionic gonadotropin (CG) apoptosis. Apoptotic GCs in some small antral follicles and no apoptotic cells in preovulatory follicles 48 h and 72 h after eCG stimulation were also reported previously, suggesting GCs from small follicles are liable to apoptosis and sensitive to atretogenic factors (Luo and Zhu, Reference Luo and Zhu2000). This gonadotropin inhibits the expression of target genes that induce apoptosis (Wang et al., Reference Wang, Wu, Tan and Bin Zeng2012).

Figure 4 Transmission electron microscopy. (A) Healthy granulosa cells. (B) High magnification of selected area from (A) showing tight junctions (arrowheads). (C) Nucleus with compacted chromatin underling the nuclear envelope (asterisk), indicating apoptosis. (D) Apoptotic bodies (AB) of granulosa cells in apoptosis. ER, endoplasmic reticulum; G, Golgi apparatus; Mit, mitochondria; N, nucleus.
In the present study, the animals were immature and the preovulatory LH surge would not happen. The animals were 25 days old and puberty in rats occurs when they are around 42 days old (Sengupta, Reference Sengupta2014). Without a preovulatory LH surge, the follicles recruited by eCG would undergo atresia. Luo and Zhu (Reference Luo and Zhu2000) documented apoptotic cells from preovulatory follicles 120 h after eCG stimulation. In the present study, we did not observe any preovulatory follicle 120 h after eCG treatment and the ovaries exhibited corpora lutea at this moment, indicating that the preovulatory follicles had ovulated. The absence of atretic preovulatory follicles might also be related to the high levels of ovarian E2 prior to gonadotropin surge. It is well known that oestrogen is associated with good follicular growth and exhibits anti-atresia effects (Revelli et al., Reference Revelli, Piane, Casano, Molinari, Massobrio and Rinaudo2009). E2 acts by binding to two nuclear receptors (ERα and ERβ) and a recently discovered G protein-coupled oestrogen receptor 1 (GPER), all expressed in GCs (Pavlik et al., Reference Pavlik, Wypior, Hecht, Papadopoulos, Kupka, Thaler, Wiest, Pestka, Friese and Jeschke2011). These receptors stimulate the phosphoinositide 3-kinase/protein kinase B (IP3K–Akt) pathway that is linked to cellular survive (Heublein et al., Reference Friese, Jarrin-Franco, Lenhard, Mayerhofer and Jeschke2014). PI3K–Akt, which inactivates the protein Bad, a member of the family pro-apoptotic Bcl-2 (Quirk et al., Reference Quirk, Cowan, Harman, Hu and Porter2004), and caspase-9 (Johnson and Bridgham, Reference Johnson and Bridgham2002), prevents apoptosis (Franke et al., Reference Franke, Kaplan and Cantley1997; Quirk et al., Reference Quirk, Cowan, Harman, Hu and Porter2004).
In summary, the present study reported the spontaneous ovulation in a well established rat model of atresia in immature eCG-treated rats and GCs apoptosis in early follicles. We also recommend that researchers should take on board the possibility of an endogenous gonadotropin surge in this model that culminates in ovulation that might prevent studies of atresia in preovulatory follicles.
Acknowledgements
We are grateful to the Center of Microscopy at the Universidade Federal de Minas Gerais (http://www.microscopia.ufmg.br) for providing the equipment and technical support for experiments involving electron microscopy and Dr Cleida A. Oliveira for generous donation of the anti-cleaved caspase-3 antibody.
Financial support
This study was supported by Fundação de Amparo a Pesquisa do Estado de Minas Gerais FAPEMIG/Brazil (protocol APQ-00743–14) and Pró-Reitoria de Pós-Graduação e Pesquisa/UFVJM (grant to PIBIC scholarship to F. S. B.).
Conflict of interest
The authors declare no conflicts of interest.
Ethical standards
The experimental procedures were approved by the institutional Ethics Committee on Animal Use (Process no. 40/2016 CEUA/UFVJM).