Introduction
Sexual reproduction is the primary means by which genetic diversity is introduced into populations of higher plants or animals. The union of genes that occurs during fertilization is a critical evolutionary mechanism and the means by which humans have improved cultivars through selective plant breeding. The location of the gametes, however, particularly the egg cell, within paternal or maternal tissue, restricts our ability to explore the fertilization process of higher plants. As a result, few detailed studies have been conducted that enhance our understanding of the fertilization process. The technique of in vitro fertilization, fusing isolated sperm and egg cells under controlled conditions, removes possible interference by somatic tissue, thereby providing opportunities for more detailed studies of angiosperm fertilization. Kranz et al. (Reference Kranz, Bautor and Lörz1991) first fused the sperm and egg cells of maize to produce an ‘artificial’ zygote by electrofusion. Ultimately, this artificial maize zygote developed into a fertile plant (Kranz & Lörz, Reference Kranz and Löra1993). Now, in vitro fertilization techniques have been developed that can be used to study important applied reproductive topics or fields such as the molecular biology of male and female gametes, distant hybridization and cellular genetic engineering (Wang et al., Reference Wang, Kuang, Russell and Tian2006). Until recently, the technique of in vitro fertilization has been successful only in maize and wheat, with most of the available data about zygote activation coming only from maize. Recent methodological advances have allowed for the application of in vitro fertilization to other, particularly dicotyledon, angiosperms (Wang et al., Reference Wang, Kuang, Russell and Tian2006).
The isolation of egg cells is difficult in angiosperms because the egg cell is deeply embedded within the ovule. To date, egg cells of tobacco (Hu et al., 1985), maize (Kranz et al., Reference Kranz, Bautor and Lörz1991), petunia (Van Went & Kwee, Reference Van Went and Kwee1990), perennial ryegrass (Van der Mass et al., Reference Van Der Maas, Zaal, de Jong, Krens and Van Went1993), wheat (Kovács et al., Reference Kovács, Barnabás and Kranz1994), barley (Holm et al., Reference Holm, Knudsen, Mourtzen, Negri, Olsen and Roué1994) and rice (Zhang et al., Reference Zhang, Dong, Galli and Potrykus1999) have been isolated. Torenia fournieri is a suitable species for fertilization research because its embryo sac protrudes partially through the micropyle and its egg cell, two synergids and part of the central cell can be clearly observed using light microscopy. Based on the unique embryo sac of T. fournieri, some studies on its in vivo fertilization mechanism had been reported (Higashiyama et al., Reference Higashiyama, Kuroiwa, Kawano and Kuroiwa1998, Reference Higashiyama, Kuroiwa, Kawano and Kuroiwa2000, Reference Higashiyama, Yabe, Sasaki, Nishimura, Miyagishima, Kuroiwa and Kuroiwa2001; Fu et al., Reference Fu, Yuan, Huang, Yang, Zee and O'Brien2000; Han et al., Reference Han, Huang and Zee2000, Reference Han, Huang, Guo, Zee and Gu2002; Wallwork & Sedgley, Reference Wallwork and Sedgley2000). The advantageous features of the T. fournieri embryo sac make it easier – relative to many other angiosperms – to isolate vigorous egg cells. Mól (Reference Mól1986), for example, isolated egg cells of T. fournieri using enzymatic digestion. Imre & Kristóf (Reference Imre and Kristóf1999) also isolated protoplasts of embryo sac cells including egg cells using 2% cellulase Onozuka-R10 and macerozyme R10. Based on the successful isolation of nearly mature sperm from the pollen tube of T. fournieri (Chen et al., Reference Chen, Liao, Kuang and Tian2006), we present a protocol for isolating mature, living egg cells and zygotes of T. fournieri using micromanipulation or a combination method of enzyme maceration and micromanipulation. Considering sperm cell of Plumbago zeylanica (Zhang & Russell, Reference Zhang and Russell1999) and Nicotiana tabacum (Yang et al., 2005) displaying the velocity in a current field we measured the velocity of the egg cell and zygote and compared the mobility of both cells using electrophoresis.
Materials and Methods
Torenia fournieri L. plants were grown under a 14 h: 10 h, light : dark cycle at 22 °C (dark) and 28 °C (light) at Xiamen University. Flower buds were emasculated before anthesis. The emasculated flowers were collected just at anthesis; 1 or 2 days after anthesis for egg cell isolation. The artificially pollinated flowers were collected at about 14 h and 18 h for zygote isolation.
Several ovaries were dissected under a stereomicroscope and the ovules were removed from the placenta by gentle scraping. All of the ovules were put into 2 ml of an isolation solution containing 5 mM CaCl2, 5 mM KH2PO4, 0.7 mM MgSO4, 3 mM MES (2-N-morpholino ethanesulfonic acid) and 3–9% (w/v) mannitol (197–500 mOsmol/kg H2O) at a pH of 5.8. For mechanical isolation of an egg cell, the embryo sacs were dissected with an ultra-fine needle under an inverted microscope. The partial protrusion of the embryo sac through the micropyle of ovule facilitated penetration into the embryo sac wall and incision near the egg cell. The egg cell was then released by pressing the micropylar end of the embryo sac. Two synergid cells could be released together with the egg.
For isolation of egg cell and zygote using an enzyme, 0.1–0.2% cellulase (Onozyka R-10) and 0.1–0.2% pectinase (Serva) were added into the isolation solution. The ovules were incubated in the solution for 0.5 h with gentle shaking (30 rpm) and then dissected using a needle.
Micro-electrophoresis was conducted in a 6-cm Petri dish. Two small pieces of glass (cut from microscope slides) were attached with a glue to form a 5 mm wide, 5 cm long trough in the centre of the dish. Both ends of the trough were sealed with 2% agarose to form two ‘reservoirs’ on either side of the trough. The electrophoresis trough and the reservoirs were filled with low ionic buffer (van Oss & Fike, Reference Van Oss, Fuke, Righetti, Oss and Vanderhoff1979), containing 10 mM NaH2PO4, 0.3 mM citric acid and 7% mannitol. Buffers with different pH (4–9) were tested to find the optimal pH. Two electrodes were separately immersed into the ‘reservoirs’. Egg cells or zygotes isolated from the embryo sac were transferred with a microinjector into the trough in the centre of the optical field. Selected voltages were applied with two electrodes with constant current and the mobility of egg or zygote was observed using an inverted phase-contrast microscope. Velocity was measured using the recorded images and electrophoretic mobility was calculated by the equation (van Oss & Fike, Reference Van Oss, Fuke, Righetti, Oss and Vanderhoff1979):

where μ is the electrophoresis mobility, d is the distance (in micrometres) travelled by cells during measurement, t is the time (in seconds) required by cells to travel that distance, V is the potential applied to the electrodes (in volts) and day is the length of the trough (in centimetres) (Zhang & Russell, Reference Zhang and Russell1999).
Results
Isolation of living egg cells
The stigma of T. fournieri consists of two halves or slivers, which are closed just when the petal opens. The two halves of the stigma usually open the next day after anthesis and are receptive to pollination for 2 days following anthesis. An egg and two synergids can be observed clearly at anthesis in the partially protruding embryo sac. The day after anthesis, a second nucleus in the central cell moves to the side of egg cell, developing to a physiological mature state for fertilization. Egg and synergid cells in the embryo sac are pear-like. The egg cell is bigger than both synergids and has several large vacuoles, which makes it easy to identify and separate from the two synergids and also from the nuclear cells (Fig. 1).
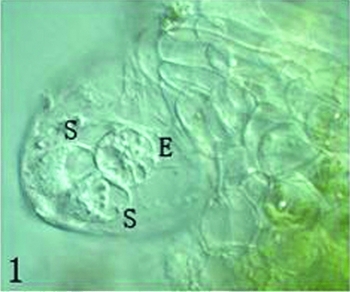
Figure 1 In an ovule, naked embryo sac partly protruding out micropylar and an egg cell (E) and two synergids (S) could be seen clearly. Scale ×400.
The protruded embryo sac was first excided in its central part using a glass needle and then the micropylar part of embryo sac was gently extruded; the egg and synergid cells spilled out from the cut end of embryo sac. Just released egg and synergid cells were still connected by some cytoplasm material (Figs. 2, 3), but soon became separated each other following the cytoplasm dissolving (Figs. 4, 5). Several large vacuoles occupied much of the egg cell volume, with the nucleus and most of the cytoplasm being located along the cell periphery (Fig. 4). The average surface area and volume of egg cells are 2590.87 ± 328.16 μm2 and 12473.45 ± 234.20 μm3, respectively (Table 1). Mechanical isolated living egg cells are especially effective for the recognition of male and female gametes in non-enzyme in vitro fertilization assays.
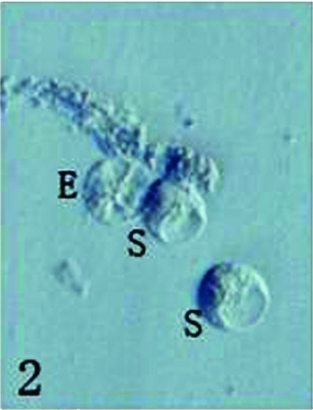
Figure 2 Just released one egg cell (E) and two synergid cells (S). Scale ×400.

Figure 3 The viability of isolated egg cell and synergids was shown by FDA reaction. Scale ×400.
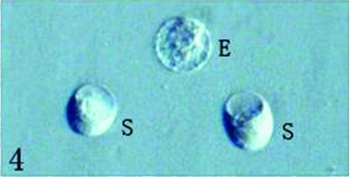
Figure 4 Egg cell (E) is different with two synergids (S). Scale ×400.
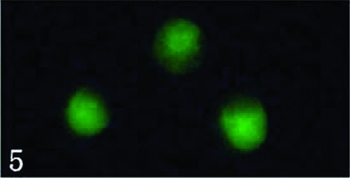
Figure 5 The viability of three cells was evaluated by FDA reaction. Scale ×400.
Table 1 The surface, size and mobility of Torenia fournieri egg cells and zygotes

Egg cells in embryo sacs at different stages displayed various sensitivities to the osmolarity of the isolation media (Table 2). The egg cells in embryo sacs of all stages were ruptured or shrunken in an isolation solution containing either 3% and 9% mannitol, indicating the osmotic pressures of these isolation media were too low or high. The egg cells in the flowers at anthesis and 1 day after anthesis could easily be isolated in a 5% mannitol isolation solution but it became difficult to isolate eggs cells that were collected 3 days after anthesis in the 5% solution. In the 7% mannitol solution, isolation was difficult with at-anthesis egg cells but easier with 1 day postanthesis egg cells. The isolated egg cells, however, from the 7% mannitol solution would not ‘keep’ as long as those obtained in the 5% mannitol. The isolation solution, therefore, containing 5% mannitol was judged to be the most suitable for egg cell isolation.
Table 2 Effect of different osmolalities of isolation media on the isolation and conservation of egg cells at different stages

Our studies demonstrated that enzymes could evidently increase the egg cell isolation rate from 5% (2–3 egg cells in 50 intact ovules) to 18% (8–10 egg cells in 50 intact ovules) when 0.1% cellulase and 0.1% pectinase were added to the isolation solution (Table 3). When the enzyme levels were increased to 0.2%, the released egg cells were more difficult to identify because the ovules also released numerous protoplasts of various sizes. In addition, in 0.2% enzymes egg cells easily became anamorphic, suggesting a deleterious effect from the enzymes at the higher concentration. Isolated living egg cells can be combined into a pure population using a micromanipulator (Fig. 6), which is especially effective for making a cDNA library of egg cell. The viability of isolated egg cells can apparently be determined by the presence of cytoplasmic fluorescence in the fluorescein diacetate (FDA) test at 4 h after isolation (Fig. 7).
Table 3 Concentration of enzymes and mannitol in the maceration medium (%) and their relationship to egg and zygote isolation (2 d after anthesis)


Figure 6 Seven collected egg cells after 4 h isolated. Scale ×400.

Figure 7 The viability of these egg cells was evaluated by FDA reaction. Scale ×400.
Isolation of living zygotes
Most ovules displayed a pollen tube in its micropyle at 14 h after pollination and one synergid that could not be seen clearly (Fig. 8), suggesting that fertilization of the egg cell occurred at this time. Eighteen hours after pollination, the fertilized egg cell began to elongate in the embryo sac. The nucleus with a larger nucleolus migrated to the chalazal end of the cell, resulting in polarity of the cell (Fig. 9).

Figure 8 In an ovule 14 h after pollination, a pollen tube (arrow) residue could be seen. Scale ×400.

Figure 9 In an ovule 18 h after pollination, zygote began to elongate. Scale ×400.
Isolation of the zygote occurred in the same manner as the above-mentioned egg-cell isolation method. Most zygote isolations were completed using cellulase and pectinase because the enzymes substantially improved this process. In the 7% mannitol solution, 2–4 zygotes could be isolated from 50 ovules (about 5%). when 0.1% cellulose and 0.1% pectinase were added to the isolation solution, however, the number of isolated zygotes could exceed 10 (>20%) in 50 ovules. Zygotes took on a spherical shape at 0.5 h after the ovule was incubated in an enzymatic maceration mixture. When the zygote was released from the embryo sac and assumed a round shape, its nucleus was located in the center of the cell with more cytoplasm surrounding nucleus (Fig. 10). The average surface area of zygotes was 2089.93 ± 423.24 μm2 and their volume 9115.49 ± 279.54 μm3 (Table 1), which was smaller than that of egg cell. The isolated zygotes could be collected into a pure population using a micromanipulator (Fig. 8). In the viability test with FDA, zygote fluorescence remained viable for 5 h in the 7% mannitol solution and was mainly located in its central region, which was different from the egg cell (Fig. 11).

Figure 10 Six collected zygotes. Scale ×400.

Figure 11 The viability of these zygotes was shown by FDA reaction. Scale ×400.
Compared with egg isolation, zygote isolation was most effectively completed in a solution of higher osmolality. While zygotes could be isolated in a 5% mannitol solution, it was necessary to press the micropylar end to release them when the embryo sac was excided by a needle. In addition, zygotes swelled soon after excision and were easily broken after only 2 h, as opposed to 5 h of viability in the 7% mannitol solution.
Micro-electrophoresis of egg and zygote
Buffer pH can have a major effect on the viability and electrophoresis of both egg cell and zygote. The movement of egg cell or zygote in an electric field depends on its surface charge. The pH of the buffer can alter egg cell and zygote mobility and can even poison both cells. In either pH 4 or 9 buffers, both egg cell and zygote became brown and died immediately upon release into the buffer. In the pH 5 or 8 buffers, egg cells and zygotes all migrated toward the negative pole at various speeds; both cells also broke shortly after voltage was applied to the electrophoretic solution. In the pH 6 buffer, both egg and zygote migrated toward the negative pole, moved faster than in the pH 7 buffer and remained intact for 40 min during electrophoresis. The optimal buffer pH for electrophoresis of egg cells and zygotes in T. fournieri, therefore, is 6 (Table 4).
Table 4 pH of buffer effect on egg cells and zygotes during both electrophoresis

aThe best condition.
When egg cell or zygote was placed separately into an electric field (voltage 70 mV, current 10 mA) filled with the optimal buffer, they both migrated toward the negative pole, indicating positive charges on the surface of the cells (Figs. 12, 13). The egg cell moved slower than zygote. The average velocity of 10 egg cells was 1.078 ± 0.020 μm/s, while the average velocity of 25 zygotes was 1.379 ± 0.023 μm/s (Table 1), suggesting that zygotes may have a larger surface charge.

Figure 12 A egg cell was moving toward the negative pole in an electric field. The time interval was 4 s. Scale ×200.

Figure 13 A zygote was moving toward the negative pole in an electric field. The time interval was 4 s. Scale ×200.
Discussion
At present, the biggest obstacle to using in vitro fertilization to perform research on reproduction in higher plants is the isolation of male and female gametes, especially the isolation of the egg cell. Egg cells were isolated using two methods, either mechanical micromanipulation or a combination of enzyme maceration and micromanipulation. These two methods have both advantages and disadvantages. Mechanical isolation of an egg cell was more difficult and fewer cells were isolated, but this method avoided a potential problem with enzyme digestion of the proteins located on the surface of egg plasma membrane (Cao & Russell, Reference Cao and Russell1997). In this assay, the egg cells of T. fournieri can be isolated without enzymatic digestion, although the number recovered was only 4–6% (2–3/50 ovules). The isolated egg cells are comparatively similar to those in vivo, so that the results achieved using isolated cells are appropriate for defining fertilization mechanisms, especially in male and female gametic recognition studies. Enzymes can poison the isolated cell and digest some membrane proteins that may interfere with the recognition of male and female gametes (Leduc et al., Reference Leduc, Matthys-Rochon and Dumas1995). In this assay, about 18% of the egg cells (8–10/50 ovules) could be isolated in a 0.1% cellulase and 0.1% pectinase maceration solution. These isolated egg cells were followed by subsequent preparation of cDNA libraries in order to separate egg genes. The successful isolation of the male and female gametes provided the opportunity to study zygote formation outside the female gametophyte or embryo sac.
Isolation of the zygote is also an important new field in the study of plant developmental biology (Kumlehn et al., Reference Kumlehn, Lörz and Kranz1999). With the successful isolation of egg cells and zygotes, comparisons of cDNA libraries between egg and zygote can be used to isolate specific genes of the egg cell and zygote, which may shed light on the mechanisms of egg activation. Dresselhaus et al. (Reference Dresselhaus, Hagel, Lörz and Kranz1996) first constructed two cDNA libraries from 128 maize egg cells and another library from 104 maize zygotes generated by in vitro fertilization (18 h after in vitro fertilization), to isolate newly expressed genes in zygotes or genes expressed more abundantly after fertilization. Plant zygotes exhibit a high degree of vigor in vivo and a higher rate of cell division than somatic cells. The isolated in vitro and in vivo zygotes divided easily and essentially underwent embryogenesis to produce fertile plants when cultured (Kranz & Lörz, Reference Kranz and Löra1993; Holm et al., Reference Holm, Knudsen, Mourtzen, Negri, Olsen and Roué1994; Leduc et al., Reference Leduc, Mattys-Rochon, Rougier, Mogensen, Holm, Magnard and Dumas1996; Kumlehn et al., Reference Kumlehn, Brettschneider, Lörz and Kranz1997, Reference Kumlehn, Lörz and Kranz1998). Then, successful isolation and culture of zygotes provides the opportunity for using zygotes as recipient cells in the transformation of higher plants, which may improve transformation frequency (Wang et al., Reference Wang, Kuang, Russell and Tian2006).
The electrophoretic analysis of isolated egg cells and zygotes of T. fournieri indicated that the surfaces of both were positively charged, with an average electrophoretic mobility of 1.078 ± 0.020 μm/s and 1.379 ± 0.023 μm/s, respectively. The significance, if any, of the surface charge difference between egg cell and zygote is not clear. The more rapid movement of the fertilized egg cell (zygote) in an electric field under the conditions of this study is probably caused by the higher surface charge. Whether this surface charge increase was associated directly with the sperm, some other influence or a combination of factors, is unknown. Further research to explore the cause and consequence of this alteration in charge may further elucidate the intricate reproductive mechanisms of higher plants.
Acknowledgement
This work was supported by a grant from the National Natural Science Foundation of China (No. 30670126).