Introduction
Nitric oxide (NO) is a gaseous free radical involved in many physiological processes in mammals, and is synthesized from cofactors and the oxidation of l-arginine, which results in l-citrulline and NO production (Ignarro et al., Reference Ignarro, Cirino, Casini and Napoli1999, Reference Ignarro, Buga, Wood, Byrns and Chaudhuri1987). The synthesis of NO is catalyzed by the enzyme nitric oxide synthase (NOS; Basini & Grasselli, Reference Basini and Grasselli2015), which has been detected in ovaries, oocytes and embryos (Tesfaye et al., Reference Tesfaye, Kadanga and Bauch2006, Pires et al., Reference Pires, Santos, Adona, Natori, Schwarz, De Bem and Leal2009).
NO has been shown to influence oocyte maturation and effects may be stimulatory or inhibitory depending on its concentration (Bu et al., Reference Bu, Xie, Tao, Wang and Xia2004, Reference Bu, Xia, Tao, Lei and Zhou2003). Low concentrations stimulate meiosis while high concentrations are inhibitory and such an effect has been described previously in rats (Jablonka-Shariff & Olson, Reference Jablonka-Shariff and Olson1998; Jablonka-Shariff et al., Reference Jablonka-Shariff, Basuray and Olson1999), mice (Bu et al., Reference Bu, Xie, Tao, Wang and Xia2004), pigs (Tao et al., Reference Tao, Fu, Zhang, Xia, Yang and Xie2004) and cattle oocytes (Bilodeau-Goeseels, Reference Bilodeau-Goeseels2007).
NO mainly acts by activating soluble guanylate cyclase (sGC), which synthesizes cyclic guanosine monophosphate (cGMP). Increased cGMP activates cGMP-dependent protein kinase (PKG) and/or affects the activity of different phosphodiesterases (PDE) (Denninger & Marletta, Reference Denninger and Marletta1999). PDEs are enzymes that hydrolyze cGMP and/or cAMP (dual-specifity PDEs 1/2/3/10/11, cAMP-specific PDEs 4/7/8 and cGMP-specific PDEs 5/6/9, reviwed by Francis et al., Reference Francis, Blount and Corbin2011). Increased cGMP levels may inhibit PDE3, resulting in elevated cAMP levels, demonstrating the cross-talk between the two nucleotide pathways (Francis et al., Reference Francis, Blount and Corbin2011).
High levels of cAMP contribute towards meiosis arrest in immature germinal vescicle stage oocytes (GV), while its decrease leads to meiosis resumption (Eppig & Downs, Reference Eppig and Downs1984). Cyclic GMP has also been shown to participate in meiosis resumption as its decrease is observed during this period (Törnell et al., Reference Törnell, Billig and Hillensjö1990). Therefore, PDEs are involved in oocyte maturation by decreasing the high levels of cAMP in immature oocytes in order to permit meiosis resumption (Sadler & Maller, Reference Sadler and Maller1989).
Different PDEs are expressed in oocytes and cumulus cells, and can therefore influence levels of nucleotides and meiosis resumption. PDE3, the dual-specific enzyme that is influenced by cGMP levels, is mostly active in oocytes (Sasseville et al., Reference Sasseville, Albuz, Côté, Guillemette, Gilchrist and Richard2009). In cumulus cells, the most active cAMP-specific enzyme is PDE8 (Sasseville et al., Reference Sasseville, Albuz, Côté, Guillemette, Gilchrist and Richard2009). Cyclic GMP-specific PDEs are less characterized, but PDE5 was shown to be expressed in oocytes and cumulus cells in mice (Wang et al., Reference Wang, Ning, Chen, Yang, Ouyang, Zhang, Tai, Mu, Zhou, Zhang and Xia2008), pigs (Sasseville et al., Reference Sasseville, Côté, Gagnon and Richard2008) and cattle (Schwarz et al., Reference Schwarz, Pires, Mesquita, Chiaratti and Leal2014).
The aim of the present study was to assess the effect of increased levels of NO by S-nitroso-N-acetylpenicillamine (SNAP) on meiotic resumption and on cGMP and cAMP levels. In addition, the effects of associating SNAP and different PDE inhibitors (cilostamide for dual-specifity PDE3, sildenafil for cGMP-specific PDE5 and dipyridamole for cAMP-specific PDE7 and PDE8), on meiosis resumption and embryo development were evaluated as well.
Materials and Methods
All chemicals used in this study were purchased from Sigma-Aldrich (USA) unless otherwise indicated.
Oocyte collection
Ovaries were collected at a commercial abattoir immediately after slaughter and transported in sterile saline solution at 30°C. In the laboratory, 3–8 mm follicles were aspirated. The aspirated follicular fluid (FF) was placed in 50 ml conical tubes and maintained for 10 min for sedimentation. The upper portion of the liquid was removed and centrifuged at 1800 g for 5 min. The supernatant (5 ml) was transferred to a Petri dish (100 mm) and 1 ml of the sediment from the aspirated FF was added. Cumulus–oocyte complexes (COCs) were selected in FF (Thomas et al., Reference Thomas, Thompson, Armstrong and Gilchrist2004a), only COCs grades I and II were used for maturation (de Loos et al., Reference de Loos, Kastrop, Van Maurik, Van Beneden and Kruip1991). Additionally, care was taken to select COCs with similar sizes of cumulus investments for all treatments (Bilodeau-Goeseels, Reference Bilodeau-Goeseels2007) to decrease variation due to COC size in the NO production and cGMP levels measurements.
In vitro maturation
For IVM, the selected COCs were cultured in defined maturation medium (TCM199 with Earle's salts and 20 mmol/l sodium bicarbonate (Gibco), 0.25 mmol/l sodium pyruvate, 0.1% polyvinyl alcohol and 10 µg/ml gentamicin). Maturation was carried out in 100-µl droplets overlaid with mineral oil and incubated at 38.5°C, in 5% CO2 in air, and maximum humidity for different periods of time according to the experiment. Gonadotropins were not added to maturation medium in order not to interfere with cyclic nucleotides levels (Izadyar et al. Reference Izadyar, Zeinstra and Bevers1998), as we aimed to assess the exclusive effects of nucleotide modulators.
Nitric oxide concentration (Griess method)
Nitric oxide levels were measured indirectly by quantifying the concentration of one of its stable metabolites, nitrite concentration in maturation media by the Griess method. In vitro maturation media from control and SNAP treatment groups were collected at the end of culture (9 h IVM) and stored at −20°C until subjected to testing. Assays were performed using the Griess Reagent System kit (Promega, Madison, WI, USA) following the manufacturer's instructions. The reading of absorbance was 540 nM (Multiskan™ FC Microplate Photometer, Thermo Scientific, Vantaa, Finland). To determine the nitrite concentrations in samples, a standard curve was performed with the experimental samples for which they were calculated by standard curve equation.
Cyclic GMP and AMP measurements
Cyclic GMP and AMP levels were measured by enzyme immunoassay. Pools of 40 and 10 COCs were used for cGMP and cAMP measurements, respectively. Briefly, COCs were removed from culture and washed rapidly in Ca2+/Mg2+-free phosphate-bufffered saline (PBS) and transferred to 200 µl of 0.1 N HCl for 20 min to lyse the cells. After this period, the samples were centrifuged at 12,000 g for 5 min. The supernatant was transferred to new tubes and stored at −20°C until subjected to enzymatic immunoassay with acetylation protocol according to the instructions of the kits (Direct Cyclic GMP enzyme immunoassay method; Enzo Life Sciences, Ann Arbor, MI, USA).
Assessment of meiotic resumption
In order to visualize the nuclear envelope and determine meiosis resumption, COCs were transferred into 5-ml tubes containing 200 µl PBS and vortexed for 5 min to remove cumulus cells. Denuded oocytes were placed between a glass slide and a coverslip and fixed (ethanol:acetic acid, 3:1) for at least 24 h. The oocytes were then stained with 1% aceto-orcein and evaluated under a light microscope to examine nuclear morphology. Oocytes with an intact nuclear envelope were considered in germinal vesicle (GV) stage (immature) and those without a visible nuclear envelope were considered as having resumed meiosis [germinal vesicle breakdown (GVBD)] (Lodde et al., Reference Lodde, Modina, Galbusera, Franciosi and Luciano2007).
Assessment of meiotic progression (maturation rates)
Oocytes were denuded from cumulus cells as described previously, incubated for 10 min in the dark in PBS containing 1 μg/ml Hoechst 33342 and placed between a glass slide and a coverslip. Chromatin configuration was evaluated under an inverted epifluorescence microscope (Zeiss Axiovert 40. Carl Zeiss Optical, Inc., Chester, VA, USA). Oocytes with a metaphase plate and a polar body were classified as metaphase II (MII) and considered as matured.
In vitro embryo production
Matured oocytes were transferred to drops (25 oocytes/drop) containing 90 µl fertilization medium and covered with mineral oil. Oocytes were subjected to in vitro fertilization (IVF) using frozen semen of the same bull and of proven fertility. Spermatozoa were selected using the Percoll method, and the concentration was adjusted to 2 × 106 sperm cells/ml. Fertilization occurred in TALP medium (Parrish, Reference Parrish2014), supplemented with 5 mg/ml BSA (fatty acid-free), 0.2 mM/ml pyruvate, 30 µg/ml heparin (Sudano et al., Reference Sudano, Paschoal, da Silva Rascado, Magalhães, Crocomo, de Lima-Neto and da Cruz Landim-Alvarenga2011), 18 µm/ml penicilamine, 10 µm/ml hypotaurine, 1.8 µm/ml epinephrine, 100 µg/ml streptomycin sulfate and 100 IU/ml penicillin (Gibco-BRL, Grand Island, NY, USA). Oocytes and spermatozoa were co-incubated under the same conditions as the in vitro maturation procedure for 20 h. The day of fertilization was defined as Day 0 (D0). Presumptive zygotes were denuded by repeated pipetting 20 h after IVF and transferred to culture plates and placed into drops containing 90 µl culture medium (25 presumptive zygotes per drop), covered with mineral oil and cultured under the same conditions as for in vitro maturation. The culture medium was synthetic oviduct fluid with amino acids (SOFaa, Holm et al., Reference Holm, Booth, Schmidt, Greve and Callesen1999) supplemented with 2.7 mm/ml myo-inositol, 0.2 mm/ml pyruvate, 2.5% fetal calf serum (FCS; v/v), 5 mg/ml BSA (fatty acid-free), 100 µg/ml streptomycin sulfate and 100 IU/ml penicillin (Gibco). After 48 h of culture, cleavage was checked and structures that were not cleaved were discarded. The embryos remained in culture until Day 8 (D8). Blastocyst production was evaluated on D7 and D8 and hatching on D8.
Experiments
Experiment I – Influence of SNAP on meiosis resumption and levels of NO and cyclic nucleotides
Influence of SNAP through stimulation of sGC on meiosis resumption and cyclic nucleotides levels were assessed in this experiment. COCs were matured in vitro for 9 h (time of GVBD) in the following treatments: (1) control (no drugs); or (2) 0.1 µM SNAP (Schwarz et al., Reference Schwarz, Pires, de Bem, Adona and Leal2010) and 0.1 µM SNAP associated with sGC inhibitor 1H-[1,2,4]oxadiazolo[4,3-a]quinoxalin-1-one (10 µM ODQ, Bilodeau-Goeseels, Reference Bilodeau-Goeseels2007). To validate the action of SNAP as nitric oxide donor, the culture media from control and SNAP treatments were collected at the end of culture to assess nitric oxide levels by the Griess method. COCs from the three treatment groups were collected at 9 h IVM to determine meiotic resumption rates and for assessments of cGMP and cAMP levels samples were collected at 0 h and 1 h of culture. As PDE3, which is the dual-specific PDE isoform prevalent in the oocyte can be inhibited by increased cGMP and thereby raise cAMP levels, we also determined, in independent experiments, the cAMP levels in the same treatments and timepoints as cGMP. For this experiment 250 oocytes/treatment were analysed in four or five replicates.
Experiment II – Effect of SNAP associated with PDEs inhibitors on meiosis resumption
In this experiment we evaluated the influence of SNAP associated with inhibition of different phosphodiesterases (PDE5 inhibitor sildenafil, PDE3 inhibitor cilostamide and PDE7 and PDE8 inhibitor dipyridamole) to determine the most effective treatment in delaying of meiosis resumption. COCs were matured in: (1) control; (2) SNAP; (3) SNAP + sildenafil (10 µM sildenafil, Sasseville et al., Reference Sasseville, Côté, Gagnon and Richard2008); (4) SNAP + cilostamide (20µM cilostamide, Mayes and Sirard, Reference Mayes and Sirard2002); or (5) SNAP + dipyridamole (50 µM dipyridamole, Sasseville et al., Reference Sasseville, Albuz, Côté, Guillemette, Gilchrist and Richard2009). GVBD rates were determined at 9 h IVM. For this experiment 95–100 oocytes/treatment were analysed in five replicates.
Experiment III – Effect of SNAP associated with cilostamide on meiotic progression and embryo production
In this experiment the influence of SNAP associated or not with cilostamide on meiotic progression was evaluated at 24 h maturation. Extended maturation to 28 h was also examined as treatments had caused delayed meiosis resumption and could lead to delayed maturation progession to MII. Embryo development was evaluated after IVM for 28 h in the same goups and another one cultured with cilostamide alone. Controls were matured without drugs. Cleavage rate was assessed on D2, blastocyst rates on D7 and D8 and hatched blastocysts on D8. For this experiment 250–265 oocytes/treatment were analysed in four replicates.
Statistical analysis
Statistical analyses were performed using the SAS System (V 5.1; SAS Institute, Inc., Cary, NC, USA). Data were tested for normal distribution and homogeneity of variance and were transformed (arcsine or log) when these criteria were not met. Maturation and embryo development rates were analysed by one-way analysis of variance (ANOVA) followed by Tukey post hoc test. The effects of the treatments on NO, cGMP levels were analysed by two-way ANOVA followed by Tukey post hoc testing for NO and Bonferroni for nucleotides. Differences with probabilities of P < 0.05 were considered significant.
Results
Experiment I – Influence of SNAP on meiosis resumption and levels of NO and cyclic nucleotides
Nitric oxide levels observed in the control group were 40.7 ± 1.9 µM and in the group treated with SNAP levels raised to 47.3 ± 0.8 µM (P < 0.05), confirming that SNAP treatment was able to significantly increase the availability of NO measured as nitrite in the culture media (Fig. 1).

Figure 1 Nitrite concentrations in culture medium after 9 h of in vitro maturation for the control and SNAP (NO donor) treatments. a,bDifferent letters indicate significant difference between treatments (P < 0.05). Bars indicate standard error of the mean. Results from five replicates.
Regarding oocyte maturation, SNAP reduced the proportion of oocytes undergoing meiosis resumption compared with the control group (53.4 ± 1.2 and 78.4 ± 2.4%, respectively, P < 0.05, Fig. 2). Moreover, the association with ODQ reversed the effect of SNAP, as GVBD rates were higher (73.4 ± 6.3%, P < 0.05) and similar to controls (P > 0.05).

Figure 2 Proportion of oocytes in germinal vesicle (GV) and germinal vesicle breakdown stages (GVBD) after 9 h in vitro maturation with SNAP (NO donor) associated or not with ODQ (sGC inhibitor). a,b,y,zDifferent letters indicate significant difference between treatments (P < 0.05). Bars indicate standard error of the mean. Results are from four replicates.
When cGMP levels were evaluated (Fig. 3), immature COCs, used as reference for starting levels (0 h), showed highest amounts (4.36 ± 0.16 pmol/COC, P < 0.05). After 1 h, SNAP treatment had higher cGMP levels (3.94 ± 0.18 pmol/COC, P < 0.05) compared with controls (3.03 ± 0.24) and SNAP + ODQ (2.48 ± 0.13 pmol/COC), which had the lowest levels (P < 0.05).
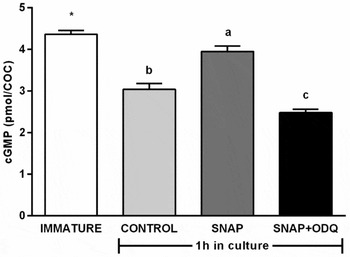
Figure 3 Levels of cGMP in bovine cumulus–oocyte complexes (COCs) matured in vitro for 0 h and 1 h in the presence of 0.1 µM SNAP (NO donor) alone or in combination with 10 µM ODQ (sGC inhibitor). a–cDifferent letters indicate significant difference between treatments (P < 0.05) *Difference between treatments and immature group (0 h) (P < 0.05). Bars indicate standard error of the mean. Results are from four replicates.
Regarding cAMP levels (Fig. 4), after 1 h in culture, all groups had lower levels than that in immature COCs (1,058 ± 62 fmol/COC, P < 0.05), and controls had highest levels (380 ± 42 fmol/COC, P < 0.05) among treatments. Cyclic AMP levels in SNAP were higher than in SNAP + ODQ (258 ± 14 and182 ± 29 fmol/COC, respectively, P < 0.05).

Figure 4 Levels of cAMP in bovine cumulus–oocyte complexes (COCs) matured in vitro for 0 h and 1 h in the presence of 0.1 µM SNAP alone or in combination with 10 µM ODQ. a–cDifferent letters indicate significant difference between time in treatment (P < 0.05). *Difference between treatments and immature group (0 h) (P < 0.05). Bars indicate standard error of the mean. Results from four replicates.
Experiment II – Effect of SNAP associated with PDEs inhibitors on meiosis resumption
As increasing cGMP levels did not result in increased cAMP levels, in this experiment the effects of associating SNAP with inhibitors of cAMP-specific PDE families were tested. Cilostamide was used to inhibit PDE3, the oocyte prevalent family, and dipiridamole was used to inhibit PDE7 and PDE8, the cumulus cells prevalent family. Association with cGMP-specific (PDE5) inhibitor (sildenafil) to avoid cGMP hydrolysis was evaluated as well. SNAP alone and no treatment (control) were also evaluated. COCs were assessed for meiotic resumption rates (Fig. 5).
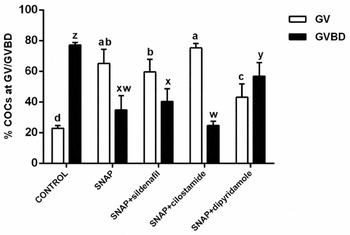
Figure 5 Proportion of oocytes in germinal vesicle (GV) and germinal vesicle breakdown stages (GVBD) after 9 h in vitro maturation with SNAP associated with inhibitors of different phosphodiesterase families. a–d,w–zDifferent letters indicate significant difference between treatments (P < 0.05). Bars indicate standard error of the mean. Results are from four replicates.
SNAP decreased GVBD (34.9 ± 9.2%, P < 0.05) compared with controls (77.1 ± 1.8%), delaying meiosis resumption. When SNAP was associated with PDE5 inhibitor (sildenafil), no further decrease in GVBD (40.4 ± 8.2, P > 0.05) was observed compared with SNAP alone. When the PDE3 inhibitor was included (cilostamide) with SNAP, even less oocytes resumed meiosis (24.7 ± 2.8% GVBD) compared with SNAP alone, although the difference was not significant (P > 0.05). Conversely, when SNAP was associated with dipiridamole (PDE7 and PDE8 inhibitor), GVBD was still reduced when compared with controls (56.9 ± 8.7%, P < 0.05), but the efficiency of the nitric oxide donor in delaying the resumption of meiosis was lower.
Experiment III – Effect of SNAP associated with cilostamide on meiotic progression and embryo production
As SNAP and its association with the PDE3 inhibitor cilostamide were the treatments that mostly delayed meiosis resumption, these treatments were evaluated for meiosis progression to metaphase II (MII) at 24 and 28 h IVM (Table 1). At 24 h IVM, most oocytes were at MII stage of meiosis, but the control and SNAP groups had similar proportions of mature oocytes (69.8 ± 5.5 and 67.1 ± 7.4%, P > 0.05), while SNAP + cilostamide had lower MII rates (50.0 ± 2.0%, P < 0.05), suggesting that meiosis progression kinetics was slower in this group. After extended IVM culture up to 28 h, however, all groups had similar MII rates (66.6 to 71.4%, P > 0.05).
Table 1 Nuclear maturation rates after 24 and 28 h IVM culture with SNAP alone or associated with cilostamide

a,b Different letters within the same column indicate significant difference between treatments (P < 0.05). Results are from three replicates.
As association of cilostamide with SNAP slowed meiotic progression, in vitro embryo development was also evaluated after IVM for 28 h, and the same groups were evaluated plus an additional one with cilostamide alone (Table 2). Cleavage rates were lower in the SNAP + cilostamide association (55.1 ± 7.6%, P < 0.05) compared to all other treatments, which were similar to each other (72–74%, P > 0.05). Blastocyst rates on D7 were similar for control, SNAP and cilostamide (38.7 ± 5.8, 37.9 ± 6.2 and 40.5 ± 5.8%, P > 0.05), but lower for SNAP + cilostamide (25.7 ± 6.9%, P < 0.05). A similar trend was observed for D8 blastocyst and hatching rates, for which SNAP + cilostamide had the lowest rates for both parameters (P < 0.05).
Table 2 Embryo development rates from oocytes fertilized in vitro afer 28 h IVM in the presence of SNAP, cilostamide or both

a,b Different letters within the same column indicate significant difference between treatments (P < 0.05). Results are from four replicates.
Discussion
In the present study, bovine COCs subjected to IVM with increased availability of nitric oxide, by using a NO donor (SNAP), showed decreased meiotic resumption and increased cGMP levels. This effect was mediated through activation of sGC, which is one of the common pathways for NO (Potter, Reference Potter2011). These results confirm previous observations that NO donors SNAP (Schwarz et al., Reference Schwarz, Pires, Adona, Camara de Bem and Leal2008, Reference Schwarz, Pires, Mesquita, Chiaratti and Leal2014) and SNP (Viana et al., Reference Viana, Caldas-Bussiere, Matta, Faes, de Carvalho and Quirino2007) decrease meiotic resumption rates and increase cGMP levels (Schwarz et al., Reference Schwarz, Pires, Mesquita, Chiaratti and Leal2014), and also confirm that the treatment with SNAP was effective in increasing NO levels, as nitrite metabolites were higher in this group. The NO/sGC/cGMP pathway has been shown to be functional in rodent oocytes (Nakamura, Reference Nakamura2002; Sela-Abramovich et al., Reference Sela-Abramovich, Galiani, Nevo and Dekel2008) and present findings give further envidence that it is also functional in bovine COCs, contributing, at least in part, in the regulation of meiotic resumption.
In rodents, cGMP has been shown to inhibit PDE3, thus preventing cAMP hydrolysis and maintaining oocytes in the GV stage (Norris et al., Reference Norris, Ratza, Freudzon, Mehlmann, Krall, Movsesian, Wang, Ke, Nikolaev and Jaffe2009). When cAMP levels were measured in bovine COCs they declined sharply in all groups, suggesting that the increased cGMP levels that delay meiosis resumption, appeared not to inhibit PDE3 under the conditions studied. This lack of increase in cAMP after treatments that would increase cGMP was also reported in other studies (Bilodeau-Goeseels, Reference Bilodeau-Goeseels2007; Schwarz et al., Reference Schwarz, Pires, Mesquita, Chiaratti and Leal2014). Dubeibe et al. (Reference Dubeibe, Caldas-Bussiere, Maciel, Sampaio, Quirino, Gonçalves, De Cesaro, Faes and Paes de Carvalho2017) also showed that nucleotides had opposite and not parallel levels in bovine COCs. Using a different strategy, they reported that COCs matured in the presence of follicle walls with or without l-arginine, the precursor for NO synthesis, cGMP levels declined, while cAMP levels increased. Therefore, the signaling pathway downstream from cGMP in cattle differs from that in rodents, as suggested by Bilodeau-Goeseels (Reference Bilodeau-Goeseels2007), and other pathways may be acting. Cyclic GMP may activate or inhibit other phophodiesterases and activate PKG (Francis et al., Reference Francis, Busch and Corbin2010). Activated PKG may inhibit sGC, leading to a negative feedback loop that decreases cGMP levels (Murthy, Reference Murthy2001). This, relieving the block on PDE activity, which will hydrolyze cAMP. However, little information is known regarding PKG in COCs, but its transcripts have been detected in bovine oocytes and cumulus cells (Schwarz et al., Reference Schwarz, Pires, Mesquita, Chiaratti and Leal2014) and in mice (Sriraman et al., Reference Sriraman, Rudd, Lohmann, Mulders and Richards2006), so this possibility remains an open question.
Recently, PDE5 mRNA was detected in bovine oocytes and cumulus cells (Schwarz et al., Reference Schwarz, Pires, Mesquita, Chiaratti and Leal2014). As SNAP alone attenuated the decline in cGMP levels during the first hour of IVM, but did not maintain levels similar to that in immature oocytes, it is possible that PDE5 could reduce cGMP produced by sGCs. PDE5 is also activated by increased cGMP levels, and participates in the negative feedback loop for this nucleotide (Francis et al., Reference Francis, Busch and Corbin2010). This event could because SNAP only delays meiotic resumption in part of the oocytes.
In addition to cGMP, the nucleotide cAMP is involved in maintenance of meiotic block (Eppig & Downs, Reference Eppig and Downs1984) and was not increased by the treatments evaluated. Therefore, the association of SNAP with specific phosphodiesterase inhibitors to avoid nucleotide hydrolysis and sustain their levels in COCs was also tested on meiotic resumption.
In this study, the tested associations did not show much difference on meiotic resumption when compared with SNAP alone, although all associations delayed GVBD when compared with untreated controls. The PDE3 inhibitor (cilostamide) showed the lowest rate of meiotic resumption, but was still similar to SNAP. In addition, PDE5 inhibitors (sildenafil) and PDE7 and PDE8 inhibitors (dipirydamole) did not improve the efficiency of SNAP alone. Therefore, it seems that the combination of increased cGMP by stimulating production (SNAP) and inhibiting hydrolysis (sildenafil), as well as increasing both cGMP (SNAP) and cAMP (PDE3 inhibition by cilostamide and PDE7/PDE8 inhibition by dipyridamole), does not result in further delay in meiotic resumption.
Few studies have been carried out on the functions of PDEs in bovine oocytes. Regarding cAMP-specific PDE8, Sasseville et al. (Reference Sasseville, Albuz, Côté, Guillemette, Gilchrist and Richard2009) reported that bovine oocytes cultured for 9 h with a PDE7/PDE8 inhibitor (dipyridamole) had lower meiotic resumption rates, agreeing with results in this study. PDE8 is the most active cAMP-specific PDE in cumulus cells (Sasseville et al., Reference Sasseville, Albuz, Côté, Guillemette, Gilchrist and Richard2009), but its inhibition in association with SNAP did not increase GV rates. Conversely, when COCs were matured with SNAP and a PDE3 inhibitor (cilostamide), the lowest rates of meiosis resumption were observed. Although not different from SNAP alone, these rates were different from the association with the inhibitor for the cAMP-specific PDE (dipyridamole for PDE7 and PDE8). PDE3 is the most active cAMP-specific PDE in oocytes (Sasseville et al., Reference Sasseville, Albuz, Côté, Guillemette, Gilchrist and Richard2009). When we compared the results for inhibiting PDE8 with that for PDE3 in association with SNAP, it appears that inhibition of cAMP degradation within the oocyte is more effective than within cumulus cells, possibly because gap junctions could be closed and changes in cumulus cells would not affect the oocyte (Thomas et al., Reference Thomas, Armstrong and Gilchrist2004b). Based on our results, there seems to be a slight effect of avoiding cGMP decrease (SNAP) and inhibiting cAMP hydrolysis by PDE3 in oocytes on maintaining them in the GV stage.
Association of SNAP and sildenafil to avoid cGMP hydrolysis by PDE5 resulted in GVBD rates similar to those of SNAP alone. Therefore, inhibition of cGMP hydrolysis (PDE5 inhibition) together with stimulation of its synthesis (sGC stimulation by NO) did not show any additional effect in maintaining meiotic block. To our knowledge there have been no other similar studies on this aspect, except from Hanna et al. (Reference Hanna, Yao, Wu and Jensen2012) who associated a cGMP analogue and a PDE9 inhibitor (another cGMP-hydrolizing PDE), and observed increased GV arrest in mice oocytes. Possibly other cGMP-degrading enzymes (such as PDE9; Francis et al., Reference Francis, Blount and Corbin2011) hydrolyze the nucleotide, leading to meiosis resumption in part of the oocytes.
PDE3 inhibitors during IVM were shown to delay spontaneous nuclear maturation in mice (Nogueira et al., Reference Nogueira, Cortvrindt, De Matos, Vanhoutte and Smitz2003; Masciarelli et al., Reference Masciarelli, Horner, Liu, Park, Hinckley, Hockman, Nedachi, Jin, Conti and Manganiello2004), rats (Tsafriri et al., Reference Tsafriri, Chun, Zhang, Hsueh and Conti1996), pigs (Dieci et al., Reference Dieci, Lodde, Franciosi, Lagutina, Tessaro, Modina, Albertini, Lazzari, Galli and Luciano2013), sheep (Gharibi et al., Reference Gharibi, Hajian, Ostadhosseini, Hosseini, Forouzanfar and Nasr-Esfahani2013), cattle (Mayes & Sirard, Reference Mayes and Sirard2002; Thomas et al., Reference Thomas, Thompson, Armstrong and Gilchrist2004a) and humans (Shu et al., Reference Shu, Zeng, Ren, Zhuang, Liang, Shen, Yao, Ke and Wang2008; Vanhoutte et al., Reference Vanhoutte, De Sutter, Nogueira, Gerris, Dhont and Van der Elst2007). In some studies, delay in spontaneous nuclear maturation improved embryo development (Luciano et al., Reference Luciano, Pocar, Milanesi, Modina, Rieger, Lauria and Gandolfi1999; Thomas et al., Reference Thomas, Thompson, Armstrong and Gilchrist2004a; Albuz et al., Reference Albuz, Sasseville, Lane, Armstrong, Thompson and Gilchrist2010; Dieci et al., Reference Dieci, Lodde, Franciosi, Lagutina, Tessaro, Modina, Albertini, Lazzari, Galli and Luciano2013).
SNAP alone or combined with cilostamide were similarly efficient in delaying meiotic resumption, so the effect of such treatments was tested on embryo development. As meiotic resumption was lower in these groups, progression to metaphase II was evaluated prior to in vitro fertilization to determine the necessity of extended maturation. SNAP with cilostamide had the slowest progression and at 24 h only half of the oocytes reached MII compared with the control group. SNAP was similar to controls, so while it delays meiotic resumption, it seems not to have lasting effects on meiotic progression. Similar delay in meiotic progression using cilostamide has been reported previously (Thomas et al., Reference Thomas, Armstrong and Gilchrist2002). After 28 h, all groups were similar in terms of MII rates, so embryo development after such treatments was assessed.
For embryo development for control, SNAP and cilostamide were all similar in terms of cleavage, blastocysts on D7 and D8, and also hatching rates. However, when both drugs were associated during IVM all embryo development parameters were lower. Oocyte aging could occur after the extended maturation, but this appears not to be the case, as this would probably affect the control group, which had developmental rates similar to cilostamide and SNAP groups. As maturation for SNAP + cilostamide was still lower at 28 h, although not significantly, maybe an even longer maturation period for this group would be necessary, as only 67% of oocytes reached MII at this time. Thomas et al. (Reference Thomas, Thompson, Armstrong and Gilchrist2004a), using a different PDE3 inhibitor (milrinone) with FSH, observed improved embryo production when performing fertilization after 28 h IVM. Albuz et al. (Reference Albuz, Sasseville, Lane, Armstrong, Thompson and Gilchrist2010) reported using up to 30 h IVM before fertilization under conditions of delayed meiotic progression, which improved embryo development. However, the possibility that such extended maturation under the culture conditions used may have caused deleterious effects on oocyte quality that were not observed in terms of MII rates, but became only evident after fertilization, cannot be ruled out and needs further investigation.
According to the results obtained in the present study, it was confirmed that NO sustains cGMP levels due to sCG activation, which is sufficient to delay meiotic resumption in bovine COCs, but not to increase cAMP levels through PDE3 inhibition. Combined stimulation of cGMP synthesis and inhibition of different PDE families to further increase nucleotides levels did not increase the delay in meiotic resumption, suggesting that other pathways are involved. Delaying spontaneous meiosis by combining SNAP and cilostamide decreases embryo development and may need an extended maturation time.
Conflict of interest
The authors have no conflict of interest to declare.
Acknowledgments
The authors thank FAPESP for funding this work (#2010/18023–9) and CAPES (RCB; FCC) and FAPESP (KRLS; FGZ; MDC; HF) for the scholarships.