Introduction
The yellowtail tetra Astyanax altiparanae has recently been used as model organism for basic and applied studies such as early embryology (Pereira-Santos et al., Reference Pereira-Santos, Yasui, Xavier, de Macedo Adamov, do Nascimento, Fujimoto, Senhorini and Nakaghi2016) and chromosome set manipulation (Adamov et al., Reference Adamov, Nascimento, Maciel, Pereira-Santos, Senhorini, Calado, Evangelista, Nakaghi, Guerrero, Fujimoto and Yasui2017; Nascimento et al., Reference Nascimento, Pereira-Santos, Piva, Manzini, Fujimoto, Senhorini, Yasui and Nakaghi2017b; Piva et al., Reference Piva, de Siqueira-Silva, Goes, Fujimoto, Saito, Dragone, Senhorini, Porto-Foresti, Ferraz and Yasui2018). This small characin (8–14 cm SL) can breed throughout the year, is resistant to suboptimal environmental conditions, presents rapid growth and reaches sexual maturity at approximately 4 months (Garutti, Reference Garutti2003; Porto-Foresti et al., Reference Porto-Foresti, Castilho-Almeida, Senhorini and Foresti2010), all of which facilitate its use for laboratory conditions. In this species, studies on the production of triploids were conducted by our group. A protocol for in vitro fertilization was established previously that permits control of timing of fertilization (Yasui et al., Reference Yasui, Senhorini, Shimoda, Pereira-Santos, Nakaghi, Fujimoto, Arias-Rodriguez and Silva2015). The timing for extrusion of the second polar body has also been evaluated by Pereira-Santos and colleagues (2016), supporting high percentages of produced triploids (Adamov et al., Reference Adamov, Nascimento, Maciel, Pereira-Santos, Senhorini, Calado, Evangelista, Nakaghi, Guerrero, Fujimoto and Yasui2017). First feeding within triploids has also been established for laboratory studies, permitting the evaluation of triploids in adult stages. In addition, evaluation of germ cells (Nascimento et al., Reference Nascimento, de Siqueira-Silva, Pereira-Santos, Fujimoto, Senhorini, Nakaghi and Yasui2017a), performance of triploids (Nascimento et al., Reference Nascimento, Pereira-Santos, Piva, Manzini, Fujimoto, Senhorini, Yasui and Nakaghi2017b) and hybrid triploids (Piva et al., Reference Piva, de Siqueira-Silva, Goes, Fujimoto, Saito, Dragone, Senhorini, Porto-Foresti, Ferraz and Yasui2018) have all been examined previously. However, in the genus Astyanax, some spontaneous triploids may have arisen under natural conditions (Centofante et al., Reference Centofante, Bertollo and Moreira-Filho2001), this event was generally linked with thermal shock in the environment or production of unreduced gametes during interspecies hybridization (Alves et al., Reference Alves, Coelho and Collares-Pereira2001). The origin of the natural triploids was not clearly established, but this phenomenon may affect studies of induced triploidization as increase in the number of triploids may cause a change in triploid percentages.
Some studies have also associated ageing of oocytes with problems in gametes, such as aneuploidy and production of triploidy (Aegerter and Jalabert, Reference Aegerter and Jalabert2004; Flajšhans et al., Reference Flajšhans, Kohlmann and Rab2007). Oocyte ageing can be defined as the time between ovulation and fertilization and occurs in vivo or in vitro (Mohagheghi Samarin et al., Reference Mohagheghi Samarin, Policar and Lahnsteiner2015). After ovulation, oocytes retain their capacity for fertilization for lengths of time that vary depending on fish species and temperature (Rizzo et al., Reference Rizzo, Godinho and Sato2003; Mohagheghi Samarin et al., Reference Mohagheghi Samarin, Policar and Lahnsteiner2015). As oocyte ageing occurs, a reduction in survival rate (Mohagheghi Samarin et al., Reference Mohagheghi Samarin, Policar and Lahnsteiner2015) and increased abnormal larvae are described (Sohrabnezhad et al., Reference Sohrabnezhad, Kalbassi, Nazari and Bahmani2006). This process of ovulation without spawning can lead to oocyte ‘over-ripening’, which is responsible for morphological, physiological, hormonal and molecular alterations and is considered to be the main factor affecting survival of early embryos (Mohagheghi Samarin et al., Reference Mohagheghi Samarin, Policar and Lahnsteiner2015).
In our previous study with A. altiparanae, we found that in vitro storage of oocytes may increase the percentage of triploids without any of the treatments commonly used to induce them such as temperature, pressure or chemical procedures (Pereira-Santos et al., Reference Pereira-Santos, Nascimento, Yasui, Pereira, Fujimoto, Senhorini and Nakaghi2018). This finding indicated that ageing of ovulated oocytes may give rise to triploids. If such a phenomenon also occurred in vivo, this may be a good explanation for the rise of natural triploids in Astyanax. Therefore, the aim of this study was to analyze the effect of in vivo storage of oocytes on early survival in A. altiparanae and measure its ploidy status.
Materials and methods
Ethics
This study was performed in accordance with the Care and Use of Laboratory Animals of the National Research and Conservation of Continental Fish (CEUA/CEPTA #02031.000033/2015-11).
Broodstock induction
Three adult couples of A. altiparanae were used in this study. The fish were previously maintained in 1000 m2 ponds at the National Center for Research and Conservation of Continental Fish/Chico Mendes Institute of Biodiversity Conservation (CEPTA/ICMBio) in Pirassununga City, São Paulo State, Brazil. Procedures for artificial fertilization were performed according to Yasui et al. (Reference Yasui, Senhorini, Shimoda, Pereira-Santos, Nakaghi, Fujimoto, Arias-Rodriguez and Silva2015) and Nascimento et al. (Reference Nascimento, Pereira-Santos, Piva, Manzini, Fujimoto, Senhorini, Yasui and Nakaghi2017b). The fish were anesthetized in eugenol solution (100 mg l–1) and hormonally induced with a single injection of pituitary gland from carp fish (3 mg kg−1 body weight). At 8 h after induction, reproduction behaviour was observed, in which the males started following the females, the fish were then separated for gamete collection. Males were anesthetized as describe above and sperm collected using a micropipette (Eppendorf, Hamburg, Germany) and immediately transferred to 1.5 ml tubes containing 400 µl of modified Ringer’s solution (NaCl 128.3 mM, KCl 23.6 mM, CaCl2 3.6 mM, MgCl2 2.1 mM) and maintained refrigerated (4°C) for later use. Only males with sperm motility higher than 80% were used. Females were immediately separated for fertilization trials.
Fertilization trials
During fertilization trials, females were individually maintained in a covered aquarium (40 l) with the temperature set at 26°C and under constant aeration. Samples of oocytes were stripped on a 90-mm Petri dish covered with a polyvinylidene chloride film (SaranWrap) and fertilized immediately after ovulation (positive control group) and after 60, 120, 180 or 240 min. Every time, females were anesthetized and 20 µl of oocytes (~150 eggs) were inseminated with 30 µl of diluted sperm (see above). Activation of gametes was performed by adding 5 ml of distilled water. These procedures were performed in triplicate with different couples. Development of embryos, and survival at the 2-cell, blastula, gastrula, and somite stages, as well hatching and subsequent normal and abnormal larvae, were observed using a stereomicroscope (Nikon SMZ 1500, Tokyo, Japan). Digital images were obtained using a charged coupled device (CCD) camera and Nis-Ar Elements software (Nikon, Tokyo, Japan).
Ploidy confirmation
At hatching, 20 larvae from each treatment group (n=60) were analyzed by flow cytometry. Samples were processed using the protocol developed by Xavier et al. (Reference Xavier, Senhorini, Pereira-Santos, Fujimoto, Shimoda, Silva, dos Santos and Yasui2017) using the nuclear stain 4,6-diamidino-2-phenylindole (DAPI) in a Partec CyFlow Ploidy Analyzer (Partec GmbH, Münster, Germany). All samples were analyzed using A. altiparanae spermatozoa from confirmed diploid males, and used as a reference for 1C DNA content.
Statistical analysis
Data were presented as mean±standard error (SE). Data were checked for normality and homogeneity using the Liliefors and Levene test (5%), respectively, and submitted to analysis of variance (ANOVA) followed by Tukey test. The software STATISTICA (Version 10.0, Statsoft, Tulsa, USA) was used and significance was set at a P-value<0.05.
Results
Early development and flow cytometry
In vivo storage of oocytes influenced the survival rates in all treatments (Table 1). No difference among treatments was observed in fish in the non-fertilized (P=0.7681), 2-cell (P = 0.7681) and blastula (P = 0.2459) stages (Table 1). However, except for the control group (Fig. 1A), some morphological alterations were observed at the blastula stage, mainly in the 180 min and 240 min groups. Cells with irregular patterns of cleavage were the most common malformations described (Fig. 1B, C). Treatments for 180 and 240 min presented significant reduction in survival rates at gastrula (P=0.0008), somite (P=0.0008) and hatching (P=0.0000) stages (Table 1). These cells did not present a regular pattern of cleavage and several morphological alterations were observed as irregular epiboly movements (Fig. 1E, F), which led to an increased number of abnormal larvae.
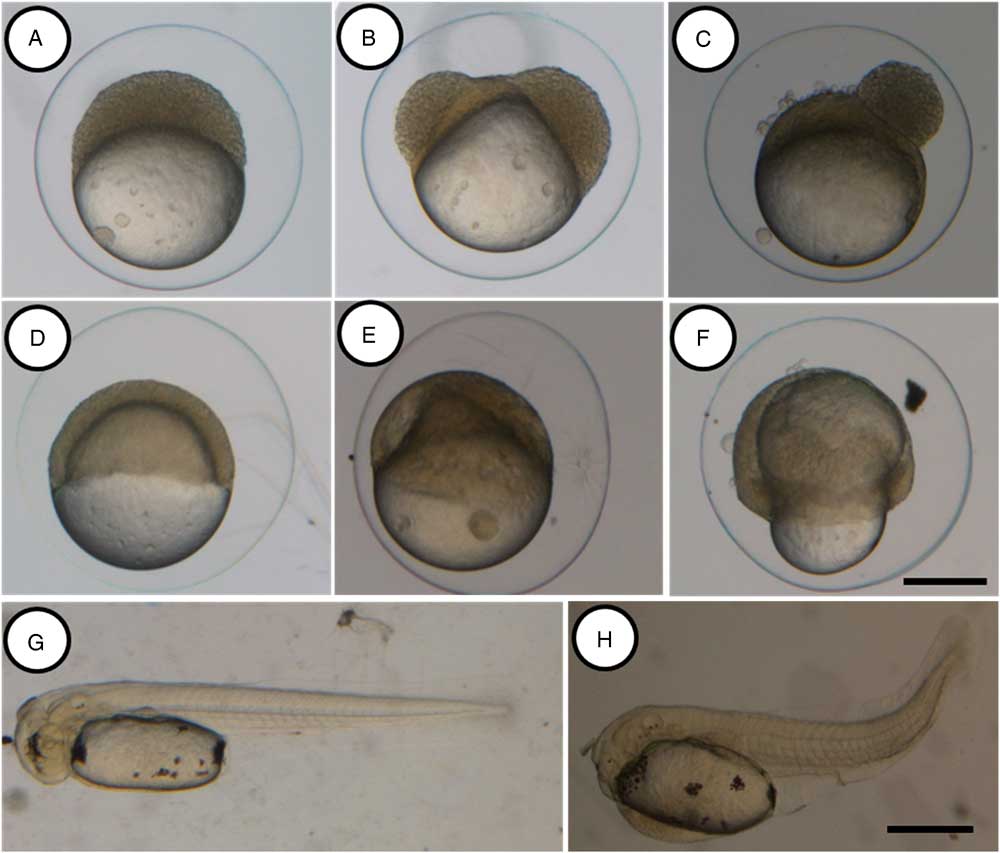
Figure 1 Morphological alterations during the early development of Astyanax altiparanae after in vivo storage of oocytes. Embryos from the control group at blastula (A), gastrula (D) and hatching (G) stages showed normal development, embryos after 180 and 240 min of storage showing irregular patterns of division at the blastula stage (B and C respectively) and irregular epiboly movements (E and F, respectively). Abnormal larvae after 240 min of storage (H). Scale: A–F, 200 µm (scale bar in F); G, H, 500 µm (scale bar in H).
Table 1 Survival rates (%) during development in Astyanax altiparanae after in vivo storage of oocytes
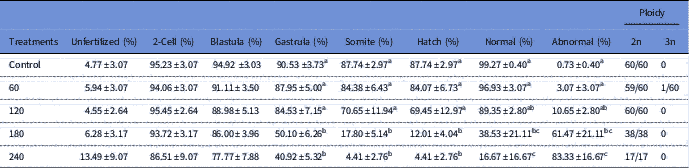
Data are show as mean ± standard error. Distinct superscript letters indicate significant difference (ANOVA, P < 0.05).
At hatching, the control group presented higher percentages (99.27±0.40%) of normal larvae (Fig. 1G) and just a few abnormal larvae (0.73±0.40%). This observation did not differ from that observed in the 60 min treatment group (P=0.9976). A significant increase in the percentage of larval abnormality was observed in the other treatment groups, and the 240 min treatment group presented the highest number of abnormal larvae (83.33±16.67%). Larvae malformations were mainly observed in the tail region (Fig. 1H), resulting in irregular swimming and consequent mortality.
Ploidy analysis
Ploidy analysis is detailed in Table 1. As expected, in the control group, all individuals were diploid (100%). After 60 min of storage, 0.6% triploid larvae were observed. However, in the other treatments, all samples were diploid (100%).
Discussion
In this study, we observed that in vivo storage of oocytes affected their survival and could influence the ploidy status of Astyanax altiparanae, giving rise to triploid fish. Similar results were observed under in vitro conditions for the same species (Pereira-Santos et al., Reference Pereira-Santos, Nascimento, Yasui, Pereira, Fujimoto, Senhorini and Nakaghi2018). In both studies, we observed a low percentage of triploid individuals and these results were similar to those observed by Fauaz et al. (Reference Fauaz, Vicente and Moreira-Filho1994) who detected one triploid fish from 64 individuals in Astyanax scabripinnis. For the same species, Maistro et al. (Reference Maistro, Lúcia Dias, Foresti, Oliveira and Filho1994) detected various frequencies of natural triploids, such as 1/86 and 1/40. However, Da Silva et al. (Reference Da Silva, Matoso, Ludwig, Gomes, Almeida, Vicari and Artoni2011) detected increased numbers of triploid individuals in Rhamdia quelen under natural conditions. As natural triploidy can vary with species and the local sampling environment (Tsuda et al., Reference Tsuda, Moraes, Giuliano-Caetano and Dias2010), we believe that the absence of triploidy in the other treatments could be due the random collection process, which means that if more samples were analyzed, more triploid fish would be obsereved. Additionally, as the temperature affects the triploid larval yields during oocyte ageing (Flajšhans et al., Reference Flajšhans, Kohlmann and Rab2007), different storage temperatures must be tested in the future for A. altiparanae.
The triploid individual observed in our study probably arose due to spontaneous retention of the second polar body, as observed in other studies. This affirmation is supported by Linhart et al. (Reference Linhart, Flajshans and Kvasnicka1995) who obtained spontaneous gynogenesis using overmaturated ova fertilized with irradiated spermatozoa (without any diploidization procedure). The authors generally stated that natural triploid fish may be produced by thermal shock in the environment (Centofante et al., Reference Centofante, Bertollo and Moreira-Filho2001). Based in our previous studies in the genus Asyanax (Adamov et al., Reference Adamov, Nascimento, Maciel, Pereira-Santos, Senhorini, Calado, Evangelista, Nakaghi, Guerrero, Fujimoto and Yasui2017; Nascimento et al., Reference Nascimento, de Siqueira-Silva, Pereira-Santos, Fujimoto, Senhorini, Nakaghi and Yasui2017a, Reference Nascimento, Pereira-Santos, Piva, Manzini, Fujimoto, Senhorini, Yasui and Nakaghi2017b; Piva et al., Reference Piva, de Siqueira-Silva, Goes, Fujimoto, Saito, Dragone, Senhorini, Porto-Foresti, Ferraz and Yasui2018), high temperatures (~40°C) and an adequate timing for heat shock are necessary to induce triploid individuals. This combination of conditions does not occur very often in nature, therefore we have excluded this explanation for the rise of natural triploids in the Astyanax.
Astyanax species are asynchronic intertidal spawners (Vazzoler, Reference Vazzoler1996; Mazzoni et al., Reference Mazzoni, Mendonça and Caramaschi2005; De Carvalho et al., Reference De Carvalho, Paschoalini, Santos, Rizzo and Bazzoli2009) in which groups of oocytes mature and then are fertilized by a male. During spawning, one or more males follow the female over a long period, and then groups of ovulated oocytes are released into the external medium and are immediately fertilized by the male. Fertilization is highly successful due to oocyte morphology, as described in our recent study (Pereira-Santos et al., Reference Pereira-Santos, Nascimento, Yasui, Pereira, Fujimoto, Senhorini and Nakaghi2018). We hypothesized that some of the ovulated oocytes may remain inside the ovary and become aged and fertilization of such oocytes may give rise to triploids. Another explanation for the increase in the number of triploids could be the production of unreduced gametes due interspecies hybridization (Alves et al., Reference Alves, Coelho and Collares-Pereira2001; Fujimoto et al., Reference Fujimoto, Yasui, Yoshikawa, Yamaha and Arai2008; Arias-Rodriguez et al., Reference Arias-Rodriguez, Yasui and Arai2009); this aspect may be investigated for Astyanax in future studies.
Previous studies on the genus have described the rise of spontaneous triploids in fish, including Astyanax schubartii (Morelli et al., Reference Morelli, Bertollo and Moreira1983); Astyanax scabripinnis (Maistro et al., Reference Maistro, Lúcia Dias, Foresti, Oliveira and Filho1994; Machado et al., Reference Machado, Ferreira-Neto, Bakkali, Vicari, Artoni, Oliveira and Foresti2012); and Astyanax (Fauaz et al., Reference Fauaz, Vicente and Moreira-Filho1994; Kantek et al., Reference Kantek, Noleto, Fenocchio and Cestari2007). These studies did not focus on hybrids, emphasizing a need for future studies on triploids. Other studies have demonstrated the effects of oocyte ageing on aneuploidy rate and triploid larvae in fish (Aegerter & Jalabert, Reference Aegerter and Jalabert2004; Flajšhans et al., Reference Flajšhans, Kohlmann and Rab2007). This process can also occur in nature. Salmonids, for example, release ovulated eggs only when natural conditions are optimal (Barlaup et al., Reference Barlaup, Lura, Sægrov and Sundt1994) and this delay might affect the ploidy status of the offspring. Therefore, we believe that in vivo storage of oocytes may be an important cause of natural triploidy in fish.
Our results showed that in vivo storage of oocytes may lead to non-viable embryos. Consequently, the decreased survival among all treatment groups may be due to oocyte ageing and ‘over-ripening’, a phenomenon that leads to alteration in eggs for example in their morphology and physiology (Lahnsteiner et al., Reference Lahnsteiner, Soares, Ribeiro and Dinis2008; Mohagheghi Samarin et al., Reference Mohagheghi Samarin, Policar and Lahnsteiner2015). Therefore, in captivity, in which stripping of oocytes is performed manually, this event needs to be controlled.
Conversely, short-term storage of oocytes might be necessary. In some cases, the male may not be available at the same time as the female or, for particular procedures such as chromosome set manipulation, short-term storage could be essential. For this aspect, the length of time that the oocytes remain viable can vary among species, for example 2 h for Asian catfish (Pangasius hypophthalmus) (Legendre et al., Reference Legendre, Slembrouck, Subagja and Kristanto2000) and 9 h for the South American catfish (Rhamdia sapo) (Ros et al., Reference Ros, Amutio, Arceredillo, Orti and Nani1984). In our study, for up to 60 min of in vivo storage, non-significant effects were observed for survival during early development. Therefore, determination of the time for storage for each species is necessary to optimize seed production.
In conclusion, we showed that oocyte ageing had a significant effect on survival and may affect the ploidy status of A. atiparanae. These data provide new information that would be applicable for basic and applied sciences in the field of chromosome set manipulation and karyological studies.
Financial support
The authors thank the São Paulo Research Foundation (FAPESP 2016/12383-0) for the PhD scholarship; the Centro de Aquicultura da UNESP (CAUNESP), FAPESP (FAPESP 2010/17429-1 e 2011/11664-1), AES Tietê, CEPTA/ICMBio for providing the fish; and the FCAV/UNESP (Faculdade de Ciências Agrárias e Veterinárias) for assistance with the histological analysis.
Conflicts of interest
None
Ethical standards
Not applicable