Introduction
The genus Brycon is part of the order Characiformes and the Characidae family and has 42 valid species that are distributed widely from the south of Mexico to the Prata River in Argentina (Hilsdorf et al., Reference Hilsdorf, Oliveira, de Lima and Matsumoto2008). Fish species that belong to the Brycon genus have great aquaculture potential due to their fast adaptation to captivity, fast weight gain, and easy commercialization of their meat (Zaniboni-Filho et al., Reference Zaniboni-Filho, Reynalte-Tataje and Weingartner2006). Brycon orthotaenia Günther, 1864, previously known as Brycon lundii Reinhardt 1874, which may reach up to 7 kg of body weight, is an important fish for commercial and sport fishing. It breeds from October to January with total spawning and is endangered in some regions of the São Francisco River Basin, Brazil (Gonçalves et al., Reference Gonçalves, Bazzoli and Brito2006). The species is also included in the International Union for Conservation of Nature Red List, in which it is included in the vulnerable category (Reis & Lima, Reference Reis and Lima2007).
Analyses of the embryonic and larvae development of fish are important for studies in the following areas: fishing biology, systematics, taxonomy, phylogenetics, stock monitoring, spawning site identification and incorporation of new species into aquaculture (Kendall et al., Reference Kendall, Ahlstrom and Moser1984; Virta & Cooper, Reference Virta and Cooper2009; Perini et al., Reference Perini, Sato, Rizzo and Bazzoli2010).
The early stages of fish development are critical, with a high mortality rate when the environmental conditions are not suitable, especially in the transition from endogenous to exogenous feeding (Braum, Reference Braum and Bagenal1978; Balon, Reference Balon2001). During the larvae stage, weight and length increase due to the progressive differentiation of the body systems and to the characteristic development of larvae into adults. (Kendall et al., Reference Kendall, Ahlstrom and Moser1984).
The yolk syncytial layer (YSL) or periblast is located between the blastoderm and the yolk, and is formed by the incomplete division of the blastomeres. Embryos and larvae get their nutrients from the yolk through the YSL (Kimmel et al., Reference Kimmel, Ballard, Kimmel, Ullmann and Schilling1995; Ninhaus-Silveira et al., Reference Ninhaus-Silveira, Foresti, De Azevedo, Agostinho and Veríssimo-Silveira2007). Lack of food, after the complete absorption of the yolk, may be responsible for low growth, abnormalities and larvae mortality (Xiu-Juan et al., Reference Xiu-Juan, Cao, Huang and Dou2008). Species with cannibalistic habits, such as those from the Brycon genus, present an obstacle to pisciculture, and yield low survival rates (Braum, Reference Braum and Bagenal1978; Vandewalle et al., Reference Vandewalle, Germeau, Besancenet, Parmentier and Baras2005; Zaniboni-Filho et al., Reference Zaniboni-Filho, Reynalte-Tataje and Weingartner2006). Larvae of several species have temporary structures that decrease these high mortality rates, such as adhesive organs located on the head, with the main function of adhering larvae to the substrate (Britz et al., Reference Britz, Kirschbaum and Heyd2000; Meijide & Guerrero, Reference Meijide and Guerrero2000; Balon, Reference Balon2001; Gomes et al., Reference Gomes, Scarpelli, Arantes, Sato, Bazzoli and Rizzo2007).
There is no study to date that details the early development of B. orthotaenia, therefore this study aimed to analyse the embryogenesis and larvae ontogenesis of this species, with the purpose of contributing to its conservation, taxonomy and the improvement of induced reproduction and larviculture techniques.
Material and methods
Animals
B. orthotaenia breeders (n = 108) were kept in 200 m2 aquaculture tanks, with an mean depth of 1 m, in the Três Marias Hatchery and Hydrobiology Station, CODEVASF, Minas Gerais, Brazil (18°11′58″S, 45°15′07″W). The fish were fed with pelleted commercial feed, with 36% crude protein content, in a proportion of 1.5–2.0% of live weight per day. Ten females that weighed between 1.100 to 2.100 g and 10 males that weighed 300 to 670 g were transferred to hypophysation tanks at 26°C water temperature and were submitted to induce reproduction by hypophysation.
Spawning, fertilization and egg sample collection
Two doses (0.8 mg and 5.6 mg/kg of body weight) of crude common carp (Cyprinus carpio) pituitary extract were used to induce spawning in females, with a 14 h interval between the doses. A single extract dose 2.7 mg/kg was used for the males. The injections were administered in the coelomic cavity, and the extrusion of oocytes and semen was performed manually 7 h after the second hormone dose. Fertilization was carried out using the dry method and the fertilized eggs were kept in funnel-type 60-litre incubators, with a constant water flow at 24°C. A total of 20 non-hydrated oocytes and 20 fertilized and hydrated oocytes were collected after extrusion to measure their diameter, using a micrometric ocular coupled to a stereomicroscope (and expressed as mean and standard deviation). Egg samples were collected for analysis every 10 min until hatching, and a photographic documentation was made using a stereomicroscope.
Histology and histochemical analysis
After hatching, the larvae were fed with Prochilodus costatus larvae during the study period, i.e. 7 days. Every day, 10 larvae were collected until the complete resorption of the yolk sac occurred. The larvae were anaesthetized using MS222, fixed in Bouin's fluid for 12 h and embedded in historesin. Histological sections of 3 μm diameter were stained with toluidine blue–sodium borate. For histochemical analysis, larvae fixed in Bouin's fluid were embedded in paraffin, cut into 5 μm thick sections and submitted to the following staining techniques: periodic acid–Schiff (PAS); Alcian blue (AB) pH 2.5; and Alcian blue (AB) pH 0.5.
Each day, an additional 20 larvae were fixed in 4% neutral formaline for morphometric analysis under the stereomicroscope to determine the total length, body weight, yolk sac length and yolk sac height.
Statistical analysis
The Pearson correlation coefficient (r) was used to analyse data on the relationship between day after hatch with total length, body weight, yolk sac height and yolk sac length and between total length with body weight, yolk sac height with yolk sac length. The degree of association between the variables was evaluated by the correlation coefficient (r).
Results
Recently extruded B. orthotaenia oocytes were spherical, dark green, and non-adhesive, with a diameter of 1479.67 ± 53.18 and 3094.60 ± 80.34 μm after hydration. Blastopore closure occurred within 7 h 30 min of fertilization and the fertilization rate was 50.0 ± 5.5% at 24°C. The duration of embryogenesis, from fertilization to hatching, was 21 h and 30 min at 24 °C. The main phases of embryonic development with respective times were: cleavage (1 h 30 min), blastula (2 h 30 min), gastrula (6 h), blastopore closure (7 h 30 min), differentiation of embryonic germ layers (9 h), tail release (15 h) and hatching (21 h 30 min). The beginning of the successive divisions of the blastodisc into blastomeres was observed during the cleavage phase (Fig. 1A). The stratification of the blastodisc occurred in the blastula phase, and formed a protuberance on the animal pole (Fig. 1B). The start of epiboly movement was observed in gastrula and covered half of the vegetal pole (Fig. 1C); formation of the epiblast and hypoblast were also observed. The end of the epiboly movement (Fig. 1D) culminated with the blastopore closure. Somitogenesis and the formation of the optic cup were observed during the differentiation of embryonic germ layers, (Fig. 1E). In conjunction with the beginning of myomere contractions, the posterior part of the body separated from the yolk sac, and led to tail release (Fig. 1F). Hatching occurred due to chorion rupture (Fig. 1G).

Figure 1 B. orthotaenia main embryogenesis phases. (A) Cleavage. (B) Blastula. (C) Gastrula. (D) End of the epiboly movement. (E) Differentiation of embryonic germ layers (arrow: optic cup), myomeres (M). (F) Tail release (arrow). (G) Hatching.
First day: The following phases were identified during larval ontogenesis, time after fertilization is given and in parentheses the respective larvae total length mean ± standard deviation. The recently hatched larvae (first day) (2.87 ± 0.15 mm) presented a wide yolk sac with spherical yolk globules, and evident notochord and digestive tube with a closed lumen (Fig. 2A).
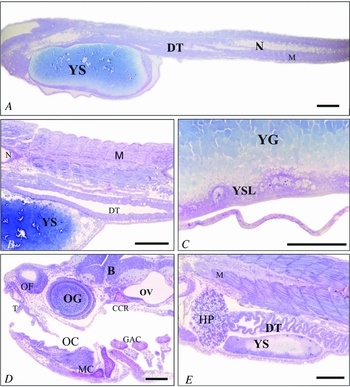
Figure 2 B. orthotaenia larval ontogenesis phases. (A) First day: yolk sac (YS), notochord (N), myomeres (M), digestive tube with closed lumen (DT). (B) Second day: open and undifferentiated digestive tube (DT), reduced yolk sac (YS), distinct myomeres (M), notochord (N). (C) Second day: yolk syncytial layer (YSL), spherical yolk globules (YG). (D) Third day: otic vesicle (OV), olfactory chamber (OF), ocular globe (OG), brain (B), teeth (T), oral cavity (OC), gill arches cartilages (GAC), and jaw (MC) and skull cartilage (CCR). (E) Third day: Digestive tract in differentiation (DT), and residual yolk sac (YS), myomeres (M), hepatopancreas (HP). Scale bars = 200 μm in (A, D, E); 100 μm in (B, C).
Second day: larvae length (3.69 ± 0.33 mm) yolk sac reduction, open digestive tube lumen, conspicuous YSL (Fig. 2B,C).
Third day: larvae length (4.58 ± 0.46 mm) mouth opening, differentiation of encephalic grey and white matter, early formation of the jaw cartilage, gill arches and the skull. The nasal cavity was evident and the ocular globe was in the process of becoming pigmented. On the third day we also observed the beginning of the digestive tract differentiation; the yolk sac almost completely resorbed, and presented only residual yolk globules (Fig. 2E). We observed the nasal chamber covered with olfactory ciliated stratified prismatic epithelium on the anterior region of the skull.
The adhesive organ, located on the dorsal region of the skull, presented prismatic cells with apical cytoplasmic projections (Fig. 3B). Mucosubstances in these cells were detected, due to a positive reaction to PAS (Fig. 3C) and AB 2.5 (Fig. 3D). From the third day onwards, after the mouth opened, there was intense cannibalism, including formation of a larvae chain. (Fig. 4A–D).

Figure 3 B. orthotaenia larval ontogenesis phases. Third day: (A) Olfactory chamber covered with olfactory ciliated stratified prismatic epithelium (arrow). (B) Adhesive organ with apical cytoplasmatic projections (arrows), brain (B). (C) Positive PAS reaction (arrows) at the adhesive organ cells, brain (B). (D) Positive AB 2.5 reaction (arrows) at the adhesive organ cells, brain (B). Fourth day: (E) Differentiated esophagus (ES), stomach with food particles (*). (F) Detail of the small intestine mucous (SI) (foregut) with simple prismatic epithelium with striated border. Scale bars = 200 μm in (E); 100 μm in (A–D); 50 μm in (F).

Figure 4 (A–D) Cannibalism amongst B. orthotaenia larvae from the third day after hatching onwards.
Fourth day: larvae length (6.05 ± 0.69 mm), we observed a differentiated esophagus, stomach mucous with simple prismatic epithelium and lumen with food particles (Fig. 3E); the small intestine or foregut had villi and mucous with characteristic simple prismatic epithelium with a striated border (Fig. 3F).
Fifth day: larvae length (8.93 ± 0.71 mm) the large intestine or hindgut presented the characteristic goblet cells and open urogenital papilla (Fig. 5A). The gills, heart, cranial kidney, cranial cartilages, hepatopancreas and gas bladder were also observed (Fig. 5B).

Figure 5 B. orthotaenia larval ontogenesis phases. Fifth day: (A) Differentiated large intestine (hindgut) with characteristic goblet cells (arrows) and open urogenital papilla (*). (B) Evident: Gill (GA), heart (H), cranial kidney (CK), hepatopancreas (HP), myomeres (M) and gas bladder (GB). Sixth and seventh days: (C) Cranial region with gill arches (GA), brain (B), cranial kidney (CK), hepatopancreas (HP), otic vesicle (OV), ocular globe (OG), stomach (S), heart (H), myomeres (M), gas bladder (GB), chondrocranium cartilages (CCR). (D) Conspicuous cartilages of the anal fin rays (*). (E) Ocular globe detail showing retina's rods and cones (arrows). (F) Sixth and seventh days: Vascularized gills (*), and developed gill filaments (arrows). Scale bars = 200 μm in (B, C, F); 100 μm in (A, D, E).
Sixth and seventh day: larvae length (sixth day: 8.98 ± 0.59; seventh day 9.58 ± 0.67 mm) the skull cartilages (Fig. 5 C) and the cartilages of the anal fins (Fig. 5D). During this period of time, the eyes became pigmented with retinal rods and cones (Fig. 5E) and developed and vascularized gill arches (Fig. 5F).
The larvae's average total length (Fig. 6A) and average body weight (Fig. 6B) increased gradually from the first to the seventh day, with a high correlation coefficient (r) (Fig. 6C), while the yolk sac height (Fig. 6D) and yolk sac length (Fig. 6E) decreased from the first to the third day, also with a high correlation coefficient (r) (Fig. 6F).

Figure 6 Growth graphic representation of B. orthotaenia larvae kept in funnel-type incubators with circulating water at 24°C for 7 days. (A) Total length (mm). (B) Weight (g). (C) Weight and length correlation. (D) Yolk sac height (mm). (E) Yolk sac length (mm). (F) Yolk sac height and length correlation. Pearson correlation coefficient (r).
Discussion
The majority of the neotropical teleost species that have migratory reproduction, including B. orthotaenia, does not reproduce naturally in captivity, therefore reproduction needs to be induced. This study analysed B. orthotaenia embryogenesis and larval ontogenesis by induced reproduction through hypophysation, in order to contribute to improving handling and breeding of this species.
The results of the induced reproduction techniques obtained during this study were similar to those of Sato et al. (Reference Sato, Fenerich-Verani, Godinho, Verani, Godinho and Godinho2003b). In this study, the fertilization rate was 50.0 ± 5.5 % at 24°C. Oocyte fertilization rates vary among the species, depending upon the oocyte quality and the hormone protocol used for induction of final oocyte maturation and ovulation (Mylonas et al., Reference Mylonas, Fostier and Zanuy2010).
Teleost oocytes vary in colour, but green is common amongst fish of the genus Brycon (Andrade-Talmelli et al., Reference Andrade-Talmelli, Kavamoto, Romagosa and Fenerich-Verani2001; Alexandre et al., Reference Alexandre, Ninhaus-Silveira, Veríssimo-Silveira, Buzollo, Senhorini and Chaguri2010), as also observed in this study. Brycon orthotaenia non-hydrated oocytes in this study presented a small diameter when compared with other Characiformes (Sato et al., Reference Sato, Fenerich-Verani, Nuñer, Godinho, Verani, Godinho and Godinho2003a). Oocyte diameter is related to the reproductive strategy: in species with parental care, non-hydrated oocytes are larger than those without parental care (Kolm & Ahnesjö, Reference Kolm and Ahnesjö2005).
Oocyte hydration in teleosts is carried out by incorporation of water through the crude protein of the membrane named aquaporin (Lubzens et al., Reference Lubzens, Young, Bobe and Cerdà2010). The oocyte diameter increases after hydration due to the formation of the perivitelline space, which protects the embryo against mechanical shocks (Andrade-Talmelli et al., Reference Andrade-Talmelli, Kavamoto, Romagosa and Fenerich-Verani2001). In this study, the hydrated oocyte presented a diameter twice as large as the non-hydrated oocyte, due to the formation of a wide perivitelline space, contrary to that found for Siluriformes, which present a small perivitelline space (Suzuki et al., Reference Suzuki, Agostinho and Winemiller2000; Perini et al., Reference Perini, Sato, Rizzo and Bazzoli2010).
Brycon orthotaenia oocytes, as with the majority of teleost fish, are classified as telolecitic because they contain a large amount of evenly distributed yolk (Marques et al., Reference Marques, Nakaghi, Faustino, Ganeco and Senhorini2008, Perini et al., Reference Perini, Sato, Rizzo and Bazzoli2010). The cleavage of B. orthotaenia oocytes followed the meroblastic pattern, restricted to the animal pole, as is observed commonly in teleosts (Meijide & Guerrero et al., Reference Meijide and Guerrero2000, Ninhaus-Silveira et al., Reference Ninhaus-Silveira, Foresti and de Azevedo2006, Gomes et al., Reference Gomes, Scarpelli, Arantes, Sato, Bazzoli and Rizzo2007, Amorim et al., Reference Amorim, Gomes, Martins, Sato, Rizzo and Bazzoli2009, Perini et al., Reference Perini, Sato, Rizzo and Bazzoli2010). The identification of the blastopore closure is important in aquaculture, as it indicates that fertilization was successful. It also indicates the effective moment to estimate the fertilization rate, as non-fertilized oocytes develop parthenogenetically until blastopore closure (Perini et al., Reference Perini, Sato, Rizzo and Bazzoli2010). In B. orthotaenia, blastopore closure occurred at 7 h and 30 min, similar to the time found for other species of Brycon (Reynalte-Tataje et al., Reference Reynalte-Tataje, Zaniboni-Filho and Esquivel2004; Alexandre et al., Reference Alexandre, Ninhaus-Silveira, Veríssimo-Silveira, Buzollo, Senhorini and Chaguri2010).
The embryonic development of B. orthotaenia lasted 21 h and 30 min, at 24°C water temperature, similar to the time registered for B. moorei (Baras et al., Reference Baras, Ndao, Maxi, Jeandrain, Thome, Vandewalle and Melard2000) and B. orbignyanus (Reynalte-Tataje et al., Reference Reynalte-Tataje, Zaniboni-Filho and Esquivel2004). In contrast, at 26°C, the embryonic development of B. cephalus (Alexandre et al., Reference Alexandre, Ninhaus-Silveira, Veríssimo-Silveira, Buzollo, Senhorini and Chaguri2010) and B. insignis (Andrade–Talmelli et al., Reference Andrade-Talmelli, Kavamoto, Romagosa and Fenerich-Verani2001) was faster, at 11 h and 14 h respectively. It has been shown previously that high temperatures, up to a certain limit, accelerate embryogenesis, chorion rupture and, consequently, hatching (Borçato et al., Reference Borçato, Bazzoli and Sato2004; Marques et al., Reference Marques, Nakaghi, Faustino, Ganeco and Senhorini2008, Rodrigues-Galdino et al., Reference Rodrigues-Galdino, Maiolino, Forgati, Donatti, Mikos, Carneiro and Rios2010). Brycon orthotaenia embryogenesis was considered fast, similar to other reproductive migrating Characiformes with reproductive strategies of total spawning without parental care, such as Prochilodus lineatus (Ninhaus-Silveira et al., Reference Ninhaus-Silveira, Foresti and de Azevedo2006) and Leporinus piau (Borçato et al., Reference Borçato, Bazzoli and Sato2004). These results corroborate the results of Sato et al. (Reference Sato, Fenerich-Verani, Godinho, Verani, Godinho and Godinho2003b) that related the short duration of embryogenesis in species of Brycon to the small, free oocytes with large perivitelline space, and to the need for reproductive migrations and the lack of parental care. Conversely, the time to embryogenesis was relatively long, 44 to 50 h in three species of Characiformes Erythrinidae that present a reproductive strategy of fractionated spawning and parental care (Gomes et al., Reference Gomes, Scarpelli, Arantes, Sato, Bazzoli and Rizzo2007).
Brycon orthotaenia larvae hatched with a total length of 2.87 ± 0.11 mm, and a large yolk sac (1.04 ± 0.05 mm long and 0.53 ± 0.03 mm high). In 7 days they reach a total length of 9.58 ± 0.67 mm and have a completely reabsorbed yolk sac, similar to other species of Brycon (Baras et al., Reference Baras, Ndao, Maxi, Jeandrain, Thome, Vandewalle and Melard2000; Vandewalle et al., Reference Vandewalle, Germeau, Besancenet, Parmentier and Baras2005), showing fast growth, which is a desirable trait in aquaculture fish. Yolk absorption is carried out through the YSL, which was clearly visible on histological slides of B. orthotaenia larvae 2 days after hatching.
In teleost larvae, the time of the opening of the mouth varies amongst the species (Kendall et al., Reference Kendall, Ahlstrom and Moser1984; Baras et al., Reference Baras, Ndao, Maxi, Jeandrain, Thome, Vandewalle and Melard2000; Andrade–Talmelli et al., Reference Andrade-Talmelli, Kavamoto, Romagosa and Fenerich-Verani2001; Borçato et al., Reference Borçato, Bazzoli and Sato2004; Reynalte-Tataje et al., Reference Reynalte-Tataje, Zaniboni-Filho and Esquivel2004; Amorim et al., Reference Amorim, Gomes, Martins, Sato, Rizzo and Bazzoli2009; Perini et al., Reference Perini, Sato, Rizzo and Bazzoli2010). For B. orthotaenia, it occurred on the third day, at the same time as the development of structures for food detection, capture and assimilation, which shows the need for exogenous feeding. The transition stage, between endogenous and exogenous feeding, is critical for larvae survival, and limits pisciculture procedures (Baras et al., Reference Baras, Ndao, Maxi, Jeandrain, Thome, Vandewalle and Melard2000; Zaniboni-Filho et al., Reference Zaniboni-Filho, Reynalte-Tataje and Weingartner2006).
Larvae of some species may present intense cannibalism, during the transition stage between endogenous and exogenous feeding, as observed in the present study's larvae. This behaviour seems to be a characteristic of the Brycon fish, as it is observed in many species (Baras et al., Reference Baras, Ndao, Maxi, Jeandrain, Thome, Vandewalle and Melard2000; Andrade–Talmelli et al., Reference Andrade-Talmelli, Kavamoto, Romagosa and Fenerich-Verani2001; Reynalte-Tataje et al., Reference Reynalte-Tataje, Zaniboni-Filho and Esquivel2004; Vandewalle et al., Reference Vandewalle, Germeau, Besancenet, Parmentier and Baras2005; Zaniboni-Filho et al., Reference Zaniboni-Filho, Reynalte-Tataje and Weingartner2006). In tropical species, cannibalism is more intense in larvae and fingerlings than it is in adults, due to the fast development of the structures related to food capture. This factor is an advantage for the piscivorous species with seasonal breeding that can, during this stage, consume occasional prey (Baras et al., Reference Baras, Ndao, Maxi, Jeandrain, Thome, Vandewalle and Melard2000), as observed in B. orthotaenia. On the other hand, cannibalism is an obstacle to productivity in piscicultures for several species, such as Thunnus orientalis (Sabate et al., Reference Sabate, Sakakura, Tanaka, Kumon, Nikaido, Eba, Nishi, Shiozawa, Hagiwara and Masuma2010).
The mucosubstances detected on the adhesive organ of B. orthotaenia may be related to larvae adhesion to the substrate, which is an important characteristic for survival. These organs, also observed in some teleost species (Meijide & Guerrero, Reference Meijide and Guerrero2000; Gomes et al., Reference Gomes, Scarpelli, Arantes, Sato, Bazzoli and Rizzo2007) may constitute a taxonomic characteristic (Britz et al., Reference Britz, Kirschbaum and Heyd2000). The presence of the adhesive organ on fish in this genus was first described in the present study.
Acknowledgements
The authors thank the partnership of CEMIGGT/CODEVASF and the Brazilian research foundations of CNPq, CAPES and FAPEMIG for financial support.