Introduction
Characidae is the largest and the most complex family within Characiformes, comprising the highest number of species in relation to the other families in this order (Howes, Reference Howes1982; Nakatani et al., Reference Nakatani, Agostinho, Baumgartner, Bialetzki, Sanches and Cavicchioli2001) and it includes a great number of subfamilies with quite distinctive features. The subfamily Bryconinae encompasses several medium- to large-sized species, widespread throughout South and Central America (Britski et al., Reference Britski, Silimon and Lopes1999).
Fish species of the genus Brycon are severely threatened by dams and deforestation along river margins once they are rheophilic, undergoing reproductive upstream migration, and feed upon alloctone sources such as fruits and seeds (Castagnolli, Reference Castagnolli1992). According to IBAMA (2009), the genus Brycon represents many species in the List of Threatened Aquatic Species. These are: Brycon devillei and Brycon insignis, both known as piabanha; Brycon nattereri, popularly referred to as pirapitinga; Brycon opalinus, usually known as pirapitinga or pirapitinga-do-sul; Brycon orbignyanus, the piracanjuba, picaranjuva or bracanjuva; and Brycon vermelha, known as vermelha.
According to Junk & Nunes de Mello (Reference Junk and Nunes De Mello1990), the construction of dams causes a huge impact leading to losses of both fauna and flora, including some completely or relatively unknown species such as Brycon gouldingi, studied in the present work.
This species was recently described by Lima (Reference Lima2004) as an endemic fish from Tocantins-Araguaia River Basin that feeds mainly on fruits and insects, inhabiting tropical benthopelagic freshwater environments. There are no available reports about this species, although it has been used as a food source by local communities for a long time and raised empirically in captivity by some fish farmers (personal communication).
Morphologically, this species is characterized by the presence of a wide fifth supra-orbital bone, several narrow longitudinal and curving stripes throughout the body, dark pectoral and pelvic fins, a distinctive V-shaped spot in the peduncle and caudal fin, and 66–82 scales in the lateral line (Lima, Reference Lima2004).
A successful rearing of a fish species depends on knowledge about its early biology, once it provides essential information to accomplish a mass laboratory production (Matkovic et al., Reference Matkovic, Cussac, Cukier, Guerrero and Maggese1985). The embryonic process begins when the oocyte is fertilized by the spermatozoon via micropyle, and it basically focuses on the reorganization of egg elements. In order to be understood, embryonic studies should be combined with topological analyses of fertilized eggs (Depêche & Billard, Reference Depêche and Billard1994).
In Brazil, there are reports about these aspects in species like Rhamdia hilarii (Godinho et al., Reference Godinho, Fenerich and Narahara1978), Prochilodus lineatus (Castellani et al., Reference Castellani, Tse, Santos, Faria and Santos1994), Collosoma macropomum (Albuquerque et al., Reference Albuquerque, Bezerra e Silva and Kóvacs1994; Ribeiro et al., Reference Ribeiro, Leme dos Santos and Bolsan1995), Piaractus mesopotamicus (Ribeiro et al., Reference Ribeiro, Leme dos Santos and Bolsan1995), Pseudoplatystoma corruscans, (Cardoso et al., Reference Cardoso, Alves, Ferreira and Godinho1995), Brycon cephalus (Lopes et al., Reference Lopes, Senhorini and Soares1995; Romagosa et al., Reference Romagosa, Narahara and Fenerich-Verani2001), Pimelodus maculatus (Luz et al., Reference Luz, Reynalte-Tataje, Ferreira and Zaniboni-Filho2001), Brycon orbignyanus (Nakatani et al., Reference Nakatani, Agostinho, Baumgartner, Bialetzki, Sanches and Cavicchioli2001) and Brycon insignis (Andrade-Talmelli et al., Reference Andrade-Talmelli, Kavamoto, Romagosa and Fenerich-Verani2001).
Besides these important reports, other works have been recently carried out in Brycon orbignyanus (Maciel, Reference Maciel2006; Ganeco et al., Reference Ganeco, Franceschini-Vicentini and Nakaghi2008), Prochilodus lineatus (Ninhaus-Silveira et al., Reference Ninhaus-Silveira, Foresti and Azevedo2006), Brycon orthotaenia, Leporinus obtusidens, Prochilodus argenteus, Salminus brasiliensis (Nakaghi et al., Reference Nakaghi, Marques, Faustino and Senhorini2006; Sampaio, Reference Sampaio2006), the hybrid Pseudoplatystoma corruscans × Pseudoplatystoma fasciatum (Faustino et al., Reference Faustino, Nakaghi, Marques, Makino and Senhorini2007), Brycon amazonicus (Neumann, Reference Neumann2008), Pseudoplatystoma corruscans (Landines et al., 2003; Marques et al., Reference Marques, Nakaghi, Faustino, Ganeco and Senhorini2008) and Zungaro jahu (Marques, Reference Marques2008).
Because of the importance of basic studies on the embryonic development of fish, particularly to phylogenetics, biology, and production, combined with the lack of reports in B. gouldingi, the goal of the present work was to provide information about the structure and ultrastructure of oocytes and the embryonic development of this species of aquaculture potential through induced reproduction of adult specimens.
Material and methods
Ten collections were performed in December 2007 and January 2008, at Buriti fish farm, Nova Mutum, in the state of Mato Grasso, Brazil, after induced reproduction of specimens of B. gouldingi according to the methodology described by Woynarovich & Horváth (Reference Woynarovich and Horváth1983). In the 10 selected females, the concentration of the first hypophysis extract inoculation was 0.5 mg/kg, and the second one was applied 10 h later in a proportion of 5.0 mg/kg. Single shots at 1.0 mg/kg were applied in males. Fertilization took place in dry conditions by adding the spermatozoa onto the eggs. Then, water was added to the mixture to promote the egg's hydration. Afterwards, these eggs were gently rinsed in water to remove any excess semen and each set was individually placed in 200-l conical glass fiber incubators, with water renewal of 6 l s−1 and at a temperature of 26°C.
The samples were collected at the moment of extrusion, fertilization (time zero), 10, 20, and 30 s post-fertilization, each minute up to 10 min, each 5 min up to 45 min, and then each hour up to larval hatching. The samples were fixed in modified Karnovsky's solution (2.5% glutaraldehyde and 2.5% paraformaldehyde) for 24 h. Afterwards, they were washed and transferred into 0.1 M sodium cacodylate buffer (pH 7.4) and stored at low temperature.
This material was transported to the Faculty of Agricultural and Veterinary Sciences at São Paulo State University (FCAV/UNESP), Jaboticabal campus – SP. The sample analysis using light microscopy was carried out in the Histology and Embryology Laboratory at the Morphology and Animal Physiology Department, whereas the analyses by scanning electron microscopy were performed in the Electron Microscopy Laboratory.
Light microscopy
The selected samples were dehydrated for 24 h in alcohol 80% and for 30 min each in 95% and 100% alcohol. Then, they were immersed for 4 h in a pre-embedding GMA solution (glycol methacrylate) + 100% alcohol at a ratio of 1:1, and 16 h in the embedding GMA solution for further inclusion in histomolds. The samples were placed in a drier at 50°C for 24 h. Semi-serial 2-μm thick sections were obtained using a LEICA RM2255 microtome with tungsten carbide blades. The first five cuts were discarded and the others were stained with heamatoxylin–eosin (HE) (Tolosa et al., Reference Tolosa, Behmer and Freitas Neto2003). Analysis and photodocumentation were carried out in a Leica DM 5000 B photomicroscope coupled with Leica Application Suite (LAS) software.
Scanning electron microscopy
The samples were selected and post-fixed in 1% osmium tetroxide for 2 h, and washed again in the same buffer. They were subsequently dehydrated in a graded series of ethanol at 30, 50, 70, 80, 90 and 95% plus three baths at 100% for 7 min each. Then, they were dried to the critical point in a liquid CO2 drier (BAL-TEC), mounted in copper grid, metallized with gold (DENTON VACUM DESK II) and electron-micrographed under a scanning electron microscope (JEOL-JSM 5410).
Results
In the present study, we used the term ‘egg’ to refer to the stages from fertilization up to the end of gastrulation, when the formation of the embryonic axis takes place and it is then referred as ‘embryo’. The term ‘larva’ was used to the moment of hatching. The word ‘oocyte’ indicates the feminine gamete, prior to fertilization.
The oocytes of B. gouldingi were slightly egg shaped with a mean diameter of 1.13 ± 0.06 mm and a greyish-green colouration at the moment of extrusion. A single micropyle (region through which a spermatozoon can enter) was visualized in each oocyte (Fig. 1A), being composed of a micropyle canal and a vestibule (depression) with longitudinal folds on the walls (Fig. 1B). It was possible to detect several cortical alveoli of different sizes at the peripheral region of oocytes (cortical cytoplasm) as well as the presence of chorion (protective oocyte membrane) (Fig. 1C,D).
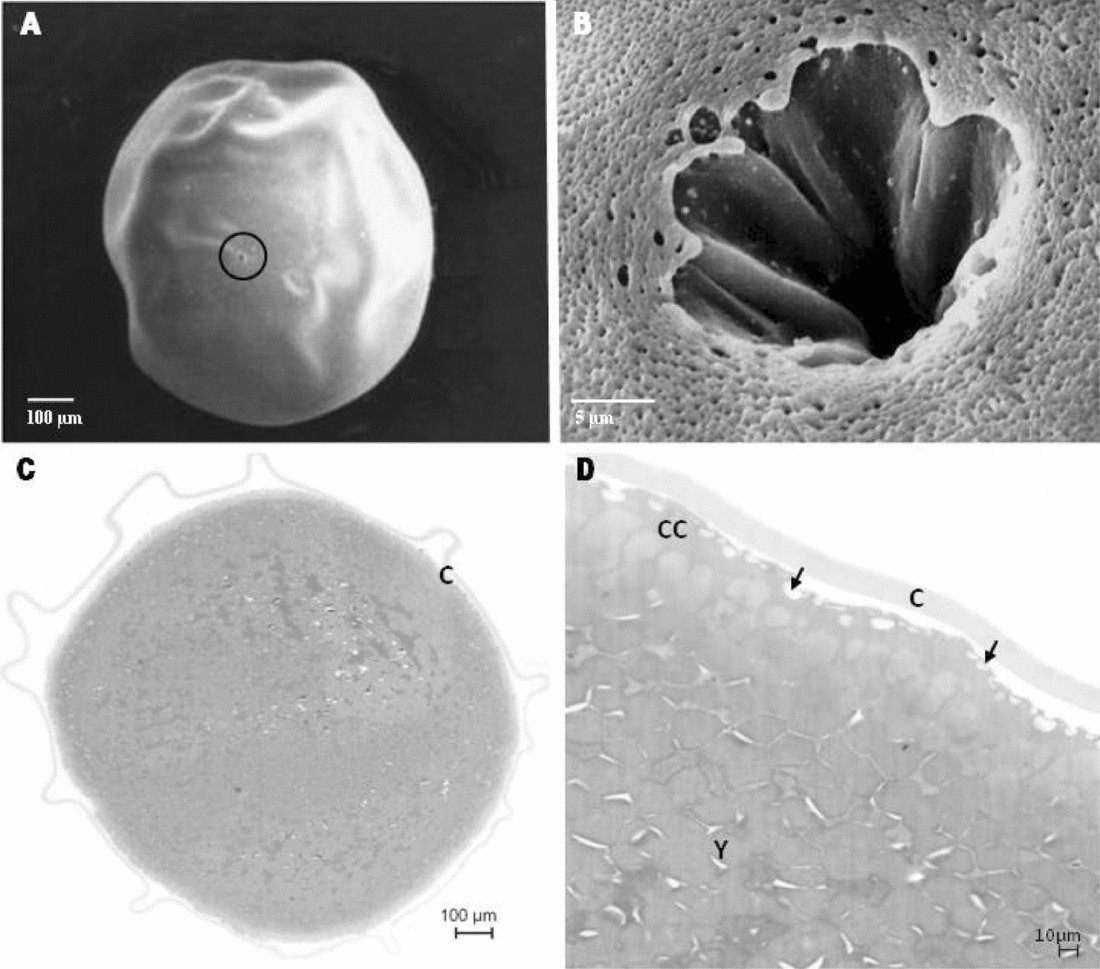
Figure 1 Scanning electron micrographs (A,B) and photomicrographs (C,D) of oocytes of B. gouldingi. Extrusion: (A) Oocyte showing the micropyle (circle); (B) Detail of oocyte micropyle: funnel-shaped with longitudinal folds; (C) Oocyte with presence of chorion (C); (D) Detail of the oocyte with yolk (Y), cortical cytoplasm (CC) filled with cortical alveoli (→) and chorion (C).
The embryonic development of B. gouldingi in the 10 collections (from fertilization up to larval hatching) has taken, in average, 13.9 h at 26.4 ± 1.12°C. During this period, we observed six stages: zygote, cleavage (including the morula phase), blastula, gastrula, histogenesis/organogenesis and hatching, with particular features as follows:
Zygote stage
This stage comprised the moment of fertilization up to the formation of the zygote (or egg cell), with the distinction between the animal and vegetative poles. At time zero (when spermatozoa and oocytes were mixed for fertilization), several spermatozoa were observed over the vestibule towards the micropyle canal (Fig. 2A) up to 1 min after fertilization (mpf) (Fig. 2B). The fertilization cone was seen blocking the entrance of the micropyle canal within 30 s post-fertilization (spf) (Fig. 2C). This evidence reveals that several eggs had been fertilized and protected from polyspermy by the formation of such cones, which trespass the micropyle canal and resemble a spherical structure.

Figure 2 Scanning electron micrographs (A–C) and photomicrographs (D–H) of eggs of B. gouldingi. (A) Fertilization (time zero) – spermatozoa on the micropyle vestibule. (B) 1 min post fertilization (mpf) spermatozoa in the micropyle vestibule. (C) 30 s post fertilization (spf) formation of the fertilization cone (FC). (D) 3 mpf: Beginning of cytoplasm movement (arrows) to define the animal and vegetative poles. (E) 3 mpf: Detail of the animal pole (AP). (F) 3 mpf: Detail of vegetative pole (VP) with cortical alveoli (→). (G) 10 mpf: cytoplasm movement defining the animal (AP) and the vegetative pole (VP). (H) 45 mpf: complete formation of animal (AP) and vegetative poles (VP).
Within 1 min and 30 s after fertilization, the spermatozoa were no longer detectable and the eggs assumed a flattened aspect because of the cytoplasm movements towards the animal pole, suggesting that the spermatozoa had already fertilized the oocytes at this moment.
At 3 mpf, the eggs were remarkably flattened as a result of the cytoplasm movements (Fig. 2D) that defined the animal and vegetative poles. Furthermore, the cortical alveoli were more concentrated in the vegetative pole than in the animal pole (Fig. 2E,F). This feature allowed us inferring that the cortical reaction (alveoli rupture) in B. gouldingi has started in the region of the animal pole following up to the vegetative pole, also avoiding the polyspermy. At 5 mpf, these alveoli were unnoticeable and, at 10 mpf, the differentiation between both animal and vegetative poles were more conspicuous (Fig. 2G), being totally differentiated at 45 mpf, thus forming the egg cell (Fig. 2H).
Cleavage stage
This stage has taken place between 45 mpf and 1 h post-fertilization (hpf) at the animal pole, which has firstly divided into two blastomeres (embryonic cells) that further split into four blastomeres, and then successively up to 32 cells, while the number of small cells became increasingly higher (data not shown).
Morula phase
At 2 hpf, the successive cell divisions (typical of cleavage stage) yielded overlapped layers of blastomeres (more than 64 blastomeres) arranged in a ‘half-berry’ shape, characterizing the morula phase (data not shown).
Blastula stage
The blastula stage was visualized at 3 hpf, when the blastoderm region (embryonic cells) and periblast became clear. This stage extended from the moment that the animal pole presented a cup shape, without identification of embryonic cell boundaries, up to the beginning of the cell movement (data not shown).
Gastrula stage
This stage was characterized by the cell movement, being observed in the present study between 4 and 7 hpf. Figure 3A,B shows the blastoderm (embryonic cells) and periblast at the beginning of the cell movement. This first movement is called epiboly, in which the blastoderm cells encompass the egg. After the blastoderm cells involve nearly half the egg, the second movement got started, representing the involution of the most superficial cells (Fig. 3C). Such movement, named involution, led to a thickness of the egg margins, known as germ ring (Fig. 3D). The involution movement proceeded towards the animal pole, whereas the epiboly undergone the opposite direction (towards the vegetative pole), forming two layers the epiblast (outer layer) and the hypoblast (inner layer), culminating with the formation of the yolk plug (a portion of the yolk that remains uncovered by the embryonic cells) (Fig. 3E,F). Cell division was intense during this stage (Fig. 3D).

Figure 3 Photomicrographs of eggs of B. gouldingi. (A) Beginning of epiboly. (B) Beginning of epiboly with blastoderm (bl) and periblast (pe) in detail. (C) 6 hpf: Epiboly (↓) and involution (↑) movements with formation of the germ ring (circle). (D) 6 hpf: Detail of several embryonic cells undergoing mitosis (→). (E) 7 hpf: Gastrula stage highlighting the epiboly (↓) and involution (↑) movements in embryonic cells and formation of yolk plug (YP). (F) 7 hpf: Late gastrula stage with details of the formation of both layers: epiblast (e) and hypoblast (h).
Histogenesis/organogenesis stage
The formation of the embryo axis and the differentiation of cephalic and caudal region were observed at 8 hpf (Fig. 4A,B), characterizing the organogenesis/histogenesis stage. The neural tube was evident in the cephalic region (Fig. 4B). The first somites could be noticed at 9 hpf (Fig. 4C–E) as well as the notochord (Fig. 4D). The optic vesicle was visualized at 10 hpf (Fig. 4G) and, at 11 hpf, tail detachment and embryo elongation through the cephalic–caudal axis were detected (Fig. 4H) along with the beginning of the differentiation of miomeres.

Figure 4 Scanning electron micrographs (B,C,G,H) and photomicrographs (A,C,E,F) of embryos of B. gouldingi. (A,B) 8 hpf: Formation of the embryonic axis with differentiation of cephalic (CE) and caudal (CA) regions and presence of neural tube (*). (C,D) 9 hpf: Observation of the first somites (S) and notochord (no). (E) 9 hpf: Detail of somites (S). (F) 9 hpf: Detail of notochord (no). (G) 10 hpf: Appearance of optic vesicle (inbox). (H) 11 hpf: Detachment of caudal region (arrow).
At 12 hpf, the olfactory placodes started developing (Fig. 5A,B), as well as the central nervous system (Fig. 5C) and the otic vesicle (Fig. 5C,E), besides the visualization of the notochord throughout a large extension of the embryo (Fig. 5D). At this moment, the optic cup started involving the crystalline primordium (Fig. 5F).

Figure 5 Scanning electron micrographs (A,B) and photomicrographs (C–H) of embryos of B. gouldingi. (A) 12 hpf: Embryo elongation by the cephalic–caudal axis and development of olfactory placodes (arrow). (B) 12 hpf: Detail of olfactory placodes. (C) 12 hpf: Observation of optic cup and optic vesicle (circle), central nervous system (CNS), otic vesicle (OT). (D) 12 hpf: Detail of notochord (no). (E) 12 hpf: Detail of otic vesicle. (F) 12 hpf: Detail of optic cup (OC) and crystalline primordium (CP). (G) 13 hpf: Larval cephalic region: otic vesicle (OT), rudimentary heart (circle); development of central nervous system with division of prosencephalon (PRO), mesencephalon (MES) and rhombencephalon (ROM), presence of epiphysis (E) and cerebellum primordium (*). (H) 13 hpf: Larval cephalic region: optic cup (OC), crystalline vesicle (CV), mouth primordium (MO), olfactory placode (OP).
At 13 hpf, a rudimentary heart was noticeable (Fig. 5G) and a tegument-like epithelium extended continually right in front of the yolk sac (Fig. 5H), signalizing the site where the mouth primordium is going to develop. The crystalline vesicle was completely involved by the optic cup (Fig. 5H). At this moment, we also detected the development of central nervous system, forming the three primary vesicles: anterior brain (prosencephalon), medium brain (mesencephalon) and posterior brain (rhombencephalon). The local of cerebellum formation was also observed (anterior region of rhombencephalon), as well as the epiphysis, derived from the posterior region of prosencephalon (Fig. 5G).
Larval hatching stage
The larval hatching took place at 14 hpf and, at this moment, the larvae presented the head attached to the anterior portion of the yolk sac, a distended posture (Fig. 6A,B) and no fins, except for a embryonic fin surrounding the entire caudal region (Fig. 6A). In the anterior dorsal region, the central nervous system was under development, being observed as a neural tube yet (Fig. 6C,F).

Figure 6 Scanning electron micrographs (A–D) and photomicrographs (E–G) of larvae of B. gouldingi. 14 hpf: Larval hatching. (A) Distended posture, yolk sac (YS) and presence of embryonic fin (→). (B) Dorsal view of recently hatched larva showing the yolk sac (YS). (C) Detail of cephalic region of hatched larva showing rudimentary gill arches (*), optic vesicle (OP), otic vesicle (OT) and development of branchiostegal membrane (BM) and central nervous system (SNC). (D) Detail of olfactory placodes filled with ciliated cells. (E) Distended posture and presence of final portion of the digestive tract (highlighted), yolk sac (YS). (F) Detail of cephalic region of hatched larva: mouth primordium (MO), optic cup (OP), crystalline vesicle (CV) CNS, central nervous system. (G) Detail of hatched larva: differentiation of miomeres (→), visualization of pronephros (PRO) and intestine (IN).
At this moment, the larvae presented primordial gill arches while the primordium of the branchiostegal membrane, that eventually will cover such arches, was under formation (Fig. 6C). The olfactory placodes were seen as shallow rounded depressions filled with tiny rudimentary cilia (Fig. 6D). The digestive tract was undifferentiated, with only an epithelium indicating the region of the further development of the buccopharyngeal cavity (Fig. 6F), represented by a single cell layer throughout the extension of the yolk sac, that changed onto several undifferentiated cell layers where the intestine will develop (Fig. 6E). At this moment, the pronephros (rudimentary kidney) was being formed, right above the intestine (Fig. 6G). It was also possible to observe the differentiation of miomeres (muscular segment) (Fig. 6G).
Discussion
The presence of a single micropyle with a funnel-like vestibule and several rectilinear folds in the oocytes of B. gouldingi, is a common reproductive feature within species of the genus Brycon, as also reported by Ganeco & Nakaghi (Reference Ganeco and Nakaghi2003) and Ganeco et al. (Reference Ganeco, Franceschini-Vicentini and Nakaghi2008) in B. orbignyanus, Sampaio (Reference Sampaio2006) in B. orthotaenia and Neumann (Reference Neumann2008) in B. amazonius. The micropyle represents a small opening in the animal pole of the cell (Laale, Reference Laale1980), through which the spermatozoon penetrates the oocyte (Riehl, Reference Riehl1993).
According to Rizzo & Bazzoli (Reference Rizzo and Bazzoli1993), funnel-like micropyles are observed in most of teleosteans and allow the entrance of a single spermatozoon through the intern aperture.
Riehl (Reference Riehl1993) and Li et al. (2000) stated that the micropyle microstructure is an important criterion to characterize and identify fish eggs. Chen et al. (Reference Chen, Shao and Yang1999) also suggested that the micropyle can be an important tool in the identification of eggs and for phylogenetic studies in fishes, once it might present differences among species of the same genus or family, as a way to prevent inter-specific hybridization. However, some studies reported similarities between the micropyle apparatus in different species, indicating putative relationships among systematic groups (Rizzo et al., Reference Rizzo, Sato, Barreto and Godinho2002).
The sequence of events observed in the embryogenesis of the species studied in the present work is similar to that described for other Brycon species, like B. insignis (Andrade-Talmelli et al., Reference Andrade-Talmelli, Kavamoto, Romagosa and Fenerich-Verani2001), B. cephalus (Lopes et al., Reference Lopes, Senhorini and Soares1995; Romagosa et al., Reference Romagosa, Narahara and Fenerich-Verani2001), B. orbignyanus (Landinez et al., Reference Landinez, Senhorini, Sanabria, Baldan and Urbinati2004; Reynalte-Tataje et al., Reference Reynalte-Tataje, Zaniboni-Filho and Esquivel2004) and other teleosteans as well, such as the hybrid between P. corruscans and P. fasciatum (Faustino et al., Reference Faustino, Nakaghi, Marques, Makino and Senhorini2007), P. lineatus (Ninhaus-Silveira et al., Reference Ninhaus-Silveira, Foresti and Azevedo2006) and P. corruscans (Marques et al., Reference Marques, Nakaghi, Faustino, Ganeco and Senhorini2008), being differentiated only by the chronology of the events.
The presence of several spermatozoa in the micropyle vestibule from the moment the semen and the oocytes were mixed to 1 mpf suggested that fertilization had taken place within this interval. As some fertilization cones could be seen after 30 s, it is possible to infer that several eggs were rapidly fertilized. Kudo (Reference Kudo1980) reported that the time between gamete fusion and subsequent formation of the fertilization cone is usually very short. He also stated that this cone would be composed of a granular material with few cell organelles. Iwamatsu (Reference Iwamatsu, Tarin and Cano2000) observed that the fertilization cone is composed of perivitelline fluid released through the micropyle canal, forming a bubble. The beginning of formation of such cone occurs simultaneously to the exocytose of cortical alveoli (Iwamatsu & Ohta, Reference Iwamatsu and Ohta1981).
Right after the fertilization, the eggs of B. gouldingi became flattened. This behaviour was also observed by Neumann (Reference Neumann2008) in B. amazonicus. The author also relates such ‘compression’ to the cell movements to form the animal pole. The egg cleavages in B. gouldingi occurred only in the animal pole, while the vegetative pole was composed of yolk. Such division pattern is typical of fish eggs and it is known as meroblastic or partial, once it involves exclusively the animal pole (Balinsky, Reference Balinsky1970; Leme dos Santos & Azoubel, Reference Leme dos Santos and Azoubel1996).
The mitotic divisions during the cleavage take place in order to promote a new balance in the relationship between the nucleus and the cytoplasm, i.e., the high volume of the zygote cytoplasm is divided into increasingly smaller cells, while the cytoplasm volume does not increase (Gilbert, Reference Gilbert2003). This feature was visualized in B. gouldingi: as the number of blastomeres increased, their size decreased corroborating the reports by Wourms & Evans (Reference Wourms and Evans1974) and Castellani et al. (Reference Castellani, Tse, Santos, Faria and Santos1994), being possible to observe several events of mitotic divisions as previously reported by Marques (Reference Marques2008).
In the blastula stage, the differentiation of blastoderm (embryonic cells) and periblast could be detected. The periblast (also called yolk syncytial layer) plays an important role once, before taking exogenous food sources, the embryo/larvae feeds on the yolk which is constantly reabsorbed via yolk syncytial layer (Balinsky, Reference Balinsky1970). According to Kimmel et al. (Reference Kimmel, Ballard, Kimmel and Ullmann1995), this layer is an organ and it is found only in teleosteans, as an extra-embryonic structure, thereby not contributing to the embryo formation.
The appearance of periblast was also reported at blastula stage by other authors, such as Cardoso et al. (Reference Cardoso, Alves, Ferreira and Godinho1995) in P. coruscans, Ninhaus-Silveira et al. (Reference Ninhaus-Silveira, Foresti and Azevedo2006) in P. lineatus and González-Doncel et al. (Reference González-Doncel, Okihiro, Villalobos, Hinton and Tarazona2005) in O. latipes. However, it should be pointed out that the appearance of this layer was observed at morula stage by Long and Ballard (Reference Long and Ballard1976) in C. commersoni, by Matkovic et al. (Reference Matkovic, Cussac, Cukier, Guerrero and Maggese1985) in R. sapo and by Marques (Reference Marques2008) in Z. jahu.
The initialization of cell movement characterizes the epiboly (Warga & Kimmel, Reference Warga and Kimmel1990, Leme dos Santos, Reference Leme dos Santos and Azoubel1996). During gastrulation, the blastodisc cells are moved, differentiating the germ follicles, with the separation of epiblast and hypoblast and the beginning of notochord formation (Stickney et al., Reference Stickney, Barresi and Devoto2000). During epiboly, the epiblast and the yolk syncytial layer (periblast) expand over the yolk until the blastopore is closed (Kimmel et al., Reference Kimmel, Ballard, Kimmel and Ullmann1995), as observed in B. gouldingi and also in B. insignis (Andrade-Talmelli et al., Reference Andrade-Talmelli, Kavamoto, Romagosa and Fenerich-Verani2001), L. piau (Borçato et al., Reference Borçato, Bazzoli and Sato2004), and P. lineatus (Ninhaus-Silveira et al., Reference Ninhaus-Silveira, Foresti and Azevedo2006).
Following the development, comes the organogenesis/histogenesis stage. The formation of somites (somitogenesis stage) is necessary in the organization of the segmental pattern of vertebrate embryos. These structures are transitory muscular precursors that develop into cell blocks (Gilbert, Reference Gilbert2003).
The visualization of notochord in B. gouldingi occurred at this stage and, according to Gilbert (Reference Gilbert2003), it is a transitory organ whose main function is to induce the formation of the neural tube (further central nervous system) and establish the cephalic-caudal corporal axis. Most part of notochord degenerates, but the portion between the vertebras originates the tissue of inter-vertebral disks. According to Falk-Petersen (Reference Falk-Petersen2005), the notochord is composed of vacuolated cells separated by thin cell membranes.
In B. gouldingi, the anterior region of the neural tube was expanded, giving rise to anterior brain (prosencephalon), medium brain (mesencephalon) and posterior brain (rhombencephalon) as described in D. rerio by Kimmel et al. (Reference Kimmel, Ballard, Kimmel and Ullmann1995), G. morhua by Hall et al. (Reference Hall, Smith and Johnston2004), P. lineatus by Ninhaus-Silveira et al. (Reference Ninhaus-Silveira, Foresti and Azevedo2006) and by Marques et al. (Reference Marques, Nakaghi, Faustino, Ganeco and Senhorini2008) in P. corruscans.
In the present study, we observed the epiphysis, derived from diencephalon. This structure is the pineal primordium (Kimmel et al., Reference Kimmel, Ballard, Kimmel and Ullmann1995) and it appears on the upper limit of diencephalon (Hall et al., Reference Hall, Smith and Johnston2004). The cerebellum primordium was also identified in B. gouldingi and, according to Kimmel et al. (Reference Kimmel, Ballard, Kimmel and Ullmann1995) and Hall et al. (Reference Hall, Smith and Johnston2004); it is derived from the metencephalon, the most posterior portion of the rhombencephalon.
With the heart formation during the somitogenesis of the embryo, the development of the circulatory system is initiated, and it continues throughout the whole larval development (Langeland & Kimmel, Reference Langeland, Kimmel, Gilbert and Raunio1997; Yelon & Stainier, Reference Yelon and Stainier1999; Hu et al., Reference Hu, Sedmera, Post and Clark2000).
The heart primordium visualized in the anterior region of the yolk in B. gouldingi, has also been observed by several authors who described that the heart initially appears as a simple tube with a wall of several layers (Morrison et al., Reference Morrison, Miyake and Wright2001; Hall et al., Reference Hall, Smith and Johnston2004).
After the organogenesis/histogenesis stage follows the larval hatching. Most freshwater fish larvae hatch with non-pigmented eyes, large yolk sac and underdeveloped jaw and mouth (Nakatani et al., Reference Nakatani, Agostinho, Baumgartner, Bialetzki, Sanches and Cavicchioli2001), just like the larvae of B. gouldingi. Furthermore, the B. gouldingi larvae lacked fin primordia (only an embryonic fin surrounded the whole tail) and the rudimentary digestive tract without oral and anal apertures at hatching. According to Önal et al. (2009), larvae that hatch with a large yolk sac and undifferentiated digestive tract, such as B. gouldingi, can be classified as altricial larvae.
The information obtained in the present work is described for the first time as being important because several biological traits were characterized about this new Brycon species, endemic to the Brazilian Tocantins-Araguaia basin, with great interest to fish farmers for captivity rearing as a food source to many riverine communities, but threatened by several projects of dam constructions for hydroelectric power along the basin. The present data are useful to elucidate questions on the rearing of Brycon gouldingi under laboratory conditions during the embryonic stage, besides being a tool for taxonomic, ecological and conservationist studies in this species.
Acknowledgements
The authors are grateful to the Buriti fish farm for providing the biological material; to Mr Orandi Mateus, the technician in the Histology and Embryology Laboratory and Ms Cláudia Aparecida Rodrigues, the technician in the Electron Microscopy Laboratory (both at FCAV/UNESP) for assistance in sample processing; and to FAPESP for the financial support with the master scholarship granted (2007/57826-7); and CNPq (473712-2007-5).