Introduction
BMP active compounds have originally been identified in demineralized bone extracts that were capable to induce bone formation in ectopic sites (Urist, 1965). BMP family proteins have amino acid sequences similar to members of the TGF-β superfamily, which has over 35 members (Chang et al., Reference Chang, Brown and Matzuk2002). BMPs can be classified into several subgroups, including the BMP-2 and -4 group, the BMP-5, -6, -7 and -8 group and the BMP-9, -10 and -15 group (Ducy & Karsenty, Reference Ducy and Karsenty2000). The pattern of BMP expression has been described in various tissues and their proteins have become commercially available. It has been demonstrated in recent studies in vitro that BMP-2, -4 and -8 control primordial germ cell (PGC) formation (Dudley et al., Reference Dudley, Palumbo, Nalepka and Molyneaux2010), while BMP-6, -7 and -15 regulate primordial follicle activation and viability (Araújo et al., Reference Araújo, Lima-Verde, Name, Bao, Campello, Silva, Rodrigues and Figueiredo2010b; Celestino et al., Reference Celestino, Lima-Verde, Bruno, Matos, Chaves, Saraiva, Silva, Faustino, Rossetto, Lopes, Donato, Peixoto, Campello, Silva and Figueiredo2011), growth of secondary follicles (Frota et al., Reference Frota, Leitão, Costa, van den Hurk, Brito, Saraiva, Figueiredo and Silva2011; Passos et al., Reference Passos, Vasconcelos, Silva, Brito, Saraiva, Magalhães, Costa, Donato, Ribeiro, Cunha, Peixoto, Campello, Figueiredo, van den Hurk and Silva2013) and maturation of antral follicles (Shimasaki et al., Reference Shimasaki, Moore, Otsuka and Erickson2004). Several BMPs have been described as autocrine and/or paracrine factors that regulate the development of ovarian follicle development.
Folliculogenesis is regulated by a delicate balance between endocrine and intra-ovarian factors (Artini et al., Reference Artini, Monteleone, Toldin, Matteucci, Ruggiero, Cela and Genazzani2007). Oocyte growth and maturation within pre-antral and antral follicle stages, respectively, are dependent on intra-ovarian factors, which in antral stages particularly are factors present in follicular fluid (Hsieh et al., Reference Hsieh, Zamah and Conti2009; Padhy et al., Reference Padhy, Sathya and Varma2009). These follicular fluid factors are positively related to levels of these factors in blood serum (Qiao & Feng, Reference Qiao and Feng2011). Any imbalance or dysfunction among intra-ovarian factors can result in abnormal folliculogenesis and disorder in oocyte growth and maturation (Frank et al., Reference Frank, Stark and Hardy2008). Altered balance of different factors, along with the intrafollicular fluid microenvironment may directly impair the potential development of the oocyte (Artini et al., Reference Artini, Monteleone, Toldin, Matteucci, Ruggiero, Cela and Genazzani2007; Padhy et al., Reference Padhy, Sathya and Varma2009), which would have a negative impact on fertilization, embryonic development and evolution of pregnancy using oocytes of antral follicles in in vitro fertilization systems (Qiao & Feng, Reference Qiao and Feng2011).
In this report, we discuss the roles of the BMP system in regulating mammalian ovarian functions. We will focus on four main areas: (1) the structure of the BMP ligands and receptors; (2) their expression in the ovary; (3) BMP signal transduction and regulation of action; and (4) the role of BMPs on oogenesis and folliculogenesis.
BMP family members
According to Shimasaki et al. (Reference Shimasaki, Moore, Otsuka and Erickson2004), the BMP family comprises 18 members. As the discovery of the same or similar genes occurred simultaneously in different groups, different names were given for the same family of genes related to BMP as shown in Table 1. BMPs are regulatory in a series of physiological processes directly related to the functions of ovarian follicles at different stages of development.
Table 1 Members of the BMP family and their alternative names
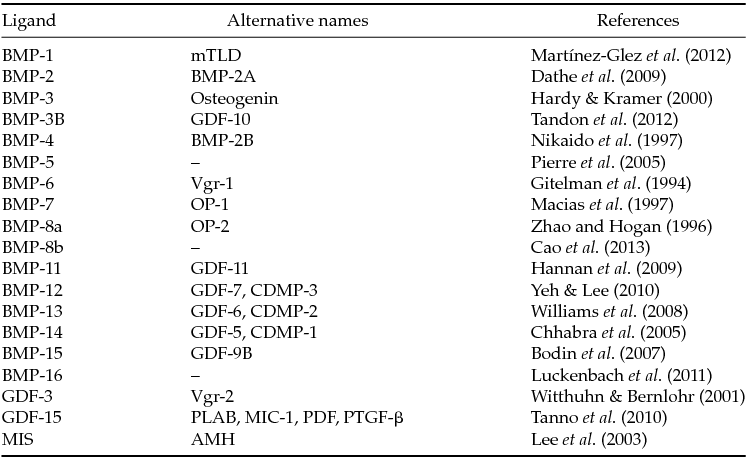
AMH: anti-Müllerian hormone; CDMP: cartilage-derived morphogenetic protein; GDF: growth differentiation factor; MIC: macrophage inhibiting cytokine; mTLD: mammalian Tolloid protein; MIS: Müllerian inhibiting substance; OP: osteogenic protein; PDF: prostate-derived fator; PLAB: placental bone morphogenetic protein; PTGF-β: placental TGF-β; Vgr: Vg-related protein;
Among members of the TGF-β superfamily, BMP stands out among the intra-ovarian factors. A structural feature of members of this superfamily is the presence of seven conserved cysteines, which are involved in the folding of the molecule into a single three-dimensional structure called a cystine knot (reviewed by Vitt et al., Reference Vitt, Hsu and Hsueh2001), the conserved cysteine residue that is not involved in the formation of cystine knot makes a disulfide bound between the two subunits. This results in the formation of covalently bound dimer, which allows the biological activity of these proteins (Wang et al., Reference Wang, Rosen, D’Alessandro, Bauduy, Cordes, Harada, Israel, Hewick, Kerns, Lapan, Luxenberg, McQuaid, Moutsatsos, Nove and Wozney1990).
Members of the TGF-β superfamily are synthesized as precursor proteins that are composed of a signal sequence amino-terminal domain and a pro-mature domain (carboxy-terminal domain). The amino-terminal signal directs the precursor to a signalling pathway, while the pro-domain variable can facilitate dimerization. The signalling of the TGF-β superfamily is regulated at multiple levels, including connection and processing of extracellular ligand interactions and intracellular receptors (Kingsley, Reference Kingsley1994; Bragdon et al., Reference Bragdon, Moseychuk, Saldanha, King, Julian and Nohe2011).
The BMP family members act through a family of transmembrane receptor, serine/threonine kinase receptors. Based on their structural and functional properties, the BMP receptors are divided into two subfamilies: receptors type I and type II. Receptors type I and II are glycoproteins of approximately 55 kDa and 70 kDa, respectively. The extracellular regions of these receptors contain about 150 amino acids with 10 or more cysteines that determine the folding area. A unique feature of type I receptors is an intracellular region of 30 amino acids immediately preceding the kinase domain. This stretch of 30 amino acids is called the GS (glycine- and serine-rich sequence) domain because of the sequence SGSGSG contained in this region (Wrana et al., Reference Wrana, Attisano, Wieser, Ventura and Massagué1994).
The type I receptors have received different names, for example, similar to the activin receptor kinase 4 (ALK-4) (Attisano et al., Reference Attisano, Wrana, Montalvo and Massagué1996). Due to the promiscuity of the type I receptor for different ligands of the TGF-β superfamily in most cases, the most suitable nomenclature is probably ALK. Receptors for various BMPs and their nomenclature are listed in Table 2.
Table 2 Ligands, receptors and SMADs of the BMP family

BMP signal transduction
To exert their biological functions, BMPs bind to BMP receptor IA or IB types, inducing its activation. Then, BMPRII is recruited to promote phosphorylation of BMPRIA or BMPRIB. The binding induces phosphorylation of the GS domain in the type I receptor (Wieser et al., Reference Wieser, Wrana and Massagué1995).
Although receptors type I and type II participate in the transduction of intracellular signalling of BMPs, the receptor binding and activity of certain ligands are regulated by co-receptors. The glycosylphosphatidylinositol (GPI) protein family members linked to repulsive molecular orientation (RGM), which includes the RGM-a, -b and -c, are co-receptors for BMP-2 and BMP-4 and reinforce the signalling of BMPs (Samad et al., Reference Samad, Rebbapragada, Bell, Zhang, Sidis, Jeong, Campagna, Perusini, Fabrizio, Schneyer, Lin, Brivanlou, Attisano and Woolf2005). Xia et al. (Reference Xia, Yu, Sidis, Beppu, Bloch, Schneyer and Lin2007) stated that RGM-b, also known as hemojuvelin and DRAGON, respectively, interacts with BMP receptors of which type I or type II, bind to BMP-2 and BMP-4, but not BMP-7 and TGF-β1. However, cells transfected with the RGM use both BMPRII and ActR-II for signalling BMP-2/4, suggesting that RGM facilitates the use of ActR-II by BMP-2/4.
SMAD proteins are components of intracellular signal transduction pathways of the TGF-β superfamily. The first member of this family is the protein Mothers Against Decapentaplegic (MAD) that has been identified in Drosophila melanogaster (Sekelsky et al., Reference Sekelsky, Newfeld, Raftery, Chartoff and Gelbart1995). Other members of this family have been identified based on their homology with MAD. Three MAD counterparts found in Caenorhabditis elegans are named as SMA-2, SMA-3, SMA-4 and mutation in their respective gene prevents development (Savage et al., Reference Savage, Das, Finelli, Townsend, Sun, Baird and Padgett1996). Homologues of SMA and MAD found in vertebrates are called SMADs, a combination of SMA and MAD (Derynck et al., Reference Derynck, Gelbart, Harland, Heldin, Kern, Massagué, Melton, Mlodzik, Padgett, Roberts, Smith, Thomsen, Vogelstein and Wang1996). At least 10 vertebrate SMAD proteins have been identified so far (Massagué & Wotton, Reference Massagué and Wotton2000).
The interaction between receptors induces phosphorylation of intracellular messengers (SMAD types 1, 5 and 8), which form complexes with SMAD co-regulator (SMAD-4), that accumulate in the nucleus and act as transcription factors by binding directly to specific binding sites in the promoters of a target gene (Ohta et al., Reference Ohta, Nakagawa, Imai, Katagiri, Koike and Takaoka2008) or to other transcription factors (Lembong et al., Reference Lembong, Yakoby and Shvartsman2008), activating or inhibiting the expression of a gene (Massagué et al., Reference Massagué, Seoane and Wotton2005). Figure 1 shows that BMP receptors I and II interaction induces phosphorylation of intracellular messengers (SMADs) that are moved to the nucleus to regulate the expression of specific genes.

Figure 1 The interaction between BMP receptors I and II induces phosphorylation of intracellular messengers (SMADs) that are moved to the nucleus to regulate the expression of specific genes.
BMP signalling can be monitored using antibodies that recognize the phosphorylated SMADs, because the signalling pathway is presented in a highly conserved manner across all species (Lembong et al., Reference Lembong, Yakoby and Shvartsman2008). The subcellular localization of phospho-SMAD1/5 passes from the nucleus to the cytoplasm during differentiation of PGCs in oogonia (Childs et al., Reference Childs, Kinnell, Collins, Hogg, Bayne, Green, McNeilly and Anderson2010). Mutations in SMAD-2, -3 and -4 were found in human tumors, suggesting that these genes function as tumor suppressors in vivo (Massagué, Reference Massagué1998). For example, human SMAD-4 is also known as DPC4 (deleted in pancreatic carcinoma locus 4), which is often deleted or mutated in pancreatic cancer (Hahn et al., Reference Hahn, Schutte, Hoque, Moskaluk, Costa, Rozenblum, Weinstein, Fischer, Yeo, Hruban and Kern1996).
Since the discovery of expression of BMP family members in ovarian cells (Israel et al., Reference Israel, Nove, Kerns, Moutsatsos and Kaufman1992), many studies have been performed to demonstrate the importance of BMP system in regulating reproductive processes (Childs et al., Reference Childs, Kinnell, Collins, Hogg, Bayne, Green, McNeilly and Anderson2010; Otsuka et al., Reference Otsuka, McTavish and Shimasaki2011). At the extracellular level, BMP antagonists (e.g. noggin) interfere with ligand binding to the receptors of BMPs, as noggin binds to BMP receptors. The expression of noggin is potentially induced by the activity of BMPs and thus may contribute to the negative feedback mechanism controlling the action of BMPs (Song et al., Reference Song, Krause, Shi, Patterson, Suto, Grgurevic, Vukicevic, van Dinther, Falb, Ten Dijke and Alaoui-Ismaili2010), and inhibiting the growth of PGCs in culture systems (Ying & Zhao, Reference Ying and Zhao2001).
Expression of BMP receptors in the ovary
Several studies have reported the mRNA expression of BMP receptors in different follicular compartments of pre-antral and antral follicles of ruminants (bovine: Fatehi et al., Reference Fatehi, van den Hurk, Colenbrander, Daemen, van Tol, Monteiro, Roelen and Bevers2005; ovine: Wilson et al., Reference Wilson, Wu, Juengel, Ross, Lumsden and Lord2001, Souza et al., Reference Souza, Campbell, McNeilly and Baird2002; caprine: Lima et al., Reference Lima, Brito, Rossetto, Duarte, Rodrigues, Saraiva, Costa, Donato, Peixoto, Silva, Figueiredo and Rodrigues2012). In the ovaries of murine (Erickson & Shimasaki, Reference Erickson and Shimasaki2003) and caprine (Silva et al., Reference Silva, van den Hurk, van Tol, Roelen and Figueiredo2005), the expression of mRNA for BMPRII was demonstrated in oocytes and granulosa cells from primordial, primary, secondary and antral follicles. In goat antral follicles, the mRNA level of BMPRII showed a peak in 0.5 mm follicles, when compared with those of 0.2 and 1.0 mm (Costa et al., Reference Costa, Passos, Leitao, Vasconcelos, Saraiva, Figueiredo, van den Hurk and Silva2012). Higher levels of BMPRII may potentiate the action of BMP-15 and GDF-9 in antral follicles, as these factors bind to BMPRII with high affinity (Edwards et al., Reference Edwards, Reader, Lun, Western, Lawrence, McNatty and Juengel2008). However, the expression of BMPRII and activin receptor-like kinase type 5 (ALK-5) in caprine follicles cultured in vitro is downregulated by follicle-stimulating hormone (FSH) (Frota et al., Reference Frota, Leitão, Costa, van den Hurk, Brito, Saraiva, Figueiredo and Silva2011; Costa et al., Reference Costa, Passos, Leitao, Vasconcelos, Saraiva, Figueiredo, van den Hurk and Silva2012).
The mRNA for BMPRIB was reported in ovaries of murine (Erickson & Shimasaki, Reference Erickson and Shimasaki2003) and goats (Silva et al., Reference Silva, van den Hurk, van Tol, Roelen and Figueiredo2005), especially in oocytes, granulosa and theca cells from all classes of follicles. The mRNA level of BMPRIB was highest in goat ovarian follicles of 0.2 mm in relation to those with 1.0 mm (Costa et al., Reference Costa, Passos, Leitao, Vasconcelos, Saraiva, Figueiredo, van den Hurk and Silva2012). Edwards et al. (Reference Edwards, Reader, Lun, Western, Lawrence, McNatty and Juengel2008) showed that BMP-4 binds to BMPRIB. With regard to BMP-15, it was found that BMP-15 could bind to multiple receptors in granulosa cells, i.e. BMPRII, activin type II receptor, ALK-2 and ALK-6. Among these receptors, ALK-6 is the most efficient in binding to BMP-15, while BMPRII is more effective in the bioactivity of BMP-15 (Wu et al., Reference Wu, Wang, Chen, Zhu, Dong, Sheng, Xu and Huang2012). Thus, BMP-15 signalling may be mediated by initial binding with ALK-6, which recruits BMPRII (Edwards et al., Reference Edwards, Reader, Lun, Western, Lawrence, McNatty and Juengel2008). In human, BMPRIA, BMPRIB, and BMPRII receptors were detectable in granulosa cells (Khalaf et al., Reference Khalaf, Morera, Bourret, Reznik, Denoual, Herlicoviez, Mittre and Benhaim2013). In addition, Kristensen et al. (Reference Kristensen, Andersen, Clement, Franks, Hardy and Andersen2014) observed that, comparing transcript abundance in five size-matched populations of isolated pre-antral follicles, BMPRII is the most expressed receptors. Recently, a study in humans found that type 1 receptors ALK-3, ALK-4, ALK-5 and ALK-6 are expressed at moderate levels. In comparison, ALK-1, ALK-2, and ALK-7; are generally expressed at a low level in the human pre-antral follicles (Kristensen et al., Reference Kristensen, Andersen, Clement, Franks, Hardy and Andersen2014). Table 3 shows the sites of expression of BMP receptors in ovarian follicles.
Table 3 Locations of expression of BMP receptors in mammalian ovarian follicles

Murine (Erickson & Shimasaki, Reference Erickson and Shimasaki2003; Edwards et al., Reference Edwards, Reader, Lun, Western, Lawrence, McNatty and Juengel2008); caprine (Silva et al., Reference Silva, van den Hurk, van Tol, Roelen and Figueiredo2005; Lima et al., Reference Lima, Brito, Rossetto, Duarte, Rodrigues, Saraiva, Costa, Donato, Peixoto, Silva, Figueiredo and Rodrigues2012); bovine (Fatehi et al., Reference Fatehi, van den Hurk, Colenbrander, Daemen, van Tol, Monteiro, Roelen and Bevers2005; Selvaraju et al., Reference Selvaraju, Folger, Gupta, Ireland and Smith2013); ovine (Wilson et al., Reference Wilson, Wu, Juengel, Ross, Lumsden and Lord2001; Souza et al., Reference Souza, Campbell, McNeilly and Baird2002).
Expression of BMP ligands and their role in oogenesis and folliculogenesis
The expression of BMPs in ovarian tissue has been reported in various studies. Table 4 shows the sites of expression of BMP family members in ovarian follicles. In human, it was demonstrated that BMP-2, BMP-4, BMP-5, BMP-6, BMP-7, and BMP-8A are expressed in ovarian granulosa cells from healthy ovulatory women (Khalaf et al., Reference Khalaf, Morera, Bourret, Reznik, Denoual, Herlicoviez, Mittre and Benhaim2013). Recently, in a study with human follicles that compared transcript abundance in five size-matched populations of isolated pre-antral follicles, BMP-15 and BMP-6 were the most expressed ligands. By comparison, BMP-1, BMP-2, BMP-3, BMP-4 and BMP-8B were generally expressed at a low level, whereas BMP-5, BMP-7, BMP-8A and BMP-10 were undetectable in the human pre-antral follicles (Kristensen et al., Reference Kristensen, Andersen, Clement, Franks, Hardy and Andersen2014).
Table 4 Locations of expression of BMP family members in mammalian ovarian follicles

Rat (Erickson & Shimasaki, Reference Erickson and Shimasaki2003; Pierre et al., Reference Pierre, Pisselet, Dupont, Bontoux and Monget2005; Juengel et al., Reference Juengel, Reader, Bibby, Lun, Ross, Haydon and McNatty2006); bovine (Glister et al., Reference Glister, Kemp and Knight2004; Fatehi et al., Reference Fatehi, van den Hurk, Colenbrander, Daemen, van Tol, Monteiro, Roelen and Bevers2005); ovine (Juengel et al., Reference Juengel, Reader, Bibby, Lun, Ross, Haydon and McNatty2006; Canty-Laird et al., Reference Canty-Laird, Carré, Mandon-Pépin, Kadler and Fabre2010); caprine (Silva et al., Reference Silva, van den Hurk, van Tol, Roelen and Figueiredo2005; Frota et al., Reference Frota, Leitão, Costa, van den Hurk, Saraiva, Figueiredo and Silva2013); human (Abir et al., 2008; Sugiyama et al., 2010; Wu et al., Reference Wu, Wang, Chen, Zhu, Dong, Sheng, Xu and Huang2012).
BMP-1, -2 and -4
In ovine ovaries, immunohistochemical localization demonstrated that BMP-1 was present in granulosa cells at all stages of follicular development, from primordial to large antral follicles, and that the levels of BMP-1 were not affected by the final follicle selection mechanism (Canty-Laird et al., Reference Canty-Laird, Carré, Mandon-Pépin, Kadler and Fabre2010). BMP-1 belongs to the family of metalloproteases and, through its procollagen C-proteinase activity, it can regulate the formation of extracellular matrix (ECM) during ovarian folliculogenesis (Canty-Laird et al., Reference Canty-Laird, Carré, Mandon-Pépin, Kadler and Fabre2010). The importance of the ECM for follicular development is exemplified by the observation that both the growth and atresia of ovine ovarian follicles are associated with dramatic changes in the composition of the collagenous ECM in which the theca and granulosa cells are embedded (Huet et al., Reference Huet, Monget, Pisselet and Monniaux1997, Reference Huet, Monget, Pisselet, Hennequet, Locatelli and Monniaux1998; Berkholtz et al., Reference Berkholtz, Lai, Woodruff and Shea2006).
Expression of mRNAs for BMP-2 was reported in granulosa cells of primary, secondary and antral follicles in murine ovaries (Erickson & Shimasaki, Reference Erickson and Shimasaki2003), as well as in theca cells of bovine antral follicles (Fatehi et al., Reference Fatehi, van den Hurk, Colenbrander, Daemen, van Tol, Monteiro, Roelen and Bevers2005). According to Juengel et al. (Reference Juengel, Reader, Bibby, Lun, Ross, Haydon and McNatty2006), antral follicles in ovine ovaries express BMP-2 mRNA, present in granulosa cells. BMP-2 interacts with BMP-4 and has an additive effect on stimulating PGC generation in mice (Ying & Zhao, Reference Ying and Zhao2001). Studies that evaluate the effects of BMP-2 on early folliculogenesis are scarce, but in vitro studies with granulosa cells from ovine antral follicles have shown that BMP-2 increases the production of estrogen and inhibin-A after stimulation with FSH, thus promoting the in vitro differentiation of granulosa cells (Souza et al., Reference Souza, Campbell, McNeilly and Baird2002). However, BMP-2 suppresses the synthesis of estradiol and androstenedione, while progesterone was shown to stimulate proliferation of porcine theca cells (Brankin et al., Reference Brankin, Quinn, Webb and Hunter2005).
BMP-4 mRNA and protein are expressed in murine theca cells (Erickson & Shimasaki, Reference Erickson and Shimasaki2003), as well as in granulosa, theca cells (Glister et al., Reference Glister, Kemp and Knight2004) and oocytes from antral follicles in bovine species (Fatehi et al., Reference Fatehi, van den Hurk, Colenbrander, Daemen, van Tol, Monteiro, Roelen and Bevers2005). Several studies have demonstrated that BMP-4 is the main regulator of PGC formation in mammals (reviewed by Saitou & Yamaji, Reference Saitou and Yamaji2010). Analysis by real-time PCR showed that expression of BMP-4 increases during the transition from the PGC proliferation stage to the meiotic stage of differentiation (Lawson et al., Reference Lawson, Dunn, Roelen, Zeinstra, Davis, Wright, Korving and Hogan1999; Ying et al., Reference Ying, Liu, Marble, Lawson and Zhao2000; Ross et al., Reference Ross, Tilman, Yao, MacLaughlin and Capel2003). Additionally, BMP-4 causes a significant increase in expression of genes in PGCs and appears to be required for differentiation of human embryonic stem cells into PGCs (West et al., Reference West, Roche-Rios, Abraham, Rao, Natrajan, Bacanamwo and Stice2010). BMP-4 furthermore promotes proliferation of PGCs in mouse (Ross et al., Reference Ross, Tilman, Yao, MacLaughlin and Capel2003) and human (Childs et al., Reference Childs, Kinnell, Collins, Hogg, Bayne, Green, McNeilly and Anderson2010) gonads, and acts as a regulator of gametogenesis in multiple stages of development.
The earliest stages of folliculogenesis are also regulated by BMP-4 (Nilsson & Skinner, Reference Nilsson and Skinner2003). Ding et al. (Reference Ding, Zhang, Mu, Li and Hao2013) showed that BMP-4/SMAD signalling pathway initiates primordial follicle growth and prevents oocyte apoptosis. Moreover, BMP-4 was found to contribute in the reduction of the apoptosis levels of granulosa cells cultured in vitro. In this respect, BMP-4 stimulated the expression of survivin mRNA, but did not affect the expression of B-cell lymphoma-extra large (bcl-xL) or B-cell lymphoma-2-associated X (bax), which are pro-apoptotic genes (Kayamori et al., Reference Kayamori, Kosaka, Miyamoto and Shimizu2009). BMP-4 also acts as an inhibitor of premature luteinization of granulosa cells from antral follicles enabling the required growth to become preovulatory follicles (Shimasaki et al., Reference Shimasaki, Moore, Otsuka and Erickson2004). Regarding the effects of BMP-4 in the final stages of oocyte maturation, Fatehi et al., (Reference Fatehi, van den Hurk, Colenbrander, Daemen, van Tol, Monteiro, Roelen and Bevers2005) reported that addition of BMP-4 or BMP-2 during in vitro maturation of bovine COCs does not affect the process of cumulus cell expansion and oocyte maturation, as well as the formation and quality of blastocysts after in vitro fertilization. However, during culture of granulosa cells from antral follicles, BMP-4 potentiates the action of FSH by increasing the production of estradiol and inhibiting the synthesis of progesterone (rat: Shimasaki et al., Reference Shimasaki, Zachow, Li, Kim, Iemura, Ueno, Sampath, Chang and Erickson1999; ovine: Mulsant et al., Reference Mulsant, Lecerf, Fabre, Schibler, Monget, Lanneluc, Pisselet, Riquet, Monniaux, Callebaut, Cribiu, Thimonier, Teyssier, Bodin, Cognié, Chitour and Elsen2001). In addition, BMP-2 and -4 reduced the mRNA expression of inhibitory SMAD-6 and -7 in cultured rat granulosa cells, leading to enhancement of BMP actions detected by DNA binding proteins of type 1 (Id-1) transcription (West et al., Reference West, Roche-Rios, Abraham, Rao, Natrajan, Bacanamwo and Stice2010). These latter results revealed that ovarian steroidogenesis was modulated by specific somatostatin receptor (SSTR) activation, which occurs by upregulating endogenous BMP activity in growing follicles.
At different levels, the BMPs signalling pathway is controlled by positive and negative regulators. BMP-4 interacts with activin and GnRH to modulate the secretion of FSH in gonadotropic cells (Lee et al., Reference Lee, Khivansara, Santos, Lamba, Yuen, Sealfon and Bernard2007; Nicol et al., Reference Nicol, Faure, McNeilly, Fontaine, Taragnat and McNeilly2008). Ho & Bernard, (2009) demonstrated that BMP-2 induces phosphorylation of SMAD-1/-5 and promotes an increase in the expression of the β subunit of FSH in these cells. At different concentrations, BMP-4 increases FSH-induced estradiol production in rat granulosa cells and does not alter forskolin-induced estradiol production. Moreover, BMP-4 inhibits the FSH-induced progesterone production. However, the latter effects were not detected when rat granulosa cells were co-cultured with oocytes. In co-cultures of rat granulosa cells with oocytes both BMP-2 and BMP-4 inhibit synthesis of progesterone and estradiol, and stimulate synthesis of the p38-mitogen-activated protein kinase (p38MAPK) pathway by suppressing cAMP (Inagaki et al., Reference Inagaki, Otsuka, Miyoshi, Yamashita, Takahashi, Goto, Suzuki and Makino2009).
In human granulosa cells there is an interrelationship between the BMP system and FSH. BMPs initially inhibit expression of the FSH receptors, while FSH stimulates endogenous BMP signalling. This balance is important for in vitro maintenance and development of granulosa cells (Miyoshi et al., Reference Miyoshi, Otsuka, Suzuki, Takeda, Inagaki, Kano, Otani, Mimura, Ogura and Makino2006). Table 5 shows the effects of BMP-1, -2 and -4 on oogenesis and folliculogenesis in the different mammalian species.
Table 5 Effects of BMP-1, -2 and -4 on oogenesis and folliculogenesis in the different mammalian species

Murine (Shimasaki et al., Reference Shimasaki, Zachow, Li, Kim, Iemura, Ueno, Sampath, Chang and Erickson1999; Lawson et al., Reference Lawson, Dunn, Roelen, Zeinstra, Davis, Wright, Korving and Hogan1999; Ying et al., Reference Ying, Liu, Marble, Lawson and Zhao2000; Ross et al., Reference Ross, Tilman, Yao, MacLaughlin and Capel2003; Ho & Bernard, Reference Ho and Bernard2009; Inagaki et al., Reference Inagaki, Otsuka, Miyoshi, Yamashita, Takahashi, Goto, Suzuki and Makino2009; West et al., Reference West, Roche-Rios, Abraham, Rao, Natrajan, Bacanamwo and Stice2010); bovine (Fatehi et al., Reference Fatehi, van den Hurk, Colenbrander, Daemen, van Tol, Monteiro, Roelen and Bevers2005); ovine (Mulsant et al., Reference Mulsant, Lecerf, Fabre, Schibler, Monget, Lanneluc, Pisselet, Riquet, Monniaux, Callebaut, Cribiu, Thimonier, Teyssier, Bodin, Cognié, Chitour and Elsen2001; Souza et al., Reference Souza, Campbell, McNeilly and Baird2002); human (Miyoshi et al., Reference Miyoshi, Otsuka, Suzuki, Takeda, Inagaki, Kano, Otani, Mimura, Ogura and Makino2006; Childs et al., Reference Childs, Kinnell, Collins, Hogg, Bayne, Green, McNeilly and Anderson2010); porcine (Brankin et al., Reference Brankin, Quinn, Webb and Hunter2005).
BMP-5, -6, -7 and -8
BMP-5 is expressed in porcine and murine ovaries (Shimizu et al., Reference Shimizu, Yokoo, Miyake, Sasada and Sato2004; Pierre et al., Reference Pierre, Pisselet, Dupont, Bontoux and Monget2005). Pierre et al. (Reference Pierre, Pisselet, Dupont, Bontoux and Monget2005) studied the expression of BMP-5 in rat ovaries by in situ hybridization, and found that mRNA was mainly expressed in granulosa and cumulus cells of early to large antral follicles from both healthy and atretic follicles; whereas low expression was detected in oocytes of small pre-antral follicles. In early follicles, BMP-5 is assumed to be involved in the development of secondary follicles but not primary (Shimizu et al., Reference Shimizu, Yokoo, Miyake, Sasada and Sato2004). In mice, BMP-5 enhanced the proliferation of granulosa cells from antral follicles, which was associated with an increase in cyclin D2 expression (Pierre et al., Reference Pierre, Pisselet, Dupont, Bontoux and Monget2005). Additionally, these authors also showed BMP-5 caused a marked dose-dependent inhibition of the FSH-induced progesterone production.
It has been demonstrated that BMP-6 is present in oocytes of all follicular categories in different species (murine: Erickson & Shimasaki, Reference Erickson and Shimasaki2003; ovine: Juengel et al., Reference Juengel, Reader, Bibby, Lun, Ross, Haydon and McNatty2006; cattle: Glister et al., Reference Glister, Kemp and Knight2004; goats: Frota et al., Reference Frota, Leitão, Costa, van den Hurk, Saraiva, Figueiredo and Silva2013), as well as in granulosa and theca cells of several species (murine: Erickson & Shimasaki, Reference Erickson and Shimasaki2003; ovine: Juengel et al., Reference Juengel, Reader, Bibby, Lun, Ross, Haydon and McNatty2006; cattle: Glister et al., Reference Glister, Kemp and Knight2004). In caprine species, the levels of mRNA for BMP-6 in primary and secondary follicles are significantly higher than those observed in primordial follicles (Frota et al., Reference Frota, Leitão, Costa, van den Hurk, Saraiva, Figueiredo and Silva2013). In this same species, addition of BMP-6 to culture medium promoted atresia in primordial follicles in organic culture (Araújo et al., Reference Araújo, Lima-Verde, Name, Bao, Campello, Silva, Rodrigues and Figueiredo2010b). Additionally, in in vitro cultured caprine secondary follicles, BMP-6, FSH and a combination of these compounds significantly increase follicular diameter and antrum formation (Frota et al., Reference Frota, Leitão, Costa, van den Hurk, Saraiva, Figueiredo and Silva2013). In swine, in bigger follicles, Brankin et al. (Reference Brankin, Quinn, Webb and Hunter2005) reported that BMP-6 maintained the theca cell viability during a culture period of 6 days. However, differences in cell types and animal species must be considered to understand the modes of BMP-6 action.
BMP-6 acts on bovine granulosa cells in vitro by stimulating their proliferation, promoting their viability and increasing their production of inhibin-A, activin-A and follistatin (Glister et al., Reference Glister, Kemp and Knight2004). BMP-6 treatment reduces the secretion of forskolin-induced progesterone by cultured granulosa cells and luteinized theca cells in vitro, in a dose-dependent manner, without affecting the number of viable cells at the end of the growing period. Furthermore, treatment of cultured granulosa cells with BMP-6 decreased levels of forskolin-induced cytochrome P450 family, but had no significant effects on transcription levels of StAR and 3β-hydroxysteroid dehydrogenase/isomerase (HSD3B1) in the presence or absence of forskolin. BMP-6 also increased basal expression of cytochrome P450 mRNA. Follistatin partially reverses the suppressive effect of BMP-6 on the secretion of progesterone in granulosa cells and theca cells. Additionally, BMP-6 decreases the secretion of activin-A in presence of forskolin (Kayani et al., Reference Kayani, Glister and Knight2009).
In mouse granulosa cells, BMP-6 inhibits the expression of LH receptors and progesterone synthesis, through inhibition of steroidogenic enzymes (Otsuka et al., Reference Otsuka, Moore and Shimasaki2001). BMP-6 acts by retarding the follicular differentiation process, providing the rapid growth of follicles through the multiplication of granulosa cells. This growth factor decreases drastically during the selection of the dominant follicle, the reduction possibly being related to the mechanism by which dominant follicles are selected (Shimasaki et al., Reference Shimasaki, Moore, Otsuka and Erickson2004). Administration of BMP-6 to aged female mice during superovulation significantly stimulates ovarian mRNA and protein expression of vascular endothelial growth factor (VEGF), which is involved in angiogenesis (Park et al., Reference Park, Park, Joo, Joo, Son and Lee2012). Developing follicles require a higher nutrient availability carried by newly vessels, formed from the previous stimulation of angiogenic factors, such as VEGF. Thus, during ovulation induction, BMP-6 plays an important role in the improvement of oocyte quality and ovarian response of aged female, possibly by regulating of ovarian VEGF expression. Moreover, BMP-6 might play a role in ovulation by increasing the accumulation of neutrophils in the ovulatory follicle and suppressing the effect of protease inhibitors (Akiyama et al., Reference Akiyama, Yoshino, Osuga, Shi, Takamura, Harada, Koga, Hirota, Hirata, Fujii and Saito2014).
BMP-7 is produced by theca cells of secondary and antral follicles (Shimasaki et al., Reference Shimasaki, Moore, Otsuka and Erickson2004). Human fetal follicles also express the mRNA for BMP-7 in granulosa cells, oocytes and oogonia (Abir et al., Reference Abir, Ben-Haroush, Melamed, Felz, Krissi and Fisch2008). In goat antral follicles, the levels of BMP-7 mRNA are significantly higher in mural granulosa/theca cells from large antral follicles (>3 mm) than those observed in small antral follicles (<3 mm) (Frota et al., Reference Frota, Leitão, Costa, van den Hurk, Saraiva, Figueiredo and Silva2013). In follicles of murine species, however, the mRNA expression of BMP-7 was restricted to granulosa cells (Lee et al., Reference Lee, Yoon, Yoon, Cha, Lee, Shimasaki, Lee and Lee2004), but in ovine follicles, BMP-7 was detected in all follicular compartments (Juengel et al., Reference Juengel, Reader, Bibby, Lun, Ross, Haydon and McNatty2006).
In vitro studies demonstrated that BMP-7 promotes growth and activation of primordial follicles, and stimulates the expression of receptors for FSH in murine ovaries (Lee et al., Reference Lee, Yoon, Yoon, Cha, Lee, Shimasaki, Lee and Lee2004). BMP-7 also contributed to maintain the survival and ultrastructure of primordial follicles after caprine ovarian tissue culture (Araújo et al., Reference Araújo, Silva, Magalhães, Silva, Báo, Silva, Figueiredo and Rodrigues2010a). After injection of BMP-7 in the rat ovarian bursa increased the number of primary, secondary and antral follicles, indicating that, in murine, the BMP-7 is able to promote the growth and activation of primordial follicles (Lee et al., Reference Lee, Otsuka, Moore and Shimasaki2001). Additionally, BMP-7 alone or together with FSH significantly increased growth and antrum formation in cultured caprine follicles (Frota et al., Reference Frota, Leitão, Costa, van den Hurk, Brito, Saraiva, Figueiredo and Silva2011).
Human granulosa cells from antral follicles cultured in presence of BMP-6 or -7 showed a significant increase in expression of GDF-3 mRNA (Shi et al., Reference Shi, Yoshino, Osuga, Akiyama, Harada, Koga, Fujimoto, Yano and Taketani2012). The authors furthermore found that growth of cultured human granulosa cells was linked to the presence of GDF-3, BMP-6 or BMP-7. In a previous study with in vitro human granulosa cells, Shi et al. (Reference Shi, Yoshino, Osuga, Nishii, Yano and Taketani2010) reported a BMP-7 induced reduction in LH receptor gene expression. In addition, cultured human granulosa cells show a reduction in the order of 50–60% in the expression of PCSK6, when cultured in the presence of BMP-2, -6, -7 or -15 (Akiyama et al., Reference Akiyama, Yoshino, Osuga, Shi, Hirota, Hirata, Harada, Koga, Fujimoto, Yano and Taketani2012). PCSK is a protease that cleaves NODAL (protein belonging to the TGF-β superfamily; see Table 1) into an active form (Constam & Robertson, Reference Constam and Robertson2000). In addition, BMP-7 increases the expression of cytochrome P450 enzyme aromatase favoring the production of estradiol in vivo (Lee et al., Reference Lee, Otsuka, Moore and Shimasaki2001) and in vitro (Miyoshi et al., Reference Miyoshi, Otsuka, Inagaki, Otani, Takeda, Suzuki, Goto, Ogura and Makino2007). Granulosa cells showed a reduction in the level of carbamoyl-phosphate synthetase 2, aspartate transcarbamylase, and dihydroorotase (CAD) protein after their culture in medium supplemented with BMP-7. BMP-7 significantly increased mRNA expression of X-linked inhibitor of apoptosis protein (XIAP), and BMP-7 furthermore significantly decreased the expression of caspase-3 and caspase-9 (Kayamori et al., Reference Kayamori, Kosaka, Miyamoto and Shimizu2009). Because progesterone is important for the process of ovulation in mammals (Yoshimura & Wallach, Reference Yoshimura and Wallach1987), inhibition of progesterone production by BMP-7 can be related to the mechanisms of ovulation inhibition (Lee et al., Reference Lee, Otsuka, Moore and Shimasaki2001).
Expression of BMP-8 in the ovary was still not reported, but its effect on the formation of PGCs was demonstrated by Ying et al., (Reference Ying, Liu, Marble, Lawson and Zhao2000). These authors showed that BMP-8 is expressed in the extra-embryonic ectoderm of mouse embryos, and that a mutation in the gene which produces this protein results in a decrease in the population of PGCs. Such a decrease may lead to a reduction in the follicle population in adult animals. Table 6 shows the effects of BMP-5, -6, -7 and -8 on oogenesis and folliculogenesis in the different mammalian species.
Table 6 Effects of BMP-5, -6, -7 and -8 on oogenesis and folliculogenesis in the different mammalian species
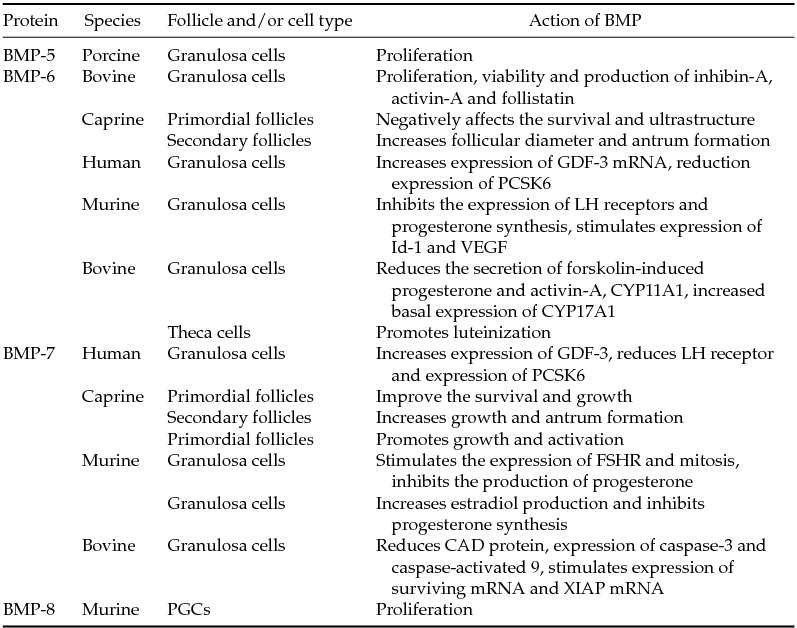
Murine (Shimasaki et al., Reference Shimasaki, Zachow, Li, Kim, Iemura, Ueno, Sampath, Chang and Erickson1999; Ying et al., Reference Ying, Liu, Marble, Lawson and Zhao2000; Lee et al., Reference Lee, Otsuka, Moore and Shimasaki2001; Otsuka et al., Reference Otsuka, Moore and Shimasaki2001; Lee et al., Reference Lee, Yoon, Yoon, Cha, Lee, Shimasaki, Lee and Lee2004; Park et al., Reference Park, Park, Joo, Joo, Son and Lee2012); bovine (Fatehi et al., Reference Fatehi, van den Hurk, Colenbrander, Daemen, van Tol, Monteiro, Roelen and Bevers2005; Kayani et al., Reference Kayani, Glister and Knight2009; Kayamori et al., Reference Kayamori, Kosaka, Miyamoto and Shimizu2009); caprine (Araújo et al., Reference Araújo, Silva, Magalhães, Silva, Báo, Silva, Figueiredo and Rodrigues2010a,Reference Araújo, Lima-Verde, Name, Bao, Campello, Silva, Rodrigues and Figueiredob; Frota et al., Reference Frota, Leitão, Costa, van den Hurk, Brito, Saraiva, Figueiredo and Silva2011, Reference Frota, Leitão, Costa, van den Hurk, Saraiva, Figueiredo and Silva2013); human (Seidah & Chretien, Reference Seidah and Chretien1999; Shi et al., Reference Shi, Yoshino, Osuga, Nishii, Yano and Taketani2010, Reference Shi, Yoshino, Osuga, Akiyama, Harada, Koga, Fujimoto, Yano and Taketani2012); porcine (Shimizu et al., Reference Shimizu, Yokoo, Miyake, Sasada and Sato2004). FSHR, follicle stimulating hormone receptor.
BMP-15
Several studies have shown that BMP-15 is involved in the normal fertility in mammals, playing a role in the regulation of follicular (Kedem et al., Reference Kedem, Fisch, Garor, Ben-Zaken, Gizunterman, Felz, Ben-Haroush, Kravarusic and Abir2011; Passos et al., Reference Passos, Vasconcelos, Silva, Brito, Saraiva, Magalhães, Costa, Donato, Ribeiro, Cunha, Peixoto, Campello, Figueiredo, van den Hurk and Silva2013) and cumulus cell functions (Erickson & Shimasaki, Reference Erickson and Shimasaki2001; Teixeira Filho et al., Reference Teixeira Filho, Baracat, Lee, Suh, Matsui, Chang, Shimasaki and Erickson2002; Gilchrist et al., Reference Gilchrist, Lane and Thompson2008; Chen et al., Reference Chen, Zhao, Qiao, Liu, Lian and Zheng2009). The expression of mRNAs for BMP-15 was detected in primordial, primary and secondary follicles, as well as in oocytes and granulosa cells from goat antral follicles (Silva et al., Reference Silva, van den Hurk, van Tol, Roelen and Figueiredo2005). BMP-15 protein was detected in oocytes of all types of follicles and in granulosa cells from primary follicles onward (Silva et al., Reference Silva, van den Hurk, van Tol, Roelen and Figueiredo2006). BMP-15 was shown to be expressed in primary oocytes from follicles of rodents, ovine and humans (Juengel & McNatty, Reference Juengel and McNatty2005). BMP-15 was also detected in follicular fluid of human follicles (Wu et al., Reference Wu, Tang, Cai, Lu, Xu, Zhu, Luo and Huang2007). In addition, data from real-time PCR showed a significant reduction in the levels of BMP-15 transcripts in cultured follicles, with a decrease of two times in its levels during follicular development (Sánchez et al., Reference Sánchez, Adriaenssens, Romero and Smitz2009).
Mutations in the BMP-15 are related with the occurrence of diseases directly related to reproductive activity, such as polycystic ovary syndrome and premature ovarian failure (Takebayashi et al., Reference Takebayashi, Takakura, Wang, Kimura, Kasahara and Noda2000). Immunization studies showed that BMP-15 proteins are involved in the normal ovine follicular development, including both the early and later stages of growth (Juengel et al., Reference Juengel, Hudson, Heath, Smith, Reader, Lawrence, O’Connell, Laitinen, Cranfield, Groome, Ritvos and McNatty2002; Wu et al., Reference Wu, Tang, Cai, Lu, Xu, Zhu, Luo and Huang2007). During transition from primordial to primary follicles, BMP-15 is involved in the ovine granulosa cell proliferation or for the prevention of their differentiation (Galloway et al., Reference Galloway, McNatty, Cambridge, Laitinen, Juengel, Jokiranta, McLaren, Luiro, Dodds, Montgomery, Beattie, Davis and Ritvos2000). Lima et al. (Reference Lima, Brito, Rossetto, Duarte, Rodrigues, Saraiva, Costa, Donato, Peixoto, Silva, Figueiredo and Rodrigues2012) showed that BMP-15 is effective in promoting antral cavity formation and maintenance of follicular growth during culture of goat pre-antral follicles. A recent study demonstrated that BMP15 down-regulates connexin 43 (Cx43) in human granulosa cells. In this study, the reduction of Cx43 contributes to a decrease in the activity of intercellular communication, which indicates that the Smad1/5/8 signalling pathway is required for the BMP15-induced down-regulation of Cx43 (Chang et al., Reference Chang, Cheng, Taylor and Leung2014). However, Sugimura et al. (Reference Sugimura, Ritter, Sutton-McDowall, Mottershead, Thompson and Gilchrist2014) showed that BMP15 was equally effective in maintaining gap-junctional communication (GJC) even in the presence of amphiregulin. They also reported that amphiregulin stimulation of COC glycolysis and BMP15 preservation of GJC may facilitate efficient transfer of metabolites from cumulus cells to the oocyte thereby enhancing oocyte developmental competence. In bovine species, BMP15 stimulates expansion of in vitro-matured bovine COCs by driving glucose metabolism toward hyaluronic acid production and controlling the expression of genes in the ovulatory cascade (Caixeta et al., Reference Caixeta, Sutton McDowall, Gilchrist, Thompson, Machado, Lima and Buratini2013).
BMP-15 is capable of maintaining a low incidence of apoptosis in cumulus cells (Wu et al., Reference Wu, Tang, Cai, Lu, Xu, Zhu, Luo and Huang2007). Studies on rat granulosa cells demonstrated that recombinant BMP-15 stimulates the proliferation of these cells independent of FSH, but decreased the effects of FSH on progesterone production without affecting that of estradiol (Otsuka et al., Reference Otsuka, Yao, Lee, Yamamoto, Erickson and Shimasaki2000). BMP-15 furthermore stimulates expression of kit ligand (KL) in rat granulosa cells (Otsuka & Shimasaki, Reference Otsuka and Shimasaki2002), and it also stimulates the expression of epidermal growth factor (EGF) in cumulus cells of mice (Yoshino et al., Reference Yoshino, McMahon, Sharma and Shimasaki2006).
Oocytes recovered from follicles with high levels of BMP-15 in the follicular fluid had a higher rate of fertilization, cleavage and better quality in the developing embryo. BMP-15 showed a positive correlation with the levels of estradiol and a negative correlation with those of FSH in the follicular fluid. In addition, BMP-15 can serve as an indicator of mature oocyte cytoplasm. BMP-15 can inhibit the action of stimulating FSH production of pregnancy-associated plasma protein-A (PAPP-A) in a dose-dependent manner. In addition, by controlling the expression of PAPP-A in granulosa cells, BMP-15 and FSH play a role in the selection of the dominant follicle and oocyte maturation (Wu et al., Reference Wu, Wang, Chen, Zhu, Dong, Sheng, Xu and Huang2012). Table 7 summarizes the effects of BMP-15 on oogenesis and folliculogenesis in the different mammalian species.
Table 7 Effects of BMP-15 on oogenesis and folliculogenesis in the different mammalian species

Murine (Otsuka et al., Reference Otsuka, Yao, Lee, Yamamoto, Erickson and Shimasaki2000, Reference Otsuka and Shimasaki2002; Yoshino et al., Reference Yoshino, McMahon, Sharma and Shimasaki2006; McIntosh et al., Reference McIntosh, Lawrence, Smith, Juengel and McNatty2012; Peng et al., Reference Peng, Li, Wigglesworth, Rangarajan, Kattamuri, Peterson, Eppig, Thompson and Matzuk2013); bovine (Passos et al., Reference Passos, Vasconcelos, Silva, Brito, Saraiva, Magalhães, Costa, Donato, Ribeiro, Cunha, Peixoto, Campello, Figueiredo, van den Hurk and Silva2013); caprine (Celestino et al., Reference Celestino, Lima-Verde, Bruno, Matos, Chaves, Saraiva, Silva, Faustino, Rossetto, Lopes, Donato, Peixoto, Campello, Silva and Figueiredo2011; Lima et al., Reference Lima, Brito, Rossetto, Duarte, Rodrigues, Saraiva, Costa, Donato, Peixoto, Silva, Figueiredo and Rodrigues2012); human (Teixeira Filho et al., Reference Teixeira Filho, Baracat, Lee, Suh, Matsui, Chang, Shimasaki and Erickson2002; Gilchrist et al., Reference Gilchrist, Lane and Thompson2008; Chen et al., Reference Chen, Zhao, Qiao, Liu, Lian and Zheng2009; Qiao & Feng, Reference Qiao and Feng2011; Kedem et al., Reference Kedem, Fisch, Garor, Ben-Zaken, Gizunterman, Felz, Ben-Haroush, Kravarusic and Abir2011); ovine (Galloway et al., Reference Galloway, McNatty, Cambridge, Laitinen, Juengel, Jokiranta, McLaren, Luiro, Dodds, Montgomery, Beattie, Davis and Ritvos2000; Juengel et al., Reference Juengel, Hudson, Heath, Smith, Reader, Lawrence, O’Connell, Laitinen, Cranfield, Groome, Ritvos and McNatty2002).
Recent studies have demonstrated in different animals (i.e. mouse, rat, pig, ovine, cow and red deer) that the expression of BMP-15 and GDF-9 is tightly co-regulated (Crawford & Mcnatty, Reference Crawford and Mcnatty2012). In fact, BMP-15 and GDF-9 play roles in regulating cumulus cell functions through the processes of mitosis, proliferation, apoptosis, luteinization, metabolism and expansion through mitogenic signalling transduction mechanisms (Qiao & Feng, Reference Qiao and Feng2011). After immunizing mice against selected proregion peptide sequences of BMP-15 and GDF-9 or a recombinant full-length mouse proregion GDF-9 protein, McIntosh et al. (Reference McIntosh, Lawrence, Smith, Juengel and McNatty2012) showed that both factors have physiologically important roles in regulating ovulation rate and litter size in mice. Peng et al. (Reference Peng, Li, Wigglesworth, Rangarajan, Kattamuri, Peterson, Eppig, Thompson and Matzuk2013) demonstrated that GDF-9:BMP-15 heterodimers are the most bioactive ligands in the regulation of cumulus expansion genes in mice and human. GDF-9 and BMP-15 play crucial roles in follicular development, ovulation, oocyte maturation and embryo development (Juengel & McNatty, Reference Juengel and McNatty2005; Knight & Glister, Reference Knight and Glister2006; Hutt & Albertini, Reference Hutt and Albertini2007; Gilchrist et al., Reference Gilchrist, Lane and Thompson2008). Under in vitro conditions, the positive role of BMP-15 and GDF-9 in the oocyte maturation and rate of blastocyst production was observed in a co-incubation of cumulus–oocyte complexes in the presence of these factors that promotes oocyte maturation and enhances blastocyst production (Hussein et al., Reference Hussein, Thompson and Gilchrist2006). Interestingly, both GDF-9 and BMP-15 are involved in the folliculogenesis in humans and their abnormal expression may be related to female infertility (Gilchrist et al., Reference Gilchrist, Lane and Thompson2008). Results suggested that BMP-15 may be a good indicator of oocyte maturity and fertilization ability (Wu et al., Reference Wu, Tang, Cai, Lu, Xu, Zhu, Luo and Huang2007). As GDF-9 and BMP-15 are both present in follicles throughout most stages of follicular growth, it is important to consider whether these growth factors are synergistic or redundant in processes where they have similar activity alone (McNatty et al., Reference McNatty, Smith, Moore, Reader, Lun, Hanrahan, Groome, Laitinen, Ritvos and Juengel2005). There are clinical implications for BMP-15 and GDF-9, specifically as a potential tool to help overcome female infertility. Figure 2 summarizes the stages of follicular development in which each BMP acts.

Figure 2 Action of BMPs in the different stages of follicle development in mammalian species. The BMP system acts to promote follicular development and cellular growth and regulates the functions of different components of the ovarian follicles. Legend: GCC, germ cell cohorts; PF, primordial follicle; PrF, primary follicle; SF, secondary follicle.
Conclusions and final considerations
In summary, this review shows the importance of BMPs for the control of oogenesis and folliculogenesis in mammalian species. BMP-2 and -4 modulate hormone production of granulosa and theca cells contributing to follicular growth. BMP-5, -6, -7 and -8 act in follicular development and BMP-15 contributes to the oocyte and follicular maturation. These data show that a complex combination of BMPs is involved in the control of primordial germ cell formation, oocyte growth and maturation, as well as on follicle development and ovulation.
Acknowledgements
J.R.V. Silva is an investigator of the National Council for Scientific and Technological Development (CNPq, Brazil).