Introduction
Weeds are one of the major biotic stress factors that significantly affect agricultural production and threaten food security around the world (Costa et al. Reference Costa, Cechin, Rizzardi, Martin, Sauder, Adegas and Grando2021), with an estimated cost of over US$30 billion yr−1 (Gould et al. Reference Gould, Brown and Kuzma2018). Favorable characteristics such as high stress tolerance and genetic variability enable weeds to adapt to various environments and survive in different cropping systems (Costa et al. Reference Costa, Cechin, Rizzardi, Martin, Sauder, Adegas and Grando2021). Ludwigia prostrata is an annual dicotyledonous weed of the Onagraceae family, widely distributed in rice fields in the south of China. In recent years, the distribution of weed species in paddy fields has changed in the lower Yangtze River delta with the development of rice cultivation systems and herbicide use, and L. prostrata has gradually become a dominant weed species in some regions (Tian et al. Reference Tian, Yuan and Shen2020). Control of L. prostrata mainly relies on the use of herbicides, especially the acetolactate synthase (ALS)-inhibiting herbicides.
Bensulfuron-methyl, which belongs to the sulfonylurea group of ALS-inhibiting herbicides, can be applied both POST and PRE, and it has been used for decades to control broadleaf weeds in rice fields (Zhang et al. Reference Zhang, Liu, Cai, Zhou, Wang, Lu, Zhou, Liu, Liang, Wang and Zhu2020). ALS-inhibiting herbicides are mainly absorbed by the leaves and roots and are transported to the growth points, resulting in the inhibition of biosynthesis of isoleucine, valine, and leucine (Duggleby and Pang 2000). ALS-inhibiting herbicides have been applied widely over the past 30 yr due to their efficacy, broad-spectrum weed control, relatively low cost and toxicity, and favorable crop safety (Yu and Powles Reference Yu and Powles2014). The strong selection pressure and single target of action led to the widespread development of resistance to ALS-inhibiting herbicides in at least 165 species of weeds, increasing both the cost and complexity of weed control (Heap Reference Heap2021; Yu and Powles Reference Yu and Powles2014).
Resistance to ALS-inhibiting herbicides has been reported to be endowed by target-site and non–target site alterations (Délye et al. Reference Délye, Jasieniuk and Le Corre2013; Powles and Yu Reference Powles and Yu2010; Yu and Powles Reference Yu and Powles2014). Target-site resistance (TSR) usually involves ALS gene mutations, which impact ALS enzyme structural changes at various binding sites, and/or the overexpression or the ALS gene. To date, 29 TSR mutations have been established in eight resistance-related sites of ALS, including Ala-122-Asn/Val/Ser/Thr/Tyr, Pro-197-Leu/Ser/His/Ala/Thr/Arg/Tyr/Glu/Gln/Asn/Ile, Ala-205-Phe/Val, Asp-376-Glu, Arg-377-His, Trp-574-Gly/Arg/Leu/Met, Ser-653-Ile/Asn/Thr, and Gly-654-Asp/Glu (Tranel et al. Reference Tranel, Wright and Heap2021). The ALS mutations often endow high-level resistance but may also enable variable reductions in plant sensitivity and the spectrum of cross-resistance, depending on each mutation and species. For example, the Trp-574 resistance mutation usually resulted in a higher level of resistance and provided a wider range of cross-resistance than the Pro-197 resistance mutation (Yu and Powles Reference Yu and Powles2014). ALS gene overexpression occurs less frequently than target-gene mutations in weeds resistant to ALS-inhibiting herbicides. Yu et al. (Reference Yu, McCullough, McElroy, Jespersen and Shilling2020) and Sen et al. (Reference Sen, Hamouzová, Mikulka, Bharati, Košnarová, Hamouz, Royc and Soukupa2021) have discovered that target-gene overexpression is associated with resistance to ALS-inhibiting herbicides in annual sedge (Cyperus compressus L.) and barren brome (Bromus sterilis L.), respectively. non–target site based resistance (NTSR) mechanisms include reduction of herbicide translocation or uptake, increased sequestration, and detoxification (Délye Reference Délye2013; Yuan et al. Reference Yuan, Tranel and Stewart2007). In most cases of NTSR, enhanced metabolism is the primary cause of resistance and has been identified in many weed species resistant to ALS-inhibiting herbicides, including flixweed (Descurainia sophia L.) (Yang et al. Reference Yang, Deng, Li, Yu, Bai and Zheng2016, Reference Yang, Li, Shen, Xu, Liu, Deng, Li and Zheng2018), corn poppy (Papaver rhoeas L.) (Rey-Caballero et al. Reference Rey-Caballero, Menéndez, Osuna, Salas and Torra2017), large crabgrass (Digitaria sanguinalis L.) (Mei et al. Reference Mei, Si, Liu, Qiu and Zheng2017), arrowhead (Sagittaria trifolia L.) (Zhao et al. Reference Zhao, Fu, Yu, Huang, Yan, Li, Shafi, Zhu, Wei and Ji2017), American sloughgrass (Beckmannia syzigachne (Steud.) Fernald) (Wang et al. Reference Wang, Li, Li, Han, Li, Yu and Cui2018), and water chickweed (Myosoton aquaticum L.) (Liu et al. Reference Liu, Bai, Zhao, Jia, Li, Zhang and Wang2018).
Recently, farmers have found that bensulfuron-methyl at the recommended dose cannot effectively control L. prostrata in a rice field near Yangzhou City in Jiangsu Province, which seriously compromised rice production. Therefore, the seeds of this L. prostrata biotype with suspected resistance to bensulfuron-methyl were harvested. The aims of this study were: (1) to determine the resistance level to bensulfuron-methyl in the L. prostrata biotype; (2) to characterize the potential ALS resistance mutations causing resistance to bensulfuron-methyl; and (3) to identify the cross-resistance pattern of this resistant population to other ALS-inhibiting herbicides.
Materials and Methods
Plant Material
In October 2019, the putative bensulfuron-methyl–resistant L. prostrata biotype (JS-R) was harvested from a paddy field in Guangling District, Yangzhou City, Jiangsu Province (32.30°N, 119.55°E), where bensulfuron-methyl had been used for over 10 yr and failed to control the occurrence of L. prostrata. The susceptible L. prostrata biotype (JS-S) was collected at the edge of a paddy field at Yangzhou University with no history of ALS-inhibiting herbicide applications (32.40°N, 119.43°E). Mature seeds were harvested randomly from 15 to 20 plants in the same field for each population. Seeds were then dried naturally and put into envelope bags for storage at 4 C.
Plant Cultivation and Dose Response
Seeds of the JS-S and JS-R L. prostrata biotypes were sown into the plastic pots (7 by 7 cm), filled with moist loam soil. A total of 16 seeds were planted in each pot with a small brush, and these pots were moved into an illumination incubator (Changzhou Haibo Instrument Equipment, Changzhou, China) with 14-h /30 C diurnal and 10-h /25 C nocturnal conditions and watered regularly. Seedlings of L. prostrata were thinned to 9 to 12 plants of similar size per pot before herbicide treatment. Approximately 3 wk later, when the seedlings had grown to the 4- to 5-leaf stage, plants were treated with bensulfuron-methyl at rates of 0, 0.18, 0.70, 2.81, 11.25, 45, and 180 g ai ha−1 for the JS-S and JS-R biotypes. The labeled field use rate of bensulfuron-methyl is 45 g ai ha−1. No additional adjuvants were included, because the formulations utilized did not require additional adjuvant. The spray volume was maintained at 400 L ha−1 with a pressure of 0.20 MPa. The fresh weight of aboveground plants was sampled and weighed at 21 d after application. Three replicate pots were made for each herbicide treatment, and the dose–response assay was performed twice independently.
ALS Gene Amplification and Sequencing
Total RNA was extracted from leaf tissues of 10 JS-S and 10 JS-R L. prostrata plants using the MiniBEST Plant RNA extraction Kit (Takara, Beijing, China), and cDNAs were synthesized with the FastQuant RT Kit (Tiangen, Beijing, China). Based on the ALS gene sequences of D. sophia (GenBank accession FJ715633.1), cleavers (Galium aparine L.) (GenBank accession GU377313.1), horseweed (Conyza canadensis (L.) Cronq.) (GenBank accession HM067014.1), shepherd’s purse (Capsella bursa-pastoris (L.) Medik.) (GenBank accession HQ880660.1), and P. rhoeas (GenBank accession AJ577316.1), two pairs of primers (ALS-F1/ALS-R1 and ALS-F2/ALS-R2) (Table 1) were designed to amplify the partial L. prostrata ALS gene.
Table 1. Primer sets designed for the amplification of Ludwigia prostrata partial ALS gene.

The PCR amplification reaction was carried out in a 40-μl volume, consisting of 2 μl of cDNA, 20 μl of 2×Taq PCR Mix II (Tiangen), 1 μl (10 μM) of forward (ALS-F1 or ALS-F2) primer, 1 μl (10 μM) of reverse (ALS-R1 or ALS-R2) primer, and 16 μl ddH2O. The amplification protocol was run in a thermal cycler as follows: 10 min at 94 C for denaturation; 34 cycles of denaturation at 94 C for 20 s, 50 C for 30 s, and 72 C for 60 s; and elongation at 72 C for 5 min. The identified PCR products were purified using the TIANgel Purification Kit (Tiangen) and then sequenced from both ends by Sangon Biotech Corporation (Shanghai, China); DNAMAN v. 5.22 software (Lynnon Biosoft, QC, Canada) was then used to analyze and align the sequence data.
Cross-Resistance to Other ALS-inhibiting Herbicides
Pyrazosulfuron-ethyl (sulfonylurea [SU] herbicide), imazethapyr (imidazolinone [IMI] herbicide), bispyribac-sodium (pyrimidinyl thiobenzoate [PTB] herbicide), and penoxsulam (triazolopyrimidine [TP] herbicide), representing four distinct chemical families of ALS-inhibiting herbicides, were chosen for the cross-resistance assessment. The cross-resistance levels to ALS-inhibiting herbicides were determined by dose–response assays as described earlier. At the 4- to 5-leaf stage of plants, the herbicides were sprayed at a series of rates (Table 2).
Table 2. ALS-inhibiting herbicides used in this study for the cross-resistance assessment.

a IMI, imidazolinone herbicide; PTB, pyrimidinyl thiobenzoate herbicide; SU, sulfonylurea herbicide; TP, triazolopyrimidine herbicide.
b AS, aqueous solution; OD, oil dispersion; SC, suspension concentrate; WP, wettable powder.
Statistical Analysis
The average fresh weights per treatment from the dose–response assays were converted to the percentage value of the control data, and data from each treatment were analyzed with one-way ANOVA (SPSS Statistics v. 20.0, IBM, Armonk, NY) to test for significant difference. No significant difference between two separate experimental runs was observed, and the data were pooled for analysis. The herbicide rate that resulted in 50% inhibition of plant growth (GR50) was calculated by the three-parameter log-logistic equation with SigmaPlot v. 12.0 (Systat Software, Inc., San Jose, CA) (Seefeldt et al. Reference Seefeldt, Jensen and Fuerst1995). The resistance index (RI) was calculated by dividing the GR50 value of the resistant population by that of the susceptible population.

Results and Discussion
Dose–Response Curve to Bensulfuron-Methyl
Dose–response assays indicated the JS-R biotype displayed a 21.2-fold resistance to bensulfuron-methyl in comparison with the JS-S biotype (Figure 1). The GR50 value was 0.55 g ai ha−1 for the JS-S biotype and 11.65 g ai ha−1 for the JS-R biotype. As shown in Figure 1A, the susceptible L. prostrata plants at the 4- to 5-leaf stage could be controlled at 1/16× the recommended field dose of bensulfuron-methyl. However, the resistant plants had new tillers and could continue to grow at the recommended field dose of 45 g ai ha−1.
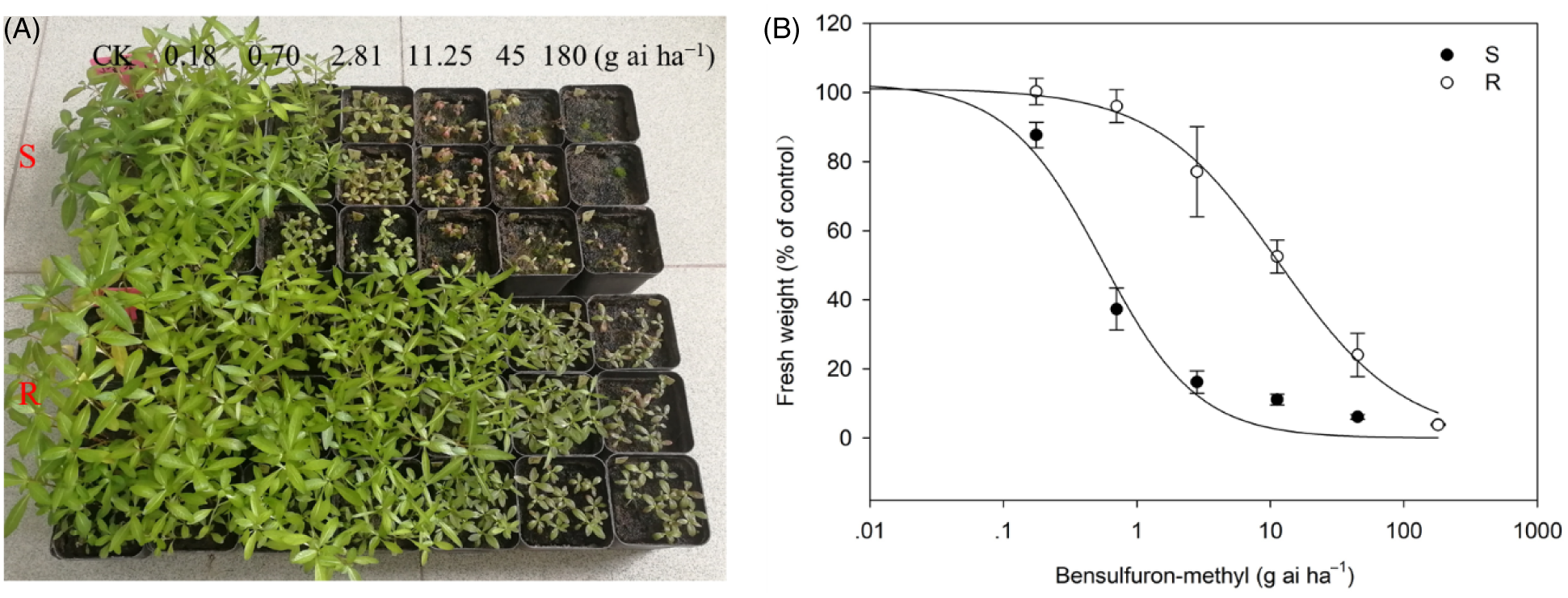
Figure 1. The symptoms of the susceptible (S) and resistant (R) Ludwigia prostrata plants at 21 d after treatment with different doses of bensulfuron-methyl (A). The dose–response curves of JS-S and JS-R L. prostrata biotypes for bensulfuron-methyl (B).
Herbicide resistance has become a global concern with the rapid development of resistant weeds, and the number of ALS inhibitor–resistant weeds is the largest, accounting for 32.1% of the total number of resistant weeds (Heap Reference Heap2021). ALS-inhibiting herbicides are prone to evolution of herbicide resistance, and weed resistance can develop after fewer than 10 applications (Beckie Reference Beckie2006). Bensulfuron-methyl is one of the main herbicides that have been used for nearly 30 yr in rice fields in China; and several weed species, including Monochoria korsakowii (Lu et al. Reference Lu, Zhang, Fu, Li and Li2009), S. trifolia (Wei et al. Reference Wei, Li, Ji, Dong and Wang2015), eclipta (Eclipta prostrata) (Li et al. Reference Li, Li, Yu, Wang and Cui2017), and Ammannia arenaria H. B. K. (Zhang et al. Reference Zhang, Liu, Cai, Zhou, Wang, Lu, Zhou, Liu, Liang, Wang and Zhu2020), have evolved resistance to this herbicide. The widespread occurrence of bensulfuron-methyl–resistant weeds has led to the low control effect of the herbicide in rice fields, which greatly reduces the use life of the herbicide and poses a threat to the yield and quality of rice. Thus, the potential resistance mechanism and cross-resistance pattern in L. prostrata were investigated further.
ALS Gene Amplification and Sequencing
In this study, 10 JS-S and 10 JS-R plants were randomly selected for RNA extraction and ALS sequencing. The amplified ALS gene fragment, with a length of 1,533 bp, showed high homology with the documented ALS gene from D. sophia (73.0%), C. canadensis (75.5%), C. bursa-pastoris (73.5%), G. aparine (72.8%), and P. rhoeas (71.6%), indicating that the ALS gene from L. prostrata was successfully amplified. In addition, the ALS gene obtained from JS-S and JS-R L. prostrata biotypes showed 98.9% similarity, and a single nucleotide mutation (C/T) CA resulting in a proline (CCA) to serine (TCA) substitution at site 197 was detected in all the JS-R plants but not in JS-S plants (Figure 2).

Figure 2. Domain A of the ALS gene in the JS-S and JS-R Ludwigia prostrata biotypes. The boxed codon indicates a Pro-197-Ser mutation resulting from a CCA to TCA nucleotide change in the JS-R biotype.
The sequence analysis results indicated that the target-site ALS gene Pro-197-Ser mutation was mainly responsible for bensulfuron-methyl resistance in the JS-R L. prostrata biotype. High-level ALS-inhibiting herbicide resistance conferred by the same Pro-197-Ser substitution has been reported in some resistant weed species, including D. sophia (Cui et al. Reference Cui, Zhang, Wei, Zhang, Li, Zhang and Wang2011; Deng et al. Reference Deng, Liu, Yang, Mei, Li and Zheng2015), rigid ryegrass (Lolium rigidum Gaudin) (Yu et al. Reference Yu, Han, Vila-Aiub and Powles2010), C. bursa-pastoris (Cui et al. Reference Cui, Li, Wang, Wang, Wei and Cao2012; Zhang et al. Reference Zhang, Liu, Cai, Zhou, Wang, Lu, Zhou, Liu, Liang, Wang and Zhu2020), M. aquaticum (Liu et al. Reference Liu, Wu, Guo, Du, Yuan and Wang2015), wild radish (Raphanus raphanistrum L.) (Yu et al. Reference Yu, Zhang, Hashem, Walsh Michael and Powles2003), wild buckwheat (Polygonum convolvulus L.) (Pandian et al. Reference Pandian, Friesen, Laforest, Peterson, Prasad and Jugulam2020), and B. syzigachne (Wang et al. Reference Wang, Chen, Li, Li, Li and Cui2020). However, to our knowledge, this is the first report of the Pro-197-Ser resistance mutation identified in L. prostrata. Target-site mutations are the most commonly reported resistance mechanism for ALS inhibitor–resistant weed species (Powles and Yu Reference Powles and Yu2010). Among the 29 resistance-endowing mutations in weeds identified so far, the frequencies of Pro-197-Ser and Trp-574-Leu mutations were much higher than the frequency of the other mutations, and different ALS gene mutations may be associated with herbicide use patterns, selection pressure, and the lack of a fitness cost (Yu and Powles Reference Yu and Powles2014). In our work, resistance to ALS-inhibiting herbicides was identified in L. prostrata. However, with the increase of selection pressure posed by different ALS-inhibiting herbicides, the development of resistance in L. prostrata may accelerate, and the mutation types and resistance mechanisms may also become diversified.
Cross-Resistance to Other ALS-inhibiting Herbicides
The dose–response assays were performed to assess the cross-resistance pattern to ALS-inhibiting herbicides in the JS-R L. prostrata biotype carrying the Pro-197-Ser resistance mutation. The JS-R biotype displayed high resistance to pyrazosulfuron-ethyl, but was sensitive to penoxsulam, bispyribac-sodium, and imazethapyr. The JS-R biotype RI values for pyrazosulfuron-ethyl, penoxsulam, bispyribac-sodium and imazethapyr were 12.0, 1.3, 0.7, and 0.8, respectively (Table 3; Figure 3).
Table 3. Herbicide dose that caused 50% reduction in fresh weight (GR50) and resistance index (RI) values for five different ALS-inhibting herbicides for the JS-S and JS-R Ludwigia prostrata biotypes.


Figure 3. Dose–response curves of the JS-S and JS-R Ludwigia prostrata biotypes for different ALS inhibitors: (A) pyrazosulfuron-ethyl; (B) penoxsulam; (C) bispyribac-sodium; (D) imazethapyr).
ALS resistance mutations at different positions of the ALS gene usually endow different spectra of cross-resistance to ALS-inhibiting herbicides. Classically, Pro-197 mutations result in resistance to SU and TP herbicides; Ala-122, Ser-653, and Gly-654 mutations provide resistance to IMI herbicides; and Asp-376 and Trp-574 substitutions tend to endow resistance to all five chemical families of ALS-inhibiting herbicides (Deng et al. Reference Deng, Yang, Jiao, Zhang, Li and Zheng2016; Wang et al. Reference Wang, Ge, Zhao, Zhang, You, Wang, Liu and Wang2019; Yu and Powles Reference Yu and Powles2014). However, for the same amino acid substitution at the 197 amino acid position, the cross-resistance pattern is variable and weed-species dependent. For example, the Pro-197-Ser mutation in D. sophia exhibited cross-resistance to SU and TP herbicides and showed no cross-resistance to PTB and IMI herbicides (Deng et al. Reference Deng, Cao, Yang, Liu, Mei and Zheng2014). The same mutation displayed resistance to SU, TP, and PTB herbicides in M. aquaticum (Liu et al. Reference Liu, Bi, Li, Yuan, Du and Wang2013) and broad-spectrum resistance to SU, TP, PTB, and IMI herbicides in E. prostrata (Li et al. Reference Li, Li, Yu, Wang and Cui2017) and G. aparine (Deng et al. Reference Deng, Di, Cai, Chen and Yuan2019). Therefore, it is necessary to determine the specific cross-resistance patterns for the newly identified herbicide-resistant biotype. Fortunately, in this study, the JS-R L. prostrata biotype with the Pro-197-Ser mutation only conferred resistance to SU herbicides; the biotype was still sensitive to TP, PTB, and IMI herbicides. Penoxsulam and bispyribac-sodium, two commonly used ALS-inhibiting herbicides in rice fields, could effectively control the occurrence of L. prostrata. Furthermore, other integrated weed management practices, the use of herbicide rotations and mixtures, and PRE herbicide use should be encouraged to reduce the risk of development of resistance in L. prostrata.
In conclusion, we have identified the first case of bensulfuron-methyl resistance in an L. prostrata biotype from a rice field in China. Bensulfuron-methyl resistance is due to a proline-to-serine substitution at position 197 of the ALS gene in the JS-R L. prostrata biotype. In addition, this resistant biotype showed cross-resistance to pyrazosulfuron-ethyl, but was still sensitive to penoxsulam and bispyribac-sodium, which will provide alternative herbicides for the control of resistant L. prostrata biotypes.
Acknowledgments
This work was funded by the National Natural Science Foundation of China (no. 32001925) and the Agricultural Science and Technology Innovation Fund (CX (20)3131). No conflicts of interest have been declared.