Introduction
Cover crop hectares in the United States almost doubled from 2012 to 2017 (CTIC 2017), with most cover crop users residing in the mid-Atlantic and southeastern areas (USDA ERS 2012). Cover crops have many benefits, including nitrogen fixation, erosion mitigation, protection of water quality, increasing soil health, and weed suppression (Hayden et al. Reference Hayden, Brainard, Henshaw and Nqouajio2012; Krutz et al. Reference Krutz, Locke and Steinriede2009; Pittman et al. Reference Pittman, Barney and Flessner2019; Snapp et al. Reference Snapp, Swinton, Labarta, Mutch, Black, Leep, Nyiraneza and O’Neil2005). In a survey of farmers who use cover crops, 69% said they sometimes or always see increased control of herbicide-resistant weeds after a cereal rye cover crop (CTIC 2017).
Incorporating cover crops into an integrated weed management (IWM) plan reduces herbicide inputs and selection for herbicide-resistance development (Price et al Reference Price, Balkcom, Duzy and Kelton2012; Snyder et al. Reference Snyder, Curran, Karsten, Malcolm, Duiker and Hyde2016). Combining a cereal rye cover crop with deep tillage reduced Palmer amaranth [Amaranthus palmeri (S.) Watson] germination by as much as 98% in soybeans (DeVore et al. Reference DeVore, Norsworthy and Brye2013). Wiggins et al. (Reference Wiggins, McClure, Hayes and Steckel2015) found incorporating a legume cover crop into an IWM system in corn reduced Palmer amaranth height, allowed greater grower flexibility in herbicide application timing. Cover crops provide an additional method of early-season weed control to reduce pressure on herbicides alone to create an effective IWM plan.
To achieve these benefits, successful cover crop establishment is critical. Weed suppression, in particular, relies on successful establishment leading to greater cover crop biomass, which can be achieved through an earlier planting date and delayed termination (Mirsky et al. Reference Mirsky, Curran, Mortensen, Ryan and Shumway2011; Mohler and Teasdale Reference Mohler and Teasdale1993). However, herbicides applied during the preceding cash crop may persist in the soil and injure subsequent cover crops (Cornelius and Bradley Reference Cornelius and Bradley2017, Palhano et al. Reference Palhano, Norsworthy and Barber2018), thus reducing establishment and mitigating these benefits. Research on herbicide carryover to cover crops is limited, particularly in regions of the United States such as the mid-Atlantic that have high rates of cover crop adoption.
Herbicide persistence in the soil depends on the herbicide’s interactions with the soil and climate, which affect the physical or chemical removal or degradation of the herbicide (Braschi et al. Reference Braschi, Gessa, Blasioli and Kortekamp2011). Soil texture, CEC, pH, and organic matter (OM) content affect herbicide persistence in soil. The more herbicide is adsorbed to soil, the more likely it is to persist, because less of the herbicide is in soil solution to be taken up by plants, leached, or degraded (Anderson Reference Anderson1983). Generally, greater clay content and CEC increase the amount of herbicide bound to soil particles and decrease herbicide loss to volatilization and other mechanisms (Braschi et al. Reference Braschi, Gessa, Blasioli and Kortekamp2011; Loux and Reese Reference Loux and Reese1993; Ranie et al. Reference Ranie, Duhan, Punia, Yadav and Duhan2018). For example, pyroxasulfone’s half-life ranged from 104 to 134 d in finer-textured clay loam soils, compared with 46 to 48 d in a sandy loam soil in Colorado (Westra et al. Reference Westra, Shaner, Westra and Chapman2014). Fomesafen and imazethapyr carryover is correlated with extended half-life in clay soils (Mueller et al. Reference Mueller, Boswell, Mueller and Steckel2014). The CEC of soil is increased by soil OM and is greater in finer-textured soils (Kerr et al. Reference Kerr, Stahlman and Dille2004). Agrochemicals are strongly adsorbed to OM (Braschi et al. Reference Braschi, Gessa, Blasioli and Kortekamp2011). However, increased OM is associated with increased microbial activity, which can increase herbicide degradation (Gunapala and Scow Reference Gunapala and Scow1998; Kramer et al Reference Kramer, Doane, Horwath and van Kessel2002). Depending on the herbicide, changes in soil pH can increase its persistence. As pH decreases, chemical and microbial breakdown of herbicides slows, so some herbicides will persist longer (Ball et al. Reference Ball, Yenish and Alby2003; Braschi et al. Reference Braschi, Gessa, Blasioli and Kortekamp2011). The net charge of the herbicide molecules and herbicide degradation mechanism can affect how they react in soils of varying pH (Monaco et al. Reference Monaco, Weller and Ashton2002). For example, imidazolinones have a net negative charge at higher pH values, but as the pH decreases, the charge becomes neutral. At a neutral charge, the herbicide is less water soluble (i.e., hydrophobic) and more likely to associate with soil OM, which causes the herbicide to be less available for microbial degradation and persist in the soil longer. Sulfonylurea herbicides are mainly degraded through nonmicrobial processes that are more active at lower soil pH, so as the soil pH increases, the degradation process of sulfonylurea herbicides slows (Monaco et al. Reference Monaco, Weller and Ashton2002).
Environmental conditions, such as moisture and temperature, can affect herbicide persistence in the soil. Herbicide degradation is faster in warm temperatures and with adequate moisture, because of increased microbial activity and chemical processes (Ball et al. Reference Ball, Yenish and Alby2003; Cobucci et al. Reference Cobucci, Prates, Falcao and Rezende1998; Kotoula-Syka et al. Reference Kotoula-Syka, Hatzios, Berry and Wilson1997; Zimdahl Reference Zimdahl2007). However, as soil moisture increases to greater than 41%, microbial activity decreases (Prado and Airoldi Reference Prado and Airoldi1999). Herbicide persistence in the soil can increase in drought conditions because more herbicide is bound to soil particles and less is leached through the soil profile. The interaction between herbicide degradation and environmental conditions is also affected by the herbicide structure and chemical properties.
Herbicide physiochemical properties, such as water solubility, volatility, and binding affinity to soil and OM, affect herbicide persistence in soil. Herbicides that are readily water soluble remain in soil solution and are more likely to be leached or taken up by plants. However, herbicides that are strongly adsorbed to soil may not be displaced by water and some herbicides can react with chemicals in the soil to form water-insoluble compounds (Anderson Reference Anderson1983; Braschi et al. Reference Braschi, Gessa, Blasioli and Kortekamp2011). The amount of herbicide in soil solution also determines the amount at risk for volatilization. Herbicides with higher vapor pressures are more likely to volatilize and less likely to persist in soil (Braschi et al. Reference Braschi, Gessa, Blasioli and Kortekamp2011). Increased soil moisture at the soil surface also increases herbicide volatilization losses (Beestman and Deming Reference Beestman and Deming1974).
The duration that the herbicide remains in the soil is described by the herbicide’s half-life. Herbicide soil half-life is a statistic used to measure the average time it takes to lose half of the herbicide through all possible pathways that act on herbicide in the soil environment (Anderson Reference Anderson1983). The longer the soil half-life, the longer the herbicide has the potential to remain at concentrations high enough to affect plant growth and the greater potential for carryover to future crops (Monaco et al. Reference Monaco, Weller and Ashton2002).
The risk of herbicide carryover to cover crops is influenced by soil, weather, and herbicidal properties, but the sensitivity of cover crop species to a specific herbicide also determines the potential risk of injury from an herbicide. Anderson (Reference Anderson1983) stated that the extent to which a plant responds to herbicide is a measure of its susceptibility to that herbicide. If a plant is more susceptible to the herbicide, it will show a greater response to the herbicide. The susceptibility of the plant is a factor of the selectivity of the herbicide on that plant species. Herbicides are selective in their ability to affect and control plants (Anderson Reference Anderson1983). Herbicide selectivity is complex, and differences can be due to, but not limited to, plant morphology, herbicide dose, plant uptake of the herbicide, translocation within the plant, metabolism within the plant, and the herbicide’s mode of action (Cobb Reference Cobb1992).
Cornelius and Bradley (Reference Cornelius and Bradley2017) in Missouri reported cover crop stand reduction from carryover of 13 herbicides used in soybean and nine herbicides used in corn. Carryover from pyroxasulfone reduced Italian ryegrass and winter oats biomass by 67%. Treatments containing imazethapyr, fomesafen, and flumetsulam resulted in the greatest herbicide carryover symptoms across all cover crop species evaluated (Cornelius and Bradley Reference Cornelius and Bradley2017). In Arkansas, Palhano et al. (Reference Palhano, Norsworthy and Barber2018) observed carryover injury on crimson clover from atrazine, pendimethalin, pyroxasulfone, and S-metolachlor, and on cereal rye and wheat from pyroxasulfone. Other research indicates that atrazine and metsulfuron injured wheat a year after it was applied (Moyer et al. Reference Moyer, Coen, Dunn and Smith2010).
Herbicides applied during the cash crop growing season have the potential to persist in the soil and injure cover crops (Cornelius and Bradley Reference Cornelius and Bradley2017; Palhano et al. Reference Palhano, Norsworthy and Barber2018). Because of the complex interactions and variation in herbicide degradation among climate and soil conditions, research is needed to corroborate previous studies and evaluate herbicide carryover potential to reduce establishment of cover crops commonly used in the mid-Atlantic region of the United States. The objective of this study was to determine the potential for 30 different residual herbicides commonly used in summer cash crops in Virginia to persist in the soil and injure subsequent cover crops.
Materials and Methods
Studies were conducted in 2016 and 2017 to determine the carryover potential of various herbicides to fall-planted cover crops. Locations were Blacksburg, VA, at Kentland Farm (37.19ºN, 80.57ºW) and Suffolk, VA, at the Tidewater Agriculture Research and Extension Center (36.66ºN, 76.73ºW). Studies were repeated at both locations in both years, for a total of 4 site-years. The Blacksburg location is in the New River flood plain. It has a Ross silt loam (fine-loamy, mixed, superactive, mesic Cumulic Hapludolls) containing 33.3% sand, 49.1% silt, and 17.6% clay; a pH of 6.5; 4.4% OM; and a CEC of 10.1 cmol kg−1. The Suffolk location was on a Kenansville loamy sand (loamy, siliceous, subactive, thermic Arenic Hapludults) with 84% sand, 9% silt, and 7% clay; a pH of 6.3; 0.5% OM; and a CEC of 4.5 cmol kg−1. Both sites were tilled with a disc to prepare the seed bed; afterward, glyphosate (Roundup PowerMAX; Monsanto Co., St. Louis, MO) was applied at 1.26 kg ae ha−1 to control weeds before application of the residual herbicides used in this study.
A randomized complete block design with a split-plot treatment structure with four replications was used for the experiments. The main plot was herbicide treatment and the split plot was cover crop species. Plots were 3 m by 9.1 m. Each of the 10 cover crop species (Table 1) was planted in two rows spaced 16.5-cm apart using a Tye drill (AGCO Corp., Duluth, GA). There were 30 residual herbicide treatments: 12 were applied at a PRE timing, 14 wk before cover crop planting (Table 2), and 18 were applied at a POST timing, 10 wk before cover crop planting (Table 3). A nontreated check was included. Herbicides were applied at a PRE timing if their label prevents a POST application or on the basis of when growers usually apply them. Timings were selected to be the shortest, yet realistic, time between application and cover crop planting, representing a realistic, maximum potential for carryover. PRE treatments were applied on June 1 in 2016 and June 2 in 2017 in Suffolk, and on June 13 in 2016 and June 12 in 2017 in Blacksburg. POST treatments were applied on June 27 in 2016 and July 6 in 2017 in Suffolk, and on July 11 in 2016 and 2017 in Blacksburg. These timings were late compared with traditional timings in Virginia but not unrealistic for a late planting or double cropping behind a winter annual cereal or brassica crop. Herbicide treatments were applied using a CO2-pressurized backpack sprayer delivering 140 L ha−1 with a hand boom equipped with 4 XR 11002 nozzles (TeeJet®; Spraying Systems Co., Wheaton, IL) on 46-cm spacing. Cover crops were planted in Suffolk on September 2 in 2016 and on September 13 in 2017, and on September 19 in 2016 and 2017 in Blacksburg. Cover crop seeding dates were early, but still realistic, for the area to simulate the maximum potential for the herbicides used in this trial to affect cover crop establishment.
Table 1. Cover crop species, cultivar, and seeding rate used in the herbicide carryover experiments in Blacksburg and Suffolk, VA, in 2016 and 2017.
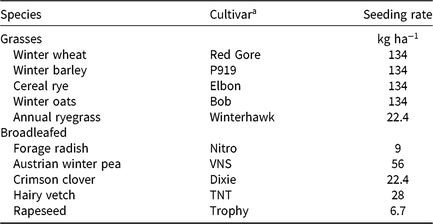
a Abbreviation: VNS, variety not stated.
Table 2. PRE timing herbicide treatments, applied 14 wk before cover crop planting, used in the herbicide carryover experiment in Blacksburg and Suffolk, VA, in 2016 and 2017.

a Location and website only listed at the first mention of the manufacturer.
Table 3. POST timing herbicide treatments, applied 10 wk before cover crop planting, used in the herbicide carryover experiment in Blacksburg and Suffolk, VA, in 2016 and 2017.

a Location and website only listed at the first mention of the manufacturer.
Cover crops were assessed for visible injury 3 and 6 wk after planting (WAP). Injury was scored on a scale of 0 to 100, where 0 is no visible injury and 100 is complete plant necrosis (Frans et al. Reference Frans, Talbert, Marx, Crowley and Camper1986). Aboveground cover crop biomass for 0.6 row m was collected 6 WAP. Cover crop biomass was then dried at 65.5 C for 3 d and weighed.
Data were analyzed using JMP Pro 14 (SAS Institute Inc., Cary, NC). Herbicide treatment, year, and interaction in treatment by year were considered fixed effects, and block was considered a random effect for the visible injury and biomass data analyses. Data were subjected to ANOVA. In many, but not all, instances, treatment by location or year and treatment by species interactions were detected. Herbicide carryover is known to vary by location, because of factors such as soil and weather (Braschi et al. Reference Braschi, Gessa, Blasioli and Kortekamp2011; Loux and Reese Reference Loux and Reese1993; Ranie et al. Reference Ranie, Duhan, Punia, Yadav and Duhan2018) and individual cover crop species (Anderson Reference Anderson1983). Therefore, all data were analyzed by species, location, and when necessary, by year, when a significant interaction in treatment by year existed, including biomass data. Biomass data were subjected to ANOVA followed by Dunnett test at a significance level of α = 0.1, using the nontreated control as the control group.
The nontreated control was excluded from the visible injury analysis. Many treatments resulted in no visible injury (0%), leading to a non-normal, zero-inflated data set. Therefore, a 0 (no injury) or 1 (any injury) was assigned to all visible-injury rating data points and analyzed using a generalized linear model with a binomial distribution and logit link to determine which herbicide treatments resulted in injury to each cover crop species. Based on this analysis, data from treatments that did not cause visible injury were excluded from further analysis. Data from treatments that did cause visible injury were subjected to ANOVA followed by means separation using Tukey honestly significant difference at a significance level of α = 0.1.
Results and Discussion
Cover Crop Biomass Response
Cover crop biomass was positively correlated with precipitation between the 2 yr (Table 4). No differences were detected in cover crop biomass regardless of treatment for all cover crop species relative to the nontreated check (Supplementary Tables S1 and S2). The lack of difference can be explained by the observed injury being more in the form of discoloration and irregular growth and less as stunting. Cornelius and Bradley (Reference Cornelius and Bradley2017) reported biomass reductions from herbicide carryover onto wheat, forage radish, cereal rye, crimson clover, oats, Austrian winter pea, annual ryegrass, and hairy vetch. Palhano et al. (Reference Palhano, Norsworthy and Barber2018) observed results similar to what we found, with no biomass reduction of Austrian winter pea, cereal rye, wheat, hairy vetch, or rapeseed, but they did observe a biomass reduction of crimson clover from atrazine, pendimethalin, pyroxasulfone, and S-metolachlor. Differences between our findings and the results from this previous research are most likely due to differences in the environmental and soil conditions in Virginia compared with those of Arkansas and Missouri.
Table 4. Rainfall totals and average temperature for Suffolk and Blacksburg, VA, in 2016 and 2017 during the studies.

a Abbreviation: WAP; weeks after planting.
Visible Herbicide Carryover to Grass Species
According to the generalized linear model, many treatments resulted in 0% visible injury. Of the treatments that did result in visible injury, less injury was generally observed on grass cover crop species when compared with broadleaf species. There were no differences among treatments that caused injury on all grass species and injury was less than 20% (Tables 5 and 6).
Table 5. Visible injury from herbicide carryover on grass cover crop species 3 wk after planting pooled across year in Suffolk and Blacksburg, VA, in 2016 and 2017.

a Cover crops were planted in early September, approximately 14 wk after PRE and 10 wk after POST treatment application to a fallow field.
b Dashes in the data set indicate omitted data from Tukey honestly significant difference (HSD) means separation analysis, because that herbicide treatment did not significantly injure the cover crop species, according to a generalized linear model with a binomial distribution and logit link test. Within a column, no significant differences were detected among treatments that caused injury according to Tukey HSD at α = 0.1 level of significance.
c Derived from the ANOVA analysis comparing treatments that had some injury according to the generalized linear model with a binomial distribution and logit link test.
Table 6. Visible injury from herbicide carryover on grass cover crop species 6 wk after planting in Suffolk and Blacksburg, VA, in 2016 and 2017.
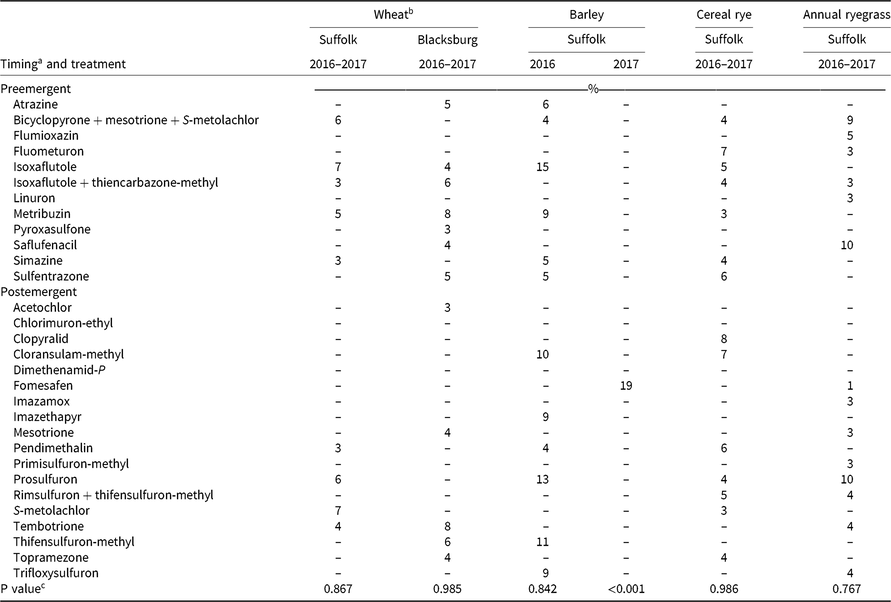
a Cover crops were planted in early September, approximately 14 wk after PRE and 10 wk after POST treatment application to a fallow field.
b Dashes in the data set indicate omitted data from Tukey honestly significant difference (HSD) means separation analysis, because that herbicide treatment did not significantly injure the cover crop species, according to a generalized linear model with a binomial distribution and logit link test. Within a column, no significant differences were detected among treatments that caused injury according to Tukey HSD at α = 0.1 level of significance.
c Derived from the ANOVA analysis comparing treatments that had some injury according to the generalized linear model with a binomial distribution and logit link test.
In general, herbicide visible injury was greater in 2016 than in 2017. Wheat was injured by 27 of the 30 herbicides evaluated in this study; however, only saflufenacil, isoxaflutole, and imazethapyr resulted in greater than 15% injury 3 WAP, and no herbicide resulted in greater than 10% injury 6 WAP (Tables 5 and 6). Dimethenamid-P, rimsulfuron plus thifensulfuron-methyl, and flumioxazin resulted in 0% injury on wheat at both locations and rating timings. Injury from isoxaflutole and imazethapyr was expected because of 4-mo rotation restrictions to wheat according to their labels (Anonymous 2017a; Anonymous 2019).
Saflufenacil resulted in 18% injury on wheat in Blacksburg 3 WAP (Table 5). Saflufenacil is registered for use preplant in wheat, but injury can occur to some sensitive varieties (Anonymous 2017b). Results were similar to those reported by Cornelius and Bradley (Reference Cornelius and Bradley2017) with injury from atrazine, imazethapyr, and isoxaflutole. However, unlike Cornelius and Bradley (Reference Cornelius and Bradley2017) and Palhano et al. (Reference Palhano, Norsworthy and Barber2018), wheat at the Blacksburg site was not injured by pyroxasulfone, topramezone, or rimsulfuron plus thifensufluron-methyl.
Acetochlor, chlorimuron ethyl, and imazamox did not result in injury on barley at either location, regardless of rating timing. All other herbicides resulted in significant injury on barley in Suffolk 3 WAP, but only cloransulam-methyl resulted in greater than 15% injury (Table 5). Cloransulam-methyl has a 12-mo rotational interval when used on barley (Anonymous 2017c). There were no herbicides that injured barley more than 10% by 3 WAP (Table 5), and no injury was observed on barley 6 WAP in Blacksburg. However, isoxaflutole injured barley greater than 15% in Suffolk at 6 WAP in 2016. Only fomesafen resulted in injury (19%) on barley in Suffolk 6 WAP in 2017 (Table 6).
No herbicide treatment resulted in significant cereal rye injury in Blacksburg. Pyroxasulfone, prosulfuron, isoxaflutole, fluometuron, and cloransulam-methyl resulted in, respectively, 10%, 12%, 14%, 14%, and 17% cereal rye injury in Suffolk 3 WAP (Table 5). Prosulfuron injury can be explained by its average half-life of 118 d when the soil pH is between 6.1 and 6.6 (Shaner Reference Shaner2014). Cereal rye injury was observed from 14 herbicides 6 WAP in Suffolk, but injury was less than 10% (Table 6). Palhano et al. (Reference Palhano, Norsworthy and Barber2018) reported similar results with carryover injury observed on cereal rye from pyroxasulfone. Similarly, Cornelius and Bradley (Reference Cornelius and Bradley2017) observed carryover to cereal rye from cloransulam-methyl and isoxaflutole. Cereal rye was not injured to the level observed from atrazine (81% to 90%) by Ivany et al. (Reference Ivany, Sadler, Kimball and McRae1985), likely due to warmer and wetter conditions in Virginia compared with Canada.
In Suffolk compared with the Blacksburg site, greater injury on barley from cloransulam-methyl and isoxaflutole and on cereal rye from cloransulam-methyl, fluometuron, isoxaflutole, and pyroxasulfone can be attributed to greater amounts of OM in the Blacksburg site soil promoting more microbial activity to degrade the herbicides in the soil (Gunapala and Scow Reference Gunapala and Scow1998; Kramer et al Reference Kramer, Doane, Horwath and van Kessel2002). Cloransulam-methyl, fluometuron, isoxaflutole, and pyroxasulfone persistence in the soil is heavily influenced by microbial activity (Shaner Reference Shaner2014).
Injury on oats was only observed in Blacksburg at 3 WAP from seven of 30 herbicides, but none of the herbicides resulted in greater than 10% injury (Table 5). No visible injury was observed in Suffolk 3 or 6 WAP at either location.
Annual ryegrass was injured by 17 herbicides in Suffolk 3 WAP and by five herbicides in Blacksburg 3 WAP, but none of the herbicides resulted in greater than 15% visible injury (Table 5). Annual ryegrass was not injured 6 WAP in Blacksburg and no herbicide resulted in greater than 10% injury in Suffolk 6 WAP.
Visible Herbicide Carryover to Broadleaf Species
Hairy vetch was not rated in Suffolk in 2016 and Austrian winter pea and rapeseed were not rated in Blacksburg in 2017, due to a poor stand in the nontreated check. For these species, data were only analyzed from the locations for which 2 yr of data existed. Stand establishment challenges also highlight real-world issues growers face in conservation tillage systems.
Fomesafen resulted in the most injury on forage radish of all herbicides tested in Suffolk at 3 and 6 WAP in 2017, and Blacksburg at 6 WAP in 2017, with 24%, 24%, and 58% injury, respectively (Tables 7 and 8). Fomesafen also resulted in 10% injury on forage radish 3 WAP in Blacksburg (Table 7). Forage radish injury from fomesafen was greater in 2017 than in 2016, most likely as a result of less total rainfall in 2017 at both locations (Table 4). Fomesafen degrades much slower under aerobic conditions compared with anaerobic conditions, so lower soil moisture can increase herbicide persistence (Cobucci et al Reference Cobucci, Prates, Falcao and Rezende1998; Shaner Reference Shaner2014). Cornelius and Bradley (Reference Cornelius and Bradley2017) also observed the greatest amount of injury on radish from fomesafen. Greater injury from fomesafen on forage radish in Blacksburg compared with Suffolk can be attributed to fomesafen’s longer half-life in clay soils; the soil at the Blacksburg site has a greater clay content (Mueller et al Reference Mueller, Boswell, Mueller and Steckel2014).
Table 7. Visible injury from herbicide carryover on broadleaf cover crop species 3 wk after planting pooled across year in Suffolk and Blacksburg, VA, in 2016 and 2017.a

a Cover crops were planted in early September, approximately 14 wk after PRE and 10 wk after POST treatment application to a fallow field.
b Dashes in the data set indicate omitted data from Tukey honestly significant difference (HSD) means separation analysis, because that herbicide treatment did not significantly injure the cover crop species according to a generalized linear model with a binomial distribution and logit link test. Shared letters within column indicate no significant difference according to Tukey HSD at α = 0.1 level of significance.
c Derived from the ANOVA analysis comparing treatments that had some injury according to the generalized linear model with a binomial distribution and logit link test.
Table 8. Visible injury from herbicide carryover on broadleaf cover crop species 6 wk after planting in Suffolk and Blacksburg, VA, in 2016 and 2017.

a Cover crops were planted in early September, approximately 14 wk after PRE and 10 wk after POST treatment application to a fallow field.
b Dashes in the data set indicate omitted data from Tukey honestly significant difference (HSD) means separation analysis, because that herbicide treatment did not significantly injure the cover crop species according to a generalized linear model with a binomial distribution and logit link test. Shared letters within column indicate no significant difference according to Tukey HSD at α = 0.1 level of significance.
c Derived from the ANOVA analysis comparing treatments that had some injury according to the generalized linear model with a binomial distribution and logit link test.
Trifloxysulfuron resulted in greater than 15% injury in Suffolk (23%) and Blacksburg (18%) 3 WAP and in Blacksburg 6 WAP (19%) on forage radish (Tables 7 and 8). Sulfonylurea persistence is determined mainly by soil pH, which determines the degradation rate by chemical hydrolysis and soil microbes. As soil pH increases to 7.0, sulfonylurea persistence increases because of increased anionic forms of the herbicide resulting in decreased microbial degradation and dissipation (Grey and McCullough Reference Grey and McCullough2012, Shaner Reference Shaner2014). Even though our soil pH was below 7, our results suggest the higher soil pH at the Blacksburg site compared with the Suffolk site led to decreased degradation of trifloxysulfuron and thus greater injury at the Blacksburg site. Trifloxysulfuron can control wild radish (Raphanus raphanistrum L.), and it has a 12-mo rotational restriction to radish following an application in cotton (Anonymous 2015).
Atrazine resulted in greater than 10% injury on forage radish in Blacksburg 6 WAP in 2016 and in Suffolk 6 WAP in 2016 with 20% and 13% injury, respectively (Table 8). Burnside et al. (Reference Burnside, Fenster and Wicks1971) reported atrazine can persist in the soil for more than 1 yr, which explains the injury on forage radish and multiple other cover crop species (Tables 5–8), but Cornelius and Bradley (Reference Cornelius and Bradley2017) did not observe atrazine carryover to forage radish. We observed forage radish injury from isoxaflutole (up to 13%) and rimsulfuron plus thifensulfuron-methyl (up to 12%) similar to Cornelius and Bradley (Reference Cornelius and Bradley2017) (Tables 7 and 8).
Austrian winter pea was injured by 15 herbicides, but only bicyclopyrone plus mesotrione plus S-metolachlor resulted in greater than 10% injury in Suffolk 3 WAP (Table 7). No herbicides resulted in significant visible injury 6 WAP. Of all broadleaf cover crop species evaluated, Austrian winter pea was generally injured less across all herbicides in Suffolk.
Trifloxysulfuron resulted in the greatest crimson clover injury (30%) 6 WAP in Suffolk in 2017, followed by linuron and cloransulam-methyl at 10% injury (Table 8). Trifloxysulfuron also resulted in 26% injury in Suffolk 3 WAP, 13% in Blacksburg 3 WAP, 13% in Suffolk 6 WAP in 2016, and 15% in Blacksburg 6 WAP in 2016 (Tables 7 and 8). Results were different compared with Palhano et al. (Reference Palhano, Norsworthy and Barber2018), who saw no trifloxysulfuron carryover injury to crimson clover.
Bicyclopyrone plus mesotrione plus S-metolachlor resulted in greater than 10% injury on crimson clover in Suffolk 6 WAP in 2016 (11%) and in Blacksburg 6 WAP in 2017 (13%) (Table 8). Flumioxazin resulted in injury in Suffolk 3 WAP (11%) and 6 WAP (18%) in 2016 (Tables 7 and 8). Flumioxazin is primarily degraded in the soil by microbes, and soil OM increases microbial activity, which explains why injury was observed in Suffolk and not Blacksburg, because Suffolk has much lower soil OM (Gunapala and Scow Reference Gunapala and Scow1998; Kramer et al Reference Kramer, Doane, Horwath and van Kessel2002; Shaner Reference Shaner2014)
There was no significant injury on hairy vetch in Blacksburg 3 WAP. There was no injury greater than 10% on hairy vetch 6 WAP in Blacksburg, and only seven herbicides caused significant injury (Table 8).
Rapeseed was injured by 27 herbicides, seven of which resulted in 15% or greater injury 3 WAP in Suffolk: simazine, bicyclopyrone plus mesotrione plus S-metolachlor, mesotrione, isoxaflutole, prosulfuron, rimsulfuron plus thifensulfuron-methyl, and sulfentrazone (Table 7). Only isoxaflutole and prosulfuron resulted in greater than 15% injury on rapeseed 6 WAP in Suffolk, with 19% and 17% visible injury, respectively (Table 8). Low amounts of soil OM at the Suffolk site can explain injury from isoxaflutole. Isoxaflutole degradation depends on microbial activity, which is influenced by soil OM (Gunapala and Scow Reference Gunapala and Scow1998; Kramer et al Reference Kramer, Doane, Horwath and van Kessel2002; Shaner Reference Shaner2014). Injury from prosulfuron can be attributed to its average half-life of 118 d in a soil with a pH of 6.1 to 6.6, which is the range of our soils for this study (Shaner Reference Shaner2014).
Management Implications
Even though there were no differences detected in cover crop biomass by herbicide treatment, visual injury suggests there is the potential for some residual herbicides applied in the previous cash crop growing season to affect cover crop establishment. All herbicides resulted in visible injury on at least one cover crop species, but most injury was low and often below what would be considered unacceptable by commercial growers. Thus, with limited exceptions, small grains and hairy vetch can be planted without significant concern of herbicide carryover from commonly used residual herbicides affecting cover crop establishment or biomass accumulation.
Fomesafen resulted in the greatest injury among all herbicides, with 58% visible injury on forage radish in Blacksburg in 2017, suggesting that forage radish should not be used as a cover crop if fomesafen was applied in the previous cash crop. Growers should avoid planting forage radish after applications of atrazine and trifloxysulfuron, because our results show they have the potential to interfere with cover crop establishment. Crimson clover should not be planted after an application of trifloxysulfuron in the previous growing season. Flumioxazin also injured crimson clover in Suffolk on a coarser textured soil compared with Blacksburg’s finer textured soil, so crimson clover should be avoided on sandy soils where flumioxazin was applied. Prosulfuron and isoxaflutole resulted in 17% and 19% injury 6 WAP, respectively, on rapeseed, suggesting it has the potential to persist in the soil and affect establishment when applied during the previous cash crop growing season.
Some results from previous studies were corroborated, and some of our results differed. Herbicide persistence varies with location (e.g., soil type) and year (e.g., weather) (Braschi et al. Reference Braschi, Gessa, Blasioli and Kortekamp2011). Results do support that soil texture has an impact on herbicide carryover potential, but the interaction between the herbicide and all the factors associated with its ability to persist in the soil is complex. Fomesafen resulted in the greatest injury at Blacksburg on a finer-textured soil with more OM; this result is supported by fomesafen’s extended half-life in clay soils documented by Mueller et al. (Reference Mueller, Boswell, Mueller and Steckel2014). However, flumioxazin and trifloxysulfuron resulted in greater injury at the Suffolk site, on a coarser-textured soil, suggesting that herbicides differ in how they interact with soil and the climate. The interaction between herbicide persistence and cover crop injury is complex and site specific, so additional localized research is needed. Little research was found on the carryover potential and the factors influencing the persistence and degradation of the sulfonylurea herbicides used in this study, suggesting the need for additional research.
Acknowledgements
The authors thank the U.S. Department of Agriculture, Natural Resource Conservation Service (award no. 69-33A7-16-1160), the Virginia Agricultural Experiment Station, and the Hatch Program of the National Institute of Food and Agriculture, U.S. Department of Agriculture, for providing funding for this research. The authors also thank Dr. Jacob Barney for help in data analysis. Although no specific funding was received related to this manuscript from manufacturers of products mentioned, funding has been provided to Virginia Tech from the following sponsors in support of M.L.F.’s research and extension program, either through unrestricted gifts, research contracts, or grants: AMVAC Chemical Corporation, BASF Corporation, Bayer CropScience, Dow AgroSciences, E.I. du Pont de Nemours and Company, FMC Corporation, Monsanto Company, Syngenta Crop Protection, and United Phosphorus Inc.
Supplementary material
For supplementary material/s referred to in this article, please visit https://doi.org/10.1017/wet.2019.79