Introduction
Chile peppers, which are domesticated species within the Capsicum genus, are important components of the agricultural economy and heritage in New Mexico (Bosland Reference Bosland2015). In 2016, chile peppers were planted on 3,720 ha in New Mexico and provided over $50 million in cash receipts (USDA NASS 2017). In a typical year, chile pepper acreage in New Mexico is approximately 45% of the United States total for this crop (USDA NASS 2017). Chile peppers harvested in New Mexico are approximately 30% of those harvested annually in the United States (USDA NASS 2017).
Maximum chile pepper yields require prolonged periods of weed control following planting. This is because chile peppers are slow to establish and susceptible to early-season weed competition (Amador-Ramirez Reference Amador-Ramirez2002; Schroeder Reference Schroeder1992, Reference Schroeder1993). In addition to reducing fruit yield, weeds potentially inhibit sustainable chile pepper production by harboring chile plant pests or diseases (Rodriguez-Alvarado et al. Reference Rodriguez-Alvarado, Fernandez-Pavia, Creamer and Liddell2002; Sanogo et al. Reference Sanogo, Schroeder, Thomas, Murray, Schmidt, Beacham, Fiore and Liess2013) or reducing harvest efficiency (Schroeder Reference Schroeder1993; Schutte Reference Schutte2017). PRE herbicides are an effective means for controlling weeds in chile pepper in New Mexico (Skaggs et al. Reference Skaggs, Decker and VanLeeuwen2000); however, New Mexico farmers have few options for residual herbicides after crop emergence.
Flumioxazin is an N-phenylphthalimide herbicide that controls emerged and emerging weeds by inhibiting protoporphyrinogen IX oxidase (EC 1.3.3.4) (Yoshida et al. Reference Yoshida, Sakaki, Sato, Haga, Nagano, Oshio and Kamoshita1991), which consequently creates singlet oxygen that initiates lipid peroxidation (Cobb and Reade Reference Cobb and Reade2010). Flumioxazin can be applied in chile pepper grown in the United States where Special Local Needs registrations have been obtained. Special Local Needs registrations for flumioxazin in chile pepper include preplant applications on fallow beds (Anonymous 2015), preplant applications to row middles between plastic-mulched beds (Ozores-Hampton et al. Reference Ozores-Hampton, Boyd, McAvoy, Miller, Noling and Vallad2017), and shielded or hooded applications to row middles before or after transplanting (Anonymous 2011). Flumioxazin is not currently registered for chile pepper in New Mexico because this combination of crop and region has not been the setting for flumioxazin evaluations. Accordingly, little is known about the risk of crop injury from flumioxazin applied in chile pepper in New Mexico.
Soil applications of flumioxazin can severely injure crops under conditions that combine (1) environmental stresses that limit metabolism of the herbicide and (2) excessive water leading to herbicide leaching and root uptake (Burke et al. Reference Burke, Askew and Wilcut2002; Hutchinson et al. Reference Hutchinson, Boydston, Ransom, Tonks and Beutler2005; Niekamp and Johnson Reference Niekamp and Johnson2001; Taylor-Lovell et al. Reference Taylor-Lovell, Wax and Nelson2001). Flumioxazin injury from root uptake might be especially common following heavy rainfall on soil with high sand content and low levels of organic matter (Boyd Reference Boyd2014). This is because flumioxazin readily desorbs from soil with low capacities for sorption after an increase in soil water content (Alister et al. Reference Alister, Rojas, Gomez and Kogan2008; Ferrell et al. Reference Ferrell, Vencill, Xia and Grey2005). Soil applications of flumioxazin might cause injury to chile pepper in New Mexico because this state features coarse-textured soils with low levels of organic matter and surface irrigation systems that flood areas between raised beds (i.e., furrow irrigation).
The objective for this study was to determine if POST-directed applications of flumioxazin reduce fruit yield for chile pepper produced on coarse- and fine-textured soils irrigated by furrow. This objective was addressed with a field study that compared flumioxazin effects on fruit yield against a commercial standard (POST-directed carfentrazone) and the no POST–directed herbicide control. Based on results from the field study, a greenhouse study was conducted to determine if soil organic matter content influences the potential for flumioxazin-induced crop injury on coarse-textured soil.
Materials and Methods
Field Study
The field study was conducted for 3 yr at two New Mexico State University (NMSU) research farms: Leyendecker Plant Science Research Center near Las Cruces, NM (32°12.131’ N, 106°44.771’ W) and the Agricultural Science Center at Los Lunas, NM (34°46.287’ N, 106°45.578’ W). Fields where studies were conducted differed annually. The Las Cruces study site featured fine-textured soil, and the Los Lunas study site featured coarse-textured soil (Table 1). Each field was subjected to a sequence of preparatory procedures that included tilling, laser leveling, listing, and shaping raised beds into rows spaced 1-m apart at Las Cruces and 0.75-m apart at Los Lunas.
Table 1 Soil properties and soil classifications for study site-years.

a Organic matter and textural information was provided by a commercial laboratory (Ward Laboratories, Inc., Kearney, NE).
Chile pepper was grown using practices typical for the specific region. At Las Cruces, chile pepper was seeded into raised beds at 6.7 kg ha–1 to a depth of 2 cm using a mechanical planter (MaxEmerge Plus, John Deere, Moline, IL) on April 23, 2015 and April 27, 2016. At times of seeding at Las Cruces, fields were treated with napropamide at 1.1 kg ai ha–1. POST control of weeds at Las Cruces was accomplished with cultivation, hand hoeing, and herbicide (clethodim at 140 g ai ha–1). At Los Lunas, chile pepper was seeded into raised beds at 5.8 kg ha–1 to a 2-cm depth using a mechanical planter (Cole Planet Jr., Cole Planter Company, Albany, GA). Seeding at Los Lunas took place on May 12, 2015, May 17, 2016, and May 15, 2017. Weeds at Los Lunas were controlled with cultivation and hand hoeing. Site-specific weed control programs resulted in weed-free conditions throughout chile pepper growing seasons.
For each site-year, six chile pepper cultivars were arranged in one field separated into sections (1 cultivar per section). Within each cultivar section, treatments were arranged in a randomized complete block design with four replications. Experimental units were plots 6-m long by three beds wide. On each side of center beds, a herbicide treatment was applied using a CO2-powered backpack sprayer calibrated to deliver 187 L ha–1 and equipped with a hooded, even-flat nozzle (TeeJet 8002EVS, TeeJet Technologies, Wheaton, IL). Hereafter, center beds with herbicide treatments are referred to as “treatment rows.” Beds flanking treatment rows were designated as border rows. Herbicide treatments were implemented at times relative to crop thinning, which occurred 9 to 10 wk after crop seeding. In 2015, chile pepper cultivars were ‘AZ 1904’, ‘Big Jim’, ‘Jalapeño’, ‘NM 6-4’, ‘Paprika LB-25’, and ‘Sandia’. In 2016 and 2017, chile pepper cultivars were ‘AZ 88’, Big Jim, Jalapeño, NM 6-4, Paprika LB-25, and Sandia. The selected cultivars are commonly grown in New Mexico (SJ Walker, personal communication).
Treatments at Las Cruces included the no POST–directed herbicide control and the following four POST-directed herbicides applied to the raised beds: (1) flumioxazin at 107 g ai ha–1 applied 4 wk after crop thinning, (2) carfentrazone at 35 g ai ha–1 applied 4 wk after crop thinning, (3) flumioxazin at 70 g ai ha–1 applied 4 and 6 wk after crop thinning, and (4) carfentrazone at 35 g ai ha–1 applied 4 and 6 wk after crop thinning. Carfentrazone was included because this herbicide is registered for POST-directed applications in chile pepper and is a commercial standard.
Treatments at Los Lunas in 2015 included the no POST–directed herbicide control and the following two POST-directed herbicides applied to raised beds 4 wk after crop thinning: (1) flumioxazin at 107 g ai ha–1 and (2) carfentrazone at 35 g ai ha–1. During 2016 and 2017 at Los Lunas, treatments included the absence of POST-directed herbicides and the following three POST-directed herbicides applied 4 wk after crop thinning: (1) flumioxazin at 107 g ai ha–1 applied to raised beds, (2) flumioxazin at 107 g ai ha–1 applied to furrows, and (3) carfentrazone at 35 g ai ha–1 applied to raised beds. Carfentrazone treatments were applied with crop oil concentrate at 1% v/v (Agri-Dex®, Helena Chemical Company, Collierville, TN). Flumioxazin treatments were applied with nonionic surfactant at 1% v/v (Dyne-Amic®, Helena Chemical Company, Collierville, TN). Herbicide trade names and product concentrations were as follows: flumioxazin, Chateau® SW, 0.51 g ai g–1 (Valent U.S.A. LLC, Walnut Creek, CA; http://www.valent.com); carfentrazone, Aim® EC, 240 g ai L–1 (FMC Corporation, Philadelphia, PA; http://www.fmccrop.com).
At 14 days after spraying (DAS), chile pepper plants in treatment rows were evaluated for visual estimates of herbicide injury. Fresh weights of marketable chile pepper fruits were determined in September to early October. Fruits were harvested by hand from central, 4-m sections of treatment rows. Criteria for marketable fruits were specific to cultivar, but, in general, marketable fruits were straight and free of disease symptoms. Chile peppers were harvested once per cultivar per site-year.
Greenhouse Study
A study was conducted in a greenhouse located at the NMSU Leyendecker Plant Science Research Center. The greenhouse was set to maintain an air temperature of 24 C (± 3 C). A photoperiod of 14 hr light and 10 hr dark was maintained by operating high-intensity discharge metal halide lamps from 6:00 to 8:00 AM and from 5:00 to 8:00 PM. The study occurred from December 14, 2016 to March 22, 2017 and was repeated from January 4, 2017 to April 11, 2017. Each experimental run was arranged in a randomized complete block with four replications. Experimental units were 8.7-L pots (25-cm diam, 23-cm depth) containing one chile pepper plant. Plants were started from seeds buried 2 cm in soil within the pots. The chile pepper cultivar was NM 6-4. Soil moisture levels were evaluated daily. When soil surfaces appeared dry, individual pots were irrigated with 300 ml of water. Three days prior to herbicide applications (described below), all pots were watered to saturation and not watered again until after herbicide application. Chile pepper plants were grown for 8 wk before herbicide treatments were applied.
Treatments were factorial combinations of two soil types and four flumioxazin rates. The experimental soils were obtained from two fields at the NMSU Agricultural Science Center at Los Lunas, NM. One soil was Gila series (Thermic Typic Torrifluvents) collected from an approximate 30-yr stand of cottonwood trees (Populus fremontii S. Wats.). The other soil was Bluepoint series (Thermic Typic Torripsamments) collected from an annual crop field that was fallow for 4 consecutive yr prior to this study. Soils were sieved to remove debris that did not pass through a 4-mm screen. Soil samples were characterized by a commercial laboratory (Ward Laboratories Inc., Kearney, NE). Analyses indicated that the cottonwood stand provided soil that was 77% sand, 8% silt,and 15% clay, with 2.5% organic matter. The fallow field provided soil that was 79% sand, 4% silt, and 17% clay, with 0.7% organic matter. Hereafter, soil from the cottonwood stand is referred to as “high–organic-matter soil” and soil from the fallow field referred to as “low–organic-matter soil.” Flumioxazin treatments were 0, 70, 107, and 177 g ai ha–1. The 70, 107, and 177 g ai ha–1 treatments included nonionic surfactant at 1% v/v.
Flumioxazin treatments were administered by first preparing 2-L stock solutions necessary for applications at 187 L ha–1. Then, 1-ml stock solution was mixed with 200 ml of water to form the solution that was applied to a soil surface. Soil was then wetted with 150 ml of water. Herbicide and irrigation solutions did not contact aboveground portions of chile pepper plants.
At 14 d after herbicide application, chile pepper plants were evaluated for visual estimates of herbicide injury. Injury symptoms included stunting, leaf chlorosis, and leaf necrosis. Symptom ratings were based on a scale of injury ranging from 1 to 10, with 1 = none and 10 = severe. After rating, aboveground biomass was harvested and immediately weighed to determine plant fresh biomass. Aboveground biomass was then dried at 65 C for 7 d to determine plant dry biomass.
Data Analysis
All statistical analyses were performed with R software v. 3.0.1 (R Development Core Team 2008). For the field study, cultivars at a site-year were separately assessed for herbicide treatment effects on fruit yield. This was done using ANOVA followed by Tukey’s honest significant difference (HSD) tests.
For the greenhouse study, relationships between flumioxazin rate and plant biomass were modeled with:

where y is fresh biomass or dry biomass, a is fresh or dry biomass in the absence of flumioxazin, b is a rate constant, and x is flumioxazin application rate. Relationships between flumioxazin rate and visual estimates of herbicide injury were modeled with:

where y is injury rating, y 0 is injury rating in the absence of flumioxazin, a is the asymptotic maximum injury rating constrained to be ≤10, b is a rate constant, and x is flumioxazin rate. Equation 1 and Equation 2 were fit using the R package nls.
Models (Equation 1 and Equation 2) were first fit separately for soil type within a replicate. Model parameters (a, b, and y 0) were then assessed for soil type effects using linear mixed models (LMMs) developed with the R package lme4. In these LMMs, soil type was the fixed effect. Random effects were run and replicate within run. If LMMs indicated that a parameter was conditioned by soil type, the corresponding model was refit using means within soil type. If soil type did not affect model parameters, the model was refit using means across soil types.
Results and Discussion
Field Study
POST-directed applications of both carfentrazone and flumioxazin caused speckling to lower leaves on chile pepper plants at 14 DAS. This injury symptom is considered characteristic damage for protoporphyrinogen IX oxidase inhibitors (Shumway et al. Reference Shumway, Scott and Boyd2018).
POST-directed applications of flumioxazin did not reduce chile fruit yield on fine-textured soil (Las Cruces study site; Table 2). On coarse-textured soil (Los Lunas study site), POST-directed flumioxazin on raised beds reduced fruit yield of some cultivars in 2015 and 2017, but not in 2016 (Table 3). Relative to the no POST–directed herbicide control, combinations of cultivars and years with flumioxazin-induced reductions in fruit yield at Los Lunas included AZ 88 in 2017, Big Jim in 2015, NM 6-4 in 2015, Paprika LB-25 in 2015, and Sandia in 2015. These cultivar-years, with the exception of Sandia in 2015, also featured flumioxazin-induced reductions in fruit yield relative to POST-directed carfentrazone. Although fruit yields for Sandia in 2015 were similar between flumioxazin and carfentrazone treatments, POST-directed applications of flumioxazin caused reductions in Sandia fruit yield (relative to the no POST–directed herbicide control) that did not occur with POST-directed applications of carfentrazone.
Table 2 Fruit yields for chile pepper cultivars treated with herbicides applied POST-directed at the Leyendecker Plant Science Research Center near Las Cruces, NM. Cultivars were kept free of weeds with combinations of cultivation, hand hoeing, PRE applications of napropamide (1.1 kg ai ha–1) and POST applications of clethodim (140 g ai ha–1). Data are means of four replications. Within a cultivar-year, means followed by the same letter are not significantly different according to Tukey’s HSD test (α = 0.05).

a The study included a treatment in which POST-directed herbicides were not applied.
Table 3 Fruit yields for chile pepper cultivars treated with herbicides applied POST-directed at the Agricultural Science Center at Los Lunas, NM. Cultivars were kept free of weeds with combinations of cultivation and hand hoeing. Data are means of four replications. Within a cultivar-year, means with the same letter are not significantly different according to Tukey’s HSD test (α = 0.05).

a The study included a treatment in which POST-directed herbicides were not applied.
b Dashed lines indicate that the treatment was not evaluated in 2015.
Flumioxazin-induced reductions in fruit yield at Los Lunas were most severe for NM 6-4 in 2015.For this cultivar-year at Los Lunas, flumioxazin directed to raised beds caused a 43% reduction in fruit yield relative to chile pepper without a POST-directed herbicide. NM 6-4 fruit yield was not affected by POST-directed applications of flumioxazin in 2017, which was a year when flumioxazin-induced reductions in fruit yield occurred for AZ 88. Thus, NM 6-4 was not consistently sensitive to POST-directed flumioxazin, and cultivar was not a primary determinant of flumioxazin-induced yield loss. Year-to-year variability in both soil organic matter content and occurrences of flumioxazin-induced fruit yield loss at Los Lunas suggested that chile pepper plants on coarse-textured, low–organic-matter soil were more susceptible to POST-directed flumioxazin than those on coarse-textured, high–organic-matter soil. Accordingly, spatial variability in soil organic matter, resulting in low levels of soil organic matter in the field section where NM 6-4 was grown, might have contributed to this cultivar’s high degree of sensitivity to flumioxazin in 2015 at Los Lunas.
Flumioxazin-induced reductions in fruit yield were associated with plant stunting and yellowing. Across the 3-yr study, chile pepper fruit yield was reduced in 9% (5 out of 54) of flumioxazin treatments. Reductions in fruit yield occurred only on coarse-textured soil when flumioxazin was applied to raised beds. When flumioxazin was applied to row middles on coarse-textured soil, fruit yield was similar to both POST-directed carfentrazone and chile pepper without a POST-directed herbicide. Compared to chile pepper without a POST-directed herbicide, chile pepper fruit yields were not affected by POST-directed applications of carfentrazone, regardless of soil type (Table 2, Table 3).
Greenhouse Study
A greenhouse study was conducted to determine if soil organic matter content influences the potential for flumioxazin-induced crop injury on coarse-textured soil. In this study, flumioxazin was applied to bases of chile pepper plants grown on two coarse-textured soils that differed in organic matter content. For both soil types, increasing flumioxazin rate decreased plant fresh biomass and plant dry biomass (Figure 1). Flumioxazin caused greater declines in fresh biomass on the low–organic-matter soil compared to the high–organic-matter soil. For dry biomass, flumioxazin effects were not conditioned by soil organic matter. Visual estimates of flumioxazin injury were influenced by soil organic matter content. Flumioxazin caused more stunting, leaf chlorosis, and leaf necrosis on the low–organic-matter soil. Results from the greenhouse study indicated that susceptibility of chile pepper to injury was greater on a coarse soil with low organic matter than on a coarse soil with high organic matter. Accordingly, variability in soil organic matter content might have contributed to annual differences in flumioxazin-induced fruit yield loss at Los Lunas.
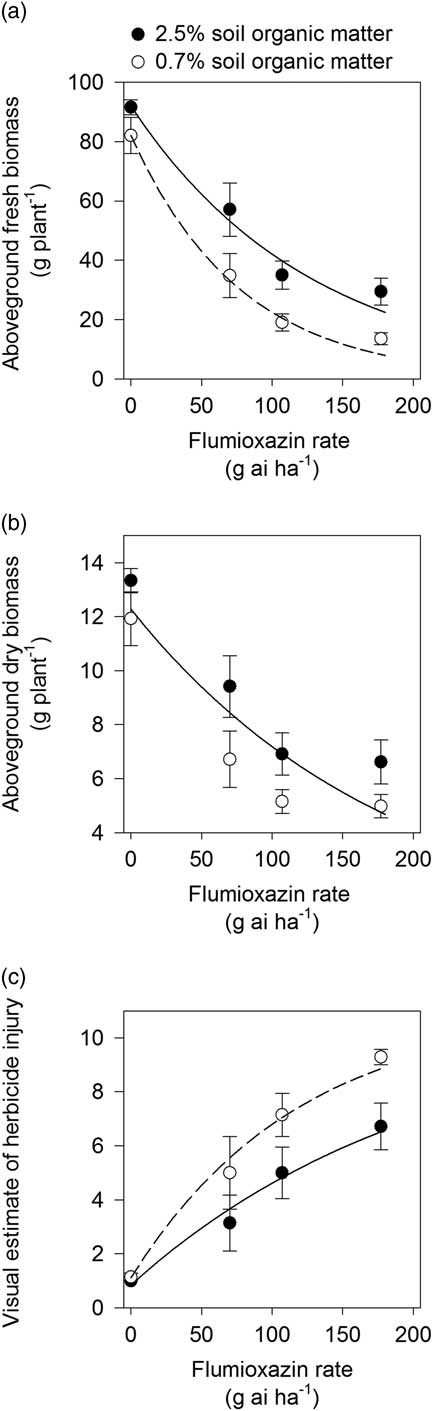
Figure 1 Chile pepper plant responses to flumioxazin applied to soil at the bases of 8-wk-old plants. The study was conducted in a greenhouse and included two soil treatments: (1) sandy loam soil with 2.5% organic matter, indicated with closed circles, and (2) sandy loam soil with 0.7% organic matter, indicated with open circles. Plant responses measured at 2wk after flumioxazin application included (a) aboveground fresh biomass, (b) aboveground dry biomass, and (c) visual estimates of flumioxazin injury. Data points are means (± SE, N = 8). Fitted lines with pseudo-r2 are as follows: fresh biomass, 2.5% soil organic matter, y = 91.2e–0.007x, r2 = 0.97; fresh biomass, 0.7% soil organic matter, y = 81.7e–0.001x, r2 = 0.98; dry biomass, 2.5% and 0.7% soil organic matter, y = 12.3e–0.005x, r2 = 0.83; injury rating, 2.5% soil organic matter, y = 0.84 + 10*(1–e(–0.005x)), r2 = 0.98; injury rating, 0.7% soil organic matter, y = 1.12 + 10*(1–e(–0.008x)), r2 = 0.98. Model fitting procedures indicated that soil organic matter influenced fresh biomass and injury rating responses to flumioxazin but did not affect dry biomass responses to flumioxazin (hence only a single fitted line on Figure 1b).
The combined results of greenhouse and field studies concurred with previous studies indicating that sorption of flumioxazin was positively correlated with soil organic matter content and negatively correlated with soil sand content (Alister et al. Reference Alister, Rojas, Gomez and Kogan2008; Ferrell et al. Reference Ferrell, Vencill, Xia and Grey2005). These characteristics of flumioxazin sorption are consistent with this herbicide’s physicochemical properties. Specifically, flumioxazin is a nonionic compound with low water solubility (1.79 m L–1; Shaner et al. Reference Shaner, Jachetta, Senseman, Burke, Hanson, Jugulam, Tan, Glenn and Turner2014), making it likely that soil organic matter is the primary sorbent for this herbicide (Wauchope et al. Reference Wauchope, Yeh, JBHJ, Kloskowski, Tanaka, Rubin, Katayama, Kordel, Gerstl, Lane and Unsworth2002). Although flumioxazin is nonionic, previous research determined that flumioxazin sorption to soil increases with clay content (Ferrell and Vencill Reference Ferrell and Vencill2003; Ferrell et al. Reference Ferrell, Vencill, Xia and Grey2005). Flumioxazin sorption to soil with higher clay content might be caused by van der Waals forces (Bailey and White Reference Bailey and White1964) and may have contributed to the absence of flumioxazin-induced reductions in fruit yield at Las Cruces.
Previous studies determined that soil applications of flumioxazin can reduce photosynthetic capacity (Bigot et al. Reference Bigot, Fontaine, Clement and Vaillant-Gaveau2007), induce expression of genes associated with environmental stress (Castro et al. Reference Castro, Saladin, Bezier, Mazeyrat-Gourbeyre, Baillieul and Clement2008), cause crop injury (Burke et al. Reference Burke, Askew and Wilcut2002; Hutchinson et al. Reference Hutchinson, Boydston, Ransom, Tonks and Beutler2005), and decrease crop yield (Taylor-Lovell et al. Reference Taylor-Lovell, Wax and Nelson2001). Species-level differences in susceptibility to soil applications of flumioxazin reflect, in part, differential abilities to metabolize this herbicide (Price et al. Reference Price, Wilcut and Cranmer2004). Chile pepper sensitivity to flumioxazin in soil was previously demonstrated by Boyd (Reference Boyd2014), who determined that root uptake and subsequent injury from flumioxazin tank-mixed with paraquat was possible for pepper (cultivar ‘Aristotle’) on sandy, low organic soil following heavy rainfall in Florida. In New Mexico, flushes of water applied during furrow irrigation might mimic conditions of heavy rainfall that induced pepper injury in Florida.
Practical Implications
For chile pepper produced with furrow irrigation in New Mexico, recommendations for POST-directed flumioxazin will need to be adjusted by soil type. On fine-textured soil, two POST-directed applications of flumioxazin at 70 g ai ha–1 or one application at 107 g ai ha–1 may be appropriate because these treatments did not reduce fruit yield. However, on coarse-textured soil, significant reductions in fruit yield can occur if POST-directed flumioxazin at 107 g ai ha–1 is directed to surfaces of raised beds. Therefore, recommendations for POST-directed flumioxazin in chile pepper on coarse-textured soil should stipulate both confinement to row middles and application rates less than 107 g ai ha–1.
Flumioxazin at 70 g ai ha–1 might be suitable for chile pepper on coarse-textured soil because flumioxazin at this rate can provide preemergence control of broadleaf weeds that challenge chile pepper production in New Mexico (BJ Schutte, unpublished data). Subsequent studies should determine the likelihood of chile pepper injury from flumioxazin at 70 g ai ha–1 applied after crop emergence and directed to row middles. Such studies should be performed on coarse-textured soil that is low in organic matter content (<1% organic matter) because these soil conditions are potentially conducive to flumioxazin injury on chile pepper. Although potential for flumioxazin-induced crop injury may limit applications of this herbicide to the spaces between rows, this herbicide is anticipated to provide chile pepper farmers opportunities to reduce production expenses by reducing need for hand hoeing.
Acknowledgements
Funding sources for this project included the USDA Specialty Crops Block Grant (USDA-AMS-SCBGP-2014) and the New Mexico Chile Commission. Salaries and research support were provided by state and federal funds appropriated to the New Mexico Agricultural Experiment Station. Paprika seeds were generously supplied by Biad Chili Ltd. Company. No conflicts of interest have been declared. We gratefully acknowledge the field assistance of Joshua Bleiweiss, David Duran, Christopher Landau, Israel Marquez, Taylor Mesman, Edward Morris, and Joseph Wood. We also thank Dr. Jill Schroeder and Dr. Bill McCloskey for their helpful insights on this project.