Introduction
Scotch broom [Cytisus scoparius (L.) Link] is a large nonnative, leguminous shrub that threatens native plant communities by rapidly invading recently disturbed sites (Bossard and Rejmánek Reference Bossard and Rejmánek1994; Peterson and Prasad Reference Peterson and Prasad1998; Wearne and Morgan Reference Wearne and Morgan2004), competing vigorously for soil water and nutrients (Carter et al. Reference Carter, Slesak, Harrington, Peter and D’Amato2019; Watt et al. Reference Watt, Whitehead, Mason, Richardson and Kimberly2003b), and imparting soil legacy effects that inhibit native plant species (Grove et al. Reference Grove, Haubensak and Parker2012, Reference Grove, Parker and Haubensak2015). Native to western Europe, the species was introduced to Virginia in the early 1800s (Mack Reference Mack2003) and to California in the 1850s (Mobley Reference Mobley1954), and it is currently found in 10 western and 19 eastern states of the United States (EDDMapS 2020). The species also has been introduced to Argentina, Australia, Bolivia, Brazil, Canada, Chile, China, India, Iran, Japan, New Zealand, and South Africa (CABI 2020; Peterson and Prasad Reference Peterson and Prasad1998).
Although typically found in open sites (Peterson and Prasad Reference Peterson and Prasad1998), C. scoparius can establish and survive in conditions of low light availability (10% of full sun; Williams Reference Williams1981), such as a forest understory (Harrington Reference Harrington2007). In its native range, C. scoparius requires disturbance of associated vegetation in order to regenerate successfully, because newly germinated seedlings are relatively poor competitors (Paynter et al. Reference Paynter, Fowler, Memmott and Sheppard1998). In its introduced range, C. scoparius stands differ from those in its native range by having higher plant densities, multiple age classes, and less understory vegetation, all of which enable the stand to be self-replacing (Paynter et al. Reference Paynter, Downey and Sheppard2003).
Cytisus scoparius is a copious seed producer, with individual shrubs producing an average of 9,000 to 10,000 seeds yr−1 (Bossard and Rejmánek Reference Bossard and Rejmánek1994; Smith and Harlen Reference Smith and Harlen1991). Ballistic dispersal transfers most seeds up to 1.5 m from the parent plant (Smith and Harlen Reference Smith and Harlen1991). Seeds have an impervious coat that causes innate dormancy (Young and Young Reference Young and Young1992), but brief thermal shocks dramatically improve germination (Abdullah et al. Reference Abdullah, Jones and El-Beltagy1989; Bossard Reference Bossard1993; Rivas et al. Reference Rivas, Reyes and Casal2006; Smith and Harlen Reference Smith and Harlen1991) by melting or cracking the seed coat to make it permeable (Keeley Reference Keeley1987). Laboratory studies have identified that temperatures of 15 to 25 C are favorable for germination of imbibed C. scoparius seeds; however, germination declines strongly for temperatures outside this range (Bossard Reference Bossard1993; Harrington Reference Harrington2009). Experimentally varying the period of cold stratification from 0 to 60 d decreased the lag time for initiation of germination from 6 to 0 d but had no significant effect on cumulative 90-d germination (Harrington Reference Harrington2009).
Once buried, C. scoparius seeds are capable of germinating from depths ≤8 cm (Bossard Reference Bossard1993; Williams Reference Williams1981). Seeds buried in the soil can have delayed germination for at least 4 yr (Bossard Reference Bossard1993; Smith and Harlen Reference Smith and Harlen1991), enabling development of seedbanks of <100 to 21,000 seeds m−2 depending on stand maturity (Sheppard et al. Reference Sheppard, Hodge, Paynter and Rees2002; Smith and Harlen Reference Smith and Harlen1991). In the absence of seed rain, C. scoparius seedbanks are estimated to decline 40% to 50% each year from germination and seed loss (Paynter et al. Reference Paynter, Fowler, Memmott and Sheppard1998; Sheppard et al. Reference Sheppard, Hodge, Paynter and Rees2002).
As a leguminous species, C. scoparius can fix over 100 kg ha−1 yr−1 of N in its aboveground biomass and return 17 kg N ha−1 yr−1 to the soil as leaf litter (Watt et al. Reference Watt, Clinton, Whitehead, Richardson, Mason and Leckie2003a). Benefits of these nitrogen amendments to associated trees, such as radiata pine [Pinus radiata D. Don] in Australia, are minimal to nonexistent, because 3 yr of C. scoparius competition can cause a 96% reduction in tree biomass relative to trees grown in the absence of C. scoparius (Watt et al. Reference Watt, Whitehead, Mason, Richardson and Kimberly2003b). Cytisus scoparius can have multiple soil legacy effects, including accumulation of soil carbon and nitrogen and depletion of soil phosphorus and potassium (Caldwell Reference Caldwell2006; Haubensak and Parker Reference Haubensak and Parker2004; Slesak et al. Reference Slesak, Harrington and D’Amato2016a), which can favor nonnative grasses (Carter et al. Reference Carter, Slesak, Harrington, Peter and D’Amato2019; Grove et al. Reference Grove, Parker and Haubensak2015; Shaben and Myers Reference Shaben and Myers2010). Reduced biomass of coast Douglas-fir (Pseudotsuga menziesii (Mirb.) Franco var. menziesii) seedlings grown in C. scoparius–invaded soils was attributed to allelopathic effects (Grove et al. Reference Grove, Haubensak and Parker2012).
Controlling C. scoparius can be expensive and challenging. Direct and indirect annual costs for treatment expenses and lost timber revenues have been estimated to be $40 million for Oregon (Hulting et al. Reference Hulting, Neff, Coombs, Parker, Miller and Burrill2008) and $143 million for Washington (CAI 2017). Tactics for controlling or preventing invasions of C. scoparius in forest and rangelands include cutting, prescribed fire, herbicide treatments, and retention of logging debris after forest harvesting (Bossard and Rejmánek Reference Bossard and Rejmánek1994; DiTomaso et al. Reference DiTomaso, Brooks, Allen, Minnich, Rice and Kyser2006; Oneto et al. Reference Oneto, Kyser and DiTomaso2010; Peter and Harrington Reference Peter and Harrington2018).
Because C. scoparius has a persistent seedbank (i.e., a longevity of at least 5 yr, and likely much longer; Bossard Reference Bossard, Bossard, Randall and Hochovsky2000), PRE control is essential to managing forest sites already invaded by this species. At operational rates, metsulfuron and hexazinone herbicides provided 75% and 90% control of emerging C. scoparius seedlings, respectively; whereas, sulfometuron only reduced biomass of emerging seedlings but did not affect their survival (Ketchum and Rose Reference Ketchum and Rose2003). Sulfometuron and metsulfuron have been shown to limit root development of C. scoparius seedlings and thereby increase their susceptibility to soil drought (Harrington Reference Harrington2009). In PRE applications, maximum labeled rates of the synthetic auxin herbicides aminopyralid, aminocyclopyrachlor, and clopyralid killed an average of 85% of emerging C. scoparius seedlings; however, even rates as low as 25% of the maximum killed more than 60% of seedlings (Harrington Reference Harrington2014).
In western Washington USA, retaining logging debris after forest harvesting (i.e., stem-only harvesting; 2 to 2.5 kg m−2 of debris) strongly reduced density and cover of C. scoparius seedlings relative to conventional whole-tree harvesting (1 to 1.5 kg m−2 of debris) (Harrington and Schoenholtz Reference Harrington and Schoenholtz2010; Peter and Harrington Reference Peter and Harrington2018). Logging debris retention also improved survival and growth of planted seedlings of coast Douglas-fir (Harrington and Schoenholtz Reference Harrington and Schoenholtz2010; Harrington et al. Reference Harrington, Peter and Slesak2018), and it fostered the development of a native plant community (Peter and Harrington Reference Peter and Harrington2018).
This report describes a series of studies that were conducted to determine some of the mechanisms by which logging debris modifies the light environment to limit germination and growth of C. scoparius. Two components of the light environment relevant to plants include light quantity (i.e., photosynthetic photon flux density [PPFD]) and light quality (i.e., spectral distribution of PPFD by active wavelengths, such as red and far-red light). The author is not aware of any studies evaluating the effects of light quantity or quality on C. scoparius germination. An improved understanding of plant responses to light is needed if the light environment of cropping systems is to be manipulated successfully to improve weed management (Holt Reference Holt1995). Laboratory studies addressed the following three research questions. (1) Is light required for C. scoparius seed germination? (2) Do C. scoparius germination and growth differ when exposed to red versus far-red light? (3) Does C. scoparius germination vary with exposure to different red/far-red light (R/FR) ratios? A field study addressed a fourth study question: (4) How does variation in mass of simulated logging debris influence microclimate and germination and growth of C. scoparius?
Materials and Methods
Seed Collection, Storage, and Quality Control
In 2012, a bulk collection of seeds from multiple C. scoparius plants was conducted near Matlock, WA (47.215°N, 123.442°W) for the simulated logging debris effects study. In that study, seed mortality from biocontrol insects accounted for almost half of the test population, as evidenced by the collapsing, liquification, and rotting of seeds hollowed out by insect larvae. To understand the biological mechanisms driving C. scoparius seed germination responses to its light environment, an experimental approach was needed in subsequent studies to account for genetic variation in seed mortality from biocontrol insects, such as the use of experimental blocking by parent-plant seed source. Beginning in 2013, seeds were collected and bagged separately from individual parent plants located near Shelton, WA (47.302°N, 123.200°W) and Tumwater, WA (46.986°N, 122.891°W). Each year a different set of parent plants was identified for seed collection, and selected plants were spaced at least 3 m apart. As soon as they began to dehisce in mid-July, seed pods were collected and air-dried in paper bags to stimulate their opening. Extracted seeds were stored in sealed glass containers at −13 C. Seeds from the previous growing season were used in each study, and during counting they were visually inspected to eliminate those that were visibly damaged, unusually small, or immature (i.e., green). The actual number of parent-plant seed sources available for a given study (i.e., the number of experimental blocks) depended on variation in annual seed production and the estimated number of potentially viable seeds found after visual inspection.
Germination and Growth Responses to Light
Effects of Light and Temperature
To determine whether light is required for C. scoparius germination, in December 2018 two sets of 50 seeds from each of nine parent plants were placed on moistened paper trays in covered petri dishes that were subjected to a daily dark/light (14/10 h) temperature regime of 10/15 C. Fluorescent lighting in the germination chamber provided an average of 65 µmole m−2 s−1 of PPFD, as measured by a quantum sensor (LI-190SA quantum sensor, Li-Cor, 4647 Superior Street, Lincoln, NE 68504, USA). The R/FR of the fluorescent lighting averaged 4.0 based on readings from a LightScout red/far-red meter (Spectrum Technologies, 3600 Thayer Court, Aurora, IL 60504, USA). Green headlamps (Apollo Horticulture 17 Watt LED High Intensity Green Light Headlamp, https://www.apollohorticulture.com) were used while handling seeds to prevent light from influencing their subsequent responses to treatment. Edges of petri dishes were wrapped in parafilm to prevent moisture loss (Parafilm M, Heathrow Scientific, 620 Lakeview Parkway, Vernon Hills, IL 60061, USA). In one set, petri dishes were also wrapped in aluminum foil to eliminate incoming light. A germinant was defined as any seed visibly showing the white hypocotyl of a C. scoparius embryo. After 20 d, germination percentages were calculated for each petri dish using the following equation:

where G is cumulative germination (%), L is the number of living germinants, and R is the number of remaining intact seeds presumed to be alive. The equation provides a more accurate estimate of seed germination responses to experimental treatments, because it includes only those seeds having the biological potential to germinate. Seed mortality percentages were calculated for each petri dish using the following equation:

where M is cumulative seed mortality (%), D is the number of dead seeds (i.e., those found to be rotten, liquified, or empty), and all other variables are defined as for Equation 1. The experiment was repeated for daily dark/light temperature regimes of 15/20 C and 20/25 C in February and March 2019, respectively.
Effects of Red versus Far-Red Light
To determine whether C. scoparius germination differs when exposed to red versus far-red light, two germination chambers were outfitted with either four red (660-nm wavelength) or four far-red LED bulbs (730-nm wavelength) (powerPAR 15 watt LED grow light, Hydrofarm, 2249 S. McDowell Ext., Petaluma, CA 94954, USA). Measurements of total irradiance taken with a Jaz® Combo spectrometer (Ocean Optics, 830 Douglas Avenue, Dunedin, FL 34698, USA) indicated that energy outputs differed about 22% between the red (1,227 W m−2) and far-red (1,007 W m−2) germination chambers. Each germination chamber was programmed for a daily dark/light (14/10 h) temperature regime of 15/20 C. In October 2017, two sets of 50 seeds from each of 12 parent plants were placed on moistened paper trays in covered petri dishes; one set was placed in the red germination chamber and the other set was placed in the far-red chamber. To confirm the experimental validity of the red and far-red light treatments, three additional petri dishes were included in each germination chamber that contained seeds of Grand Rapids leaf lettuce (Lactuca sativa L.)—a variety known to exhibit inhibition of germination in response to far-red light (Borthwick et al. Reference Borthwick, Hendricks, Parker, Toole and Toole1952). Over a 20-d period, germinants were counted and removed every 1 to 2 d. Immediately before each count, the petri dishes were rotated to remove potential location effects, and paper trays were remoistened with 1 to 2 ml of distilled water. Dead seeds (as evidenced by blackening or liquification) also were counted and removed from each petri dish. Cumulative germination and seed mortality percentages were calculated per petri dish with Equations 1 and 2, respectively. The study was repeated in May and June 2019 with seeds from eight and six parent plants, respectively (i.e., in these later trials, collections from some parent plants did not have adequate numbers of potentially viable seeds to be included in the experiment). Upon completion of the May and June 2019 studies, remaining Lactuca sativa seeds in the far-red germination chamber were moved to the red chamber to confirm the reversibility of germination inhibition (Borthwick et al. Reference Borthwick, Hendricks, Parker, Toole and Toole1952).
To compare growth of C. scoparius seedlings when exposed to red versus far-red light, in August 2017, a total of 20 plastic boxes of dimensions 17 cm by 12 cm by 6 cm with drain holes were filled with potting soil (Sunshine® Mix #1, Sun Gro Horticulture, 770 Silver Street, Agawam, MA 01001, USA). Potting soil was pre-wetted to ensure uniformity of moisture within each box. Each box was labeled to hold seeds from two parent plants, one in each half of the box. Two sets of 50 seeds each were counted out for each of the 10 parent plants. Working within the lighting of the germination chambers only, seed sets were placed on the soil surface of the appropriately labeled end of each box. Seeds were pressed into the soil to ensure good contact, but they were not fully buried. Every 2 d, the soil boxes were rotated to remove potential location effects, and approximately 10 ml of distilled water was added to each box to maintain moisture content at a moderate level. After 40 d, seedlings within each soil box were counted and measured for height (nearest 0.1 cm). Seedlings were then carefully excavated, their roots washed, and their roots and shoots composited into separate paper envelopes. Root and shoot biomass values (nearest mg) were determined after drying at 65 C to a constant weight. The study was repeated in October 2017 with seeds from nine parent plants.
Effects of Red/Far-Red Ratio
To determine whether C. scoparius germination differs when exposed to various ratios of red/far-red light, a total of six trials were conducted from July to October 2019 within a germination chamber outfitted with one of six combinations of red and far-red LED light bulbs: 0/4, 1/4, 2/3, 3/2, 4/1, or 5/0, respectively. R/FR was measured for each lighting combination with a LightScout red/far-red meter, resulting in the following values: <0.01, 0.4, 0.9, 1.6, 3.6, and 31.1. For each trial, 50 seeds from each of 15 parent plants were placed in moistened petri dishes and subjected to a daily dark/light (14/10 h) temperature regime of 15/20 C for 20 d. Every 1 to 2 d, 1 to 2 ml of distilled water was added to each petri dish, and germinated seeds and dead seeds were counted separately and removed. One additional petri dish was included in each trial that contained 50 seeds of Lactuca sativa to confirm the experimental validity of the R/FR treatments. Cumulative germination and seed mortality percentages were calculated per petri dish with Equations 1 and 2, respectively.
Simulated Logging Debris Effects on Microclimate, Germination, and Growth
To simulate the effects of various masses of logging debris on microclimate and germination and growth of C. scoparius, 15 frames were constructed of 1.9-cm-diameter PVC and 1.3-cm mesh hardware cloth in summer 2012. Three tables (i.e., experimental blocks) were placed parallel to each other in a nearby open area outside the Forestry Sciences Laboratory, Olympia, WA (46.952°N, 122.959°W), and five frames were attached to each table. Each frame had a 60 cm by 60 cm screen platform for suspending logging debris 20 cm above the table surface. The tabletops and outside vertical surfaces of each frame were wrapped in black plastic to limit light to only that entering through the screen platform. In August 2012, fresh branches of coast Douglas-fir ≤1.3 cm in diameter (i.e., simulated logging debris) were collected from nearby trees. Five evenly spaced masses of logging debris were selected to encompass the range of 0 to 2.78 kg m−2 and to include levels designated previously as typical of light (1 kg m−2) and heavy (2 kg m−2) retention after forest harvesting (Harrington et al. Reference Harrington, Peter and Slesak2018). One mass of logging debris was randomly assigned to each frame on a given table. R/FR was measured immediately after application of the fresh green debris via a Jaz® Combo spectrometer. Measurement of R/FR was repeated in October 2012 after desiccation and browning of the debris. Both measurements of R/FR were taken on cloudless days near solar noon.
In late April 2013, a 90-d experiment was initiated with application of fresh samples of logging debris to each frame according to the 2012 treatment assignments. Air temperature was recorded every 2 h via an iButton sensor (model DS1921G, Maxim Integrated, 160 Rio Robles, San Jose, CA 95134, USA) suspended within a small plastic bag approximately 10 cm below the screen platform near the center of each debris frame. PPFD was measured within each frame with an AccuPAR LP-80 ceptometer (Meter Group, 2365 NE Hopkins Court, Pullman, WA 99163, USA). Measurements of PPFD were taken on cloudless days near solar noon when the debris was green (May) and again after the debris had desiccated and turned brown (July). Two readings, taken diagonally under the suspended logging debris of each frame, were averaged and then expressed as a percentage of an open-sky reading taken facing south.
To quantify the relationship of C. scoparius germination to logging debris mass, approximately 150 seeds (i.e., 1.5 g) from a bulk collection (i.e., not separated by individual parent plants) were placed on a moistened paper tray in each of 15 covered petri dishes; each dish was enclosed within a sealed plastic bag and placed under the suspended logging debris of a given frame. Every 1 to 2 d, the paper tray within each petri dish was remoistened with 1 to 2 ml of distilled water, and germinated seeds and dead seeds were counted separately and removed. On day 90, cumulative germination and seed mortality percentages were calculated per petri dish with Equations 1 and 2, respectively.
To evaluate the relationship of C. scoparius seedling biomass to logging debris mass, approximately 150 seeds (i.e., 1.5 g) from a bulk collection (i.e., not separated by individual parent plants) were sown into each of 30 plastic soil boxes using the methods described earlier. Soil for 15 boxes came from a site near Matlock, WA (47.206°N, 123.442°W)—a very gravelly loamy sand of the Grove series (sandy-skeletal, mixed, mesic Dystric Xerorthents) formed in glacial outwash (USDA-NRCS 2019). Soil for the remaining 15 boxes came from a site near Molalla, OR (45.196°N, 122.285°W)—a cobbly loam of the Kinney series (fine-loamy, isotic, mesic Andic Humudepts) (USDA-NRCS 2019). Before seeds were sown, each soil was sieved to remove coarse fragments greater than 2 mm, each box was filled with 1 kg of soil, and distilled water was added to achieve field capacity. One Matlock soil box and one Molalla soil box were placed under the suspended logging debris of each frame. At weekly intervals, distilled water was added to each soil box to restore field capacity. Soil boxes from one experimental block were weighed immediately before watering to estimate evaporative water loss. On day 90, height (nearest 0.1 cm) of the five tallest seedlings per soil box were measured. Seedlings within each soil box were counted and carefully excavated, their roots were washed, and shoots and roots were composited into separate paper envelopes. Root and shoot biomass values (nearest mg) were determined after drying at 65 C to a constant weight.
Statistical Analyses
All statistical analyses were conducted in SAS (SAS System for Windows v. 9.4, SAS Institute, Cary, NC 27513, USA) with a significance level of α = 0.05. Most experiments were repeated one or two times, and statistical analyses were conducted separately for each trial. The experimental design of all laboratory experiments was a randomized complete block with blocks assigned to parent-plant seed source to control for genetic variation in seed mortality from biocontrol insects; experimental units were individual petri dishes or soil box halves. An angular transformation was applied to proportionate germination values, and a logarithmic transformation was applied to seedling height and biomass values to homogenize the residual variation before ANOVA (Sokal and Rohlf Reference Sokal and Rohlf1981). Residuals from ANOVA and regression were plotted against predicted values to confirm the homogeneity of the residual variances. All reported values of the coefficient of determination were adjusted for degrees of freedom (i.e., adjusted R2).
Effects of Light and Temperature
Final cumulative germination data were subjected to ANOVA in SAS PROC MIXED to test the significance of the fixed factors, light (presence vs. absence), temperature regime, and their interaction, after adjusting for random effects of parent plant (i.e., blocks). Orthogonal polynomial contrasts were included in the ANOVA to test for linear and quadratic effects of temperature regime. A similar analysis was conducted for the final cumulative seed mortality data.
Effects of Red versus Far-Red Light
Incremental values of cumulative germination of C. scoparius or Lactuca sativa were subjected to repeated-measures ANOVA in SAS PROC MIXED to test the significance of the fixed factor, light treatment (i.e., red vs. far-red light), day of trial, and their interaction, after adjusting for random effects of parent plant. If a treatment-by-day interaction was detected, slicing was used in SAS to identify individual days in which differences existed between treatments. A similar analysis was conducted for the cumulative seed mortality data. Mean values of seedling height and root and shoot biomass for each trial were subjected to ANOVA in SAS PROC MIXED to test the significance of the light treatment after adjusting for random effects of parent plant.
Effects of Red/Far-Red Ratio
Final cumulative germination data for C. scoparius were subjected to ANOVA in SAS PROC MIXED to test the significance of the fixed factor, R/FR (logarithmically transformed to provide approximately equal spacing), after adjusting for random effects of parent plant. Orthogonal polynomial contrasts were included in the ANOVA to test for linear and quadratic effects of R/FR. Polynomial regression was used to characterize the germination responses of Lactuca sativa to R/FR. A similar analysis was conducted for the final cumulative seed mortality data.
Simulated Logging Debris Effects on Microclimate, Germination, and Growth
Values of mean daily air temperature by month, PPFD, R/FR, cumulative germination, cumulative seed mortality, seedling height, and root and shoot biomass were subjected to ANOVA in SAS PROC MIXED to test the significance of the fixed factor, debris mass, after adjusting for random effects of blocks. Orthogonal polynomial contrasts were included in the ANOVA to test for linear, quadratic, and cubic effects of debris mass. Results of the contrasts were used to fit appropriate polynomial or nonlinear models via SAS PROC REG or PROC NLIN, respectively, to illustrate each variable’s response to debris mass. A negative exponential model was fit to the relationship of soil box weight loss versus logging debris mass. The coefficient of determination for nonlinear models was estimated from the linear regression of observed versus predicted values. For air temperature, analysis and reporting were focused on data from May, because the range of mean daily values for that month (12.8 to 14.3 C) was biologically relevant to potential temperature constraints on seed germination (Bossard Reference Bossard1993; Harrington Reference Harrington2009). For both PPFD and R/FR, models with separate regression coefficients for green (i.e., fresh) and brown (i.e., desiccated) debris were fit to the pooled data, and the extra sums-of-squares approach was used to select the simplest model for each variable (Neter et al. Reference Neter, Wasserman and Kutner1989). For soil box weight loss, seedling height, and root and shoot biomass, differences due to soil type were tested similarly using the extra sums-of-squares approach.
Predicting Temperature Limitations to Germination
To illustrate potential temperature limitations to C. scoparius germination as a result of logging debris retention, germination in the controls (i.e., absent thermal shock treatments) for temperatures less than 25 C were interpolated from Figures 2 and 3 of Bossard (Reference Bossard1993) for a 24-d period to match the experimental duration used by Harrington (Reference Harrington2009). Germination data for the same temperature range from Figure 1 of Harrington (Reference Harrington2009) were adjusted to remove effects of a 45-d cold-stratification period by multiplying observed germination by 0.63 based on the average values shown in Figure 2 of Harrington (Reference Harrington2009) for day 24. Differences in daily photoperiods between data sources (Bossard [Reference Bossard1993]: 15-h light/9-h dark; Harrington [Reference Harrington2009]: 10-h light/14-h dark) were assumed to be inconsequential. Temperatures for each regime in Bossard (Reference Bossard1993) were held constant, but the light and dark temperatures for each regime in Harrington (Reference Harrington2009) differed by 5 C. Linear regression was used to characterize the relationship of C. scoparius germination to temperature for the combined data from Bossard (Reference Bossard1993) and Harrington (Reference Harrington2009). Differences in the relationship due to data source were tested via SAS PROC REG using the extra sums-of-squares approach (Neter et al. Reference Neter, Wasserman and Kutner1989). Using the upper (i.e., lighted) temperature value for each regime from Harrington (Reference Harrington2009) in the regression resulted in a relationship that closely matched that found for the Bossard (Reference Bossard1993) data.
Results and Discussion
Germination and Growth Responses to Light
Effects of Light and Temperature
Cumulative germination of C. scoparius did not differ significantly among levels of light (P = 0.40), temperature (P = 0.15), or their interaction (P = 0.22) (Figure 1). Cumulative seed mortality from biocontrol insects averaged 31% and did not differ significantly among levels of light (P = 0.08), temperature (P = 0.90), or their interaction (P = 0.91). However, stem lengths of seedlings germinating in darkness were more than three times those of seedlings germinating in light. Reduced germination in the lighted 20/25 C regime was associated with partial drying in some petri dishes, but it did not change overall results of the study. This study confirms that C. scoparius is not photoblastic (i.e., germination is neither negatively nor positively responsive to light).

Figure 1. Cytisus scoparius germination (±SE) as affected by light and temperature in a laboratory study in Olympia, WA, during December 2018 to March 2019.
Effects of Red versus Far-Red Light
During the first 5 d of each trial, cumulative germination of C. scoparius was greater under far-red light than under red light – a difference that was statistically significant in the first two trials (P ≤ 0.039) (Figure 2). This brief stimulation of germination by far-red light could confer a slight, short-term advantage for C. scoparius establishment under green logging debris or plant canopies. Later in each trial, C. scoparius germination rates were greater under red light. At the end of each trial, cumulative germination of C. scoparius under red light averaged 5% to 7% greater than under far-red light, although differences were not statistically significant (P ≥ 0.29). Cumulative seed mortality from biocontrol insects averaged 38% for the three trials and did not differ significantly between red and far-red light (P ≥ 0.493). Cumulative germination of Lactuca sativa under far-red light was 32% to 38% of that observed under red light; however, moving the ungerminated seeds into the red-light germination chamber resulted in 100% germination (Figure 2). These results indicate that C. scoparius germination is not subject to phytochrome regulation, whereas Lactuca sativa germination is clearly phytochrome regulated. Gorse (Ulex europaeus L.), an invasive leguminous shrub with life history traits similar to those of C. scoparius, also has seed germination that is not photoblastic (Ivens Reference Ivens1983) or subject to phytochrome regulation (JA Zabkiewicz, personal communication, cited in Rolston and Talbot Reference Rolston and Talbot1980).
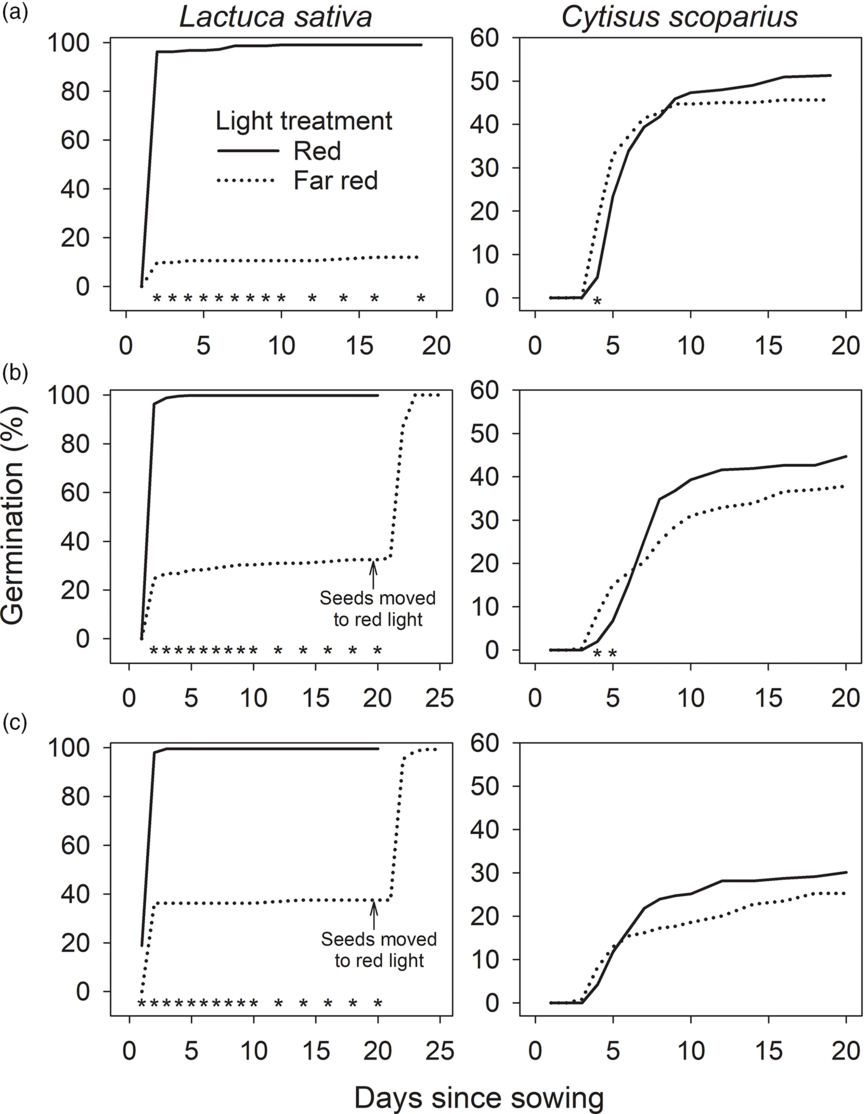
Figure 2. Germination of Lactuca sativa (Grand Rapids variety) (left column) and Cytisus scoparius (right column) when exposed to red versus far-red light in: (A) October 2017, (B) May 2019, and (C) June 2019 in laboratory studies in Olympia, WA. For each species, asterisks indicate dates in which cumulative germination differed significantly (P ≤ 0.05) between red and far-red light.
In the two experimental trials of the biomass study, seedling numbers ranged from 0 to 7 per soil box. Height of C. scoparius seedlings from each of the trials did not differ significantly between red and far-red light (P ≥ 0.110; Table 1). Root biomass of C. scoparius seedlings grown in red light averaged 2.4 and 6.8 times greater in Trials 1 and 2, respectively, than that of seedlings grown in far-red light (P ≤ 0.046; Table 1). Shoot biomass also was greater in red light than in far-red light, but differences were significant only in Trial 2 (P = 0.017). In a meta-analysis of biomass responses of terrestrial plants to environmental stress, allocation to stem increased but allocation to leaves decreased as R/FR decreased below 0.9, but there was no clear response for roots (Poorter et al. Reference Poorter, Niklas, Reich, Oleksyn, Poot and Mommer2012). In the current study, perhaps reduced biomass allocation to roots in the far-red chamber can be attributed to lower radiant energy (1,007 W m−2) than in the red chamber (1,227 W m−2).
Table 1. Average height and root and shoot biomass of Cytisus scoparius seedlings (SE in parentheses) when exposed to red versus far-red light in laboratory studies in Olympia, WA, during August (Trial 1) and October (Trial 2) 2017.a

a Seedlings were grown for 40 d in a standard potting soil.
b df for the numerator (Num) and denominator (Den) of the F-test from ANOVA.
Effects of Red/Far-Red Ratio
Cumulative germination of C. scoparius did not vary significantly among R/FR values (P = 0.397), averaging 62% (Figure 3). Linear and quadratic effects of R/FR also were nonsignificant for C. scoparius (P ≥ 0.08). Cumulative seed mortality from biocontrol insects averaged 67% and did not differ significantly among values of R/FR (P = 0.653). Cumulative germination of Lactuca sativa had a quadratic relationship with log10(R/FR) (R2 = 0.99) because of the contrasting response between R/FR = 0.01 (32% germination) and R/FR ≥ 0.38 (88% to 100% germination) (Figure 3).

Figure 3. Germination of Lactuca sativa (Grand Rapids variety) and Cytisus scoparius (±SE) when exposed to various red/far-red light ratios (R/FR) in a laboratory study in Olympia, WA, during July to October 2019. Trend lines are from polynomial regression (Lactuca sativa: Y = 97.7 + 9.67(X) − 10.7(X 2), where X = log10(R/FR), P < 0.001, R2 = 0.99, n = 6; C. scoparius: Y = 62.2, P = 0.920, n = 90).
Simulated Logging Debris Effects on Microclimate, Germination, and Growth
Mean daily air temperature in May varied significantly among debris masses (P = 0.001). Linear, quadratic, and cubic effects of debris mass all were significant (P ≤ 0.02). A cubic regression model provided the best fit to the relationship of air temperature to debris mass (R2 = 0.68; Table 2). As simulated debris mass increased from 1 kg m−2 (“light debris”) to 2 kg m−2 (“heavy debris”), predicted air temperature in May decreased 0.5 C from 13.6 to 13.1 C (Figure 4A). Harrington et al. (Reference Harrington, Peter and Slesak2018) observed reductions in soil temperature at 5-cm depth of 0.5 to 1.5 C for heavy versus light debris during late spring and summer.
Table 2. Regression models characterizing the observed relationships of air temperature in May, weekly soil box weight loss from evaporation, photosynthetic photon flux density (PPFD), red/far-red ratio (R/FR), and Cytisus scoparius seedling germination, height, root biomass, and shoot biomass versus mass of simulated logging debris (X; kg m−2) in a field study in Olympia, WA, during April to July 2013 (relationships depicted graphically in Figures 4 and 5).

a P = significance of the regression; s y·x = standard error of estimate; R2 = coefficient of determination adjusted for df; and n = sample size.
b Green indicator variable: G = 1 for green logging debris; and G = 0 for brown logging debris. Soil indicator variable: S = 1 for glacial outwash (Matlock, WA); and S = 0 for cobbly loam (Molalla, OR).

Figure 4. Regression relationships of mean values (±SE) of: (A) air temperature in May, (B) weekly soil box weight loss from evaporation, (C) photosynthetic photon flux density (PPFD), and (D) red/far-red light ratio versus varying mass of simulated logging debris in a field study in Olympia, WA, during April to July 2013. Trend lines are from the regression models listed in Table 2.
Although weekly soil box weight loss from evaporation exhibited high levels of variation, it had a significant negative exponential relationship with logging debris mass that did not differ between soil sources (R2 = 0.15; Table 2). As simulated debris mass increased from 1 to 2 kg m−2, predicted soil box weight loss decreased from 14% to 10% (Figure 4B). Decreases in soil water loss due to mulching effects of logging debris have been observed in previous research (Harrington et al. Reference Harrington, Peter and Slesak2018; O’Connell et al. Reference O’Connell, Grove, Mendham and Rance2004; Roberts et al. Reference Roberts, Harrington and Terry2005), and generally they have been attributed to reductions in soil temperature and evaporation (Devine and Harrington Reference Devine and Harrington2007).
PPFD differed significantly among debris masses for both green and brown debris (P < 0.001). For both types of debris, linear and quadratic effects of debris mass were significant (P ≤ 0.003); however, a negative exponential regression model provided the best fit for these data (R2 = 0.99; Table 2). Attenuation of PPFD occurred more rapidly with increasing debris mass for green debris than for brown debris (Figure 4C). For example, predicted PPFD values for light and heavy debris are 11% and 2% of full sun for green debris, whereas they are 30% and 12% for brown debris, respectively. Greater attenuation of PPFD under fresh debris can be attributed both to its green color, which is effective at absorbing blue and red light, and its greater surface area.
R/FR differed significantly among debris masses for both green and brown debris (P ≤ 0.002). Linear effects of debris mass were significant for both types of debris (P < 0.001); however, as found for PPFD, a negative exponential regression model provided the best fit for these data (R2 = 0.87; Table 2). R/FR declined rapidly with increasing debris mass for green debris, whereas it declined slowly for brown debris (Figure 4D). For example, predicted R/FR values for light and heavy debris are 0.5 and 0.1 for green debris, whereas they are 1.2 and 1.1 for brown debris, respectively. These model predictions suggest that only green debris can alter R/FR sufficiently to elicit a biological response in a neighboring plant; brown debris transmits enough red light to cancel potential biological effects of far-red light. Bliss and Smith (Reference Bliss and Smith1985) found that fresh litter of English oak (Quercus robur L.) attenuated red light more than far-red light, because the overlapping, pigmented material acted as a filter to screen out specific wavelengths, similar to that found for green Douglas-fir logging debris in the current study. In contrast, fresh litter of Corsican pine (Pinus nigra Arnold var. maritima) had a neutral effect on spectral transmission, similar to that found for brown Douglas-fir logging debris, because ambient light penetrated the loosely packed material and the pine needles themselves transmitted very little light because of their thickness (1 mm) (Bliss and Smith Reference Bliss and Smith1985).
Cumulative germination of C. scoparius did not differ significantly among debris masses (P = 0.199). Linear, quadratic, and cubic effects of debris mass also were not significant (P > 0.260). Mean germination for the 90-d trial was 32% (Table 2; Figure 5A). Although C. scoparius germination was variable in this study, absence of responses to varying debris mass provides additional support for the species’ non-photoblastic response. Cumulative seed mortality from biocontrol insects averaged 48% and did not differ significantly among debris masses (P ≥ 0.818).

Figure 5. Regression relationships of mean values (±SE) of Cytisus scoparius: (A) germination, (B) height, (C) root biomass, and (D) shoot biomass versus varying mass of simulated logging debris in a field study in Olympia, WA, during April to July 2013. Seedlings were grown for 90 d in soils collected near Matlock, WA, and Molalla, OR, USA. Trend lines are from the regression models listed in Table 2.
Height of C. scoparius seedlings differed significantly among debris masses (P < 0.001) and soil sources (P = 0.002), but it did not differ significantly with the interaction of debris mass and soil source (P = 0.242). Only quadratic effects of debris mass were significant for height (P = 0.001). A quadratic regression model provided the best fit to the height responses (Table 2). Based on the regression model, C. scoparius height peaked in each soil type at a debris mass of 1.64 kg m−2 (Figure 5B); R/FR under green debris of this mass is predicted to be 0.2—a value that has been definitively shown to stimulate the classic shade-avoidance response of increased stem elongation (Poorter et al. Reference Poorter, Niklas, Reich, Oleksyn, Poot and Mommer2012; Smith Reference Smith1982). Reduced height of C. scoparius seedlings grown under the highest debris mass (2.78 kg m−2) is likely indicative of severe limitations in seedling productivity from restricted light availability (i.e., predicted PPFD < 6% of full sun; see following discussion of biomass responses). Seedling height averaged 2 cm greater when grown on soil from Molalla versus that from Matlock, probably because of the superior quality of Molalla soil (Slesak et al. Reference Slesak, Harrington, Peter, DeBruler, Schoenholtz and Strahm2016b).
Root and shoot biomass of C. scoparius seedlings both differed significantly among debris masses (P ≤ 0.001), but they did not differ between soil sources (P ≥ 0.08) or with the interaction of debris mass and soil source (P ≥ 0.16). Linear effects of debris mass were significant for root biomass (P < 0.001), while both linear and quadratic effects were significant for shoot biomass (P ≤ 0.006). Cubic and quadratic regression models provided the best fits for root and shoot biomass, respectively (Table 2). Predicted root biomass decreased 84% as simulated debris mass increased from 1 to 2 kg m−2 (i.e., from light to heavy debris levels), while predicted shoot biomass decreased only 29% (Figures 5C and D). Because both PPFD and R/FR declined with increasing mass of logging debris, it is not possible to determine which light factor, quantity or quality, caused the reductions in root and shoot biomass of C. scoparius. The meta-analysis by Poorter et al. (Reference Poorter, Niklas, Reich, Oleksyn, Poot and Mommer2012) supported a general finding for terrestrial plants of decreasing allocation to root biomass and increasing allocation to leaves with decreasing light quantity. However, Poorter et al. (Reference Poorter, Niklas, Reich, Oleksyn, Poot and Mommer2012) also found increased allocation to stem as R/FR decreased below 0.9. Because aboveground biomass of C. scoparius was not partitioned into stems and leaves in the current study, it is not possible to determine which light factor, quantity or quality, is most responsible for reduced shoot biomass under high levels of logging debris.
Predicting Temperature Limitations to Germination
The observed 0.5 C reduction in air temperature for heavy versus light debris (Figure 4A) is predicted to reduce C. scoparius germination by less than 1% (Figure 6). If we assume a 1.5 C reduction in temperature for heavy versus light debris, as observed for soil temperature by Harrington et al. (Reference Harrington, Peter and Slesak2018), predicted C. scoparius germination would be reduced by 2.4%. These predictions of potential limitations to germination suggest that temperature is not the primary factor limiting regeneration of C. scoparius under heavy debris.

Figure 6. Regression relationship of mean germination of Cytisus scoparius versus air temperature, based on the laboratory experiments of Bossard (Reference Bossard1993) and Harrington (Reference Harrington2009). Regression statistics include sample size (n), standard error of estimate (s y·x), and coefficient of determination (R2).
In its native environment, C. scoparius does not successfully regenerate without disturbance to the dominant vegetation or soil (Paynter et al. Reference Paynter, Fowler, Memmott and Sheppard1998). In a nonnative environment (i.e., California, USA), density of C. scoparius germinants declined exponentially during three growing seasons from about 770 to 32 seedlings m−2, although the causes of mortality were not identified (Bossard and Rejmánek Reference Bossard and Rejmánek1994). In harvested, thinned, and intact forests of coastal Douglas-fir in western Washington, USA, less than 2% of seeds produced seedlings of C. scoparius 2 yr after sowing (Harrington Reference Harrington2007). Paynter et al. (Reference Paynter, Fowler, Memmott and Sheppard1998) suggested that mortality of new seedlings under mature C. scoparius in France was attributable to a “rain” of invertebrate herbivores and pathogens; whereas in open areas, mortality was attributable to competition for light and soil water because new seedlings are relatively poor competitors.
This research has demonstrated that C. scoparius seed germination is not responsive to variation in either light quantity or quality. Because of the limited geographic scope of the seed sources used in the studies (i.e., near Matlock, Shelton, and Tumwater, WA), reasonable caution should be used in interpreting these results; seeds from other locations could show variation in their germination and growth responses to the light environment and microclimate. In addition, the simulated logging debris effects study did not assess the effects of physical contact of the debris with the germination substrate or with germinating seedlings, nor did it consider possible debris effects on soil nutrients. Nonetheless, the weak, and potentially exploitable, link in regeneration of C. scoparius appears to be not seed germination, but seedling biomass development.
Based on findings from the laboratory and field studies, reduced biomass of seedlings, especially roots, due to shade of logging debris and subsequently developing native vine and shrub cover (Harrington et al. Reference Harrington, Slesak, Dollins, Schoenholtz and Peter2020) creates a significant vulnerability for juvenile C. scoparius that makes them susceptible to mortality from summer drought, competition, herbivory, and pathogens. A similar vulnerability was hypothesized for C. scoparius seedlings that had reduced root biomass after PRE application of sulfometuron herbicide (Ketchum and Rose Reference Ketchum and Rose2003). In a 90-d laboratory experiment, Harrington (Reference Harrington2009) confirmed the validity of the hypothesis of Ketchum and Rose (Reference Ketchum and Rose2003) by observing a 17% increase in mortality of C. scoparius seedlings grown in sulfometuron-treated soil when watering rate was reduced by half midway through the study; nontreated seedlings under the same watering regime had only a 3% to 6% increase in mortality.
Green logging debris was more effective than brown debris at modifying the light environment sufficiently to cause significant reductions in C. scoparius root biomass, particularly by reducing R/FR below 1. Forest harvesting in winter or early spring allows green debris to be in place when C. scoparius germination begins. Retention of a high level of logging debris after forest harvesting (≥2 kg m−2) has potential application on sites likely to be invaded by C. scoparius or those already having the species present in the seedbank.
Acknowledgments
Financial support for this research was provided by USDA National Institute for Food and Agriculture (Grants.gov number: GRANT11325729) and USDA Forest Service, Pacific Northwest Research Station. Thanks to James Dollins and Jessyka Williams, PNW Research Station, for technical assistance with the research. No conflicts of interest have been declared.