Introduction
Pesticides can raise productivity by reducing damage to crops, but at the same time pose a potentially negative effect on human health and an adverse effect on the environment (Oenema et al. Reference Oenema, Witzke, Klimont, Lesschen and Velthof2009; Ruegg et al. Reference Ruegg, Quadranti and Zoschke2007). Despite farmers’ interest in reducing herbicide applications, the use of herbicides is becoming more widespread globally (Peterson et al. Reference Peterson, Collavo, Ovejero, Shivrain and Walsh2018; Sarker and Muehlbauer Reference Sarker, Muehlbauer and Shoba2018). As a result, employing sustainable agronomic practices is necessary to limit environmental risks while still maximizing crop production (Cole et al. Reference Cole, Zandvakili, Xing, Hashemi, Barker and Herbert2019a; Jahanzad et al. Reference Jahanzad, Sadeghpour, Hosseini, Barker, Hashemi and Zandvakili2014). Weeds are a serious threat to corn (Zea mays L.) production in Iran (Nosratti et al. Reference Nosratti, Sabeti, Chaghamirzaee and Heidari2017). The use of mechanical weed control practices such as soil tillage and hand weeding are decreasing, while chemical control practices are on the rise in the Iranian corn production system (Nosratti et al. Reference Nosratti, Sabeti, Chaghamirzaee and Heidari2017). Nicosulfuron, a sulfonylurea herbicide, is widely used for POST weed control in corn in Iran. This herbicide is regularly and intensively used, because PRE herbicides are not particularly efficacious under no-tillage systems. This practice is not only costly but can result in environmental contamination and the evolution of herbicide-resistant weeds (Walsh and Powles Reference Walsh and Powles2007; Nosratti et al. Reference Nosratti, Sabeti, Chaghamirzaee and Heidari2017). Therefore, it is important to determine whether reduced rates of nicosulfuron will provide efficacious weed control in corn while reducing any adverse effects on the environment. Incorporating cover crops into the soil as green manures (GM) can help control weeds in a subsequent crop by releasing allelochemicals into the soil (Masilionyte et al. Reference Masilionyte, Maiksteniene, Kriauciuniene, Jablonskyte-Rasce, Zou and Sarauskis2017). Secondary metabolites such as 1,4-benzoxazine-3-ones that are released from cereal rye (Secale cereale L.) cover crop after termination and incorporation into the soil are expected to inhibit the germination of weed seedlings and suppress their growth (Cornelius and Bradley Reference Cornelius and Bradley2017).
Growing cover crops during the fall and winter before sowing corn is not a common practice in Iran (Sabet et al. Reference Sabet, Hosseini and Mashhadi2016). Little attention has been given to the use of GM in semiarid climatic conditions, because farmers in these regions face several challenges related to using GM, including selection, establishment, growth, weed control, and the availability of water for the GM crop (Sabet et al. Reference Sabet, Hosseini and Mashhadi2016). A common crop rotation in Iran is wheat (Triticum aestivum L.)–fallow–corn. The fallow period, which is during fall and winter, may lead to nutrient loss and pollution such as nitrates leaching from the application of nitrogen (N) fertilizer in a previous crop. Excessive use of fertilizer N and the inability to precisely estimate N mineralization from previous crop residues and organic matter results in surplus N that can be lost to the environment after cash crop harvest (Thapa et al. Reference Thapa, Mirsky and Tully2018). Moreover, the elevated risk of offsite losses from herbicides was recorded in runoff that occurred months after herbicide application (Nachimuthu et al. Reference Nachimuthu, Halpin and Bell2016). In addition, weeds can germinate without competition in fallow land. There has been an interest in the utilization of a mixed stand of GM as exhibited by Finney et al. (Reference Finney, White and Kaye2016), who revealed that an increase in the diversity of cover crops in agroecosystems results in enhanced ecosystem services (Picard et al. Reference Picard, Ghiloufi, Saulas and de Tourdonnet2010; Zandvakili et al. Reference Zandvakili, Ebrahimi, Hashemi, Barker and Akbari2017). The main advantages of GMs include nutrient capturing and recycling (Hooker et al. Reference Hooker, Coxon, Hackett, Kirwan, O’Keeffe and Richerds2008), suppressing weeds and volunteer crops, and enhancing yields (Blanco-Canqui et al. Reference Blanco-Canqui, Claassen and Presley2012). However, nutrient dynamics (Ruffo and Bollero Reference Ruffo and Bollero2003) and weed control (Kruidhof et al. Reference Kruidhof, Bastiaans and Kropff2009) depend on GM quality and quantity. The N contained in the GM would be available to the next crop (Akbari et al. Reference Akbari, Herbert, Hashemi, Barker and Zandvakili2019). Rapid emergence, quick soil coverage, fast growth, and high dry-matter production are a few of the desirable GM traits that can aid in weed suppression (Brennan and Smith Reference Brennan and Smith2005; Brust et al. Reference Brust, Claupein and Gerhards2014; Phan and Thorup-Kristensen Reference Phan and Thorup-Kristensen2018). Decline in corn yield has been reported when nonlegume GMs are used, because of depletion of residual soil N after corn harvest or insufficient N released from nonlegume biomass (Clark et al. Reference Clark, Meisinger, Decker and Mulford2007; Salmerón et al. Reference Salmerón, Cavero, Quílez and Isla2010). Cereal rye and hairy vetch (Vicia villosa Roth.) are considered to be winter GMs. Ceral rye is not considered to be a source of N for subsequent crops, and residue incorporation can result in net N immobilization depending on the stage of termination (Clark et al. Reference Clark, Meisinger, Decker and Mulford2007; Snapp and Surapur Reference Snapp and Surapur2018); however, hairy vetch is a legume with the ability to fix N (up to 190 kg ha−1) that can be available for subsequent crops (Miville and Leroux Reference Miville and Leroux2018; Teasdale et al. Reference Teasdale, Devine, Mosjidis, Bellinder and Beste2004). Moreover, the synergy in a mixture of cereal rye–vetch has the potential to moderate the weakness of individual single cropping of each species. Several studies have reported benefits of cereal–legume mixtures, such as weed suppression, total dry-matter production, and N availability, in comparison with GM monocultures (Baraibar et al. Reference Baraibar, Hunter, Schipanski, Hamilton and Mortensen2018; Clark et al. Reference Clark, Meisinger, Decker and Mulford2007; Lithourgidis et al. Reference Lithourgidis, Dordas, Damalas and Vlachostergios2011. The objectives of this study were to (1) evaluate the effect of replacement of fallow by cover crops on biomass and density of weed communities across two growing seasons; (2) determine the aboveground nitrogen accumulation and C:N ratios of cover crop species and their effect on subsequent corn yield; (3) assess the effects of reduced rates of nicosulfuron on weed control efficiency and corn productivity; and (4) select the most effective cover crop and/or nicosulfuron dosage, especially in semiarid climatic conditions.
Materials and Methods
Experimental Site
A 2-yr field experiment was conducted in the 2013 to 2014 and 2014 to 2015 growing seasons at the Research Farm of the College of Agriculture and Natural Resources, University of Tehran, Karaj, Iran (35.80°N, 51.00°E). Weather conditions during the experiment are presented in Figure 1. The experimental site is categorized as an arid to semiarid region with a 50-yr average air temperature of 13.5 C, soil temperature of 14.5 C, and 262 mm of rainfall.

Figure 1. Climatic data during the growth period of green manures and corn from the station situated at the experimental site. Tmax, Tmin, and Tmean represent maximum, minimum, and average temperatures respectively.
The October to April temperatures were 9.10 C in 2013 to 2014 and 9.43 C in 2014 to 2015 during cover crop establishment. However, precipitation was lower in the same period in 2013 to 2014 than in 2014 to 2015, at 117 and 163 mm, respectively. The maximum temperature was similar during this period for both seasons, but minimum values in the first season were lower than in the second season, 3.41 and 4.14 C, respectively; specifically in December 2013, when the minimum temperature dropped below 0 C 20 times. Temperature from May to September of both years and during corn establishment was the same; however, precipitation was higher early after corn planting in June of 2013 to 2014 but higher in July and September of 2014 to 2015. The soil at the location is Typic Haplocambid (Afshar et al. Reference Afshar, Jovini, Chaichi and Hashemi2014; Soil Survey Staff Reference Soil Survey1999). Soil samples from this site at 0- to 30-cm depth were analyzed for selected physicochemical attributes before planting (Table 1).
Table 1. General properties of the soil of the experimental site (depth of 0–30 cm).

Experimental Setup
The experiment was designed as a split-plot randomized complete block with four replicates. The main plots of 12 m by 20 m were allocated to three cover crop treatments including single cropping of cereal rye, single cropping of hairy vetch, and a mixture of cereal rye and vetch (50:50). Additionally, a “weedy fallow” control plot with no cover crops planted was included. The seeding rate of cereal rye with a 1,000-seed weight of 25 g was 160 kg ha−1, and the seeding rate of hairy vetch with a 1,000-seed weight of 33g was 50 kg ha−1. The mixed treatment of cereal rye and vetch was at half of the seeding rate of each crop. Planting was performed on October 20 of each year. No fertilizers or herbicides were applied before or during the fallow or cover-cropping period. There were no crops grown before the fallow, and cover crops were grown for about 6 mo during the fallow period in autumn and winter and then disked into the soil on May 28 of each growing season after cover crop and soil samples were taken for biomass and N analysis. Mostly Persian speedwell (Veronica persica Poir.), wild mustard (Sinapis arvensis L.), shepherd’s purse [Capsella bursa-pastoris (L.) Medik.], hedge mustard [Sisymbrium officinale (L.) Scop.], and volunteer wheat (Triticum sp.) and barley (Hordeum sp.) were weeds during the fallow period. The corn cultivar ‘Single Cross 704’ (Seed and Plant Improvement Institute, Karaj, Alborz Province, Iran) was planted on June 21 of each growing season. Corn seeds were planted with a no-till planter with a 75-cm distance between rows and 25-cm distance within rows at 4-cm soil depth. The subplots of 10 m by 3 m were allocated to four different rates of nicosulfuron (Sigma-Aldrich, Steinheim, Germany). Nicosulfuron was applied POST broadcast to corn at the V6 to V8 stage of growth at 80, 60, 40, or 20 g ai ha−1, equivalent to 1.0X, 0.75X, 0.5X, and 0.25X the recommended use rate, respectively. An untreated check was included. The herbicide treatments were applied using a pressurized backpack sprayer delivering 280 L ha−1 at 210 kPa. The replications were 3 m apart from one another to prevent the movement of plant nutrients present in the soil or off-target herbicide drift.
Measurements
Crop and Weed Data Collection
Cover crop samples were collected within each plot by harvesting plants at ground level in a 1- m2 quadrat that was placed at random three times within each plot. In both growing seasons and before the incorporation of cover crops into the soil, a 25 cm by 25 cm quadrat was placed randomly at four spots within each main plot as well as in the weedy fallow treatment to record weed data. Weeds were cut to ground level, counted, and identified by species and separately oven-dried at 70 C for 72 h. Weed density (plants m−2) and weed aboveground biomass (g m−2) were recorded. The height, number of leaves, number of kernel rows per ear, number of kernels per row, number of kernels per ear, and grain yield were determined at harvest for corn. Ears of corn were harvested on September 26, 2013, and September 29, 2014 by hand from 3 m of the central row within each plot.
Total N, C, and NO 3-N
Green manure total N and carbon content were determined by Kjeldahl and combustion methods, respectively (Bremner and Mulvaney Reference Bremner and Mulvaney1982; Pella Reference Pella1990). Soil samples were taken at a depth of 30 cm in each main plot at two times: before cover crop sowing and before incorporation. At each sampling time, three subsamples of soil were collected from each main plot. From each sample, 8 g of soil were shaken in 40 ml of 0.01M CaCl2 at 200 oscillations min−1 for 15 min and then filtered using medium-grade filter paper (Zandvakili et al. Reference Zandvakili, Barker, Hashemi, Etemadi, Autio and Weis2019). The filtrate was analyzed for nitrate by colorimetric determination using flow-injection analysis (QuickChem 8000, LaChat Instruments, Loveland, CO; Cole et al. Reference Cole, Zandvakili, Xing, Hashemi, Herbert and Mashayekhi2019b). All extracted samples were stored at 4 C until processing.
Weed Control Efficiency
Weed control efficiency (WCE) represents the magnitude of weed reduction due to weed control treatment. It is expressed in percentage and calculated on a dry weight basis using the following formula (Mani et al. Reference Mani, Malla and Gautam1973):

where WCE is weed control efficiency (%), DWC is dry weight of weeds in the weedy control plot (g m−2), and DWT is dry weight of weeds in the treated plot (g m−2).
Dominant Weed Species
Dominant weed species were identified using the summed dominance ratio (SDR), computed as follows (Janiya and Moody Reference Janiya and Moody1989):



Statistical Analysis
Data were analyzed using PROC MIXED in SAS software (SAS v. 9.1.3, SAS Institute, Cary, NC, USA). The cover crop and herbicide treatments were considered fixed effects, while years and replications nested within years were considered random effects. Distribution of the data was tested using the Kolmogorov-Smirnov test and was normal (Akbari et al. Reference Akbari, Herbert, Hashemi, Barker and Zandvakili2019). There was no statistically significant interaction between year and the treatments; however, if the effect of treatment was significant by F-test, means were separated by Duncan’s new multiple range test at P = 0.05 (Steel and Torrie Reference Steel and Torrie1980).
Results and Discussion
Fallow Period
Visual assessment of the observed spacing between plants showed that the emergence of cover crops was uniform at 2 wk after sowing in both years. The time of termination for cereal rye and hairy vetch was at the beginning of the flowering stage. There was no significant interaction between year and the cover crops; however, the total aboveground dry matter was significantly higher for the cover crops than for the weedy fallow, and cover crop aboveground biomass was the highest for the mixture of hairy vetch and cereal rye for both years, but it was not significantly different from the aboveground biomass for the single cropping of cereal rye (Table 2).This indicates that, cover crops significantly reduced weed density and aboveground biomass (Tables 3 and 4). Mainly V. persica, S. arvensis, C. bursa-pastoris, S. officinale, and Triticum sp. and Hordeum sp. were weeds during the experiment and at GM termination time. The SDR revealed that the most-dominant weed species was S. officinale, with 50% more total dominance in terms of density and biomass than other weed species (Figure 2).
Table 2. Aboveground dry biomass (DM), total N concentration, N content, and C:N ratio at the time of incorporation of cover crops with soil NO3-N at the time of cover crop sowing and incorporation. a

a Means followed by different letters in columns are significantly different by Duncan’s new multiple range test (P = 0.05) (average of 2 yr).
Table 3. Weed density present in cover crops at the termination of the experiment (average of 2 yr).

a Means followed by different letters in rows are significantly different by Duncan’s new multiple range test (P = 0.05).
b The sum of the number of plants across C. bursa-pastoris, V. persica, S. officinale, S. arvensis, and volunteer crops.
Table 4. Weed aboveground biomass at cover crop termination (average of 2 yr). a

a Means followed by different letters in rows are significantly different by Duncan’s new multiple range test (P = 0.05).
b The sum of the number of plants across C. bursa-pastoris, V. persica, S. officinale, S. arvensis, and volunteer crops.

Figure 2. Summed dominant ratio expressing percent of the winter weed dominance in the weedy fallow, with respect to weed species including Sisymbrium officinale, Capsella bursa-pastoris, Sinapis arvensis, Veronica persica, and volunteer crops (average of 2 yr). Error bars represent standard error.
The highest total weed aboveground biomass among GM treatments was in hairy vetch with 90 g m− 2 averaged over 2 yr (Table 4). Either cereal rye or the mixture of cereal rye and hairy vetch were the most weed-suppressive cover crops and showed higher weed suppression than weedy fallow (Table 4). The total density of the weed species was the highest in weedy fallow, with an average of 60 plants m−2; followed by hairy vetch with an average of 14 plants m−2; whereas density was the lowest in the mixture of cereal rye and hairy vetch, with an average of 7 plants m−2 (Table 3). WCE was above 90% for all the weed species under the GM mixture of cereal rye and hairy vetch (Table 5). The lowest WCE was under hairy vetch (Table 5). The N concentration significantly differed among cover crop treatments, with the highest value obtained in hairy vetch, followed by the mixed treatment, and the lowest value obtained in the weedy fallow and cereal rye (Table 2). GM treatments affected total N content. The highest N content was obtained in the mixed treatment, followed by cereal rye and hairy vetch, and the lowest N content was in weedy fallow (Table 2). In addition, the ratio of C:N was different among GM treatments, and the highest value was in cereal rye, followed by the mixed treatment and weedy fallow, and the lowest value was in hairy vetch (Table 2). Nitrate content in the soil at GM sowing did not vary by the year; however, at GM incorporation, it was different among species. The highest nitrate content was obtained with hairy vetch, with an average of 10.2 mg NO3-N kg−1 dry soil, followed by weedy fallow with 8.0 mg NO3-N kg−1 dry soil. The lowest values were with cereal rye (Table 2). At cover crop termination, the mixed and the single treatments of cereal rye were more suppressive of weeds than hairy vetch, due to their greater growth potential together with a strong competitive ability for N, which minimized the niche for weeds (Campiglia et al. Reference Campiglia, Paolini, Colla and Mancinelli2009; Silva Reference Silva2014). In fact, cover crop species such as cereal rye that are characterized by fast growth and high dry-matter accumulation ensure the highest weed-suppressive ability (Brennan and Smith Reference Brennan and Smith2005; Teasdale and Mohler Reference Teasdale and Mohler2000; White and Weil Reference White and Weil2010). The mixed treatment had the highest biomass, which corresponded to lower weed density. Similar to results reported by Finney et al. (Reference Finney, White and Kaye2016), our results show that increasing cover crop biomass is positively correlated with several ecosystem services, such as weed suppression, nitrate-leaching prevention, and aboveground biomass nitrogen accumulation. In contrast, cover crop species with low dry matter accumulation like hairy vetch had reduced weed control efficacy (Brust et al. Reference Brust, Claupein and Gerhards2014). Cereal rye and the mixed treatment controlled the most dominant weed species, S. officinale, by more than 85% and limited its density to less than 2 plants m−2. Hairy vetch had a higher N content than the cereal rye or the mixed treatment of cereal rye and vetch; however, the single treatment of cereal rye and the mixed treatment accumulated more N than hairy vetch, due to production of more aboveground biomass.
Table 5. Weed control efficiency (WCE) at cover crop termination (average of 2 yr).a

a Means followed by different letters in rows are significantly different by Duncan’s new multiple range test (P = 0.05).
b Means in columns are means of C. bursa-pastoris, V. persica, S. officinale, S. arvensis, and volunteer crops.
These results are contrary to previous reports of N accumulation being significantly greater with the legume aboveground biomass than cereal rye (Campiglia et al. Reference Campiglia, Radicetti, Brunetti and Mancinelli2014; Holderbaum et al. Reference Holderbaum, Decker, Meisenger, Mulford and Vough1990). The C:N ratio was lower in hairy vetch than with cereal rye or the mixed treatment and resulted in a fast release of N content into the soil after incorporation. These results correspond to those of Jahanzad et al. (Reference Jahanzad, Barker, Hashemi, Eaton, Sadeghpour and Weis2016), who reported a faster release of N in legume cover crops compared with cereal rye. In contrast, soil N immobilization could have followed after incorporating cereal rye into the soil due to its high ratio of C:N (Finney et al. Reference Finney, White and Kaye2016). Nitrate-N content of the soil at incorporation was lower for the cereal rye and mixture than for hairy vetch. Campiglia et al. (Reference Campiglia, Paolini, Colla and Mancinelli2009) reported that species of Poaceae are more efficient than legume cover crops in intercepting soil nitrogen (Jahanzad et al. Reference Jahanzad, Barker, Hashemi, Sadeghpour and Eaton2017a, Reference Jahanzad, Barker, Hashemi, Sadeghpour, Eaton and Park2017b). Results based on field observation indicated that the reduction in weed abundance during fallow may lead to a reduction in weed abundance in the subsequent cash crops, and this practice can be an effective method to reduce chemical inputs (Blackshaw et al. Reference Blackshaw, Moyer, Doram and Boswell2001). However, we did not see this result in our study, and the incorporation of cover crops did not affect weed density or biomass in the subsequent corn planting. This effect maybe because of the low decomposition rate and lack of synchrony between N mineralization and corn N demand.
Corn Growth
The SDR revealed that the most dominant weed species was redroot pigweed (Amaranthus retroflexus L.), with a 50% SDR, followed by common lambsquarters (Chenopodium album L.), with 26% SDR compared with other weed species (Figure 3). There was no significant interaction between year or cover crop treatments with weed biomass and density; however, nicosulfuron application rates significantly affected aboveground weed biomass and weed density (Tables 6 and 7). The total biomass of weeds was consistently lower when nicosulfuron was applied, regardless of treatment rate, compared with the no-herbicide treatment (Table 7). The recommended rate treatment showed higher weed suppression than the no-herbicide treatment; however, there was no difference between reduced rates of 60 and 40 g ai ha−1 (Figure 4). Corn yield differed with doses of nicosulfuron (Figure 5). However, corn yield was not affected by year or cover crop treatment. There was an increase in measured variables as nicosulfuron rate increased. Height, number of leaves, number of kernels per row, and grain yield were higher under reduced application of nicosulfuron than in the no-herbicide treatment (Figure 5). The greater grain yield was attributed to a significant increase in kernels per row among the yield components. However, there were no significant differences in height, number of leaves, number of kernels per row, and grain yield between nicosulfuron applied at 40, 60, or 80 g ai ha−1. The prevalent weed species in the experimental plots included A. retroflexus, C. album, black nightshade (Solanum nigrum L.), common purslane (Portulaca oleracea L.), jimsonweed (Datura stramonium L.), and johnsongrass [Sorghum halepense (L.) Pers.]. With rising concern surrounding herbicide-resistant weeds, cost of production, and public health, reduction in herbicide application rate could be one of the integrative multiple strategies (Perotti et al. Reference Perotti, Larran, Palmieri, Martinatto and Permingeat2020) that should be practiced to reduce crop injury and carryover while maintaining crop yield. However, it was reported that reductions in herbicide use in arid and semiarid areas such as the Mediterranean region are not feasible because of highly competitive dominant weeds (Meissle et al. Reference Meissle, Mouron, Musa, Bigler, Pons, Vasileiadis, Otto, Antichi, Kiss and Palinkas2010). In our study, the dominant weeds included A. retroflexus and C. album, with A. retroflexus being the most competitive regarding the size and density of the plant. As a result, A. retroflexus can cause the most damage to corn; however, corn grain yields will not be greatly influenced by the remaining weeds. Alternatively, optimization of nicosulfuron application rate could be attained based on corn yield rather than WCE, because A. retroflexus can be effectively controlled by nicosulfuron. In our study, phytotoxicity to corn was observed as leaf bleaching of less than 5% after the application of nicosulfuron at the recommended dose. However, the injury was transient and was not observed after 2 wk. implying that phytotoxicity at the recommended use rate did not affect corn growth and development. Herbicide application at reduced rates could increase profits for growers, minimize risk to the environment, and reduce injury on corn and adverse residual effects on susceptible rotational crops (Blackshaw et al. Reference Blackshaw, O’Donovan, Harker, Clayton and Stougaard2006). Faster evolution of herbicide resistance is, however, revealed under a reduced dose of herbicide application (Manalil et al. Reference Manalil, Busi Renton and Powles2011; Norsworthy et al. Reference Norsworthy, Ward, Shaw, Llewellyn, Nichols, Webster, Bradley, Frisvold, Powles and Burgos2012). Recurrent selection with reduced herbicide rates or sublethal rates over several generations has been reported to result in the rapid evolution of herbicide resistance in species such as rigid ryegrass (Lolium rigidum Gaudin) and Palmer amaranth (Amaranthus palmeri S. Watson) (Manalil et al. Reference Manalil, Busi Renton and Powles2011; Perotti et al. Reference Perotti, Larran, Palmieri, Martinatto and Permingeat2020). As a result, reduced herbicide rates in combination with an integrated weed management (IWM) program are recommended to mitigate herbicide resistance (Owen et al. Reference Owen, Beckie, Leeson, Norsworthy and Steckel2015). This study showed that there was a relationship between GM biomass and suppression of the weeds during the fallow period. Over 2 yr, the mixture of cereal rye and hairy vetch had the most weed-suppressive ability and N accumulation in its biomass. Hairy vetch alone was less effective in weed suppression and nitrogen accumulation, due to its low aboveground biomass accumulation. Therefore, in semiarid climatic conditions where there is not enough rainfall in the fall and winter seasons to guarantee the establishment and growth of GMs, it is important to select the most effective strategy in weed suppression and biomass production. It is reported that cover crops with the ability to grow quickly and develop a closed canopy or known to have allelopathic properties have the potential to control weeds in semiarid subtropical Texas (Soti and Racelis Reference Soti and Racelis2020). Moreover, in water-limited environments, additional water extraction by cover crops compared with bare soil needs to be evaluated. It was reported that plant species with rapid, early development that can germinate under dry conditions [e.g., mustard (Brassica rapa L.)] could be included in cover crop mixtures to be used under semiarid conditions (Bodner et al. Reference Bodner, Loiskandl and Kaul2007). In contrast, whole-farm simulation models revealed that fallow can maintain profit and reduce risk in semiarid areas (Cann et al. Reference Cann, Hunt and Malcolm2020). Results from our study suggest that the practice of cover cropping did not affect corn growth; however, corn yield was improved with herbicide application. Applying nicosulfuron as a POST herbicide limited weed density and biomass and resulted in higher corn biomass at all applications (Figure 5). As a result, a reduction in nicosulfuron dose by 50% is feasible without sacrificing weed control efficiency in corn. Future research may focus on the long-term impact of a wider range of cover crop species and their integration with IWM programs to further investigate weed suppression and crop productivity in arid and semiarid regions of the world.

Figure 3. Summed dominant ratio expressing percent of summer weed dominance in the weedy fallow with respect to weed species including Amaranthus retroflexus, Chenopodium album, Solanum nigrum, and volunteer crops (average of 2 yr). Error bars represent standard error.
Table 6. Weed number per species as affected by nicosulfuron application rate (average of 2 yr). a

a Means in columns are averages of 0, 20, 40, 60, and 80 g ai ha−1 nicosulfuron application rates within each weed species. Totals in columns are sum of A. retroflexus, C. album, S. nigrum, and volunteer crops within each nicosulfuron application rate. Means followed by different letters in columns are significantly different by Duncan’s new multiple range test (P = 0.05).
b Means followed by different letters across this row are significantly different by Duncan’s new multiple range test (P = 0.05).
Table 7. Weed aboveground biomass per species as affected by nicosulfuron application rate (average of 2 yr). a

a The sum of the biomass across A. retroflexus, C. album, S. nigrum, and volunteer crops within each nicosulfuron application rate. Means in columns are averages of 0, 20, 40, 60, and 80 g ai ha−1 nicosulfuron application rates within each weed species. Means followed by different letters in columns are significantly different by Duncan’s new multiple range test (P = 0.05).
b Means followed by different letters across this row are significantly different by Duncan’s new multiple range test (P = 0.05).
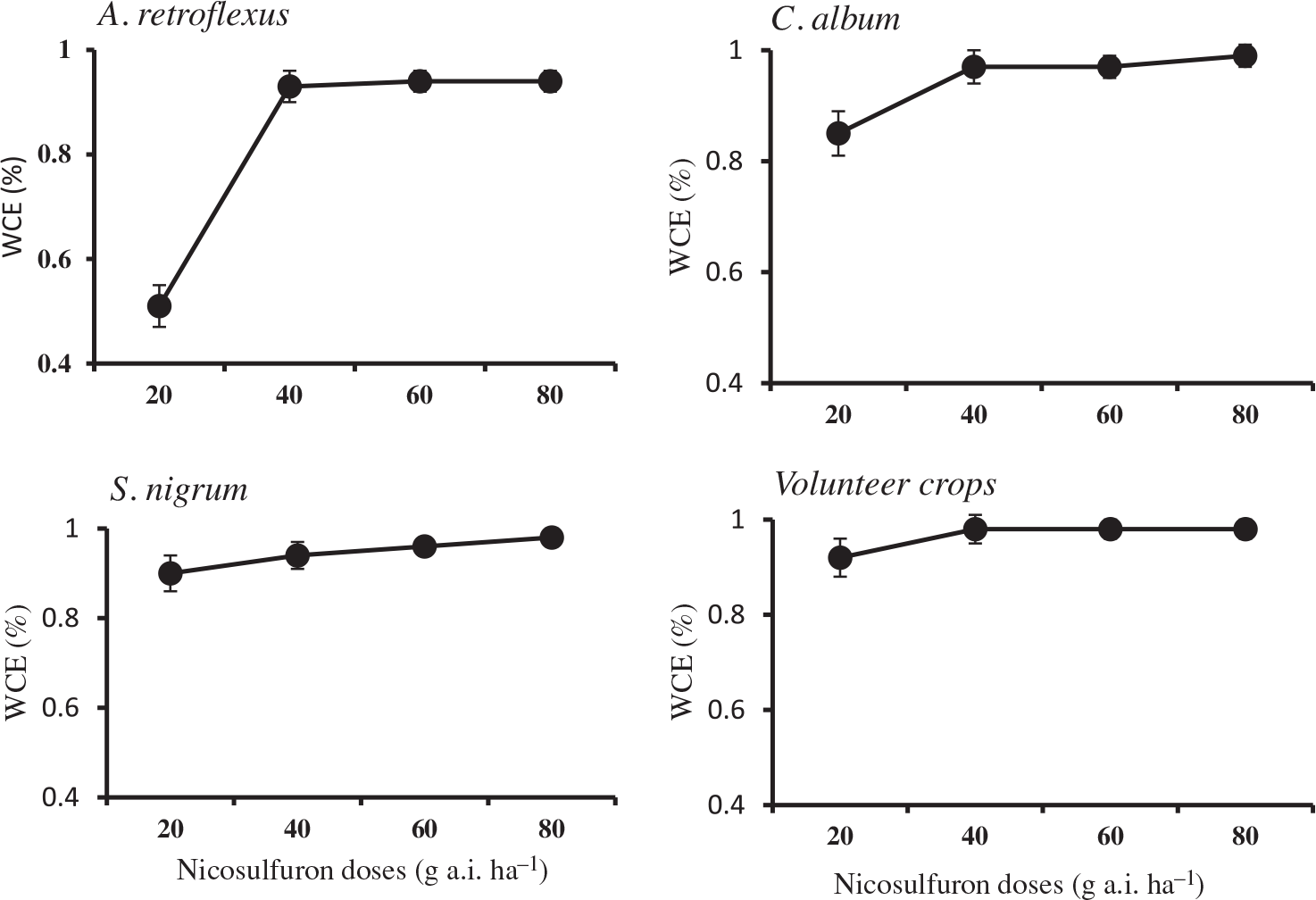
Figure 4. Weed control efficiency (WCE) per species for Amaranthus retroflexus, Chenopodium album, Solanum nigrum, and volunteer crops under nicosulfuron application rates at corn harvest (average of 2 yr).
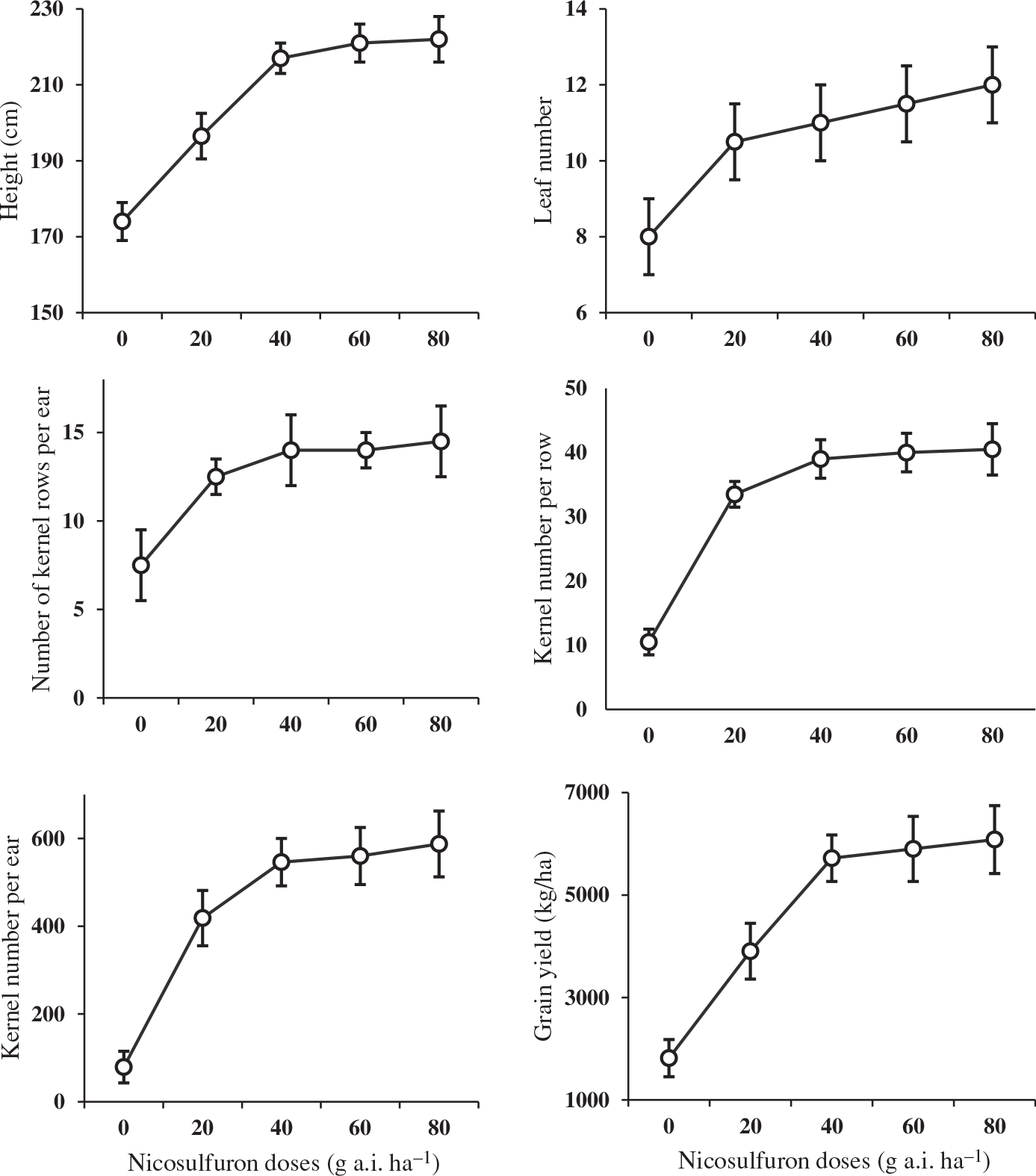
Figure 5. Effect of nicosulfuron application rates on corn height, leaf number, number of kernel rows per ear, number of kernels per row, number of kernels per ear, and grain yield (average of 2 yr).
Acknowledgments
This study was partly supported by financial aid from the College of Agriculture and Natural Resources, University of Tehran. No conflicts of interest have been declared. The authors wish to express their great appreciation to the people who worked on this experiment. The authors especially thank Wesley Autio for his guidance, suggestions, and comments for statistical analysis.