Although herbicide-resistant weeds represent a serious threat to agricultural production, when populations contain resistance to a single herbicide (or group of herbicides having the same mechanism of action), they can generally be managed successfully. However, populations that have evolved resistance to multiple herbicides spanning different mechanisms of action create significant management challenges (Tranel et al. Reference Tranel, Riggins, Bell and Hager2011). Populations of more than 50 weed species have been reported resistant to herbicides with multiple mechanisms of action (Heap Reference Heap2017). The most problematic weeds with multiple resistance in the midwestern and southern United States are waterhemp and Palmer amaranth (Amaranthus palmeri S. Wats.) (Hager and Sprague Reference Hager and Sprague2002; Webster Reference Webster2005). Each species has evolved resistance to herbicides spanning six mechanisms of action (acetolactate synthase [ALS] inhibitors, photosystem II [PSII] inhibitors, enolpyruvylshikimate-3-phosphate synthase [EPSPS] inhibitors, protoporphyrinogen oxidase [PPO] inhibitors, hydroxyphenylpyruvate dioxygenase [HPPD] inhibitors, and TIR1 auxin receptors [waterhemp] or microtubule inhibitors [Palmer amaranth]), and resistance to herbicides spanning five mechanisms of action has been identified in individual populations of waterhemp while resistance spanning three mechanisms of action has been reported in a single population of Palmer amaranth (Heap Reference Heap2017; Schultz et al. Reference Schultz, Chatham, Riggins and Tranel2015). Both species are dioecious (Costea et al. Reference Costea, Weaver and Tardif2005), assuring outcrossing and gene flow among and within populations (Trucco et al. Reference Trucco, Tatum, Robertson, Rayburn and Tranel2006). In addition, both species have high fecundity, and the combination of large genetic variability, high population density, and heavy reliance on herbicides for weed control have increased the frequency of resistant alleles and the stacking of herbicide-resistant traits in populations (Tranel et al. Reference Tranel, Riggins, Bell and Hager2011).
A TIR1 auxin receptor herbicide (2,4-D) was the first synthetic-organic herbicide commercialized (Burnside Reference Burnside1996). Because TIR1 auxin receptors (synthetic auxins) selectively control broadleaf weeds in grass crops, this mechanism of action is one of the most widely used globally (Sterling and Hall Reference Sterling and Hall1997). The frequency of weed resistance to herbicides in this group is relatively low despite their widespread use since 1946 (Gustafson Reference Gustafson2008), perhaps because they are often applied in mixtures with other herbicides or because of the complex ways they interfere with plant growth and their limited persistence in the soil (Sterling and Hall Reference Sterling and Hall1997). The first two documented 2,4-D–resistant weeds were wild carrot (Daucus carota L.) (Switzer Reference Switzer1957) and spreading dayflower (Commelina diffusa Burm. f.) (Hilton Reference Hilton1957). To date, 34 weed species have evolved resistance to synthetic auxin herbicides (Heap Reference Heap2017). Transgenic soybean [Glycine max (L.) Merr.], corn (Zea mays L.), and cotton (Gossypium hirsutum L.) genetically modified with resistance to 2,4-D (Wright et al. Reference Wright, Shan, Walsh, Lira, Cui, Song, Zhuang, Arnold, Lin, Yau, Russell, Cicchillo, Peterson, Simpson, Zhou, Ponsamuel and Zhang2010) and dicamba (Behrens et al. Reference Behrens, Mutlu, Chakraborty, Dumitru, Jiang, LaVallee, Herman, Clemente and Weeks2007) are tools that will help farmers to manage broadleaf weeds resistant to glyphosate. However, this will result in increased selection pressure for weeds, including waterhemp and Palmer amaranth, to evolve resistance to herbicides with this mechanism of action.
In 2009 a farmer contacted scientists from the University of Nebraska–Lincoln and reported a waterhemp population that had survived the maximum labeled rates of 2,4-D. The field containing the putative resistant population had also received annual applications of atrazine and S-metolachlor in addition to 2,4-D. Greenhouse and field experiments confirmed that the waterhemp population was resistant to 2,4-D (Bernards et al. Reference Bernards, Crespo, Kruger, Gaussoin and Tranel2012). Seeds from the 2,4-D–resistant waterhemp population were collected in 2010 for use in this research. Our objectives were: (1) to evaluate the population for resistance to PSII inhibitors, ALS inhibitors, HPPD inhibitors, PPO inhibitors, EPSPS inhibitors, glutamine synthetase inhibitors, and additional herbicides from the TIR1 auxin inhibitors; and (2) to more accurately quantify the level of resistance to 2,4-D using higher 2,4-D doses in a greenhouse bioassay than were used in Bernards et al. (Reference Bernards, Crespo, Kruger, Gaussoin and Tranel2012).
Materials and Methods
Waterhemp Populations
Seed from one 2,4-D–resistant (FS) and two 2,4-D–susceptible waterhemp (SE and SCAL) populations were used in this experiment. The FS population was collected in a field planted with little bluestem grass [Schizachyrium scoparium (Michx.) Nash ‘Camper’] located in Cass County, NE (Bernards et al. Reference Bernards, Crespo, Kruger, Gaussoin and Tranel2012). The SE and SCAL populations were collected from soybean fields in Nemaha County and Clay County, NE, respectively. Each population sample was a composite of at least 40 plants. Waterhemp seed was cleaned and stored at 4 C.
Plant Growth
Herbicide bioassays were conducted in greenhouses located on the East Campus of the University of Nebraska–Lincoln in Lincoln, NE. Supplemental lighting (500 µmolm−2s−1) provided a 15-h photoperiod. Day temperatures varied between 24 and 28 C and night temperatures varied between 18 and 22 C.
Waterhemp seed was germinated by placing it on moistened filter paper in petri dishes, then sealing the petri dishes and placing them in an incubator for 48 to 72 h at 35 C (Ellis et al. Reference Ellis, Hong and Roberts1985; Steckel et al. Reference Steckel, Sprague, Stoller, Wax and Simmons2007). Two or three germinated waterhemp seedlings were transferred into growing mix (BM1® Growing Mix, Berger Peat Moss, Saint-Modeste, QC, Canada) in 10 by 10 by 12.5 cm black plastic pots. Plants were watered as needed and fertilized weekly with Miracle-Gro® fertilizer (Scotts Miracle-Gro, Marysville, OH). The seedlings were thinned to 1 plant pot−1 before herbicide treatments were applied.
Herbicide Application
Herbicide treatments were applied to waterhemp plants when they were 8- to 12-cm tall (5 to 8 fully expanded leaves). A chamber sprayer (DeVries Manufacturing, Hollandale, MN) equipped with a TP8001E flat-fan nozzle tip (TeeJet Spraying Systems, Wheaton, IL) was used to make the herbicide application. The carrier volume used was 190 L ha−1 at a pressure of 207 kPa with 1.6 km h−1 application speed.
Single-Dose Bioassays
The experiments were conducted in two experimental runs. Fifty plants from each waterhemp population were treated with a single dose of each of the first six herbicides listed in Table 1. Visible injury estimates were made at 7, 14, 21, and 28 d after treatment (DAT) and were compared with estimates for untreated plants (controls) using a scale of 0 (no injury) to 100 (dead plants). At 28 DAT, plants were severed at the base and dried for 48 h in a forced-air dryer at 65 C, after which dry weight biomass was measured. Mean values and standard error bars were graphed using SigmaPlot 12.2 (Systat Software, San Jose, CA).
Table 1 List of herbicides used.
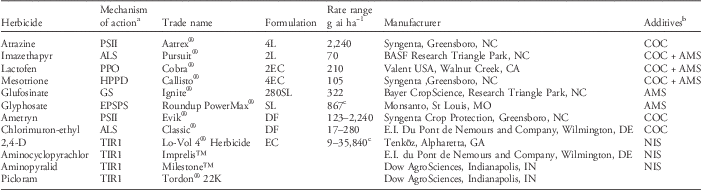
a Abbreviations for site of action: ALS, acetolactate synthase; EPSPS, enolpyruvylshikimate-3-phosphate synthase; GS, glutamine synthetase; HPPD, hydroxyphenylpyruvate dioxygenase; PPO, protoporphyrinogen oxidase; PSII, photosystem II.
b Abbreviations for additives: COC, crop oil concentrate at 1% (v/v); AMS, ammonium sulfate at 2.5% (v/v); NIS, nonionic surfactant at 0.25% (v/v).
c Acid equivalent (g ae ha−1).
Dose–Response Bioassays
Response to PSII- and ALS-inhibiting Herbicides
Dose–response experiments using ametryn or chlorimuron-ethyl (Table 1) were conducted on the FS and SE and SCAL waterhemp populations. The experimental design was a randomized complete block with 10 replications per treatment and experimental run. Five ametryn doses were applied: 0, 123, 560, 1,120, and 2,240 gaiha−1. In a separate experiment, six chlorimuron-ethyl doses were applied: 0, 17, 35, 70, 140, and 280 g ai ha−1. Treatment solutions included a 1% (v/v) crop oil concentrate adjuvant. Each dose–response experiment was conducted in two experimental runs.
Response to TIR1 Auxin Receptor Herbicides
The maximum rate of 2,4-D used in greenhouse bioassays by Bernards et al. (Reference Bernards, Crespo, Kruger, Gaussoin and Tranel2012) was 2,240 gaeha−1, which was inadequate to control the resistant population. In the greenhouse bioassay reported in this paper, we used 2,4-D doses that matched the previous field bioassay (Bernards et al. Reference Bernards, Crespo, Kruger, Gaussoin and Tranel2012) to better characterize the level of resistance. The FS waterhemp was treated with 2,4-D at 0, 140, 280, 560, 1,120, 2,240, 4,480, 8,960, 17,920, and 35,840 gha−1. The SE and SCAL waterhemp populations were treated with 2,4-D at 0, 9, 18, 37, 70, 140, 560, 1,120, 2,240, and 4,480 gha−1. Dose–response experiments were also conducted using eight doses of each of the following herbicides: aminocyclopyrachlor, aminopyralid, and picloram on the FS, SE, and SCAL populations (Table 1; see Table 2 for herbicide doses). In preliminary experiments the FS population was less injured by picloram than 2,4-D-susceptible populations (unpublished data), therefore, the FS population was treated with greater picloram doses compared with the susceptible populations. All dose–response experiments were arranged in a randomized complete block design with five replications each, and were conducted in two experimental runs. Treatments containing 2,4-D, aminocyclopyrachlor, and aminopyralid applications included nonionic surfactant (NIS) at 0.25% (v/v). Treatments containing picloram were applied without an adjuvant.
Table 2 TIR1 auxin receptor herbicides and doses applied to 2,4-D–resistant and 2,4-D–susceptible waterhemp populations.

a Both susceptible and resistant populations received the same doses of aminocyclopyrachlor and aminopyralid.
Data Collection and Statistical Analysis
Visible injury estimates were made at 7, 14, 21, and 28 DAT based on each particular herbicide injury symptom compared with untreated controls using a scale of 0 (no injury) to 100 (dead plants). At 28 DAT, all plants for each treatment at each dose–response experiment were harvested and dried for 48 h in a forced-air dryer at 65 C, after which dry weight biomass was recorded.
Visible injury estimates and dry weight at 28 DAT were analyzed using a nonlinear regression model with the ‘drc’ package in R v. 2.3.0 (Knezevic et al. Reference Knezevic, Streibig and Ritz2007; R Core Team 2014). Dose–response models were constructed using a four-parameter log-logistic equation (Equation 1) (Streibig et al. Reference Streibig, Rudemo and Jensen1993; Seefeldt et al. Reference Seefeldt, Jensen and Fuerst1995):

where y is the response based on visible injury estimate or dry weight, c is the lower limit, d is the upper limit, x is the herbicide dose, e is the herbicide dose giving a 50% response (injury estimation [I50] or dry weight reduction [GR50]) between the upper and lower limit, and is also the inflection point, and b is the slope of the line at the inflection point. The ametryn or chlorimuron-ethyl doses needed to achieve 50%, 80%, and 90% visible injury estimates (I) and dry weight (GR) at 28 DAT were calculated. The relative level of resistance was expressed by calculating the R:S ratios between the I or GR values of the least susceptible biotype and the I or GR values of the most susceptible biotype (Beckie et al. Reference Beckie, Heap, Smeda and Hall2000). Standard error bars shown in the figures were calculated for each treatment using mean and standard error functions in SigmaPlot 12.2 (Systat Software, San Jose, CA).
Waterhemp Molecular Analysis
The results of the first run of the single-dose herbicide bioassays led us to suspect that there might be resistance to ALS-, PSII- and PPO-inhibiting herbicides among the FS, SE, and SCAL populations. Prior to herbicide application in the second run of the single-dose herbicide experiment described above, a young fully expanded leaf was collected from each plant, placed in a labeled 1.5-ml Eppendorf tube, and then stored in a freezer at −20 C until sample analysis. After plants were valuated for herbicide response, tissue samples from five suspected ALS-, atrazine-, or lactofen-resistant plants and five susceptible plants for each population were selected for molecular evaluation. Genetic analyses were conducted in laboratories located at the University of Illinois at Urbana, IL. Samples were evaluated for the Trp-574-Leu mutation conferring resistance to sulfonylurea and imidazolinone herbicides and/or substitution at Ser-653, which confers resistance to imidazolinone herbicides (Patzoldt and Tranel Reference Patzoldt and Tranel2007). Additionally, we tested for the presence of Ser-264-Gly, Ser-264-Thr, Val-219-Ile, Ala-251-Val, and Asn-266-Thr mutations in the psbA gene conferring resistance to PSII-inhibiting herbicides (Foes et al. Reference Foes, Tranel, Wax and Stoller1998; Patzoldt et al. Reference Patzoldt, Dixon and Tranel2003). Samples with suspected resistance to PPO-inhibiting herbicides were evaluated for the 3-base pair deletion in the PPX2L gene (Lee et al. Reference Lee, Hager and Tranel2008).
Analysis of the ALS gene was done by isolating DNA from leaf tissue samples and using PCR to amplify region B of the ALS gene, which encompasses the Trp-574-Leu mutation. The following primers were used: AmALS-F2: 5′-TCCCGGTTAAAATCATGCTC; and AmALS-R2: 5′-CTAAACGAGAGAACGGCCAG (Foes et al. Reference Foes, Tranel, Wax and Stoller1998). The Trp-574-Leu mutation in the ALS gene creates a recognition site for the MfeI restriction enzyme, thus a PCR-RFLP assay was conducted as previously described by Foes et al. (Reference Foes, Liu, Vigue, Stoller, Wax and Tranel1999) and Schultz et al. (Reference Schultz, Chatham, Riggins and Tranel2015). After digestion, DNA fragments were separated on a 1% agarose gel and visualized with a Kodak Gel Logic 1500 Imaging System (Eastman Kodak Company, Rochester, NY). Individual plants were classified as homozygous for the L574 ALS allele and heterozygous or homozygous for the W574 allele based on the presence of DNA fragments with approximate base pair sizes of 389 bp (homozygous for L574) or 440 bp (uncut, homozygous for W574). Fragments smaller than 51 bp usually are not visible on the gel.
Additionally, we looked for mutations at the Ser-653 site of the ALS gene that are known to confer resistance to imidazolinone herbicides in waterhemp (Patzoldt and Tranel Reference Patzoldt and Tranel2007). Five FS plants that tested negative for the Trp-574-Leu mutation and two 2,4-D sensitive plants that tested positive for Trp-574-Leu were examined. Mutations at position 653 were confirmed by sequencing and by allele-specific PCR using codon-specific primers (Patzoldt and Tranel Reference Patzoldt and Tranel2007). PCR products were separated in a 1% agarose gel containing ethidium bromide and visualized with UV light.
DNA sequencing was also performed to identify the Ser-264 mutation in the psbA gene for atrazine resistance. Total DNA was extracted from leaf tissue, and a region of the chloroplast psbA gene encoding the Dl protein was selectively amplified with primers AmpsbAsF1: 5′-ATGAGGGTTACAGATTTGGTC and AmpsbAsR1: 5′-AGATTAGCACGGTTGATGATA. Digestion products were separated by electrophoresis through a 1% agarose gel and visualized under UV light with ethidium bromide staining (Schultz et al. Reference Schultz, Chatham, Riggins and Tranel2015).
Samples with suspected resistant to PPO-inhibiting herbicides were evaluated for the 3-base pair deletion in the PPX2 gene (Lee et al. Reference Lee, Hager and Tranel2008). DNA was extracted from leaf tissue samples, and allele-specific primers described previously by Lee et al. (Reference Lee, Hager and Tranel2008) were used to screen samples for the codon deletion in the gene that results in the deletion of Gly-210. Products from PCR amplification and digestion were fractionated in 2% agarose gel containing ethidium bromide and visualized with UV light (Lee et al. Reference Lee, Hager and Tranel2008; Schultz et al. Reference Schultz, Chatham, Riggins and Tranel2015).
Results and Discussion
Single-Dose Bioassays
All three populations (FS, SCAL, and SE) showed less than 10% injury from atrazine (Figure 1). Two of the populations were collected from fields with long histories of atrazine use (FS and SCAL). The FS population was exposed to annual applications of atrazine beginning in 1996 (Bernards et al. Reference Bernards, Crespo, Kruger, Gaussoin and Tranel2012), and the SCAL population was from a University of Nebraska–Lincoln research farm where atrazine was frequently used to manage weeds in corn and sorghum (unpublished data). The third population (SE) was from a soybean–corn field that likely had a history of atrazine use. Anderson et al. (Reference Anderson, Roeth and Martin1996) reported that 92% of suspected atrazine-resistant waterhemp populations from southeast Nebraska were indeed resistant. Consequently, it was not surprising that all three populations showed little injury after being treated with labeled field rates of atrazine. However, the absence of a susceptible control prevents us from definitively concluding that they are resistant to atrazine.

Figure 1 Visible injury estimates from two experimental runs of the 2,4-D–resistant (FS) and 2,4-D–susceptible (SE and SCAL) waterhemp populations to a single labeled dose of atrazine (2,240 gaiha−1), imazethapyr (70 gaiha−1), lactofen (210 gaiha−1), mesotrione (105 gaiha−1), glufosinate (322 gaiha−1), and glyphosate (867 gaiha−1). Vertical bars represent the standard error of the mean. Data represent the average of 50 plants population−1 herbicide−1 for each experimental run.
None of the three populations were completely controlled by imazethapyr (Figure 1). Plants from the FS population were not sensitive to imazethapyr at 28 DAT. Injury to plants from the SE and SCAL populations was more variable, but averaged less than 30% and 45%, respectively. Resistance to ALS-inhibiting herbicides is presumed to be widespread among waterhemp populations in Nebraska (Bernards et al. Reference Bernards, Gaussoin, Klein, Knezevic, Kruger, Lyon, Reicher, Sandell, Young, Wilson, Shea and Ogg2011), and the response observed in these bioassays supports that assumption. The lack of response in the FS population was somewhat surprising, because the field where the seed was collected had not been in corn or soybean production since 1995, and the owner did not report the use of ALS-inhibiting herbicides in the management of the little bluestem growing there. However, the first reports of ALS-resistant waterhemp in the midwestern United States were made in 1993 (Heap Reference Heap2017). The ALS resistance may have been in the population prior to the field being converted to little bluestem, or it may have been introduced through pollen-mediated gene flow from waterhemp in nearby corn and soybean fields, or introduced as a seed contaminant (Horak and Peterson Reference Horak and Peterson1995).
Waterhemp injury caused by lactofen was similar among the three populations, and ranged between 62% and 69% in the first bioassay run and 70% and 78% in the second run (Figure 1). Lactofen injury symptoms in the first 2 DAT included chlorosis, necrosis, and crinkling. Plants produced new growth within 14 DAT, and more than half of the plants in each biotype and run recovered and were actively growing at 28 DAT (unpublished data). Shoup and Al-Khatib (Reference Shoup and Al-Khatib2005) noted similar symptoms in the first case of PPO inhibitor–resistant waterhemp reported in Kansas, but less severe final injury estimates. All three waterhemp populations were sensitive to glufosinate, glyphosate, and mesotrione, and injury estimates were 80% or higher for each (Figure 1).
Dose–Response Bioassays
Response to PSII- and ALS-inhibiting Herbicides
The labeled rate of 2,240 gha−1 of ametryn resulted in visual injury ratings of 77% for the FS population and 93% for the SE and SCAL populations (Figure 2). Plants from FS population were less sensitive to ametryn than the SE or SCAL populations, based on 28 DAT visual injury estimates (Table 3; Figure 2) but not dry weight reduction (Table 4; Figure 3). The R:S ratio between the FS and most susceptible population never exceeded 2, suggesting there is no resistance to ametryn among these populations.

Figure 2 Visual injury estimate as affected by ametryn dose for 2,4-D–resistant (FS) and 2,4-D–susceptible (SE and SCAL)waterhemp biotype at 28 DAT in greenhouse bioassays. Regression parameters are provided in Table 2. Data represent the mean of two experimental runs and 10 replications per experiment. The error bars represent the standard error for each data point.

Figure 3 Percent dry weight reduction relative to untreated control as affected by ametryn dose at 28 DAT of 2,4-D–resistant (FS) and 2,4-D–susceptible (SE and SCAL) waterhemp populations in greenhouse bioassays. Regression parameters are given in Table 3. Data represent the mean of two experimental runs and 10 replications per experiment. The error bars represent the standard error for each data point.
Table 3 Visible injury estimate (I) regression parameters, ametryn (Evik® DF, Syngenta) and chlorimuron-ethyl (Classic® DF, DuPont™) doses necessary to achieve I50, I80, and I90 values, and standard errors (se) at 28 DAT for 2,4-D–resistant (FS) and 2,4-D–susceptible (SE and SCAL) waterhemp populations from Nebraska.

a Regression parameters were estimated using a four-parameter log-logistic equation, y=c+(d – c/1+exp (b (log x – log e))), where c represents the lower limit (0=no injury), d represents the upper limit (100=plant death), b represents the slope of the line at the inflection point, and e represents the herbicide dose necessary to provide 50% injury (I50).
b R:S is the resistant:susceptible ratio between the least susceptible biotype and the most susceptible biotype.
Table 4 Dry weight reduction (GR) regression parameters, ametryn (Evik® DF, Syngenta) and chlorimuron-ethyl (Classic® DF, DuPont™ ) doses necessary to achieve GR50, GR80, and GR90, and standard errors (se) at 28 DAT for 2,4-D−resistant (FS) and 2,4-D−susceptible (SE and SCAL) waterhemp populations from Nebraska.

a Regression parameters were estimated using a four-parameter log-logistic equation, y=c+(d – c/1+exp (b (log x – log e))), where, where c represents the lower limit (minimum dry weight for each biotype), d represents the upper limit (maximum dry weight for each biotype), b represents the slope of the line at the inflection point, and e represents the herbicide dose necessary to provide 50% reduction in dry matter (GR50).
b R:S is the resistant:susceptible ratio between the least susceptible biotype and the most susceptible biotype.
The FS population was less sensitive to chlorimuron-ethyl than the SE or SCAL populations based on visual injury estimates (Figure 4; Table 3) and dry weight reduction (Figure 5; Table 4). The R:S ratios were 7.1 for 50% visual injury (I50) and 3.7 for 50% dry weight reduction (GR50). None of the populations were controlled at the 80% visual injury level at the maximum rate tested of 280 gha−1, which is 21 times greater than the labeled use rate of 13 gha−1. The dose required to reduce dry weight 80% (GR80) ranged from 41 to 131 gha−1. Lovell et al. (Reference Lovell, Wax, Horak and Peterson1996) reported a 330-fold resistance based on visible injury compared with the susceptible waterhemp biotype with chlorimurom-ethyl. Other studies have used thifensulfuron in bioassays and reported 28-, 490-, 18,000- and 34,000-fold differences between resistant and susceptible waterhemp populations (Lovell et al. Reference Lovell, Wax, Horak and Peterson1996; McMullan and Green Reference McMullan and Green2011; Patzoldt et al. Reference Patzoldt, Tranel and Hager2005; Patzoldt and Tranel Reference Patzoldt and Tranel2007). This bioassay, however, did not use a known susceptible biotype, so we cannot conclusively confirm herbicide resistance (Beckie et al. Reference Beckie, Heap, Smeda and Hall2000), even though the rates required to control these populations greatly exceeded commercial use rates.

Figure 4 Visual injury estimate as affected by chlorimuron-ethyl dose for 2,4-D–resistant (FS) and 2,4-D–susceptible (SE and SCAL) waterhemp biotype at 28 DAT in greenhouse bioassays. Regression parameters are provided in Table 3. Data represent the mean of two experimental runs and 10 replications per experiment. The error bars represent the standard error for each data point.

Figure 5 Percent dry weight reduction relative to untreated control as affected by chlorimuron-ethyl dose at 28 DAT of 2,4-D–resistant (FS) and 2,4-D–susceptible (SE and SCAL) waterhemp populations in greenhouse bioassays. Regression parameters are given in Table 3. Data represent the mean of two experimental runs and 10 replications per experiment. The error bars represent the standard error for each data point.
Response to TIR1 Auxin Receptor Herbicides
The FS population was approximately 50-fold resistant to 2,4-D relative to the SCAL population based on visual injury (I80) and dry weight reduction (GR80) (Tables 5 and 6). In the current study, the maximum 2,4-D dose of 35,840 gha−1 was adequate to kill (100% visible injury at 28 DAT) waterhemp plants of the FS population. Thus, the log-logistic model estimate of the I80, I90, GR80, and GR90 for the FS population are more reliable estimates than those reported by Bernards et al. (Reference Bernards, Crespo, Kruger, Gaussoin and Tranel2012), in which the maximum 2,4-D dose was 2,240 gha−1. Doses of 2,4-D greater than 24,000 gha−1 were required to achieve 90% injury and 90% dry weight reduction in the FS population.
Table 5 Visible injury estimate (I) regression parameters, 2,4-D (Lo-Vol 4®, Tenkōz), aminocyclopyrachlor (Imprelis™, DuPont™), aminopyralid (Milestone™, Dow AgroSciences™) and picloram (Tordon® 22k, Dow AgroSciences) doses necessary to achieve I50, I80, and I90 values, and standard errors (se) at 28 DAT for 2,4-D–resistant (FS) and 2,4-D–susceptible (SE and SCAL) waterhemp populations from Nebraska.

a Regression parameters were estimated using a four-parameter log-logistic equation, y=c+(d – c/1+exp (b (log x – log e))), where c represents the lower limit (0=no injury), d represents the upper limit (100=plant death), b represents the slope of the line at the inflection point, and e represents the herbicide dose necessary to provide 50% injury (I50).
b R:S is the resistant:susceptible ratio between the least susceptible biotype and the most susceptible biotype.
Table 6 Dry weight reduction (GR) regression parameters, 2,4-D (Lo-Vol 4®, Tenkōz), aminocyclopyrachlor (Imprelis™, DuPont™), aminopyralid (Milestone™, Dow AgroSciences™), and picloram (Tordon® 22k, Dow AgroSciences) doses necessary to achieve GR50, GR80, and GR90, and standard errors (se) at 28 DAT for 2,4-D–resistant (FS) and 2,4-D–susceptible (SE and SCAL) waterhemp populations from Nebraska.
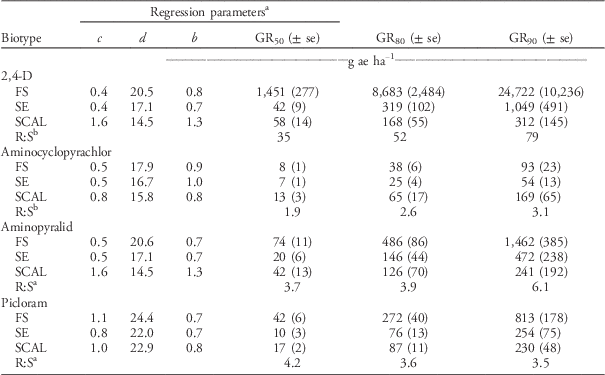
a Regression parameters were estimated using a four-parameter log-logistic equation, y=c+(d – c/1+exp (b (log x – log e))), where c represents the lower limit (0=no injury), d represents the upper limit (100=plant death), b represents the slope of the line at the inflection point, and e represents the herbicide dose necessary to provide 50% dry weight reduction (GR50).
b R:S is the resistant:susceptible ratio between the least susceptible biotype and the most susceptible biotype.
The FS population was less susceptible to aminocyclopyrachlor, aminopyralid, and picloram herbicides than the SE or SCAL populations based on visual injury estimates (Table 5). The R:S ratios for I50 were 2.4 for aminocyclopyrachlor, 4.7 for aminopyralid, and 4.7 for picloram. When the analyses were based on dry weight reduction, the FS population was less susceptible to aminopyralid and picloram than the SE or SCAL populations, but more susceptible to aminocyclopyrachlor than the SCAL population (Table 6). None of the TIR1 auxin inhibitor herbicides evaluated were exceptionally effective in controlling these waterhemp populations. In general, the labeled use rates of aminocyclopyrachlor (80 gaeha−1), aminopyralid (88 gaeha−1), and picloram (280 gaeha−1) were inadequate to achieve 90% visual injury or dry weight reduction for any of the populations (Tables 5 and 6). In particular, the FS population required 7-, 11-, and 16-fold higher doses than recommended field rates for aminocyclopyrachlor, aminopyralid, and picloram, respectively, based on visible injury estimates (Table 5). The synthetic auxin herbicides we evaluated are labeled for pasture and range applications where waterhemp is less likely to be a troublesome weed and are not used in corn or soybean. Bernards et al. (Reference Bernards, Crespo, Kruger, Gaussoin and Tranel2012) found the FS population to have 3-fold resistance to dicamba based on visual injury estimates but less than 2-fold resistance for dry weight reduction.
Waterhemp Molecular Analysis
Based on the responses of the FS, SE, and SCAL populations to atrazine, ALS-inhibiting herbicides, and lactofen, we evaluated each population for the presence of alleles that confer resistance to those herbicides. A serine to glycine substitution at amino acid number 264 of the D1 protein (encoded by the chloroplastic psbA gene) has been associated with atrazine resistance in other species (Devine and Preston Reference Devine and Preston2000; Hirschberg and McIntosh Reference Hirschberg and McIntosh1983). Sequencing results of the psbA gene of two atrazine-resistant plants of each of the waterhemp populations (FS, SE, and SCAL) did not identify the Ser-264 mutation. Patzoldt et al. (Reference Patzoldt, Dixon and Tranel2003) reported triazine resistance in some Illinois waterhemp populations conferred by a nuclear-inherited, non–target site mechanism. All three populations were sensitive to ametryn (Tables 3 and 4), another PSII-inhibiting herbicide. Ametryn binding is not affected by the Ser-264-Gly substitution. Susceptibility to ametryn is consistent with other waterhemp populations resistant to atrazine but lacking a target-site mutation (Patzoldt et al. Reference Patzoldt, Dixon and Tranel2003). Because the non–target site mechanism of triazine resistance can be transmitted by seed and/or pollen, it is expected to be distributed more rapidly than the target-site mechanism due to the long-distance dispersal of wind-borne pollen and obligate outcrossing in dioecious Amaranthus species (Costea et al. Reference Costea, Weaver and Tardif2005; Tranel et al. Reference Tranel, Riggins, Bell and Hager2011; Trucco et al. Reference Trucco, Tatum, Robertson, Rayburn and Tranel2006). Based on the complete lack of response to atrazine in the single-dose bioassay combined with the absence of the Ser-264 mutation that confers target-site resistance in all three waterhemp populations, we speculate that these populations likely have a non–target site resistance mechanism to atrazine.
Most cases of ALS resistance in Amaranthus weed species are conferred by mutations in the ALS gene. Using a PCR-RFLP technique, we analyzed the ALS locus for five plants of each of the three waterhemp populations. Broad cross-resistance to imidazolinone and sulfonylurea herbicides is conferred by the Trp-574-Leu mutation, but it was not present in the FS population. The Trp-574-Leu mutation was identified in one plant from the SCAL population and in three plants of the SE population. Using gene sequencing, we identified a Ser-653-Asn mutation that confers resistance to imidazolinone herbicides in all five FS plants that were sequenced, which provided genetic confirmation for the lack of response to imazethapyr observed in the single-dose bioassay (Figure 1). However, the FS population was less susceptible to chlorimuron-ethyl, a sulfonylurea herbicide, than the SE or SCAL populations, where the Trp-574-Leu mutation was present (Figures 4 and 5; Tables 3 and 4). We did not sequence the entire ALS gene, so it is possible that other mutations may exist or that the FS population may also metabolize sulfonylurea herbicides, as has been reported previously in waterhemp (Guo et al. Reference Guo, Riggins, Hausman, Hager, Riechers, Davis and Tranel2015).
The only mechanism reported to confer resistance to PPO-inhibiting herbicides in waterhemp is a 3-base pair deletion in the PPX2L gene, referred to as the ΔG210 mutation (Lee et al. Reference Lee, Hager and Tranel2008; Patzoldt et al. Reference Patzoldt, Hager, McCormick and Tranel2006; Shoup et al. Reference Shoup, Al-Khatib and Peterson2003; Tranel et al. Reference Tranel, Riggins, Bell and Hager2011). Despite more than 50% of the plants from all populations surviving lactofen in the single-dose bioassay, none of the plants contained the deletion. PPO resistance has not been reported in any waterhemp populations in Nebraska. Because all of the plants were severely injured immediately following the application of lactofen (unpublished data), and all three populations responded similarly to the treatment in both runs, it is unlikely that the FS population is resistant to PPO-inhibiting herbicides.
The FS waterhemp population first reported by Bernards et al. (Reference Bernards, Crespo, Kruger, Gaussoin and Tranel2012) is also resistant to ALS-inhibiting herbicides and to the PSII-inhibiting herbicide atrazine. Resistance to ALS-inhibiting herbicides was confirmed by the presence of at least one mutation known to confer resistance. Resistance to atrazine is likely due to a non–target site mechanism because mutations conferring target-site resistance to atrazine were not present and the population was susceptible to ametryn but showed no response to atrazine. Two other Nebraska waterhemp populations, SE and SCAL, also contained mutations conferring resistance to ALS-inhibiting herbicides and responded to atrazine and ametryn similarly to the FS population. The FS population was less susceptible to the TIR1 auxin receptor herbicides aminopyralid and picloram than the two other waterhemp populations. All three populations were susceptible to lactofen, mesotrione, glufosinate, and glyphosate. The field where the FS population evolved was planted to a perennial crop in 1996 that was mowed each fall and burned each spring through 2011. In addition, it received an annual spring application of a triple mechanism of action herbicide tank mix (S-metolachlor, atrazine, and 2,4-D) followed by an annual application of 2,4-D. In short, resistance evolved even where there was diversity in cultural tactics and herbicide mechanisms of action. Resistance to ALS-inhibiting herbicides and atrazine may have been present in the population prior to the little bluestem being established, based on when resistance to those herbicides was first documented in the midwestern United States. This example emphasizes the need for weed managers to prevent seeds returning to the soil, in addition to using diverse cultural tactics and mixtures of effective herbicides.
Acknowledgments
We thank the Department of Agronomy and Horticulture of the University of Nebraska–Lincoln for funding this research.