Introduction
Annual sowthistle (Sonchus oleraceus L.) is one of the most troublesome weeds in terms of revenue loss in Australia (AU$4.9 million) (Llewellyn et al. Reference Llewellyn, Ronning, Clarke, Mayfield, Walker and Ouzman2016). It is estimated that the presence of 43 to 52 S. oleraceus plants m−2 could reduce wheat (Triticum aestivum L.) yield by 50% (Manalil et al. Reference Manalil, Ali and Chauhan2020). Abundant seed production, no to very low seed dormancy, and tolerance to a broad range of environmental conditions are factors that contribute to S. oleraceus emergence throughout the year (summer and winter) in many cropping systems (Chauhan et al. Reference Chauhan, Gill and Preston2006; Manalil et al. Reference Manalil, Ali and Chauhan2018; Widderick et al. Reference Widderick, Walker, Sindel and Bell2010). In the 1990s, the first case of a S. oleraceus biotype resistant to chlorsulfuron (acetolactate synthase [ALS] inhibitor) was reported from Queensland. Sonchus oleraceus biotypes resistant to glyphosate (5-enolpyruvylshikimate-3-phosphate synthase inhibitor) and 2,4-D (synthetic auxin) have also been reported in Australia in the last decade (Heap Reference Heap2020). Low seed retention (95% wind dispersal), resistance to several herbicides, and the high competitiveness of this weed have reduced the effectiveness of existing weed control practices, making S. oleraceus a challenging issue in Australian agricultural systems (Manalil et al. Reference Manalil, Ali and Chauhan2020; Mobli et al. Reference Mobli, Matloob and Chauhan2019).
Glyphosate is a nonselective herbicide that disrupts the synthesis of aromatic amino acids (phenylalanine, tyrosine, and tryptophan) in the shikimic acid pathway (Giesy et al. Reference Giesy, Dobson, Solomon and Ware2000; Steinrücken and Amrhein Reference Steinrücken and Amrhein1980). The current uses of this herbicide include selective POST control of weeds in glyphosate-tolerant crops, preharvest desiccation of cereals, and nonselective weed control in both croplands and non-croplands (Cederlund Reference Cederlund2017). In conservation agriculture systems, glyphosate is frequently applied during seedbed preparation (fallow phase). The use of this herbicide expanded following the introduction of glyphosate-tolerant crops (Aune Reference Aune and Lichtfouse2012; Chauhan et al. Reference Chauhan, Singh and Mahajan2012). Although glyphosate is a foliar POST herbicide, the basipetal and acropetal movements of this herbicide in the plant’s body allows its translocation from the roots to the aboveground tissues (Petersen et al. Reference Petersen, Hansen, Ravn, Sørensen and Sørensen2007). In soil, glyphosate is tightly bound to clay and organic particles, but in the presence of phosphate, immobile glyphosate is remobilized due to the competition of phosphate for the glyphosate binding site (Borggaard and Gimsing Reference Borggaard and Gimsing2008; Clua et al. Reference Clua, Conti and Beltrano2012).
Hormesis refers to the stimulatory effect from low doses of an otherwise toxic substance. Herbicides are important tool to combat weed peril in contemporary agriculture, and both target and non-target plant species are frequently exposed to sublethal doses of herbicides under field conditions. Drift, incorrect sprayer calibration, herbicide interception by barriers (plants and plant residues), and dilution during herbicide retention reduce the herbicide dose that reaches the target (Velini et al. Reference Velini, Trindade, Barberis and Duke2010). Drift studies showed that plants may receive 0.1% to 0.01% of the recommended rate during herbicide application (Al-Khatib and Peterson Reference Al-Khatib and Peterson1999; Wolf et al. Reference Wolf, Grover, Wallace, Shewchuk and Maybank1993). Rainfall and dew after application can also dilute the herbicide concentration (Nadeem et al. Reference Nadeem, Abbas, Tanveer, Maqbool, Zohaib and Shehzad2017). Hormetic effects of several herbicides have been demonstrated on crops (Silva et al. Reference Silva, Duke, Dayan and Velini2016) and weeds (Nadeem et al. Reference Nadeem, Abbas, Tanveer, Maqbool, Zohaib and Shehzad2017). Nevertheless, such herbicide-induced hormesis is most extensively studied for glyphosate (Belz and Duke Reference Belz and Duke2014), as it is the most commonly used herbicide worldwide (Duke and Powles Reference Duke and Powles2008). Glyphosate runoff and drift have been acknowledged as major risk factors to non-target plants outside the intended area of application (Cederlund Reference Cederlund2017; Saunders and Pezeshki Reference Saunders and Pezeshki2015). Glyphosate drift–related effects are complex and manifest either as impaired growth and reproduction or stimulation of these aspects (Brito et al. Reference Brito, Tropaldi, Carbonari and Velini2018). Herbicide doses lower than the recommended rate may cause toxicity to plants but may not control (kill) them. For example, Kurtz and Street (Reference Kurtz and Street2003) reported that glyphosate doses of more than 70 g ae ha−1 caused injury to rice (Oryza sativa L.). Although some sublethal doses are toxic to plants, some may have a stimulatory effect (hormetic effect) on plant growth. The hormetic effect depends on the herbicide mode of action and formulation, plant species, growth stage, and environmental conditions prevailing during and directly following herbicide application (Roider et al. Reference Roider, Griffin, Harrison and Jones2007). In addition to non-target species being affected by glyphosate spray drifting from treated fields, weeds growing under genetically modified glyphosate-resistant crops could be hormetically enhanced if they receive potentially growth-stimulating doses of glyphosate (Cedergreen 2008a, Reference Cedergreen2008b).
In weed populations, herbicide-induced hormetic effects are manifested as stimulation of growth and seed production. Nadeem et al. (Reference Nadeem, Abbas, Tanveer, Maqbool, Zohaib and Shehzad2017) observed vigorous growth of common lambsquarters (Chenopodium album L.), swine cress [Coronopus didymus (L.) Sm.], yellow vetch (Lathyrus aphaca L.), and toothed dock (Rumex dentatus L.) as a result of foliar glyphosate application at 4 to 32 g ha−1. At the same doses, seed production of these weeds was increased by 25% to 60%. Asman et al. (Reference Asman, Jørgensen and Jensen2003) showed that glyphosate drift exposes weeds growing at field edges to 1% to 10% (equivalent to 5 to 60 g ha−1) of recommended application rates under field conditions. Theses doses can cause hormesis, because they lie within the stimulatory range. Greenhouse and field studies have suggested a yield increase of 12% to 175% in plants like barley (Hordeum vulgare L.), maize (Zea mays L.), soybean [Glycine max (L.) Merr.], eucalyptus (Eucalyptus grandis W. Hill ex Maid.), and pine (Pinus caribaea Morelet) when glyphosate was applied at 2 to 143 g ha−1 (Cedergreen et al. Reference Cedergreen, Felby, Porter and Streibig2009; Velini et al. Reference Velini, Alves, Godoy, Mechede, Souzaand and Duke2008). Lower glyphosate doses corresponding to 5% to 10% of field application rates stimulated barley seedling biomass by 25% (Cedergreen Reference Cedergreen2008a). Furthermore, one report showed that 17% of average cropping land in Australia has been infested by glyphosate-resistant weed populations (Llewellyn et al. Reference Llewellyn, Ronning, Clarke, Mayfield, Walker and Ouzman2016). Glyphosate-induced hormesis may play a role in the evolution of resistant biotypes by enhancing reproductive fitness (more flower and seed production) of the treated plants. The hormesis may not directly cause the selection pressure for evolution of resistance. However, the growth-promoting potential of glyphosate at low doses may indirectly favor development of herbicide resistance by rendering the enhanced biotypes more vigorous (more biomass accumulation, increased leaf area), competitive, and reproductive (Belz Reference Belz2014; Belz et al. Reference Belz, Cedergreen and Duke2011; Cedergreen Reference Cedergreen2008b). Hence, understanding the effects of sublethal doses of glyphosate on S. oleraceus is crucial due to the extensive use of glyphosate and the potential exposure of weeds to lower glyphosate doses.
There is limited research on the effects of sublethal doses of glyphosate on weeds as a major threat to productivity of agriculture systems. Therefore, the objective of the present study was to evaluate the effect of sublethal doses of glyphosate on the growth and seed production of S. oleraceus, particularly considering the role of hormesis.
Materials and Methods
Study Details
In 2016, seeds of S. oleraceus were collected from the research fields at the Gatton Campus of the University of Queensland, Australia (27.33°S, 152.16°E; altitude 94 m above sea level). A screenhouse pot experiment was conducted to evaluate the effect of low doses of glyphosate on S. oleraceus in 2017. A commercial potting mix (Scotts Australia, Bella Vista, NSW, Australia) was used in this study. Plastic pots (12 L) were filled with potting mix and placed in a screenhouse facility of the Gatton Campus of the University of Queensland. Sonchus oleraceus seeds (4 to 5) were directly sown into the center of pots, and only one healthy emerged seedling was kept per pot. At the 4- to 5-leaf stage (3-wk-old rosette), the individual plants were sprayed with glyphosate using a research track sprayer (spray volume 108 L ha−1) at 5, 10, 20, 40, 80, and 800 g ha−1. The effects of glyphosate sublethal doses were compared with a nontreated control. Pot soil moisture was kept near field capacity using sprinkler irrigation twice a day. Irrigation was stopped 1 d before spraying, and again pots were irrigated 1 d after glyphosate treatment. No fertilizer was applied during the study. The study was repeated again following the completion of the first experimental run at the same location, with inclusion of an extra glyphosate dose (2.5 g ha−1). The mean, minimum, and maximum temperatures during the course of these studies are presented in Figure 1. Height and number of leaves of S. oleraceus were recorded at 14-d intervals from each glyphosate treatment till 56 d after treatment (DAT). The number of seeds per plant was determined by counting the number of seeds from 15 buds. The average value was then multiplied by the total number of buds per plant to determine average seed production per plant. At 56 DAT, the shoots and roots were separated, and the dry biomass was recorded after being placed in an oven set at 70 C for 72 h.
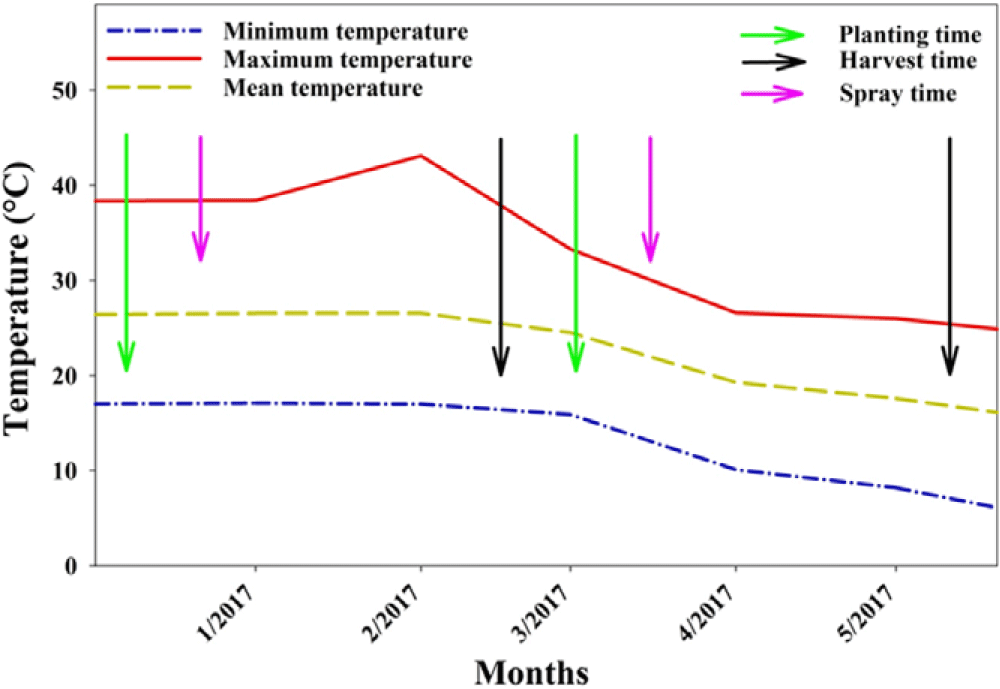
Figure 1. Mean, minimum, and maximum temperatures during the studies conducted on the hormetic effect of glyphosate on Sonchus oleraceus in a naturally ventilated screenhouse at the Gatton Campus of the University of Queensland, Australia.
Statistical Analysis
These studies were conducted in a randomized complete block design with 10 replications. Before statistical analyses, the homogeneity and normality of data were checked, and an ANOVA was performed using SAS software v. 9 (SAS Institute, Cary, NC, USA). Because data distribution was normal (Shapiro-Wilk test), original data were used for statistical analyses. Due to significant differences between two experimental runs, data of each run are presented separately. The Fisher’s protected LSD mean comparison test at 5% probability was used for data pertaining S. oleraceus biomass and seed production. A three-parameter sigmoid model was used to describe the effect of glyphosate doses on plant height and number of leaves during the period of the study with the following equation:

where X is height or number of leaves per plant at days after spray (T), X max is the maximum plant height or number of leaves at time T; T 50 is the number of days needed to reach 50% height or number of leaves, and b is the slope. Nonlinear regression was performed using SigmaPlot v. 14 (Systat Software, San Jose, CA, USA), and predicted values were compared using the standard error of the mean. Graphical representation of the data was also carried out using the same software. The fitness of the fitted models was ascertained in terms of root mean-square error (RMSE).

where P i is the predicted value, Oi is the observed value, and n is the total number of observations. As an absolute measure of fit, RMSE explains that how close the observed data points are to the model’s predicted values. Smaller RMSE value means better fit to the model due to closer observed and predicted values.
Results and Discussion
Plant Height
For both experimental runs, a three-parameter sigmoid model explained (P < 0.001) incremental differences in plant height of S. oleraceus at 56 DAT (Figure 2; Table 1). During both experimental runs, no S. oleraceus plants survived at the 800 g ha−1 glyphosate dose. The effect of the glyphosate doses on plant height was more pronounced after 14 DAT. The stimulatory effect on S. oleraceus plant height was more evident in response to 5 g ha−1 of glyphosate than the other treatments, as the height was increased by 20% and 35% in the first and second experimental runs, respectively, when compared with the no-glyphosate treatment at 56 DAT (Figures 2 and 3). The maximum plant height estimated by the model was 110.5 and 120.6 cm at a glyphosate dose of 5 g ha−1, which was reduced to 701.1 and 72.3 cm at 80 g ha−1. Application of glyphosate at 40 and 80 g ha−1 reduced height of S. oleraceus plants by 12% and 31% in the first experimental run and 31% and 27% in the second experimental run, respectively, when compared with the no-glyphosate treatment. At 5 and 10 g ha−1 glyphosate, the days required to attain 50% height (T 50) were lower than for other treatments in both experimental runs (Table 1).

Figure 2. A three-parameter sigmoid model describes the effect of low doses of glyphosate on Sonchus oleraceus height in the first and second experimental runs. Vertical bars represent standard errors (±SE) of means. Estimated parameters are presented in Table 1.
Table 1. Estimated parameters (± SE) of a three-parameter sigmoid model fit to height data for Sonchus oleraceus in the first and second experimental runs.

a X max is the maximum height at time T; T 50 is the days needed to reach a 50% height, and b is the slope. RMSE, root mean-square error.

Figure 3. Effect of low doses of glyphosate on Sonchus oleraceus plants in the second experimental run at 42 DAT.
Number of Leaves
The number of leaves of S. oleraceus was significantly (P < 0.001) affected by glyphosate doses during both experimental runs (Figure 4; Table 2). The effect of applied glyphosate doses was more pronounced after 14 DAT. At 56 DAT, the application of 5 g ha−1 of glyphosate resulted in 53% and 74% increase in number of leaves of S. oleraceus for the first and second experimental run, respectively, when compared with the no-glyphosate treatment. Application of glyphosate at 80 g ha−1 reduced the estimated maximum number of leaves per plant by 15% and 28% during first and second experimental run, respectively, compared with the no-glyphosate treatment. The prediction of the three-parameter sigmoid model for the days required to reach 50% leaf production did not show a particular trend (Table 2).

Figure 4. A three-parameter sigmoid model describes the effect of low doses of glyphosate on Sonchus oleraceus leaf count in the first and second experimental runs. Vertical bars represent standard errors (±SE) of means. Estimated parameters are presented in Table 1.
Table 2. Estimated parameters (± SE) of a three-parameter sigmoid model fit to leaf count data for Sonchus oleraceus in the first and second experimental runs.

a X max is the maximum number of leaves at time T; T 50 is the days needed to reach a 50% number of leaves, and b is the slope. RMSE, root mean-square error.
Shoot and Root Biomass
Shoot and root biomass of S. oleraceus was significantly (P < 0.001) affected by glyphosate dose (Figure 5). For both experimental runs, the highest shoot and root biomass was observed for S. oleraceus seedlings sprayed with 5 g ha−1 of glyphosate. This treatment resulted in 168% and 35% higher shoot biomass of S. oleraceus during the first and second experimental runs, respectively, compared with the no-glyphosate treatment. Similarly, the percent increase for root biomass was 84% and 41% in the first and second experimental runs, respectively, in comparison with the no-glyphosate treatment. In the first experimental run, except for 5 g ha−1 glyphosate treatment, no significant differences were observed between the shoot and root biomass at different glyphosate doses and the no-glyphosate treatment. However, in the second experimental run, 80 g ha−1 glyphosate application reduced the shoot and root biomass by 43% and 19%, respectively, compared with the no-glyphosate treatment (Figure 5).

Figure 5. Effect of low doses of glyphosate on Sonchus oleraceus shoot and root biomass in the first and second experimental runs. Vertical bars represent LSD at 5% probability, and letters above bars show group differences between means.
Buds and Seed Production
Bud and seed production of S. oleraceus was significantly (P < 0.001) affected by glyphosate doses (Figure 6). For both experimental runs, the highest numbers of buds and seeds per plant of S. oleraceus were observed in response to 5 g ha−1 glyphosate. Compared with the no-glyphosate treatment, application of 5 g ha−1 glyphosate enhanced the number of buds and seeds of this weed by 134% and 154% in the first run. The corresponding increase was 129% and 101% in the second experimental run. No significant differences were observed between the number of buds at 80 g ha−1 glyphosate and the no-glyphosate treatment. However, the seeds per plant were reduced by 33% and 46% in the first and second experimental runs, respectively, in comparison with the no-glyphosate treatment.
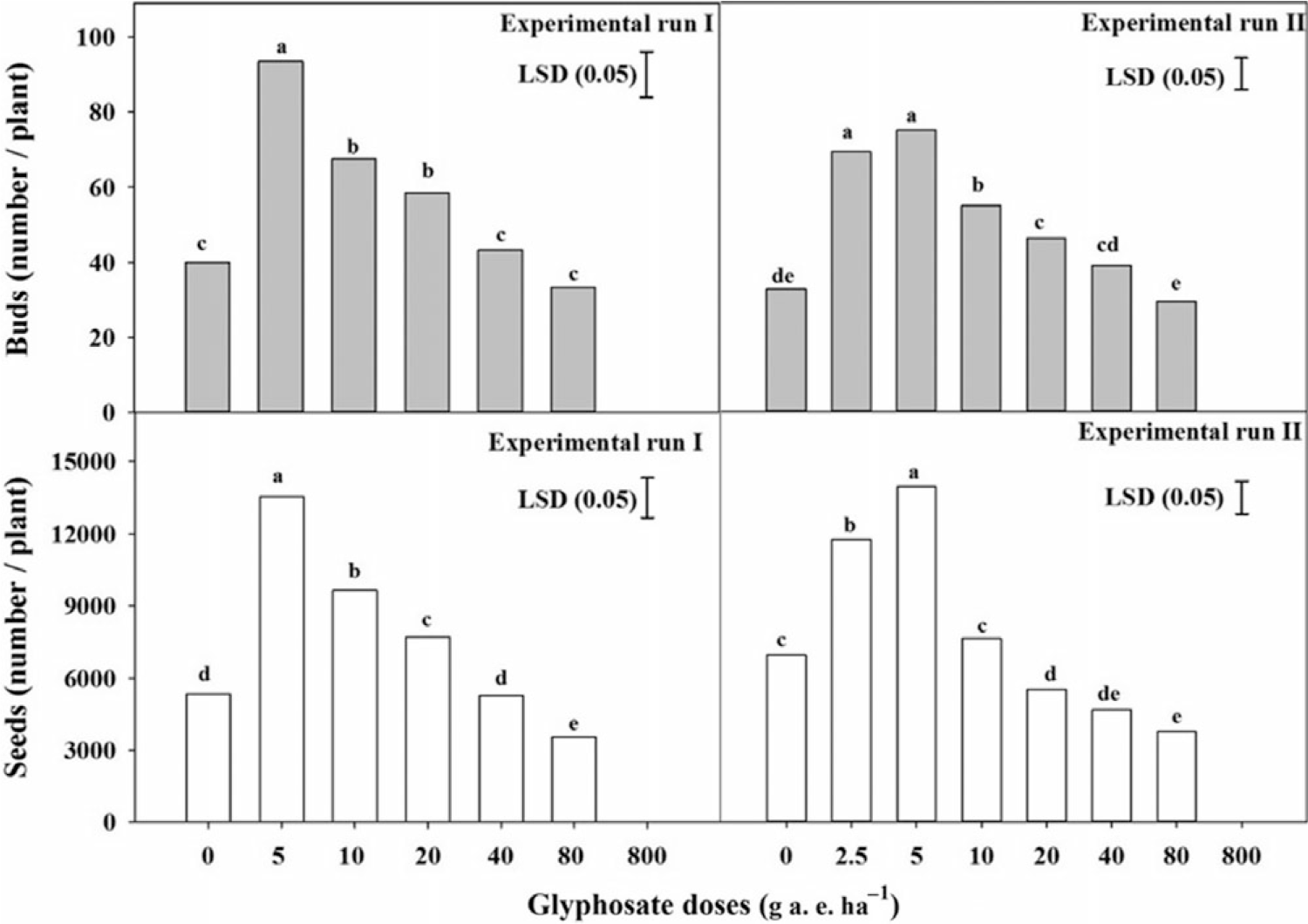
Figure 6. Effect of low doses of glyphosate on Sonchus oleraceus buds and seed production in the first and second experimental runs. Vertical bars represent LSD at 5% probability, and letters above bars show group differences between means.
The present study showed that the hormetic effects from low doses of glyphosate on S. oleraceus were manifested as stimulation of seedling growth and reproductive potential. All of the observed parameters (i.e., plant height, leaf number, shoot and dry root biomass, buds and seed production) responded positively to low doses of glyphosate. Thus, the notion that glyphosate can induce growth stimulation is primarily due to the trade-off between the studied traits (Cedergreen Reference Cedergreen2008b). Because root and shoot dry biomass of S. oleraceus was improved over the nontreated control, preferential biomass allocation in either aerial or underground parts was not the cause of the S. oleraceus root and shoot dry biomass improvement. The RMSE values for plant height and number of leaves showed a small difference between observed and predicted values indicating goodness of fit.
The duration of hormetic growth stimulation is a critical aspect to be considered, especially when the glyphosate-induced hormesis is transitory and is not sustained over time (Cedergreen Reference Cedergreen2008a). The data from the present study showed that hormetic effects of glyphosate on S. oleraceus growth were sustained over time, starting from 14 DAT till 56 DAT (harvest time). While plant height and leaf number manifested increases over time, such increases were always greater for plants sprayed with lower doses of glyphosate for the 56-d period. Cedergreen (Reference Cedergreen2008a) observed that the hormetic effect disappeared 7 wk after glyphosate application in barley, while the hormetic effect did not disappear in our study. Nadeem et al (Reference Nadeem, Abbas, Tanveer, Maqbool, Zohaib and Shehzad2017) reported that treatments of 4-wk-old seedlings of C. didymus, C. album, R. dentatus, and L. aphaca with glyphosate at 32 g ha−1 and lower increased root and shoot length. The same weeds treated with glyphosate at 64 g ha−1 recorded significant growth inhibition (Nadeem et al. Reference Nadeem, Abbas, Tanveer, Maqbool, Zohaib and Shehzad2017). The mechanism of increased photosynthesis or reduced respiration at lower doses of glyphosate is not fully understood (Cedergreen and Olesen Reference Cedergreen and Olesen2010; Nascentes et al. Reference Nascentes, Carbonari, Simões, Brunelli, Velini and Duke2018). Taller plants with more leaves could be attributed to activated phloem-loading proteins as a consequence of increased sucrose concentrations through the decreased activity of acid invertase (Ozturk et al. Reference Ozturk, Yazici, Eker, Gokmen, Römheld and Cakmak2008; Roitsch et al. Reference Roitsch, Balibrea, Hofmann, Proels and Sinha2003; Su et al. Reference Su, Cruz, Moore and Maretzki1992).
The observed differences between experimental runs could be attributed to differences in temperature during the study, as the other conditions were consistent for both experimental runs. Temperature has a vital role in plant metabolism, as well as having possible effects on hormesis, whereby suboptimal temperatures can reduce the growth stimulatory effects (Belz and Cedergreen Reference Belz and Cedergreen2010; Belz and Duke Reference Belz and Duke2014). Although the rate of biomass increase was different between the experimental runs, the overall biomass showed a significant increase at low glyphosate rates. It was hypothesized that the stimulatory effect on root growth may create a larger carbohydrate sink and result in higher biomass production (Cedergreen Reference Cedergreen2008a). Cedergreen and Olesen (Reference Cedergreen and Olesen2010) noted that increased biomass may be related to alterations in the source–sink relationship.
Sonchus oleraceus grows throughout the year in both fallow and cropped fields. In Australia, glyphosate-tolerant cotton (Gossypium hirsutum L.) and canola (Brassica napus L.) are grown during summer and winter seasons, respectively. Sonchus oleraceus growing under the canopy of these crops is expected to receive reduced, and potentially growth-stimulating, doses of glyphosate. This creates implications for the competitive balance between this weed and its associated crops. The findings of our study imply that hormesis caused by low glyphosate doses may be a crucial factor in stimulating the growth of non-target species. These species could receive such sublethal doses as herbicide drift application from herbicide-treated crop fields, and this may result in a competitive imbalance between crop and hormetically enhanced weed species. Moreover, the success of weed management practices will also be compromised when there is a greater biomass accumulation and therefore a higher seed output by S. oleraceus, rendering the main crop less competitive.
Frequent and inevitable foliar exposure of weeds to sublethal doses of herbicides could have a great impact on weed populations (Belz and Duke Reference Belz and Duke2014; De Moraes et al. Reference De Moraes, de Brito, Tropaldi, Carbonari and Velini2020; Saunders and Pezeshki Reference Saunders and Pezeshki2015). Silva et al. (Reference Silva, Duke, Dayan and Velini2016) showed that soybeans treated with sublethal doses of glyphosate had differential hormetic responses to further glyphosate exposure. Frequent exposure to subtoxic doses of herbicide may also contribute to development of herbicide-resistant weed populations (Belz Reference Belz2014; Cedergreen Reference Cedergreen2008b). A weed that is hormetically boosted has an increased chance to become more competitive than weeds that are not affected or adversely affected by glyphosate (Cedergreen Reference Cedergreen2008a, Reference Cedergreen2008b). This aspect is relevant from an agroecosystem perspective, where low glyphosate doses will appear in practice from spray drift, faultiness in herbicide application, surface contact of treated and nontreated plants, or shading by taller plants. Under such scenarios, changes in weed species composition, and hence herbicide susceptibility, are expected (Belz and Duke Reference Belz, Duke, Duke, Kudsk and Solomon2017; Cedergreen 2008a, Reference Cedergreen2008b). Growth stimulation caused by ultra-low herbicide doses has recently been demonstrated as an important factor conducive to herbicide-resistance development, and hormetically boosted resistant biotypes are expected to be more competitive (Farooq et al. Reference Farooq, Abbas, Tanveer, Javaid, Ali, Safdar, Khan, Zohaib and Shazad2019). Differential hormetic responses were exhibited by fenoxaprop p-ethyl resistant and susceptible populations of canary grass (Phalaris minor Retz.). The upper limit of stimulatory hormetic dose (16% to 24% of the recommended application rate) for the resistant population was inhibitory for the susceptible population (Farooq et al. Reference Farooq, Abbas, Tanveer, Javaid, Ali, Safdar, Khan, Zohaib and Shazad2019). Likewise, another study found a 54% increase in biomass of an acetyl-CoA carboxylase target-site resistant (TSR) biotype of blackgrass (Alopecurus myosuroides Huds.) compared with the control at doses that caused phytotoxicity to the susceptible biotype (Belz et al. Reference Belz, Cedergreen and Duke2011, Reference Belz, Farooq and Wagner2018; Petersen et al. Reference Petersen, Neser and Dresbach-Runkel2008). Other studies also documented 31% and 64% growth stimulation of ALS TSR biotypes of scentless false mayweed [Tripleurospermum perforatum (Mérat) M. Lainz] and perennial ryegrass (Lolium perenne L.) in response to ALS-inihibiting herbicides (Belz Reference Belz2014; Menegat et al. Reference Menegat, Bailly, Aponte, Heinrich, Sievernich and Gerhards2016).
At 5 g ha−1 glyphosate, compared with the no-glyphosate treatment, S. oleraceus seed production increased by 154% and 101% in the first and second experimental runs, respectively. Under normal conditions, S. oleraceus can produce more than 25,000 seeds per plant (Chauhan et al. Reference Chauhan, Gill and Preston2006). The gradual flowering of buds and low seed retention (95% wind dispersal) of S. oleraceus can reduce the efficacy of control strategies that involve harvesting weed seed (Manalil et al. Reference Chauhan, Gill and Preston2020). Furthermore, it seems that the vigorous growth of plants as a result of the hormetic effect increases their competitive ability (Nadeem et al. Reference Nadeem, Abbas, Tanveer, Maqbool, Zohaib and Shehzad2017). It could be concluded that exposure to low doses of glyphosate may result in higher weed–crop competition and lower crop success with conventional and unconventional weed control strategies. Previous screenhouse and field interference studies on S. oleraceus showed that the implementation of a single weed management strategy on such a highly competitive weed could be insufficient and might require the integration of a diverse range of weed control strategies (Manalil et al. Reference Manalil, Ali and Chauhan2020; Mobli et al. Reference Mobli, Matloob and Chauhan2019).
In the present study, glyphosate-induced hormesis was observed for almost all studied traits. The hormetic effect persisted until 56 DAT, and it also enhanced the reproductive potential of S. oleraceus. It could be concluded that the exposure to low doses of glyphosate may have a great impact on the growth and reproductive behavior of S. oleraceus. This impact could alter its ecological niche, beside posing challenges of resistance evolution due to increased selection and survival if increased fitness is expressed as enhanced reproductive potential. Spraying practices must be developed to avoid hormetic responses in non-target species. Delay in the development of herbicide-resistant populations is a major task in weed management strategies. Therefore, to avoid new challenges in the agriculture industry, more attention to integrated weed management with more emphasis on nonchemical and biological methods is required. For a better understanding of glyphosate-induced hormesis, the interaction of sublethal doses with other influencing factors must also be explored. Future studies should focus on whether the glyphosate-induced hormetic enhancements are similar between glyphosate-resistant and glyphosate-susceptible biotypes of S. oleraceus. Another relevant area for research would be to ascertain the consistency of sublethal doses that induce hormesis for glyphosate-resistant and glyphosate-susceptible populations under lab and field conditions. Influence of biotic and abiotic factors on glyphosate hormesis also needs to be studied to establish a better understanding of the mechanisms of hormesis.