Introduction
Double fertilization in angiosperms leads not only to the commencement of embryogenesis and development of the nutritive triploid endosperm, but also the differentiation of the surrounding ovule integuments to become the mature seed coat. Changes to the coat include specializations to enable protection of the embryo as well as to facilitate dispersal and eventual germination. These can include mechanical reinforcement through the synthesis of secondary cell walls that may be impregnated with impermeable polymers such as lignin or suberin, or synthesis of secondary metabolites often visible as pigments (e.g. polyphenolics) that may aid in protection from pathogens. Another common specialization is the deposition of a hydrophilic, pectinaceous mucilage in the seed coat epidermis or surrounding pericarp developed from ovary tissue (i.e. fruit) (Grubert, Reference Grubert1974; Fahn, Reference Fahn1982; Boesewinkel and Bouman, Reference Boesewinkel, Bouman and Johri1984). The production of seed mucilage, known as myxospermy, occurs in a broad range of plants, from the Acanthaceae to the Brassicaceae to the Linaceae to the Plantaginaceae, while myxocarpy (fruit mucilage) occurs in families such as the Asteraceae, Lamiaceae and Poaceae (Grubert, Reference Grubert1974; Fahn, Reference Fahn1979; Grubert, Reference Grubert1981; Ryding, Reference Ryding2001; Kreitschitz, Reference Kreitschitz and Gorb2009). Mucilage is deposited into the apoplast of epidermal cells during differentiation of the seed coat or pericarp, and is released in response to seed hydration to form a water-containing, gel-like capsule surrounding the seed. Myxodiaspory (mucilage production by the seed dispersal unit, i.e. seed coat or pericarp) has been proposed to play a number of roles, including facilitation of seed hydration, regulation of germination by affecting oxygen entry into the seed, and mediation of seed dispersal through adhesion to soil or animal vectors (Grubert, Reference Grubert1974; Fahn, Reference Fahn1982; Ryding, Reference Ryding2001; Kreitschitz, Reference Kreitschitz and Gorb2009).
In this review, I will focus on the structure and synthesis of seed and pericarp mucilages, and their roles in seed hydration, germination and dispersal. Further, I will attempt to integrate the recent detailed work on seed coat mucilage of the genetic model plant, Arabidopsis thaliana (Arabidopsis; Brassicaceae) with literature on other species. For simplicity, throughout this review, I will refer generally to both seed epidermis-derived and pericarp exocarp-derived mucilage as seed coat mucilage; species that are myxocarpous are noted in Table 1 and will be mentioned in the text as necessary.
Table 1 Partial survey of myxodiaspory in angiosperms, including role in germination and dispersal

aNotable characteristics if available; bP = mucilaginous pericarp; cn.a. = not available; dSee Fig. 1; ePericarp of nutlets; fAnthocarps; gSee Grubert (Reference Grubert1981) for comprehensive list of species with mucilage. References: (1) Witztum et al. (Reference Witztum, Gutterman and Evenari1969); (2) Gutterman et al. (Reference Gutterman, Witztum and Heydecker1973); (3) Schnepf and Deichgräber (Reference Schnepf and Deichgräber1983b); (4) Grubert (Reference Grubert1974); (5) Heydecker and Orphanos (Reference Heydecker and Orphanos1968); (6) Huang et al. (Reference Huang, Boubriak, Osborne, Dong and Gutterman2008); (7) Yang et al. (Reference Yang, Dong and Huang2010); (8) Kreitschitz and Vàlles (Reference Kreitschitz and Vàlles2007); (9) Mott (Reference Mott1974); (10) Inceer (Reference Inceer2011); (11) Garwood (Reference Garwood1985); (12) Goto (Reference Goto1985); (13) Beeckman et al. (Reference Beeckman, De Rycke, Viane and Inzé2000); (14) Western et al. (Reference Western, Skinner and Haughn2000); (15) Windsor et al. (Reference Windsor, Symonds, Mendenhall and Lloyd2000); (16) Penfield et al. (Reference Penfield, Meissner, Shoue, Carpita and Bevan2001); (17) Willats et al. (Reference Willats, McCartney and Knox2001); (18) Western et al. (Reference Western, Young, Dean, Tan, Samuels and Haughn2004); (19) Macquet et al. (Reference Macquet, Ralet, Kronenberger, Marion-Poll and North2007a); (20) McFarlane et al. (Reference McFarlane, Young, Wasteneys and Samuels2008); (21) Young et al. (Reference Young, McFarlane, Hahn, Western, Haughn and Samuels2008); (22) Rautengarten et al. (Reference Rautengarten, Usadel, Neumetzler, Hartmann, Büssis and Altmann2008); (23) Arsovski et al. (Reference Arsovski, Popma, Haughn, Carpita, McCann and Western2009a); (24) Arsovski et al. (Reference Arsovski, Villota, Rowland, Subramaniam and Western2009b); (25) Panikashvili et al. (Reference Panikashvili, Shi, Schreiber and Aharoni2009); (26) Vose (Reference Vose1974); (27) Van Caeseele et al. (Reference Van Caeseele, Mills, Sumner and Gillespie1981); (28) Van Caeseele et al. (Reference Van Caeseele, Kovacs and Gillespie1987); (29) Eskin (Reference Eskin1992); (30) Kraemer (Reference Kraemer1898); (31) Hirst et al. (Reference Hirst, Rees and Richardson1965); (32) Bouman (Reference Bouman1975); (33) Woods and Downey (Reference Woods and Downey1980); (34) Fahn (Reference Fahn1982); (35) Siddiqui et al. (Reference Siddiqui, Yiu, Jones and Kalab1986); (36) Cui et al. (Reference Cui, Eskin and Biliaderis1993); (37) Cui et al. (Reference Cui, Eskin and Biliaderis1994a); (38) Wu et al. (Reference Wu, Cui, Eskin and Goff2009); (39) Vaughan and Whitehouse (Reference Vaughan and Whitehouse1971); (40) Buth and Ara (Reference Buth and Ara1981); (41) Zeng et al. (Reference Zeng, Wang, Liu and Wu2004); (42) Young et al. (Reference Young, Evans, Gifford and Eckert1970); (43) Iglesias-Fernández et al. (Reference Iglesias-Fernández, Matilla, Pulgar and de la Torre2007); (44) Mühlethaler (Reference Mühlethaler1950); (45) Müller et al. (Reference Müller, Tintelnot and Leubner-Metzger2006); (46) Gutterman and Shem-Tov (Reference Gutterman and Shem-Tov1997); (47) Lu et al. (Reference Lu, Tan, Baskin and Baskin2010); (48) Harper and Benton (Reference Harper and Benton1966); (49) Edwards (Reference Edwards1968); (50) Fitch et al. (Reference Fitch, Walck and Hidayati2007); (51) Swarbrick (Reference Swarbrick1971); (52) Young and Evans (Reference Young and Evans1973); (53) Thapliyal et al. (Reference Thapliyal, Phartyal, Baskin and Baskin2008); (54) Jordaan (Reference Jordaan2008); (55) Khan et al. (Reference Khan, Salimath, Anjaneyalu and Tharanathan1987); (56) Anjaneyalu et al. (Reference Anjaneyalu, Khan and Tharanathan1984); (57) Anjaneyalu et al. (Reference Anjaneyalu, Khan and Tharanathan1983); (58) Razavi et al. (Reference Razavi, Mortazavi, Matia-Merino, Hosseini-Parvar, Motamedzadegan and Khanipour2009); (59) Gowda (Reference Gowda1984); (60) Bushway et al. (Reference Bushway, Belyea and Bushway1981); (61) Fuller and Hay (Reference Fuller and Hay1983); (62) Duletiae-Lauševiae and Marin (Reference Duletiae-Lauševiae and Marin1998); (63) Ryding (Reference Ryding2001); (64) Anderson and Lowe (Reference Anderson and Lowe1947); (65) Boesewinkel (Reference Boesewinkel1980); (66) Muralikrishna et al. (Reference Muralikrishna, Salimath and Tharanathan1987); (67) Fedeniuk and Biliaderis (Reference Fedeniuk and Biliaderis1994); (68) Cui et al. (Reference Cui, Mazza and Biliaderis1994b); (69) Oomah et al. (Reference Oomah, Kenaschuk, Cui and Mazza1995); (70) Warrand et al. (Reference Warrand, Michaud, Picton, Muller, Courtois, Ralainirina and Courtois2003); (71) Diederichsen et al. (Reference Diederichsen, Raney and Duguid2006); (72) Naran et al. (Reference Naran, Chen and Carpita2008); (73) Panigrahi (Reference Panigrahi1986); (74) Ibrahim et al. (Reference Ibrahim, El-Eraky, El-Gengaihi and Shalaby1997); (75) Hyde (Reference Hyde1970); (76) Sharma and Koul (Reference Sharma and Koul1986); (77) Tomoda et al. (Reference Tomoda, Yokoi and Ishikawa1981); (78) Anderson and Fireman (Reference Anderson and Fireman1935); (79) Guo et al. (Reference Guo, Cui, Wang, Goff and Smith2009); (80) Kreitschitz et al. (Reference Kreitschitz, Tadele and Gola2009); (81) Hsiao and Chuang (Reference Hsiao and Chuang1981); (82) Schnepf and Deichgräber (Reference Schnepf and Deichgräber1983a); (83) Abeysekera and Willison (Reference Abeysekera and Willison1988); (84) Hamilton et al. (Reference Hamilton, Ashmore, Drew and Pritchard2007); (85) Srinivas et al. (Reference Srinivas, Joshi and Krishnan1998); (86) Lobova et al. (Reference Lobova, Mori, Blanchard, Peckham and Charles-Dominique2003).
Occurrence and structure of seed mucilage
Mucilage, often referred to as slime, is a ‘catch-all’ term for polysaccharides (mainly pectins or hemicelluloses) or proteoglycans (e.g. heavily glycosylated arabinogalactan proteins) secreted by the plant during development. This is to contrast with gums, which are similar exudates, but are released after wounding and pathogenic cell wall degradation (Frey-Wyssling, Reference Frey-Wyssling1976). In addition to being present in seed coats, mucilages are also secreted from the root cap, where they are thought to lubricate root extension through the soil or to promote interactions with ions and/or microbes in the soil, and in the transmitting tract, where they provide a medium for pollen tube growth (Esau, Reference Esau1977; Fahn, Reference Fahn1982). While seed coat, root and transmitting-tract exudates are all considered to be mucilages, they have different compositions, as demonstrated for Arabidopsis seed coat versus root mucilage (Willats et al., Reference Willats, McCartney and Knox2001). Seed coat mucilages are found in a broad range of angiosperms, including the Acanthaceae, Amaranthaceae, Asteraceae, Bombacaceae, Boraginaceae, Brassicaceae, Cistaceae, Cucurbitaceae, Dilleniaceae, Euphorbiaceae, Fabaceae, Goodeniaceae, Hydrocaritaceae, Lamiaceae, Linaceae, Lythraceae, Malvaceae, Nyctaginaceae, Onograceae, Orchidaceae, Plantaginaceae, Poaceae, Polemoniaceae, Rosaceae, Rutaceae, Sapindaceae, Solanaceae, Urticaceae, Voilaceae and Zygophyllaceae (see Fig. 1 for examples and Table 1 for a complete list) (Kraemer, Reference Kraemer1898; Mühlethaler, Reference Mühlethaler1950; Harper and Benton, Reference Harper and Benton1966; Edwards, Reference Edwards1968; Witztum et al., Reference Witztum, Gutterman and Evenari1969; Young et al., Reference Young, Evans, Gifford and Eckert1970; Swarbrick, Reference Swarbrick1971; Vaughan and Whitehouse, Reference Vaughan and Whitehouse1971; Young and Evans, Reference Young and Evans1973; Grubert, Reference Grubert1974; Bouman, Reference Bouman1975; Bushway et al., Reference Bushway, Belyea and Bushway1981; Buth and Ara, Reference Buth and Ara1981; Fahn, Reference Fahn1982; Schnepf and Deichgräber, Reference Schnepf and Deichgräber1983a, b; Gowda, Reference Gowda1984; Garwood, Reference Garwood1985; Panigrahi, Reference Panigrahi1986; Sharma and Koul, Reference Sharma and Koul1986; Eskin, Reference Eskin1992; Oomah et al., Reference Oomah, Kenaschuk, Cui and Mazza1995; Ibrahim et al., Reference Ibrahim, El-Eraky, El-Gengaihi and Shalaby1997; Duletiae-Lauševiae and Marin, Reference Duletiae-Lauševiae and Marin1998; Huang and Gutterman, Reference Huang and Gutterman1999; Ryding, Reference Ryding2001; Lobova et al., Reference Lobova, Mori, Blanchard, Peckham and Charles-Dominique2003; Zeng et al., Reference Zeng, Wang, Liu and Wu2004; Diederichsen et al., Reference Diederichsen, Raney and Duguid2006; Müller et al., Reference Müller, Tintelnot and Leubner-Metzger2006; Fitch et al., Reference Fitch, Walck and Hidayati2007; Hamilton et al., Reference Hamilton, Ashmore, Drew and Pritchard2007; Iglesias-Fernández et al., Reference Iglesias-Fernández, Matilla, Pulgar and de la Torre2007; Kreitschitz and Vàlles, Reference Kreitschitz and Vàlles2007; Huang et al., Reference Huang, Boubriak, Osborne, Dong and Gutterman2008; Jordaan, Reference Jordaan2008; Thapliyal et al., Reference Thapliyal, Phartyal, Baskin and Baskin2008; Kreitschitz, Reference Kreitschitz and Gorb2009; Kreitschitz et al., Reference Kreitschitz, Tadele and Gola2009; Inceer, Reference Inceer2011). For a detailed listing of species in 100 families with seed coat mucilage as well as references up to 1981, see Grubert (Reference Grubert1974, Reference Grubert1981).

Figure 1 Mucilage staining for pectin and cellulose in various species (for a colour version of this figure see online at http://journals.cambridge.org). Hydrated seeds were photographed without staining, incubated with 0.1% w/v ruthenium red to stain pectin (Western et al., Reference Western, Skinner and Haughn2000) or with 0.1% w/v methylene blue to stain glycans (primarily cellulose) (Kreitschitz and Vàlles, Reference Kreitschitz and Vàlles2007). Species are Arabidopsis thaliana, Sinapis alba, Aethionema arabicum (Brassicaceae), Ocimum basilicum, Salvia hispanica (Lamiaceae) and Linum usitatissimum (Linaceae). The same magnification was used within each species. Arabidopsis columellae are apparent without staining; ruthenium red highlights the inner, adherent mucilage layer, while methylene blue stains columellae and cellulose rays within the inner mucilage layer. Sinapis alba has a thick layer of mucilage containing cellulosic fibres. Aethionema arabicum epidermal cells elongate with hydration, but remain intact, containing both mucilage and cellulose. Ocimum basilicum has a thick layer of mucilage containing many cellulosic fibres. Salvia hispanica has copious mucilage with fine cellulosic threads. Linum usitatissimum has pectinaceous mucilage without cellulose.
The polysaccharide and acidic qualities of mucilages make them extremely hydrophilic, such that they hydrate rapidly in the presence of water, forming hydrogels or possibly even superabsorbent polymers (Frey-Wyssling, Reference Frey-Wyssling1976; Fahn, Reference Fahn1982; Zwieniecki et al., Reference Zwieniecki, Melcher and Holbrook2001; Zohuriaan-Mehr and Kabiri, Reference Zohuriaan-Mehr and Kabiri2008). This enables seed coat mucilage not only to break through the containing primary cell walls of epidermal cells to surround the seed, but also to hold water around the seed. Seed coat mucilages are often characterized by the presence of the pectin rhamnogalacturonan I (RG I), which has an alternating backbone of 1 → 2-linked rhamnose and 1 → 4-linked galacturonic acid, with varying degrees of 1 → 5-linked arabinose, 1 → 3-linked galactose and/or arabinogalactan side-chains linked to the rhamnose residues (Caffall and Mohnen, Reference Caffall and Mohnen2009). Hemicelluloses such as arabinoxylan, a 1 → 4-linked xylan backbone with arabinose substitutions (Lerouxel et al., Reference Lerouxel, Cavalier, Liepman and Keegstra2006), are also prevalent. Some of the best characterized seed coat mucilages are those of Arabidopsis (see below), yellow mustard Sinapis alba (aka Brassica hirta (Brassicaceae)), basils (Ocimum spp.; Lamiaceae), linseed flax (Linum usitatissimum; Linaceae) and plantain/psyllium (Plantago spp.; Plantaginaceae) (Table 1). While Arabidopsis mucilage is primarily composed of unbranched RG I (Goto, Reference Goto1985; Western et al., Reference Western, Skinner and Haughn2000, Reference Western, Young, Dean, Tan, Samuels and Haughn2004; Penfield et al., Reference Penfield, Meissner, Shoue, Carpita and Bevan2001), flax mucilage has branched RG I and the hemicellulose arabinoxylan (Anderson and Lowe, Reference Anderson and Lowe1947; Muralikrishna et al., Reference Muralikrishna, Salimath and Tharanathan1987; Cui et al., Reference Cui, Mazza and Biliaderis1994b; Fedeniuk and Biliaderis, Reference Fedeniuk and Biliaderis1994; Warrand et al., Reference Warrand, Michaud, Picton, Muller, Courtois, Ralainirina and Courtois2003; Naran et al., Reference Naran, Chen and Carpita2008). S. alba seed coat mucilage, like that of Arabidopsis and other characterized Brassica species, is pectinaceous and contains both RG I and homogalacturonan (HG) (Hirst et al., Reference Hirst, Rees and Richardson1965; Vose, Reference Vose1974; Woods and Downey, Reference Woods and Downey1980; Siddiqui et al., Reference Siddiqui, Yiu, Jones and Kalab1986; Van Caeseele et al., Reference Van Caeseele, Kovacs and Gillespie1987; Cui et al., Reference Cui, Eskin and Biliaderis1993, Reference Cui, Eskin and Biliaderis1994a; Wu et al., Reference Wu, Cui, Eskin and Goff2009). The basil species' seed mucilage can resemble Arabidopsis mucilage, but with arabinogalactan or galactan side-chains (Becium filamentosum and Ocimum adscendens, respectively) (Anjaneyalu et al., Reference Anjaneyalu, Khan and Tharanathan1984; Khan et al., Reference Khan, Salimath, Anjaneyalu and Tharanathan1987), or contain arabinoxylan, like flax seed mucilage (Ocimum gratissimum and Ocimum basilicum) (Anjaneyalu et al., Reference Anjaneyalu, Khan and Tharanathan1983; Khan et al., Reference Khan, Salimath, Anjaneyalu and Tharanathan1987; Razavi et al., Reference Razavi, Mortazavi, Matia-Merino, Hosseini-Parvar, Motamedzadegan and Khanipour2009). O. basilicum seed mucilage has also been suggested to contain a glucomannan (Razavi et al., Reference Razavi, Mortazavi, Matia-Merino, Hosseini-Parvar, Motamedzadegan and Khanipour2009). Plantago spp. seed mucilages are less well-defined in terms of specific polysaccharides, but appear to contain both arabinoxylan and pectin (Anderson and Fireman, Reference Anderson and Fireman1935; Tomoda et al., Reference Tomoda, Yokoi and Ishikawa1981; Sharma and Koul, Reference Sharma and Koul1986; Guo et al., Reference Guo, Cui, Wang, Goff and Smith2009).
In addition to their pectin and hemicellulose compositions, seed mucilages have been classified by the presence or absence of dispersed cellulose microfibrils, as detected through techniques such as histochemical staining, polarized light microscopy (‘Maltese-cross effect’) and electron microscopy (Mühlethaler, Reference Mühlethaler1950; Vaughan and Whitehouse, Reference Vaughan and Whitehouse1971; Frey-Wyssling, Reference Frey-Wyssling1976; Schnepf and Deichgräber, Reference Schnepf and Deichgräber1983a, b; Willats et al., Reference Willats, McCartney and Knox2001; Kreitschitz and Vàlles, Reference Kreitschitz and Vàlles2007). Cellulosic slimes have been identified in a number of species, including many of the Brassicaceae (e.g. Arabidopsis, S. alba, Lepidium sativum; for a survey, see Vaughan and Whitehouse, Reference Vaughan and Whitehouse1971), plus members of the Acanthaceae, Asteraceae, Fabaceae, Laminaceae, Polemoniaceae and Rosaceae (Fig. 1) (see Table 1 for details) (Mühlethaler, Reference Mühlethaler1950; Grubert, Reference Grubert1974; Fahn, Reference Fahn1982; Schnepf and Deichgräber, Reference Schnepf and Deichgräber1983a; Abeysekera and Willison, Reference Abeysekera and Willison1988; Kreitschitz and Vàlles, Reference Kreitschitz and Vàlles2007). Grant suggests that cellulosic mucilage is an example of colloidally dispersed cellulose that is solubilized through its interaction with pectins, and notes that S. alba seed mucilage contains 50% cellulose by weight (Grant et al., Reference Grant, McNab, Rees and Skerrett1969). The presence of cellulose has been suggested to prevent mucilage from being washed away from the seed and has led to cellulosic mucilage sometimes being classified as secondary-cell-wall-like (Fahn, Reference Fahn1982). Mucilages lacking cellulose are known as ‘true slimes’ or primary-cell-wall-like (Mühlethaler, Reference Mühlethaler1950; Fahn, Reference Fahn1982), and examples are seeds of the Linaceae, Plantaginaceae and Poaceae (Fig. 1) (Mühlethaler, Reference Mühlethaler1950; Fahn, Reference Fahn1982; Kreitschitz et al., Reference Kreitschitz, Tadele and Gola2009).
Detailed analysis of Arabidopsis seed mucilage, using a combination of chemical, histochemical and immunological methods, has revealed not only that its composition is much more complex than originally thought, but that the extruded mucilage has structural domains. Hydration of seeds in the pectin stain ruthenium red, with and without agitation, demonstrated the presence of two layers: an outer, diffuse layer that is easily extracted by shaking in water or dilute chelators, and an inner, dense layer that is strongly adherent to the seed and can only be removed with enzymatic digestion or harsh chemical treatments (Figs 1 and 2A) (Western et al., Reference Western, Skinner and Haughn2000; Macquet et al., Reference Macquet, Ralet, Kronenberger, Marion-Poll and North2007a; Huang et al., Reference Huang, Bowles, Esfandiari, Dean, Carpita and Haughn2011; Walker et al., Reference Walker, Tehseen, Doblin, Pettolino, Wilson, Bacic and Golz2011). The outer, soluble layer can be easily characterized by high pressure liquid chromatography (HPLC) or gas chromatography/mass spectrometry (GC/MS), and is primarily composed of unbranched RG I (Penfield et al., Reference Penfield, Meissner, Shoue, Carpita and Bevan2001). In contrast, the inner, adherent layer has been characterized by histochemical and antibody staining to contain a complex mixture of RG I with and without arabinan and galactan side-chains, HG with varying amounts of methyl-esterification, cellulose and the hemicellulose xyloglucan (Fig. 2B) (Willats et al., Reference Willats, McCartney and Knox2001; Western et al., Reference Western, Young, Dean, Tan, Samuels and Haughn2004; Macquet et al., Reference Macquet, Ralet, Kronenberger, Marion-Poll and North2007a; Young et al., Reference Young, McFarlane, Hahn, Western, Haughn and Samuels2008). The strong attachment of this complex polysaccharide layer to the seed correlates well with the finding that cellulose promotes mucilage adhesion to the seed (Harpaz-Saad et al., Reference Harpaz-Saad, McFarlane, Xu, Divi, Forward, Western and Kieber2011; Sullivan et al., Reference Sullivan, Ralet, Berger, Diatloff, Bischoff, Gonneau, Marion-Poll and North2011). A similar organization of mucilage into layers has been observed in flax seeds, the mucilage of which contains hemicellulose rather than cellulose (Naran et al., Reference Naran, Chen and Carpita2008).

Figure 2 Arabidopsis mucilage secretory cell (MSC) differentiation. (A) Seed staining of wild-type Arabidopsis seed in ruthenium red without shaking. Note the outer, cloudy layer of mucilage as well as the denser, adherent layer directly surrounding the seed. (B) Whole-seed immunofluorescence staining of wild-type Arabidopsis seed with the anti-RG I antibody CCRC-M36. Staining of the mucilage capsule surrounding the seed represents the RG I in the inner, adherent mucilage layer. The seed surface is counterstained with propidium iodide, revealing the hexagonal-shaped MSCs with their central columellae. (C, D) Sections of resin-embedded developing seeds stained with toluidine blue. (C) A seed 7 days post-anthesis (DPA) showing mucilage-containing apoplastic pockets (arrowheads) at the junction between the outer tangential and radial cell walls. The cell on the right, cut at an oblique angle, shows mucilage forming a ring around the cytoplasmic column (arrow). (D) A seed 10 DPA showing final accumulation of mucilage (arrowheads) surrounding the columella (arrow). At this stage, the secondary cell wall is in the process of being deposited around the cytoplasm. (E) Transmission electron micrograph of a 7 DPA seed at the top of the cytoplasmic column, showing the appression of the cytoplasmic column to the primary cell wall at the top of the cell (cw). Mucilage (mu) accumulates in an apoplastic space between the plasma membrane (pm) and cell wall. Several Golgi stacks (g, stacks indicated with arrows) are apparent, along with associated secretory vesicles. (F) Cartoon of the different stages of mucilage secretory cell development with the activity of known genes indicated in terms of basic role and approximate developmental stage of activity. Gene names in bold, large font are those with known regulatory interactions; positive regulation is indicated by black arrows between genes, hormones are noted in lowercase. Genes in brackets are those whose exact function is still to be determined. See the text for details of gene function.
In addition to diffuse cellulose embedded in pectinaceous mucilage, a number of species have obvious cellulosic threads or thick fibres projecting from their epidermal cells (Fig. 1). Often these fibres take on a spiral conformation that stretches and elongates upon mucilage hydration and release [e.g. Artemisia spp. (Asteraceae), Euphorbia falcata (Euphorbiaceae), Salvia horminum (Lamiaceae), Collomia grandiflora (Polemoniaceae), members of the Acanthaceae, Boraginaceae, Brassicaceae, Cistaceae, Cucurbitaceae, Orchidacea and Zygophyllaceae] (Grubert, Reference Grubert1974; Hsiao and Chuang, Reference Hsiao and Chuang1981; Schnepf and Deichgräber, Reference Schnepf and Deichgräber1983a; Kreitschitz and Vàlles, Reference Kreitschitz and Vàlles2007). The secondary cell wall inclusions that are deposited internal to the mucilage in Arabidopsis and other Brassicaceae, known as columellae, could be considered as thickened, truncated cellulosic fibres because their production during development is similar (Figs 1, 2D–E; see below) (Vaughan and Whitehouse, Reference Vaughan and Whitehouse1971; Schnepf and Deichgräber, Reference Schnepf and Deichgräber1983a, Reference Schnepf and Deichgräberb; McFarlane et al., Reference McFarlane, Young, Wasteneys and Samuels2008). Mucilage cells can also take the form of conical cells or long hairs that extrude varying amounts of mucilage [e.g. Blepharis persica, Ruellia strepens (Acanthaceae), Aethionema arabicum (Brassicaceae), Hydrocharis morsus-ranae (Hydrocaritaceae), some species of the Asteraceae, Cucurbitaceae and Lythraceae] (Witztum et al., Reference Witztum, Gutterman and Evenari1969; Gutterman et al., Reference Gutterman, Witztum and Heydecker1973; Grubert, Reference Grubert1974; Schnepf and Deichgräber, Reference Schnepf and Deichgräber1983b; Panigrahi, Reference Panigrahi1986), or consist of only a few cells or hairs in specific regions of the seed [e.g. Chrysanthemum nivellei, Artemisia spp. (Asteraceae) and members of the Acanthaceae, Goodeniaceae, Lamiaceae] (Grubert, Reference Grubert1974; Kreitschitz and Vàlles, Reference Kreitschitz and Vàlles2007). Finally, while most seed coat and pericarp mucilage is derived from the seed or fruit epidermis, some species synthesize and release mucilage to surround the seed from subepidermal cells alone or in combination with epidermal cells [e.g. Helianthemum apeninum (Cistaceae), Oxybaphus nyctagineus (Nyctaginaceae), Sporobolus cryptandrus (Poaceae)] (Grubert, Reference Grubert1974).
Cell biology of mucilage production
Mucilage secretory cell (MSC) differentiation has been studied extensively in Arabidopsis by both light and transmission electron microscopy (TEM) (Beeckman et al., Reference Beeckman, De Rycke, Viane and Inzé2000; Western et al., Reference Western, Skinner and Haughn2000; Windsor et al., Reference Windsor, Symonds, Mendenhall and Lloyd2000; McFarlane et al., Reference McFarlane, Young, Wasteneys and Samuels2008; Young et al., Reference Young, McFarlane, Hahn, Western, Haughn and Samuels2008). Pollination triggers cell growth driven by vacuolar expansion, following which a phase of extensive mucilage production occurs. Mucilage is secreted into the junction between the radial and the tangential cell walls on the apical face of the seed coat epidermal cells (Fig. 2C–E). This deposition results in the production of a ring-shaped mucilage pocket between the apoplast and the primary cell wall on the outer face of the cell (Fig. 2C). Mucilage secretion is coupled to the shrinking of the vacuole to the bottom of the cell and formation of a volcano-shaped cytoplasmic column that sits under the mucilage pocket. Mucilage production is followed by the synthesis of a cellulosic cell wall to surround and eventually occlude the cytoplasmic column, resulting in a volcano-shaped columella (Fig. 2D) (Beeckman et al., Reference Beeckman, De Rycke, Viane and Inzé2000; Western et al., Reference Western, Skinner and Haughn2000; Windsor et al., Reference Windsor, Symonds, Mendenhall and Lloyd2000). Seed hydration results in rapid mucilage release via breakage of the upper portion of the radial cell wall that is not reinforced by the presence of the columella. Within 1 min, hydrated Arabidopsis seeds are surrounded by a gel-like capsule of swollen mucilage (Arsovski et al., Reference Arsovski, Popma, Haughn, Carpita, McCann and Western2009a). Cell wall remnants remain attached to the tip of the columella and contribute to cellulosic ‘rays’ observed in the inner layer of mucilage (Western et al., Reference Western, Skinner and Haughn2000; Macquet et al., Reference Macquet, Ralet, Kronenberger, Marion-Poll and North2007a).
Throughout Arabidopsis MSC differentiation, starch granules (amyloplasts) are evident and undergo corresponding changes in size. The starch granules are apparent after cell growth and prior to mucilage synthesis, but become enlarged and prominent as mucilage production commences. As the secondary cell wall of the columella forms, the starch granules shrink, suggesting that their role is to provide sugar for the production of the secondary cell wall, rather than for mucilage synthesis. A corresponding accumulation and reduction of starch granules occurs in the subepidermal palisade cells, which lack mucilage but form a secondary cell wall in parallel with the MSCs (Western et al., Reference Western, Skinner and Haughn2000; Windsor et al., Reference Windsor, Symonds, Mendenhall and Lloyd2000). Further support for starch depletion being correlated with secondary cell wall synthesis comes from close examination of both starchless (phosphoglucomutase1) and starch excess1 mutants, which have altered columella shape (Windsor et al., Reference Windsor, Symonds, Mendenhall and Lloyd2000).
The ultrastructural details of mucilage production have been studied in Arabidopsis using high-pressure freezing and cryofixation to preserve the endomembrane system (Fig. 2E) (McFarlane et al., Reference McFarlane, Young, Wasteneys and Samuels2008; Young et al., Reference Young, McFarlane, Hahn, Western, Haughn and Samuels2008). Mucilage synthesis in the Golgi apparatus was confirmed through immunogold staining with an RG I-specific antibody (CCRC-M36) raised from Arabidopsis mucilage. Immunostaining also shows association of mucilage with the trans-Golgi network (TGN) and secretory vesicles, suggesting that mucilage is transported to the apoplast via secretory vesicles (McFarlane et al., Reference McFarlane, Young, Wasteneys and Samuels2008; Young et al., Reference Young, McFarlane, Hahn, Western, Haughn and Samuels2008). Prior to mucilage synthesis, the Golgi stacks have long, thin cisternae and the associated TGN is relatively small. During mucilage synthesis, however, the Golgi stacks have cisternae with compressed centres and swollen margins, as well as an enlarged TGN surrounded by large numbers of secretory vesicles that also are throughout the cytoplasm (Fig. 2E) (Young et al., Reference Young, McFarlane, Hahn, Western, Haughn and Samuels2008). Further, during mucilage synthesis, the number of Golgi stacks approximately doubles, as demonstrated both by counting of fluorescently labelled stacks in live cells and of fixed stacks in electron micrographs. Comparison of the Golgi stacks in a mutant making a reduced amount of mucilage [mucilage-modified4 (mum4)/rhamnose synthase2 (rhm2)] with wild-type cells revealed that, while the doubling of Golgi stacks is developmentally programmed, the altered morphology of the Golgi stacks (compressed cisternal lumens, swollen margins, increased TGN) during mucilage synthesis results from the production of copious amounts of mucilage (Young et al., Reference Young, McFarlane, Hahn, Western, Haughn and Samuels2008). During mucilage production, the Golgi stacks are evenly distributed throughout the cytoplasm, rather than accumulating near the site of the growing mucilage pocket. This observation is in contrast to other examples of polar cell-wall secretion in plant cells, such as the formation of the cell plate and cell wall deposition in tip-growing cells (e.g. root hairs, pollen tubes, trichomes), where the Golgi stacks are concentrated near the site of secretion (Jurgens, Reference Jurgens2005; Cole and Fowler, Reference Cole and Fowler2006; Campanoni and Blatt, Reference Campanoni and Blatt2007; Young et al., Reference Young, McFarlane, Hahn, Western, Haughn and Samuels2008).
The contribution of the cytoskeleton to mucilage secretion and MSC differentiation in Arabidopsis has also been investigated via TEM and fluorescence microscopy (McFarlane et al., Reference McFarlane, Young, Wasteneys and Samuels2008). Ultrastructural studies revealed a preponderance of cortical microtubules (MTs) lining the mucilage pocket. This organization of the MTs surrounding the zone of secretion at the constricted part of the cytoplasmic column was also observed using fluorescently labelled anti-tubulin antibodies. To further investigate a role for MTs in the regulation of mucilage secretion and/or the shaping of the cytoplasmic column, the temperature-sensitive mor1-1 mutant, which has disrupted MT organization, was employed (McFarlane et al., Reference McFarlane, Young, Wasteneys and Samuels2008). There were no gross differences in morphology of the MSCs or the organization of the Golgi apparatus or secretory vesicles in mor1-1 seeds grown under restrictive conditions. Mucilage release, however, was impaired in 40% of mor1-1 seeds grown under restrictive temperatures, versus only 22% for wild-type seeds. The release defect and lack of gross changes to the mucilage pocket or columella shape in mor1-1 mutants suggests a role for MTs in establishing the physical properties of mucilage, but their exact function is unclear (McFarlane et al., Reference McFarlane, Young, Wasteneys and Samuels2008). Unlike MTs, actin is not specifically localized in MSCs (McFarlane et al., Reference McFarlane, Young, Wasteneys and Samuels2008). The use of chemical treatments to disrupt MT and actin could reveal more about the role of these cytoskeletal elements in MSC differentiation and mucilage production, but such treatments have been unsuccessful (McFarlane et al., Reference McFarlane, Young, Wasteneys and Samuels2008).
In addition to the work in Arabidopsis, seed coat mucilage synthesis has been studied at the ultrastructural level using chemical fixation and TEM in Brassica campestris, Plantago ovata, Cydonia oblonga (Rosaceae), Solanum viarum (Solanaceae), C. grandiflora and Ruellia spp. (Hyde, Reference Hyde1970; Van Caeseele et al., Reference Van Caeseele, Mills, Sumner and Gillespie1981; Schnepf and Deichgräber, Reference Schnepf and Deichgräber1983a, b; Abeysekera and Willison, Reference Abeysekera and Willison1988; Srinivas et al., Reference Srinivas, Joshi and Krishnan1998). Similar to Arabidopsis, a prominent feature of mucilage production in each case is the appearance of large numbers of swollen Golgi stacks and associated secretory vesicles that are apparent as mucilage accumulates in the apoplastic space of the seed coat epidermal cells. In studies of P. ovata, S. viarum and B. campestris, the authors also note the accumulation of large starch granules which are then lost as mucilage accumulates. Similar results have also been noted at the light microscopy level for flax, suggesting that starch is providing sugar for mucilage synthesis (Hyde, Reference Hyde1970; Boesewinkel, Reference Boesewinkel1980; Van Caeseele et al., Reference Van Caeseele, Mills, Sumner and Gillespie1981; Srinivas et al., Reference Srinivas, Joshi and Krishnan1998). While these species have a reduction in cytoplasm and/or vacuole that accompanies mucilage production, none of them has cellulosic fibres or inclusions similar to the columella of Arabidopsis.
Collomia spp. and Ruellia spp. epidermal cells have both cellulosic mucilage with dispersed cellulose microfibrils and cellulosic inclusions. Collomia spp. have well-defined spiral secondary cell wall thickenings that are contiguous with the wall at the base of the cell – these are seen to expand when the cells are wetted and mucilage is released (Hsiao and Chuang, Reference Hsiao and Chuang1981; Schnepf and Deichgräber, Reference Schnepf and Deichgräber1983a). During differentiation of Ruellia spp. seed coats, the epidermal cells elongate into hairs that have spiral fibres embedded in the mucilage (‘mucilage strands’) that appear to be cellulosic (Schnepf and Deichgräber, Reference Schnepf and Deichgräber1983b). The differentiation of C. grandiflora and several Ruellia species were followed at the ultrastructural level by Schnepf and Deichgräber (Reference Schnepf and Deichgräber1983a, Reference Schnepf and Deichgräberb), who focused on the production of both ‘elemental fibres’ (cellulose microfibrils) and the cellulosic inclusions. In both cases, they found an association between the location and orientation of cellulose microfibrils with those of a number of MTs at the plasma membrane. Consideration of their results suggests a similar role in cellulose production for the MTs identified lining the mucilage pockets in Arabidopsis.
Genetics of mucilage production and MSC differentiation in Arabidopsis
Pectin synthesis and secretion
The presence of seed coat mucilage in Arabidopsis is dispensable under laboratory conditions, allowing the isolation of mutants affecting mucilage production and MSC differentiation (Table 2 and Fig. 2F) (Western et al., Reference Western, Burn, Tan, Skinner, Martin-McCaffrey, Moffatt and Haughn2001). Mutations in genes affecting the synthesis or secretion of mucilage have been identified through the lack of its release upon seed hydration, and fall under two categories: those that lack mucilage altogether and those with reduced mucilage production. Complete lack of mucilage synthesis has been correlated with defects in post-fertilization differentiation of the ovule outer integument, the epidermal layer of which is the MSCs. apetala2 (ap2) single- and nac regulated seed morphology1 (nars1) nars2 double-mutants lack differentiation of the MSCs and subtending palisade cells after the growth phase of seed development (Bowman and Koornneef, Reference Bowman, Koornneef and Bowman1994; Debeaujon et al., Reference Debeaujon, Léon-Kloosterziel and Koornneef2000; Western et al., Reference Western, Burn, Tan, Skinner, Martin-McCaffrey, Moffatt and Haughn2001; Kunieda et al., Reference Kunieda, Mitsuda, Ohme-Takagi, Takeda, Aida, Tasaka, Kondo, Nishimura and Hara-Nishimura2008; Molina et al., Reference Molina, Ohlrogge and Pollard2008). Both also exhibit seed shape defects: ap2 seeds are heart-shaped, with rectangular seed epidermal cells, while nars1 nars2 seeds are shrivelled with irregular-shaped cells. Embryo defects in nars1 nars2 suggest delayed development, which could also explain the seed coat phenotype (Kunieda et al., Reference Kunieda, Mitsuda, Ohme-Takagi, Takeda, Aida, Tasaka, Kondo, Nishimura and Hara-Nishimura2008). All three genes encode transcription factors, with AP2 encoding an AP2-type protein that plays roles in a number of developmental pathways, including flower and ovule development (Jofuku et al., Reference Jofuku, den Boer, Montagu and Okamuro1994). NARS1 and NARS2 encode NAC family proteins (Kunieda et al., Reference Kunieda, Mitsuda, Ohme-Takagi, Takeda, Aida, Tasaka, Kondo, Nishimura and Hara-Nishimura2008). In addition to AP2, NARS1 and NARS2, mutations in ABERRANT TESTA SHAPE (ATS) also affect mucilage production. ATS encodes a KANADI family transcription factor responsible for the specification of ovule integument layers. ats mutant seeds are heart-shaped and have only a single integument, the epidermal MSCs of which make a reduced amount of mucilage (Léon-Kloosterziel et al., Reference Léon-Kloosterziel, Keijzer and Koornneef1994; McAbee et al., Reference McAbee, Hill, Skinner, Izhaki, Hauser, Meister, Venugopala Reddy, Meyerowitz, Bowman and Gasser2006).
Table 2 Genes acting in mucilage production and mucilage secretory cell differentation in Arabidopsis
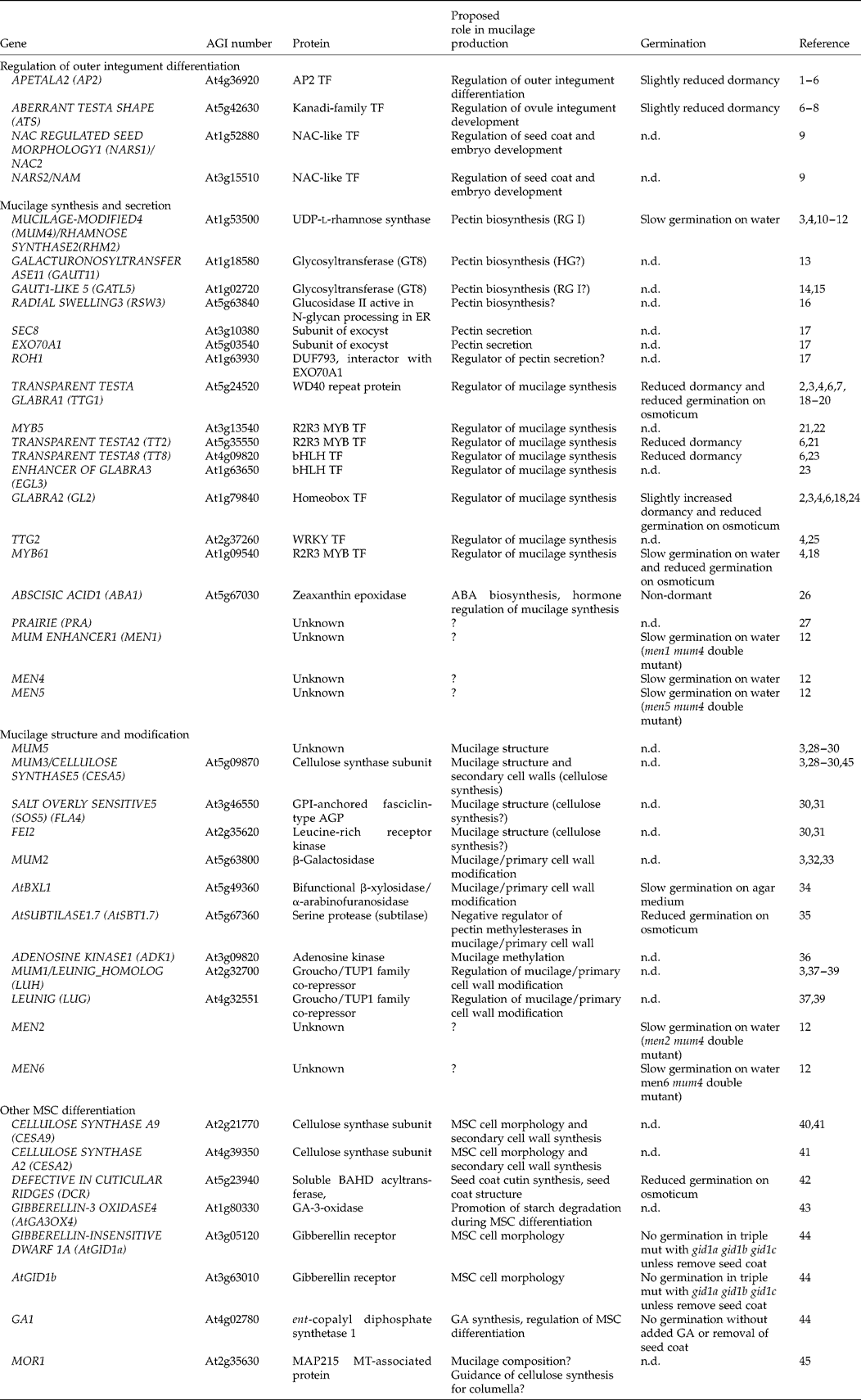
ABA, abscisic acid; AGP, arabinogalactan protein; ER, endoplasmic reticulum; GA, gibberellic acid; GPI, glycophosphatidylinositol; GT8, glycosyltransferase family 8; HG, homogalacturonan; MSC, mucilage secretory cell; MT, microtubule; n.d., not determined; TF, transcription factor. (1) Jofuku et al. (Reference Jofuku, den Boer, Montagu and Okamuro1994); (2) Bowman and Koornneef (Reference Bowman, Koornneef and Bowman1994); (3) Western et al. (Reference Western, Burn, Tan, Skinner, Martin-McCaffrey, Moffatt and Haughn2001); (4) Western et al. (Reference Western, Young, Dean, Tan, Samuels and Haughn2004); (5) Molina et al. (Reference Molina, Ohlrogge and Pollard2008); (6) Debeaujon et al. (Reference Debeaujon, Léon-Kloosterziel and Koornneef2000); (7) Léon-Kloosterziel et al. (Reference Léon-Kloosterziel, Keijzer and Koornneef1994); (8) McAbee et al. (Reference McAbee, Hill, Skinner, Izhaki, Hauser, Meister, Venugopala Reddy, Meyerowitz, Bowman and Gasser2006); (9) Kunieda et al. (Reference Kunieda, Mitsuda, Ohme-Takagi, Takeda, Aida, Tasaka, Kondo, Nishimura and Hara-Nishimura2008); (10) Usadel et al. (Reference Usadel, Kuschinsky, Rosso, Eckermann and Pauly2004); (11) Oka et al. (Reference Oka, Nemoto and Jigami2007); (12) Arsovski et al. (Reference Arsovski, Villota, Rowland, Subramaniam and Western2009b); (13) Caffall et al. (Reference Caffall, Pattathil, Phillips, Hahn and Mohnen2009); (14) Kong et al. (unpublished data); (15) Kong et al. (Reference Kong, Zhou, Yin, Xu, Pattathil and Hahn2011); (16) Burn et al. (Reference Burn, Hurley, Birch, Arioli, Cork and Williamson2002); (17) Kulich et al. (Reference Kulich, Cole, Drdová, Cvrčková, Soukup, Fowler and Žárský2010); (18) Penfield et al. (Reference Penfield, Meissner, Shoue, Carpita and Bevan2001); (19) Walker et al. (Reference Walker, Davison, Bolognesi-Winfield, James, Srinivasan, Blundell, Esch, Marks and Gray1999); (20) Koornneef (Reference Koornneef1981); (21) Gonzalez et al. (Reference Gonzalez, Mendenhall, Huo and Lloyd2009); (22) Li et al. (Reference Li, Milliken, Pham, Seyit, Napoli, Preston, Koltunow and Parish2009); (23) Zhang et al. (Reference Zhang, Gonzalez, Zhao, Payne and Lloyd2003); (24) Rerie et al. (Reference Rerie, Feldmann and Marks1994); (25) Johnson et al. (Reference Johnson, Kolevski and Smyth2002); (26) Karssen et al. (Reference Karssen, Brinkhorst-van der Swan, Breekland and Koornneef1983); (27) Western (Reference Western2006); (28) Macquet et al. (Reference Macquet, Ralet, Kronenberger, Marion-Poll and North2007a); (29) Sullivan et al. (Reference Sullivan, Ralet, Berger, Diatloff, Bischoff, Gonneau, Marion-Poll and North2011); (30) Harpaz-Saad et al. (Reference Harpaz-Saad, McFarlane, Xu, Divi, Forward, Western and Kieber2011); (31) Xu et al. (Reference Xu, Rahman, Baskin and Kieber2008); (32) Dean et al. (Reference Dean, Zheng, Tewari, Huang, Young, Hwang, Western, Carpita, McCann, Mansfield and Haughn2007); (33) Macquet et al. (Reference Macquet, Ralet, Loudet, Kronenberger, Mouille, Marion-Poll and North2007b); (34) Arsovski et al. (Reference Arsovski, Popma, Haughn, Carpita, McCann and Western2009a); (35) Rautengarten et al. (Reference Rautengarten, Usadel, Neumetzler, Hartmann, Büssis and Altmann2008); (36) Moffatt et al. (Reference Moffatt, Stevens, Allen, Snider, Pereira, Todorova, Summers, Weretilnyk, Martin-McCaffrey and Wagner2002); (37) Walker et al. (Reference Walker, Tehseen, Doblin, Pettolino, Wilson, Bacic and Golz2011); (38) Huang et al. (Reference Huang, Bowles, Esfandiari, Dean, Carpita and Haughn2011); (39) Bui et al. (Reference Bui, Lim, Sijacic and Liu2011); (40) Stork et al. (Reference Stork, Harris, Griffiths, Williams, Beisson, Li-Beisson, Mendu, Haughn and DeBolt2010); (41) Mendu et al. (Reference Mendu, Griffiths, Persson, Stork, Downie, Voiniciuc, Haughn and DeBolt2011); (42) Panikashvili et al. (Reference Panikashvili, Shi, Schreiber and Aharoni2009); (43) Kim et al. (Reference Kim, Nakajima, Nakayama and Yamaguchi2005); (44) Iuchi et al. (Reference Iuchi, Suzuki, Kim, Iuchi, Kuromori, Ueguchi-Tanaka, Asami, Yamaguchi, Matsuoka, Kobayashi and Nakajima2007); (45) McFarlane et al. (Reference McFarlane, Young, Wasteneys and Samuels2008).
The best-characterized reduced-mucilage mutant is mum4/rhm2. MUM4/RHM2 is a rhamnose synthase required for the production of UDP-l-rhamnose, a substrate for the production of the pectin RG I (Usadel et al., Reference Usadel, Kuschinsky, Rosso, Eckermann and Pauly2004; Western et al., Reference Western, Young, Dean, Tan, Samuels and Haughn2004; Oka et al., Reference Oka, Nemoto and Jigami2007). mum4/rhm2 mutants make less than half the wild-type amount of both rhamnose and galacturonic acid, the two components of the RG I backbone, and are unable to release mucilage unless treated with a metal chelator to weaken the primary cell wall. The smaller mucilage pockets of mum4/rhm2 seeds are correlated with a flattened columella, demonstrating the role of mucilage accumulation in the shaping of this secondary cell wall. MUM4/RHM2 is a member of a three-gene family and is specifically upregulated at the time of mucilage production (Usadel et al., Reference Usadel, Kuschinsky, Rosso, Eckermann and Pauly2004; Western et al., Reference Western, Young, Dean, Tan, Samuels and Haughn2004). MUM4/RHM2 transcription in MSCs is regulated by several pleiotropic transcription factors: AP2, TRANSPARENT TESTA GLABRA1 (TTG1), GLABRA2 (GL2), TRANSPARENT TESTA2 (TT2), TT8, ENHANCER OF GLABRA3 (EGL3) and MYB5. With the exception of ap2 mutants, which lack mucilage completely, single or double mutants of the other regulators of MUM4/RHM2 all exhibit a similar phenotype to mum4 (Koornneef, Reference Koornneef1981; Bowman and Koornneef, Reference Bowman, Koornneef and Bowman1994; Debeaujon et al., Reference Debeaujon, Léon-Kloosterziel and Koornneef2000; Zhang et al., Reference Zhang, Gonzalez, Zhao, Payne and Lloyd2003; Western et al., Reference Western, Young, Dean, Tan, Samuels and Haughn2004; Gonzalez et al., Reference Gonzalez, Mendenhall, Huo and Lloyd2009; Li et al., Reference Li, Milliken, Pham, Seyit, Napoli, Preston, Koltunow and Parish2009). The WD40 repeat protein TTG1 is an epidermal cell differentiation factor that acts in multiple tissues through complexes with bHLH and MYB transcription factors. In the MSCs, TTG1 interacts with the bHLH proteins EGL3 and TT8, and the R2R3 MYBs MYB5 and TT2 to upregulate the homeobox transcription factor GL2 (Rerie et al., Reference Rerie, Feldmann and Marks1994; Walker et al., Reference Walker, Davison, Bolognesi-Winfield, James, Srinivasan, Blundell, Esch, Marks and Gray1999; Gonzalez et al., Reference Gonzalez, Mendenhall, Huo and Lloyd2009; Li et al., Reference Li, Milliken, Pham, Seyit, Napoli, Preston, Koltunow and Parish2009). GL2, in turn, upregulates MUM4/RHM2 transcription, though it is not known if it interacts directly with the MUM4/RHM2 promoter. AP2 also affects the transcription of MUM4/RHM2 indirectly through upregulation of GL2, but does so independently of the TTG1–bHLH–MYB complex (Western et al., Reference Western, Young, Dean, Tan, Samuels and Haughn2004). TTG2, another downstream gene of AP2 and the TTG1–bHLH–MYB complex, also affects mucilage production in parallel with GL2. A final regulator of mucilage synthesis is MYB61, which appears to act through a parallel pathway to that regulated by AP2 and TTG1 (Fig. 2F) (Penfield et al., Reference Penfield, Meissner, Shoue, Carpita and Bevan2001; Johnson et al., Reference Johnson, Kolevski and Smyth2002). Both ttg2 and myb61 mutants exhibit reduced mucilage phenotypes similar to that of mum4/rhm2 (Penfield et al., Reference Penfield, Meissner, Shoue, Carpita and Bevan2001; Johnson et al., Reference Johnson, Kolevski and Smyth2002).
The use of microarray analyses to look for genes co-regulated with MUM4/RHM2 by TTG1 and AP2 identified the glycosyl transferase 8 family (GT8) member GALACTURONOSYLTRANSFERASE-LIKE5 (GATL5) (Y. Kong, A.A. Abdeen, J. Schafhauser, T.L. Western and M.G. Hahn, unpublished data). Similar to mum4/rhm2, gatl5 mutants have reductions in both rhamnose and galacturonic acid, though less severe. The predicted function of GATL5 as a galacturonosyl transferase (Kong et al., Reference Kong, Zhou, Yin, Xu, Pattathil and Hahn2011) in combination with this phenotype suggests that it acts directly in RG I synthesis. Another pectin biosynthetic enzyme implicated in mucilage production is the putative glycosyltransferase GALACTURONOSYLTRANSFERASE11 (GAUT11). gaut11 mutants can have somewhat reduced mucilage; however, unlike mum4/rhm2 and gatl5 mutants, only galacturonic acid content is affected (Caffall et al., Reference Caffall, Pattathil, Phillips, Hahn and Mohnen2009). This result suggests that GAUT11 acts in the production of HG in the adherent layer of mucilage.
Recent studies of mutants for two subunits of the exocyst, sec8 and exo70a1, revealed reduced mucilage and flattened columellae similar to those in mum4/rhm2 mutants (Kulich et al., Reference Kulich, Cole, Drdová, Cvrčková, Soukup, Fowler and Žárský2010). The exocyst is a plasma-membrane-associated secretory vesicle tethering complex (Zhang et al., Reference Zhang, Liu, Emons and Ketelaar2010) and is the first component of the secretory system identified to have a significant effect on mucilage accumulation. A yeast two-hybrid screen to identify exocyst interacting proteins, using EXO70A1 as bait, identified several proteins, including ROH1. While a loss of function T-DNA insertion of ROH1 (roh1-d) had no phenotype, two regulatory region insertions that resulted in increased ROH1 transcription (roh1-p and roh1-e) had reduced mucilage. ROH1 encodes a DUF793 protein and has been hypothesized to act as a negative regulator of secretion (Kulich et al., Reference Kulich, Cole, Drdová, Cvrčková, Soukup, Fowler and Žárský2010).
To identify further genes involved in mucilage production and MSC differentiation, the reduced mucilage phenotype of mum4/rhm2 seeds was used as the basis for a genetic enhancer–supressor screen. Eight mum enhancers (men) were identified, including new mutant alleles of the known reduced mucilage gene myb61 and the cell wall modification gene mum2 (see below), and six novel genes (Arsovski et al., Reference Arsovski, Villota, Rowland, Subramaniam and Western2009b). Microscopic and chemical analyses suggested that MEN1, MEN4 and MEN5 affect the amount of mucilage produced, while MEN2 and MEN6 affect mucilage release upon seed hydration (Arsovski et al., Reference Arsovski, Villota, Rowland, Subramaniam and Western2009b). Other genes with significant effects on mucilage production are PRAIRIE and the glucosidase II RADIAL SWELLING3 (Burn et al., Reference Burn, Hurley, Birch, Arioli, Cork and Williamson2002; Western, Reference Western2006). Cloning and/or further analyses of these genes will allow elucidation of their exact roles regarding mucilage synthesis and secretion.
A final gene affecting mucilage production is the hormone abscisic acid (ABA) biosynthetic gene ABSCISIC ACID1 (ABA1). The mucilage layer surrounding aba1 mutant seeds can be reduced as much as 50% and is correlated with the maternal dose of ABA received by seeds mid-way through their development. Mucilage production can be stimulated by ABA, as demonstrated by both the rescue of aba1 mutants and increased mucilage production of wild-type plants treated with ABA (Karssen et al., Reference Karssen, Brinkhorst-van der Swan, Breekland and Koornneef1983). Interestingly, increased MUM4/RHM2 transcription is induced in seedlings and developing seeds in response to ABA treatment, suggesting that it may regulate mucilage production through the amount of UDP-l-rhamnose substrate available for RG I synthesis (Hoth et al., Reference Hoth, Morgante, Sanchez, Hanafey, Tingey and Chua2002; Usadel et al., Reference Usadel, Kuschinsky, Rosso, Eckermann and Pauly2004) (U.K. Divi and T.L. Western, unpublished data).
Mucilage structure and modification
A screen for mutants specifically affected in mucilage staining, which uses the pectin dye ruthenium red, identified not only the reduced mucilage mutant mum4, but also mutants affected in mucilage staining/composition (mum3, mum5) and in mucilage release upon seed hydration (mum1, mum2) (Table 2, Fig. 2F) (Western et al., Reference Western, Burn, Tan, Skinner, Martin-McCaffrey, Moffatt and Haughn2001). mum3 and mum5 mutants stained in ruthenium red, with shaking, appear to have no, or reduced, mucilage surrounding the seed; however, when stained without shaking, substantial amounts of mucilage are observed (Western et al., Reference Western, Burn, Tan, Skinner, Martin-McCaffrey, Moffatt and Haughn2001). Further studies of mum5 using both staining and chemical analyses revealed alterations to the mucilage structure suggesting that the inner adherent layer is reduced while the outer soluble layer is increased, i.e. an increased proportion of mum5 mucilage is soluble RG I (Macquet et al., Reference Macquet, Ralet, Kronenberger, Marion-Poll and North2007a; Harpaz-Saad et al., Reference Harpaz-Saad, McFarlane, Xu, Divi, Forward, Western and Kieber2011; Sullivan et al., Reference Sullivan, Ralet, Berger, Diatloff, Bischoff, Gonneau, Marion-Poll and North2011). Presumably this change results from altered cross-linkages between pectins and/or cellulose found within the inner mucilage layer (Western et al., Reference Western, Burn, Tan, Skinner, Martin-McCaffrey, Moffatt and Haughn2001; Macquet et al., Reference Macquet, Ralet, Kronenberger, Marion-Poll and North2007a). Recently, mutants for the cellulose synthase subunit CELLULOSE SYNTHASE5 (CESA5), the leucine-rich receptor kinase FEI2 and the glycophosphatidylinositol-anchored fasciclin-like arabinogalactan protein SALT OVERLY SENSITIVE5 (SOS5) were found to have similar mucilage phenotypes of increased soluble mucilage paired with decreased adherent mucilage (Harpaz-Saad et al., Reference Harpaz-Saad, McFarlane, Xu, Divi, Forward, Western and Kieber2011; Mendu et al., Reference Mendu, Griffiths, Persson, Stork, Downie, Voiniciuc, Haughn and DeBolt2011; Sullivan et al., Reference Sullivan, Ralet, Berger, Diatloff, Bischoff, Gonneau, Marion-Poll and North2011). Sequencing and genetic complementation tests have demonstrated that the mum3 mutant is an allele of CESA5 (Sullivan et al., Reference Sullivan, Ralet, Berger, Diatloff, Bischoff, Gonneau, Marion-Poll and North2011). The identification of cesa5 mutants with reduced adherent mucilage supports the hypothesis that the inner layer of adherent mucilage is attached and organized through pectic interaction with cellulose (Willats et al., Reference Willats, McCartney and Knox2001; Macquet et al., Reference Macquet, Ralet, Kronenberger, Marion-Poll and North2007a). FEI2 and SOS5 likely regulate cellulose synthesis in the MSCs, as they have been implicated to do in seedling roots (Xu et al., Reference Xu, Rahman, Baskin and Kieber2008; Harpaz-Saad et al., Reference Harpaz-Saad, McFarlane, Xu, Divi, Forward, Western and Kieber2011). Because MUM5 is not allelic to any of the other genes affecting this process, its molecular role still remains to be elucidated (B. Forward and T.L. Western, unpublished data).
mum1 and mum2 are members of a group of mutants that make wild-type quantities of mucilage, but are defective in mucilage release upon seed hydration (Western et al., Reference Western, Burn, Tan, Skinner, Martin-McCaffrey, Moffatt and Haughn2001). Other genes include AtBETA-XYLOSIDASE1 (AtBXL1) and AtSUBTILASE1.7 (AtSBT1.7) (Rautengarten et al., Reference Rautengarten, Usadel, Neumetzler, Hartmann, Büssis and Altmann2008; Arsovski et al., Reference Arsovski, Popma, Haughn, Carpita, McCann and Western2009a). mum2 mucilage has increased galactose, arabinose and RG I branchpoints (Dean et al., Reference Dean, Zheng, Tewari, Huang, Young, Hwang, Western, Carpita, McCann, Mansfield and Haughn2007; Macquet et al., Reference Macquet, Ralet, Loudet, Kronenberger, Mouille, Marion-Poll and North2007b). This altered structure is correlated with an inability of mum2 mucilage to swell appreciably even in cut cells unless treated with sodium carbonate (Dean et al., Reference Dean, Zheng, Tewari, Huang, Young, Hwang, Western, Carpita, McCann, Mansfield and Haughn2007). Cloning of MUM2 revealed that it encodes a β-galactosidase that is implicated in the trimming of RG I side-chains such as terminal galactose, galactans and arabinogalactans (Dean et al., Reference Dean, Zheng, Tewari, Huang, Young, Hwang, Western, Carpita, McCann, Mansfield and Haughn2007; Macquet et al., Reference Macquet, Ralet, Loudet, Kronenberger, Mouille, Marion-Poll and North2007b). In contrast, bxl1 mutants have slow and patchy mucilage release that is correlated with increased pectic arabinans in both extracted mucilage and MSC cell walls. AtBXL1 encodes a bifunctional β-xylosidase/α-arabinofuranosidase that acts as an arabinofuranosidase during MSC development (Arsovski et al., Reference Arsovski, Popma, Haughn, Carpita, McCann and Western2009a). Analysis of mutants for both of these genes reveals not only the need for the trimming of RG I side-chains to enable mucilage release, but also the effect of pectin side-chain modification on developmental processes.
Unlike MUM2 and AtBXL1, AtSBT1.7 has an indirect effect on pectin structure. sbt1.7 mutants fail to release mucilage unless treated with cation chelators that weaken pectin gel structure by disruption of calcium bridges between galacturonic acid residues (Rautengarten et al., Reference Rautengarten, Usadel, Neumetzler, Hartmann, Büssis and Altmann2008). Methylation analysis of extracted sbt1.7 mucilage revealed decreased methylesterification, which was reflected by altered binding of antibodies to methylesterified HG. Quantification of pectin methylesterase (PME) activity throughout seed development found increased PME activity late in seed development compared with wild type. AtSBT1.7 encodes a subtilisin-like serine protease (subtilase) and has been hypothesized to regulate in muro pectin de-esterification in mucilage and/or the outer primary cell wall of MSCs by degradation of PMEs or activation of PME inhibitors via limited proteolysis (Rautengarten et al., Reference Rautengarten, Usadel, Neumetzler, Hartmann, Büssis and Altmann2008). Similar to MUM2 and AtBXL1, AtSBT1.7 activity is necessary for sufficient mucilage swelling to drive its release and/or weakening of the outer primary cell wall. Interestingly, decreased pectin methylesterification was also detected in antisense lines for ADENOSINE KINASE1 (ADK1), a gene encoding an enzyme involved in the salvage synthesis of adenine monophosphate from adenosine and ATP (Moffatt et al., Reference Moffatt, Stevens, Allen, Snider, Pereira, Todorova, Summers, Weretilnyk, Martin-McCaffrey and Wagner2002). adk1-deficient lines are impaired in S-adenosyl-l-methionine (SAM)-dependent methylation reactions, including those taking place in the Golgi apparatus to methylate HG. While adk1-deficient lines can have significant decreases in pectin methylesterification even below those in sbt1.7 mutants, they have normal mucilage release, suggesting that localization or developmental timing of de-esterification is important (Moffatt et al., Reference Moffatt, Stevens, Allen, Snider, Pereira, Todorova, Summers, Weretilnyk, Martin-McCaffrey and Wagner2002; Rautengarten et al., Reference Rautengarten, Usadel, Neumetzler, Hartmann, Büssis and Altmann2008).
mum1 mutants have a similar phenotype to mum2, where lack of mucilage swelling is coupled with increases in galactose, arabinose, RG I branching and HG methylesterification. Close examination of mum1 versus mum2 mutants also revealed thickening of the radial cell walls in both lines (Huang et al., Reference Huang, Bowles, Esfandiari, Dean, Carpita and Haughn2011; Walker et al., Reference Walker, Tehseen, Doblin, Pettolino, Wilson, Bacic and Golz2011). MUM1 encodes LEUNIG_HOMOLOG (LUH), a transcriptional co-repressor in the Groucho/Tup1 family (Bui et al., Reference Bui, Lim, Sijacic and Liu2011; Huang et al., Reference Huang, Bowles, Esfandiari, Dean, Carpita and Haughn2011; Walker et al., Reference Walker, Tehseen, Doblin, Pettolino, Wilson, Bacic and Golz2011). LUH is a close homologue of the developmental regulator LEUNIG (LUG). The latter forms a co-repression complex with SEUSS or a SEUSS-LIKE protein that partners with specific DNA-binding proteins to regulate flower, embryo, leaf and vascular development. LUH acts partially redundantly with LUG, both in the seed coat and in the regulation of embryo and flower development (Sitaraman et al., Reference Sitaraman, Bui and Liu2008; Bui et al., Reference Bui, Lim, Sijacic and Liu2011; Walker et al., Reference Walker, Tehseen, Doblin, Pettolino, Wilson, Bacic and Golz2011). Expression analysis of MUM2 in mum1/luh mutants revealed reduced MUM2 transcript, suggesting that a role of MUM1/LUH is to upregulate MUM2 during MSC differentiation. Transformation of mum1/luh mutants with 35Spro::MUM2 and LUH pro::MUM2 resulted in partial rescue of mucilage extrusion, both confirming the hypothesis that MUM2 is downstream of MUM1/LUH and suggesting other targets during MSC differentiation (Bui et al., Reference Bui, Lim, Sijacic and Liu2011; Huang et al., Reference Huang, Bowles, Esfandiari, Dean, Carpita and Haughn2011; Walker et al., Reference Walker, Tehseen, Doblin, Pettolino, Wilson, Bacic and Golz2011). Quantitative reverse-transcription polymerase chain reaction (qRT-PCR) of AtBXL1 and AtSBT1.7 in developing mum1/luh seeds also revealed decreased transcription, suggesting integrated positive regulation of mucilage/MSC cell wall modifications by MUM1/LUH (Fig. 2F) (Huang et al., Reference Huang, Bowles, Esfandiari, Dean, Carpita and Haughn2011).
Columella production and other aspects of seed coat differentiation affecting MSCs
When viewed with scanning electron microscopy or with fluorescent dye, the MSCs on the surface of the Arabidopsis seed coat are visible as a regular array of hexagonal cells with thickened radial cell walls and raised round or oval ‘plateaus’ in the centre of each cell, representing the top of the volcano-shaped columella (Fig. 2B). Mutants for the cellulose synthase subunit CESA9 exhibit a disorganized seed coat, including defects in columella and radial cell wall patterning, and reduced radial cell wall height (Stork et al., Reference Stork, Harris, Griffiths, Williams, Beisson, Li-Beisson, Mendu, Haughn and DeBolt2010). While cesa9 mutant seeds are smaller with decreased cellulose compared to wild-type seeds, they show no difference in mucilage composition or release. Together these data suggest a role for cesa9 in production of the secondary radial cell walls and columella (Stork et al., Reference Stork, Harris, Griffiths, Williams, Beisson, Li-Beisson, Mendu, Haughn and DeBolt2010). Recently, redundant roles in secondary cell wall development in the MSCs have also been identified for CESA2 and CESA5 (Mendu et al., Reference Mendu, Griffiths, Persson, Stork, Downie, Voiniciuc, Haughn and DeBolt2011). The defects in secondary cell wall synthesis in cesa9 mutants are associated with increases of other polymers, including neutral monosaccharides in pectins and hemicelluloses, and lipid polyester monomers that are normally associated with cutin and/or suberin. Compensative changes in polymer production are common in cell wall biosynthetic mutants (Stork et al., Reference Stork, Harris, Griffiths, Williams, Beisson, Li-Beisson, Mendu, Haughn and DeBolt2010). Another potential example of compensative cell wall changes that affect MSCs is that of mutants for the cutin biosynthetic gene DEFECTIVE IN CUTICULAR RIDGES (DCR) (Panikashvili et al., Reference Panikashvili, Shi, Schreiber and Aharoni2009). dcr mutants, in addition to having substantial decreases in several cutin monomers in the seed, are unable to release mucilage upon seed hydration. Similar to other epidermal cells, MSCs observed with TEM have a cuticle-like osmophilic layer coating their outer cell walls; alterations to this cuticular layer or compensatory changes to the subtending cell wall could prevent mucilage extrusion in dcr seeds (Panikashvili et al., Reference Panikashvili, Shi, Schreiber and Aharoni2009).
Distorted external MSC morphology is also seen in several mutants affected in the production of and response to gibberellins (GAs). These include mutants for the biosynthetic genes GA1 (ent-copalyl diphosphate synthetase 1) and GIBBERELLIN-3-OXIDASE4 (AtGA3OX4), and a double mutant for the GA receptors GIBBERELLIN-INSENSITIVE DWARF 1A (GID1A) and GID1B (Kim et al., Reference Kim, Nakajima, Nakayama and Yamaguchi2005; Iuchi et al., Reference Iuchi, Suzuki, Kim, Iuchi, Kuromori, Ueguchi-Tanaka, Asami, Yamaguchi, Matsuoka, Kobayashi and Nakajima2007). A study of the regulation of α-amylase genes by GA synthesis in Arabidopsis seeds identified a role for AtGA3OX4 in the regulation of starch metabolism in seed coats (Kim et al., Reference Kim, Nakajima, Nakayama and Yamaguchi2005). ga3ox4 knockout seeds had delayed starch degradation in the seed coat coupled with reduced mucilage release upon seed hydration. Both phenotypes could be rescued by treatment of developing seeds with GA. Thus GA may regulate substrate availability for both mucilage and columella secondary cell wall production through α-amylase-mediated starch degradation (Fig. 2F) (Kim et al., Reference Kim, Nakajima, Nakayama and Yamaguchi2005).
Ecological significance of seed coat mucilages – germination and dispersal
Seed coat mucilage production requires a significant carbon allocation during early seed development, suggesting that there must be selection for myxodiaspory. A number of hypotheses have been suggested for the presence of seed coat mucilages, including: (1) aid for seed hydration, especially under conditions of water or salt stress; (2) water reservoir for germination and/or early seedling development; (3) inhibition of germination by preventing oxygen flow to the embryo; (4) aid to germination by promoting embryo DNA repair (seed priming); (5) seed adhesion to soil to prevent removal by water or predators; (6) seed adhesion to animal vectors for dispersal (epizoochory); and (7) aid to seed dispersal by animal ingestion (endozoochory) (see Table 1).
Role of seed coat mucilages in seed hydration and germination
Due to the significant hydrophilicity of mucilages and their impressive swelling and water retention abilities, a role for mucilages in seed hydration and/or as a water reservoir for germination seems obvious (Grubert, Reference Grubert1974; Fahn, Reference Fahn1982; Kreitschitz, Reference Kreitschitz and Gorb2009). Such a role is also suggested by the high frequency of myxospermic plants in arid habitats (Grubert, Reference Grubert1974; Ellner and Shmida, Reference Ellner and Shmida1981; Ryding, Reference Ryding2001; Kreitschitz and Vàlles, Reference Kreitschitz and Vàlles2007; Kreitschitz, Reference Kreitschitz and Gorb2009). Ryding (Reference Ryding2001) performed a thorough study of myxocarpy in 400 species across the Nepetoideae (Lamiaceae), where he used both experimentation and the literature to analyse the correlations between the amount of mucilage produced, presence of nutlet trichomes, plant habitat and plant duration (annual versus perennial). He found that species from dry habitats had a greater frequency of myxospermy. Interestingly, this held for both arid and more humid regions, with tropical Africa having the highest relative proportion of myxodiaspory of the regions where Nepetoideae are found (Ryding, Reference Ryding2001).
Direct experiments for a role of mucilages in seed hydration have been performed on a number of species. Harper and Benton (Reference Harper and Benton1966) compared germination of seeds with copious mucilage, less-copious mucilage, bumpy (tuberculate) surface and smooth surface on filter paper versus sintered glass plates with 0–200 cm water tension. Seeds with copious mucilage were able to germinate in all treatments, with some slowing of germination at higher water tensions. Those with less-copious mucilage had poorer germination at higher water tensions, while those with tuberculate or smooth surfaces generally had very poor germination. Upon wetting, the swollen mucilage increased contact between the seed and the pores of the plate, presumably aiding water flow to the seed. Further investigations between a species with copious mucilage (L. sativum) and a tuberculate seed of similar size (Agrostemma githago, Caryphyllaceae) revealed that seeds with mucilage could also germinate well at high water tensions on simulated soil particles of different grades. Further, germination of A. githago under high water tension could be increased by the addition of exogenous Plantago psyllium mucilage, burying of the seed and covering the plates with plastic wrap to increase humidity. From these experiments, they concluded that germination is a balance between water uptake from the substrate and water loss to the atmosphere, and that mucilage aids the former through increased surface contact and may also reduce the latter (Harper and Benton, Reference Harper and Benton1966). Mott (Reference Mott1974) compared germination between three species from an arid region of Western Australia: Helipterum craspedioides (Asteraceae; mucilaginous pericarp), Helichrysum cassinianum (Asteraceae; pericarp with long hairs) and Aristida contorta (Poaceae; no surface features). Tests were performed comparing intact seeds with those with mucilage or hairs removed. While both the hairs and mucilage spread out when wet and promoted seed–substrate contact, there was no benefit from either when imbibed or dehydrated under non-limiting water conditions. When tested on soil with different moisture contents, however, both mucilage and hairs aided seed germination if seeds were first sprayed with water to promote mucilage release/hair spreading. This suggests that mucilage aids seed hydration and germination in the presence of some surface water (Mott, Reference Mott1974). Achenes of Artemisia sphaerocephala (Asteraceae) were tested for germination of intact achenes versus those with mucilage removed (Yang et al., Reference Yang, Dong and Huang2010). While no difference was found under non-limiting conditions, ‘demucilaged’ achenes showed greater sensitivity to both increasing osmotic potential [polyethylene glycol (PEG) concentration] and salinity (NaCl) stress. Further, mucilaginous achenes whose germination was inhibited by high concentrations of NaCl were more likely to germinate when moved to non-limiting conditions. Thus, the presence of mucilage can aid germination in desert habitats with both drought and salt-stress (Yang et al., Reference Yang, Dong and Huang2010).
Recent work in Arabidopsis has also suggested a role for mucilage in seed hydration during germination. Mutant ttg1, gl2 and myb61 reduced-mucilage seeds are more sensitive to low water potential than wild-type seeds, as determined by germination on increasing concentrations of PEG. myb61 seeds, which have more mucilage than those of ttg1 and gl2, had germination intermediate between wild type and the other two mutants (Penfield et al., Reference Penfield, Meissner, Shoue, Carpita and Bevan2001). Arsovski et al. (Reference Arsovski, Villota, Rowland, Subramaniam and Western2009b) demonstrated that seeds of the reduced mucilage mutant mum4 had slow germination under non-water-limiting conditions, which was exacerbated in double mutants with those men genes that further decrease the amount of mucilage. Preliminary studies of imbibition of mum4 and men4 mum4 double mutants suggest that imbibition is slowed and/or reduced with decreasing mucilage production (A.A. Arsovski and T.L. Western, unpublished data). Mutants affected in mucilage release also have defective germination: sbt1.7 and dcr mutants have reduced germination under low water potential conditions (PEG solutions), while bxl1 and mum1/luh mutants have slow germination under non-water-limiting conditions (Rautengarten et al., Reference Rautengarten, Usadel, Neumetzler, Hartmann, Büssis and Altmann2008; Sitaraman et al., Reference Sitaraman, Bui and Liu2008; Arsovski et al., Reference Arsovski, Popma, Haughn, Carpita, McCann and Western2009a; Panikashvili et al., Reference Panikashvili, Shi, Schreiber and Aharoni2009). A slowing of mum4 germination occurs also in double mutants for men genes proposed to be affected in mucilage release (Arsovski et al., Reference Arsovski, Villota, Rowland, Subramaniam and Western2009b). Together these results suggest that both the amount of mucilage present and the ability of mucilage to expand and extrude from epidermal cells to surround the seed are important for seed hydration and subsequent germination.
Grubert, in his comprehensive survey of angiosperm myxospermy (Reference Grubert1974), argues against mucilages as a water reservoir, citing his own and other experiments that demonstrate rapid water loss from mucilages. While there are cases, e.g. some desert plants (Kreitschitz, Reference Kreitschitz and Gorb2009), where germination may be rapid enough to occur before mucilage dehydration, it seems more likely from the above experiments that mucilage speeds initial seed hydration and/or hydration under mild water stress. Another germination-promoting role of seed mucilages was suggested by Huang et al. (Reference Huang, Boubriak, Osborne, Dong and Gutterman2008). They propose that mucilages maintain sub-germination seed hydration from overnight dew to allow seed priming in desert species. DNA repair of embryos of two species of Artemisia occurs in intact achenes but not in those where mucilage was removed. Further, DNA repair was faster in Artemisia sphaerocephala (copious pericarp mucilage) than in A. ordosica (moderate pericarp mucilage) (Huang et al., Reference Huang, Boubriak, Osborne, Dong and Gutterman2008). Garwood (Reference Garwood1985), found no effect of mucilage on cuipo (Cavanillesia plantanifolia, Bombaceae) germination. Rather, the water retention of the seed mucilage appeared to increase the speed of seedling development and to prevent wilting. Removal of mucilage from seeds of Dillenia indica (Dilleniaceae) also has no effect on germination under non-water-limiting conditions. The authors suggest that the seed mucilage acts to clump seeds together to retain them in the fruit to escape excessive drying and predation prior to germination (Thapliyal et al., Reference Thapliyal, Phartyal, Baskin and Baskin2008).
In contrast to a role in the promotion of germination, several studies suggest that mucilages can inhibit germination. Seeds of Diptychocarpus strictus, Lesquerella perforata and Lesquerella stonensis (Brassicaceae) had increased germination when mucilage was removed (Fitch et al., Reference Fitch, Walck and Hidayati2007; Lu et al., Reference Lu, Tan, Baskin and Baskin2010). Incubation of de-mucilaged D. strictus seeds with the isolated mucilage demonstrated that there was not an inhibitor in the mucilage, suggesting that the layer of gel around the seed was responsible for inhibition of germination (Lu et al., Reference Lu, Tan, Baskin and Baskin2010). Detailed studies of the mechanism of mucilage inhibition of germination have been performed for spinach (Spinacia oleracea, Amaranthaceae) and B. persica (Acanthaceae) seeds (Heydecker and Orphanos, Reference Heydecker and Orphanos1968; Witztum et al., Reference Witztum, Gutterman and Evenari1969). Similar to D. strictus, tests for both species showed no soluble chemical inhibitor in the mucilage. Spinach seeds are surrounded by a mucilaginous fruit coat. Germination decreases with increasing amounts of water and can be rescued by removal of part of the fruit coat, removal of mucilage, low temperature (increased oxygen solubility in water) and treatment with oxidizing chemicals such as hydrogen peroxide (Heydecker and Orphanos, Reference Heydecker and Orphanos1968). A similar effect was seen with seeds of B. persica, which have long mucilaginous hairs. In small amounts of water, the hairs expand separately, while under excess water the mucilage swells to fill the space between the hairs (Witztum et al., Reference Witztum, Gutterman and Evenari1969) and germination is prevented. Germination can be promoted by removing the seed coat, stripping mucilage from the seed, treatment with CaCl2 (reducing mucilage swelling), low temperature and increasing the percentage of oxygen in the germination chamber (Witztum et al., Reference Witztum, Gutterman and Evenari1969). Together these suggest that mucilage inhibits germination under excessively moist conditions by preventing the diffusion of oxygen to the embryo.
Role of seed coat mucilages in seed dispersal
Hydrated pectinaceous mucilages are very sticky, leading to hypotheses of their role as adhesives for seeds to adhere to soil or to animal vectors (Grubert, Reference Grubert1974; Fahn, Reference Fahn1982; Kreitschitz, Reference Kreitschitz and Gorb2009). Grubert (Reference Grubert1974) used binding of seeds to glass plates to assess the adhesive properties of almost 500 species across 20 families that varied in the amount of mucilage released, type of mucilage cells and mucilage structure. He found strong adhesion across all types of mucilage cells and hypothesized that the presence of cellulosic fibres and/or hair cells stabilized substrate binding. For seven species across six families, he determined precise forces for seed removal from the substrate and demonstrated substantial seed weight increase through adhesion of soil particles, especially to those seeds with mucilaginous hairs or highly soluble (mobile) mucilage (Grubert, Reference Grubert1974). Finally, he performed a time course on mucilage dehydration and found that all seven species returned to their dry weight within 2–7 h. Based on the high frequency of myxodiaspory in arid regions and his experimental results on seed adhesion and mucilage dehydration, Grubert proposed that the primary role of seed coat mucilage is fixation to the soil to prevent dispersal to less favourable habitats (antitelechory sensu Ellner and Shmida, Reference Ellner and Shmida1981) (Grubert, Reference Grubert1974). He also noted that in more humid climates, myxodiaspory could be acting in dispersal through sticking to animals (exozoochory) (Grubert, Reference Grubert1974). Increased seed weight through adhesion of sand and soil to mucilage has been demonstrated for other species, including D. strictus and Salvia columbariae (Lamiaceae) (Fuller and Hay, Reference Fuller and Hay1983; Lu et al., Reference Lu, Tan, Baskin and Baskin2010). García-Fayos et al. (Reference García-Fayos, Bochet and Cerdà2010) investigated seed characteristics affecting seed removal from slopes by rainfall runoff. Using simulated rainfall on a sandpaper-covered incline, they tested 141 species from dry regions of Spain, including 29 species with mucilaginous diaspores. Overall they demonstrated that seeds with mucilage were generally small and had a lower susceptibility to removal by runoff compared with non-myxospermous species of similar mass. Adaptations to immobilize seeds to prevent dispersal (antitelechory) are found widely among desert species, and have been proposed to keep progeny with the mother plant where conditions are appropriate for growth, a scarce commodity in arid regions. Based on a long-term survey of desert flora in Israel, Ellner and Shmida (Reference Ellner and Shmida1981) proposed that desert conditions of open ground instead favour atelechory (lack of any dispersal mechanism). Thus, they conclude any antitelechoric characteristics of dispersal units of desert species are a side-effect of mechanisms with other adaptive significance. Further, they suggested that myxodiaspory is not a mechanism of antitelechory per se, since it acts on the seed after its initial dispersal from the mother plant.
In addition to the prevention of seed removal by abiotic forces, adhesion of the seed to soil, or soil to the seed, has been suggested to prevent predation – either by increasing the mass of the seed to prevent its removal by insects such as ants, or by camouflaging the seed from various predators (Young and Evans, Reference Young and Evans1973; Fuller and Hay, Reference Fuller and Hay1983; Gutterman and Shem-Tov, Reference Gutterman and Shem-Tov1997). Fuller and Hay (Reference Fuller and Hay1983) tested the response of various desert granivores to naked S. columbariae seeds versus those that had been wetted and coated in sand. Diurnal predators that hunt by sight, such as birds and ground squirrels, had a significant preference for uncoated seeds, while nocturnal rodents, which use scent to track food, had less difference in their choice. Ants seemed less likely to notice the covered seeds and, when they did, they had trouble moving the seeds that were approximately 11 times their uncoated weight, requiring several ants to move the seeds (Fuller and Hay, Reference Fuller and Hay1983).
As mentioned above, the adhesive power of mucilage has also been suggested to aid seed dispersal by sticking to animal fur or bird feathers (Young and Evans, Reference Young and Evans1973; Grubert, Reference Grubert1974). The conclusion of Ryding's survey of Nepetoideae (Reference Ryding2001) mentioned above was that the primary role of myxocarpy in the Nepetiodeae was epizoochory – or at least that there was no evidence in this case to support antitelechory. Lobova et al. (Reference Lobova, Mori, Blanchard, Peckham and Charles-Dominique2003) described a potential role for mucilage in endozoochory in Cecropia species in French Guiana. They proposed that the slimy mucilage lubricates seeds to aid their passage through the gut of bats.
Conclusions and future perspectives
Myxodiaspory is a common adaptation in at least 100 families of angiosperms. A rich literature over the past 150 years exists on the presence and distribution of seed coat/pericarp mucilages (see Table 1 and the extensive reviews and literature lists in Grubert, Reference Grubert1974, Reference Grubert1981), though less attention has been paid to it in recent decades. A resurgence in interest, however, has occurred recently due to its study in the model genetic plant Arabidopsis, leading to a literature of over 46 papers since 2000. At least 44 genes have been identified with roles in mucilage production or MSC differentiation in Arabidopsis (Fig. 2F and Table 2), with many more under investigation. The MSCs of Arabidopsis are of particular interest in the field of cell wall biology, where they have become a model system for the identification and investigation of genes involved in pectin synthesis and post-deposition modification (reviewed in Arsovski et al., Reference Arsovski, Haughn and Western2010). Genes involved in secondary cell wall production and mucilage secretion have also been identified recently (Kulich et al., Reference Kulich, Cole, Drdová, Cvrčková, Soukup, Fowler and Žárský2010; Stork et al., Reference Stork, Harris, Griffiths, Williams, Beisson, Li-Beisson, Mendu, Haughn and DeBolt2010), suggesting further utility in other aspects of cell wall production. Despite considerable progress, many aspects of mucilage synthesis, secretion and MSC differentiation are still poorly understood, thus continued gene identification and investigation of their roles is required.
Comparisons between the recent detailed studies of mucilage composition and production in Arabidopsis and those performed in the past in other species have highlighted a number of commonalities. These include the primarily pectinaceous nature of seed coat mucilages, the frequent presence of dispersed cellulose microfibrils and cellulosic fibres or inclusions, and the hypersecretory activity of the Golgi apparatus during mucilage synthesis. While the chemical and/or physical properties have been determined in detail for a number of mucilages of economically important species, e.g. mustard (S. alba), linseed flax (L. usitatissimum) and psyllium (Plantago spp.), Arabidopsis studies have linked chemical composition with structural domains within extruded mucilage (e.g. Macquet et al., Reference Macquet, Ralet, Kronenberger, Marion-Poll and North2007a; Harpaz-Saad et al., Reference Harpaz-Saad, McFarlane, Xu, Divi, Forward, Western and Kieber2011; Sullivan et al., Reference Sullivan, Ralet, Berger, Diatloff, Bischoff, Gonneau, Marion-Poll and North2011). A layer of diffuse mucilage primarily comprised of unbranched RG I is dispersed beyond a dense layer of complex pectic and cellulosic composition that appears to be linked to the seed. The increased solubility and swelling of the outer mucilage layer may be the driver of mucilage expansion for cell wall breakage, while the inner layer acts in seed hydration (or adhesion, see below). The differential extractability of these layers means that most quantitative information on the chemical composition of Arabidopsis mucilage only reflects the outer soluble mucilage, which may also be true for other species where compositional studies were performed with mild extraction procedures. Work in Arabidopsis has also revealed the complex modifications of apoplastic mucilage and/or the outer primary cell wall that are required for mucilage to be released upon seed hydration. These include both trimming of pectin side-chains and the regulation of pectin gel status through the degree of methylesterification of HG (Dean et al., Reference Dean, Zheng, Tewari, Huang, Young, Hwang, Western, Carpita, McCann, Mansfield and Haughn2007; Macquet et al., Reference Macquet, Ralet, Loudet, Kronenberger, Mouille, Marion-Poll and North2007b; Rautengarten et al., Reference Rautengarten, Usadel, Neumetzler, Hartmann, Büssis and Altmann2008; Arsovski et al., Reference Arsovski, Popma, Haughn, Carpita, McCann and Western2009a; Bui et al., Reference Bui, Lim, Sijacic and Liu2011; Huang et al., Reference Huang, Bowles, Esfandiari, Dean, Carpita and Haughn2011; Walker et al., Reference Walker, Tehseen, Doblin, Pettolino, Wilson, Bacic and Golz2011).
Despite the obvious selection for myxodiaspory in many species, its precise ecological role is still unclear. As suggested by the studies described in this review, it may have a number of roles in germination and dispersal, depending on the species and its environmental context. However, as described here and in the recent review by Kreitschitz (Reference Kreitschitz and Gorb2009), the number of well-controlled studies performed on the role of mucilages is limited and, generally, they test only one aspect of mucilage function. While some Arabidopsis mutants affected in mucilage production and its release to surround the seed have been tested for their effects on germination, more detailed studies comparing a range of mucilage defects directly with each other under more conditions are needed. Further studies in other species, both in controlled conditions and in their ecological contexts, are also necessary.
Acknowledgements
My apologies to those researchers whose studies I was unable to include in this review due to space considerations. I would like to thank Dr Derek Bewley, Dr Andrej Arsovski, Dr Uday Divi, Bronwen Forward and Cheng-En Tsai for helpful comments on the manuscript, Dr Thomas Bureau and Dr George Haughn for seeds of Aethionema arabicum and Salvia hispanica, respectively, and the Natural Sciences and Engineering Research Council of Canada for Discovery Grant funding.