Introduction
The seed dispersal process helps seeds escape from high mortality (Howe and Smallwood, Reference Howe and Smallwood1982), high competition and predation risk (Janzen, Reference Janzen1970) near the parent plant. In particular, long-distance dispersal (>100 m) allows plant populations to cope with global warming, habitat loss and fragmentation (Cain et al., Reference Cain, Milligan and Strand2000; McConkey et al., Reference McConkey, Prasad, Corlett, Campos-Arceiz, Brodie, Rogers and Santamaria2012). Furthermore, large-sized animals such as herbivores can disperse seeds over long distances (Baltzinger et al., Reference Baltzinger, Karimi and Shukla2019). Therefore, ungulate-mediated seed dispersal can connect plant populations, help colonize new suitable habitats, shape the plant distribution regional pattern and contribute to plant adaptation to climate change (McConkey et al., Reference McConkey, Prasad, Corlett, Campos-Arceiz, Brodie, Rogers and Santamaria2012). Consequently, seed dispersal will affect genetic and species diversity and regional vegetation dynamics (Cain et al., Reference Cain, Milligan and Strand2000; McConkey et al., Reference McConkey, Prasad, Corlett, Campos-Arceiz, Brodie, Rogers and Santamaria2012).
Seed dispersal effectiveness (i.e. the contribution of the dispersal vector to producing new recruits) can be evaluated from both quality and quantity aspects (Schupp, Reference Schupp1993; Schupp et al., Reference Schupp, Jordano and Gómez2017). The probability of seed to be dispersed by a disperser to germinate and successfully colonize (quality), and the number of dispersed seeds (quantity) affect the success of seed dispersal (Schupp et al., Reference Schupp, Jordano and Gómez2017; Zwolak, Reference Zwolak2018). The seed dispersal process consists of emigration, transfer and immigration phases, and when seeds complete each stage, seed dispersal can be regarded as successful (Wang and Smith, Reference Wang and Smith2002). Therefore, to understand the efficiency of endozoochorous seed dispersal, investigating it at each seed dispersal cycle stage is necessary (Traveset, Reference Traveset1998; Wang and Smith, Reference Wang and Smith2002). In the transfer phase, mastication and gut passage affect seed germination and determine the quality of seed dispersal (Baltzinger et al., Reference Baltzinger, Karimi and Shukla2019). According to a recent meta-analysis study, the effects of gut passage on seed germination varied among animal species (Torres et al., Reference Torres, Castano and Carranza-Quiceno2020). Also, the seed germination rates after gut passage differ according to ingested plant species (Mancilla-Leytón et al., Reference Mancilla-Leytón, Fernández-Alés and Vicente2011, Reference Mancilla-Leytón, González-Redondo and Vicente2013; Torres et al., Reference Torres, Castano and Carranza-Quiceno2020).
Endozoochorous seed dispersal has been demonstrated for large herbivores, including domestic and wild ungulates (e.g. Heinken et al., Reference Heinken, Hanspach, Raudnitschka and Schaumann2002; Benthien et al., Reference Benthien, Bober, Castens and Stolter2016; Picard et al., Reference Picard, Chevalier, Barrier, Boscardin and Baltzinger2016). Endozoochory by large herbivores can facilitate the seed dispersal of tiny, tough seeds inadvertently eaten while feeding on plant leaves (Janzen, Reference Janzen1984). The seeds dispersed by ingestion have distinctive traits, such as high seed longevity, round shape and the absence of an appendage (Pakeman et al., Reference Pakeman, Digneffe and Small2002; Albert et al., Reference Albert, Auffret, Cosyns, Cousins, D'hondt, Eichberg, Eycott, Heinken, Hoffmann, Jaroszewicz, Malo, Marell, Mouissie, Pakeman, Picard, Plue, Poschlod, Provoost, Schulze and Baltzinger2015; Mouissie et al., Reference Mouissie, Vos, Verhagen and Bakker2005b). Seed traits are related to seed survival. However, according to previous studies, seed traits have different effects on seed recovery. For instance, light seeds are likely to pass undamaged through the animal gut (Cosyns and Hoffmann, Reference Cosyns and Hoffmann2005; Mouissie et al., Reference Mouissie, Van Der Veen, Veen and Van Diggelen2005a). Also, seed size and hardness are important traits for seeds to successfully survive the gut passage (Jaganathan et al., Reference Jaganathan, Yule and Liu2016). Seed hardness, which can be seen as water impermeability of seeds, is the main trait affecting seed survival through endozoochory (Gardener et al., Reference Gardener, Mcivor and Jansen1993; D'hondt and Hoffmann, Reference D'hondt and Hoffmann2011). However, some studies found neither seed size nor shape was related to seed survival (Bruun and Poschlod, Reference Bruun and Poschlod2006; D'hondt and Hoffmann, Reference D'hondt and Hoffmann2011).
The Korean water deer (Hydropotes inermis argyropus) is listed as a vulnerable species by the International Union for Conservation of Nature (Harris and Duckworth, Reference Harris and Duckworth2015). In South Korea, habitat fragmentation threatens its habitat, and human–wildlife conflicts occur due to crop damage and road kills (Kim et al., Reference Kim, Lee and Kim2016). Most of its distribution range is limited to the Korean peninsula, and its population is decreasing globally. The Korean water deer is a browser (Kim et al., Reference Kim, Lee and Lee2011) and can disperse small seeds (≤2 mm) of forbs with no special morphology for endozoochory (Lee and Lee, Reference Lee and Lee2020). However, little is known about the effects of gut passage by Korean water deer on seed recovery and germination rate. With the high dominance and increase in the population of Korean water deer in the Korean peninsula (National Institute of Biological Resources, 2018), understanding their efficiency for endozoochory will help us understand their ecological role and provide useful information for their management and conservation.
In this study, using feeding experiments, we aimed to understand the efficiency of endozoochorous seed dispersal by the Korean water deer in terms of seed recovery and seed germination rates. We fed Korean water deer with eight common plant species whose seeds vary in size and hardness. Our questions were as follows: (1) What is the recovery rate of seeds after gut passage, and how does it vary among plant species? (2) Is there a correlation between seed recovery rate and seed traits? (3) Does gut passage promote or hamper seed germination and does it vary among plant species?
Materials and methods
Seed preparation and seed traits
Eight plant species with different trait values were chosen, which were either commonly found in Korea or germinated from the faeces of the Korean water deer, according to Lee and Lee (Reference Lee and Lee2020) (Table 1). The species nomenclature followed the Korean Plant Names Index (http://www.nature.go.kr/kpni/). These species were Amaranthus mangostanus, Chenopodium album, Capsella bursa-pastoris, Digitaria ciliaris, Panicum bisulcatum, Plantago asiatica, Portulaca oleracea and Solanum americanum. Most of the seeds were bought from a local seed supplier, but the seeds of D. ciliaris and P. bisulcatum were collected from the lowland area of Paju city, Gyeonggi province, South Korea, in November 2017, as these seeds were not purchasable. When collecting the seeds from the field, we ensured to collect seeds from a minimum of 50 individuals.
Table 1. List of plant species fed to the Korean water deer, indicating native/exotic status, seed origin and four seed characteristics (seed length, width, mass and hardness)

Different letters within columns indicate significant differences between species based on multiple comparisons after the Kruskal–Wallis test (P < 0.05). Exotic species status following Son et al. (Reference Son, Kang, Jung, Kim, Lee and Oh2019). The seed length and width data were acquired from the Korea Research Institute of Bioscience and Biotechnology (2009) and Bojnanský and Fargašová (Reference Bojnanský and Fargašová2007). Seed mass and seed hardness values are mean ± SD. For seed hardness, seeds too soft to accurately measure hardness are shown as NA (not available).
The seed hardness of 50 seeds per species was measured using a digital push-pull gauge (SF-50, Yueqing Aliyiqi Instrument Co., Ltd. China), except for P. asiatica, D. ciliaris, S. americanum and C. bursa-pastoris seeds, as these were too soft to measure the hardness. The seed mass was measured using the weight of 100 seeds (except for S. americanum, only 25 seeds) three times. The values were transformed into grams per 1000 seeds. The seed length and width were obtained from references (Bojnanský and Fargašová, Reference Bojnanský and Fargašová2007; Korea Research Institute of Bioscience and Biotechnology, 2009). Native/exotic status of each species was followed by Son et al. (Reference Son, Kang, Jung, Kim, Lee and Oh2019).
Feeding experiment
A feeding experiment was conducted at Wildlife Rescue Center, Kangwon National University. Due to logistic constraints in the available number of deer to perform the feeding experiments, only three Korean water deer (one male and two females: 14, 13.8 and 13 kg) were fed with a known number of seeds (Fig. 1). Each deer was put in an individual room with access to water ad libitum. One thousand seeds per species were given, except for S. americanum (500 seeds) and D. ciliaris (100 seeds) due to their availability and relatively large seed size (Table 1). These seeds were added to their normal diet of mixed cut carrots, potatoes and cabbages. Thus, there was a low possibility of outer seed contamination; however, to check this thoroughly, the faeces defaecated by each deer before the feeding experiment were collected, air-dried and checked for seed inclusion. No seeds were found in the control faeces. Additionally, the control faeces were also checked for germination to ensure further that no seedlings germinated. Along with the feeding processes, once the deer consumed all the provided seeds, their faeces were collected after 24, 48, 72 and 96 h. The collected faeces were air-dried, and the number of seeds retrieved from the faeces was counted for every time interval (0–24, 24–48, 48–72 and 72–96 h). To avoid seed misidentification, every seed was identified using a stereomicroscope (SMZ-745 Nikon Co., Ltd., Japan), and the intact seeds were counted.
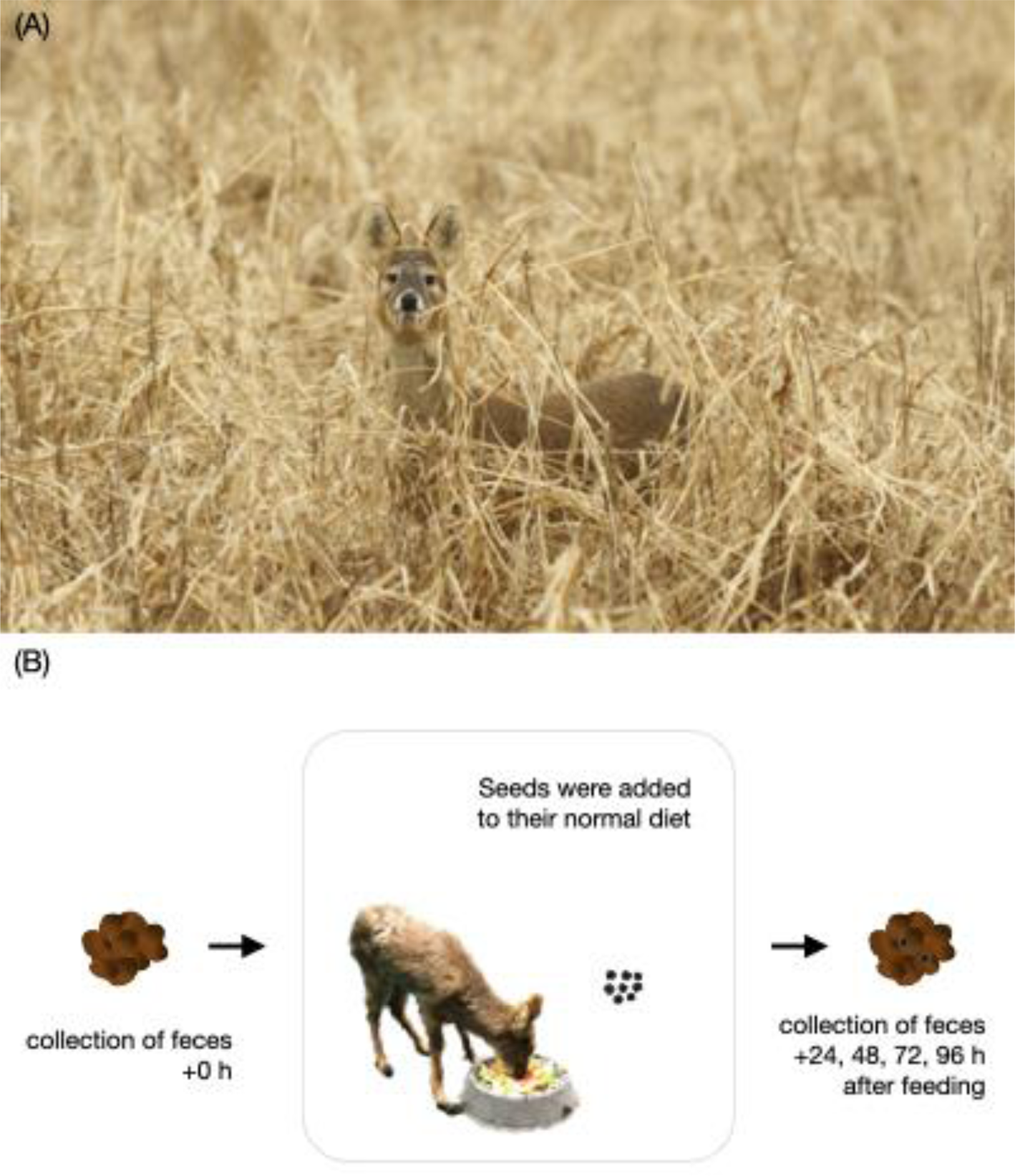
Fig. 1. (A) Korean water deer (Hydropotes inermis argyropus) in its natural habitat (photo credits: Dr. Donguk Han). (B) Procedure of feeding experiments.
Germination test
After counting the seeds retrieved from faeces, the germination rate of seeds with (0–24, 24–48 and 48–96 h) and without gut passage (uningested seeds used as control) was examined. The seeds from the 48–72 and 72–96 h defaecation period were pooled together for further examination because of the low seed recovery in both time intervals. Due to a low recovery rate for some species, the germination of only four species, that is P. bisulcatum, C. album, A. mangostanus and P. oleracea, was evaluated. Because of the low numbers of P. oleracea seeds in every time interval, seeds from all time intervals were pooled together and treated as ingested seeds. All seeds tested for germination were sterilized with 1% sodium hypochlorite solution for 10 min. Then, the seeds were thoroughly washed with distilled water more than three times. The Petri dishes were sterilized with 70% ethanol to avoid possible fungal contamination, and 1 mL of benomyl was added to every Petri dish every week using benomyl wettable powder (Farm Hannong Benomyl, Farm Hannong Co. Ltd., Korea) at a 10 g:20 l ratio. For all plant species, except P. oleracea, 25 seeds were placed in Petri dishes, with five replicates in each treatment (seeds with gut passage after 0–24, 24–48 and 48–96 h, and seeds without gut passage, resulting in a total of four treatments). A total of 125 seeds were tested in each treatment. A total of 375 seeds with gut passage and 125 seeds without gut passage (control) were tested for germination. However, for P. oleracea, 20 seeds and three replicates were tested for germination because of the number of available seeds. Thus, 60 seeds were tested for germination with gut passage, and 60 seeds without gut passage. The Petri dishes containing the seeds were placed in a growth chamber (HB-301 L Hanbaek Scientific Co., Korea) at 25/15°C (day/night), with a 12-h photoperiod. The Petri dishes were randomly displaced every 3 d to reduce the variations due to the effects of light and microclimate in the chamber. The filter papers were kept moist, and the germination rate was determined once a day for 30 d.
Data analyses
Before the statistical tests, the data were checked for normality and homogeneity of variance. For data with normal distribution and homoscedasticity, statistical significances were tested with analysis of variance (ANOVA), followed by Tukey's HSD test. Data with no normal distribution were analysed with non-parametric tests (Kruskal–Wallis test and Wilcoxon rank-sum test). The differences in seed hardness and seed mass per 1000 seeds among species were statistically tested using a Kruskal–Wallis test, followed by multiple comparisons using the functions in R package ‘pgirmess’. The seed recovery rate was compared using ANOVA, followed by Tukey's HSD test. The germination rates of ingested and non-ingested seeds were tested using the Wilcoxon rank-sum test. The germination rate of seeds defaecated in 0–24, 24–48 and 48–96 h and that of control seeds without gut passage were tested using the Kruskal–Wallis test. The statistical significance was determined at P < 0.05. To determine the overall effects of gut passage on seed recovery and germination, the mean recovery rate was multiplied by the mean germination rate, as described by Mancilla-Leytón et al. (Reference Mancilla-Leytón, González-Redondo and Vicente2013). To test the correlation between the recovery rate and seed traits (seed length, seed mass and seed hardness), we used Pearson's correlation coefficients. Graphs were drawn with the ‘ggplot2’ package (Wickham, Reference Wickham2016). All analyses were performed using R version 3.6.0 (R Core Team, 2020).
Results
Seed traits
Seed hardness was the highest for P. bisculcatum (mean, 40.97 N), followed by A. mangostanus (mean, 36.93 N), C. album (mean, 36.43 N) and P. oleracea (mean, 16.60 N). Seed mass was also higher for P. bisulcatum (0.87 g per 1000 seeds) and S. americanum (0.84 g per 1000 seeds) and significantly different from P. oleracea (Table 1).
Recovery rate
Among the eight plant species, six were successfully recovered following gut passage by Korean water deer. The seed recovery rate was significantly different among the species based on ANOVA and Tukey's HSD test (P < 0.05; Fig. 2). P. bisculcatum (33.7%) had the highest recovery rate, followed by A. mangostanus (24.5%), C. album (14.4%), P. oleracea (2.3%) and S. americanum (1.1%). For P. asiatica, only one seed was found in the 48–72 h interval. D. ciliaris and C. bursa-pastoris were well ingested by the deer, but no seeds were found in the faeces.

Fig. 2. Seed recovery rate (proportion of seeds retrieved following gut passage) of each species fed to the Korean water deer. Species with >1% recovery are shown in the graph. Significant differences in total seed recovery rate among plant species are shown with different letters (Tukey's HSD test, P < 0.05).
For the defaecation time pattern, more than 50% of seeds were recovered between 24 and 48 h after feeding for three main species (A. mangostanus, C. album and P. bisculcatum), with a significant recovery rate (Fig. 2).
Seed recovery rate and seed traits were not significantly correlated (Pearson's correlation coefficient; P > 0.05), whereas seed recovery rate and seed hardness (Pearson's correlation coefficient; r = 0.90, P = 0.10) showed a positive trend.
Germination rate after gut passage
P. bisulcatum seeds with gut passage had a significantly lower germination rate (Wilcoxon rank-sum test; P < 0.05). However, the germination rate of seeds with and without gut passage did not differ significantly in A. mangostanus, C. album and P. oleracea based on the Wilcoxon rank-sum test (P > 0.05; Fig. 3A). For both C. album and P. bisulcatum the seeds defaecated in the 24–48 h interval had the highest germination rate, even if it was not always significantly different from all other intervals (Fig. 3B).

Fig. 3. Seed germination percentage in each species. (A) Species with gut passage (gut; 0–96 h after the feeding) and without gut passage (con; control) were tested for germination. Significant differences based on the Wilcoxon rank-sum test are shown (*, P < 0.05; n.s., P > 0.05). (B) The percentages of seed germination of each species with gut passage (0–24, 24–48 and 48–96 h after feeding) and without gut passage (con; control) were tested for germination. Different letters within species indicate the significant differences among treatments based on multiple comparisons after the Kruskal–Wallis test (P < 0.05). A. mangostanus were not statistically different (P > 0.05; Kruskal–Wallis test). The error bar indicates the standard deviation.
From the global effects of gut passage on seed recovery and germination of seeds with and without gut passage, the germination rate of most species, except for A. mangostanus, decreased substantially after passing through the gut of the Korean water deer (Table 2).
Table 2. Overall effects of gut passage on seed recovery and germination calculated by multiplying the mean recovery rate with the mean germination rate of seeds after passing through the gut of Korean water deer (gut passage) and uningested seeds (control)

The number in the bracket indicates the absolute number of seeds that germinated and were tested for germination in each treatment.
Discussion
From the feeding experiments, the efficiency of endozoochorous seed dispersal by Korean water deer could be described. Among the eight plant species, intact seeds of six species were recovered after passing through the gut. D. ciliaris and C. bursa-pastoris were totally consumed by deer. The highest recovery rate was about 30% for P. bisculcatum, while the other seeds had lower recovery rates. Recovery rates often seem to differ among plant species used in feeding experiments. For example, the recovery rate after goat gut passage differed among plant species: 2–7% for legume and grass species (Grande et al., Reference Grande, Mancilla-Leytón, Vicente and Delgado-Pertíñez2016), 28–61% for cacti (Baraza and Fernandez-Osores, Reference Baraza and Fernandez-Osores2013) and <30% for shrubs (Mancilla-Leytón et al., Reference Mancilla-Leytón, Fernández-Alés and Vicente2011). Hard-coated seeds, small-sized seeds and round-shaped seeds are likely to survive from gut ingestion to defaecation (Pakeman et al., Reference Pakeman, Digneffe and Small2002; Mouissie et al., Reference Mouissie, Vos, Verhagen and Bakker2005b). Plant species with these characteristics are likely to survive both mastication and harsh gut environment (Picard et al., Reference Picard, Chevalier, Barrier, Boscardin and Baltzinger2016). These seed traits were not significantly correlated with seed recovery rate due to the limited number of species we tested. However, the correlation between the seed recovery rate and seed hardness had a positive trend, and the seeds that possess the highest seed hardness (P. bisulcatum) were recovered with the highest rate. From this, we carefully presume that seed hardness could be a factor that contributes to the successful retrieval of seeds from passing through the deer gut, as discussed in other literatures (Gardener et al., Reference Gardener, Mcivor and Jansen1993; Mancilla-Leytón et al., Reference Mancilla-Leytón, González-Redondo and Vicente2013). Furthermore, D'hondt and Hoffmann (Reference D'hondt and Hoffmann2011) suggested that seed hardness, which can be seen as the water impermeability of seeds, is the main trait for seeds to efficiently survive digestion.
Most of the seeds were recovered between 24 and 48 h after ingestion by deer. This pattern was similar to that observed for sheep and goats. Approximately 40–55% of seeds were retrieved in 24–48 h after feeding them to goats (ca 35 kg) (Abbas et al., Reference Abbas, Al-Kahtani, Mousa, Badry, Hassaneen, Ezzat-Ahmed, Mancilla-Leyton and Castillo2020). For Kazakh sheep (ca 42 kg), > 80% of seeds were retrieved 24–48 h after feeding (Wang et al., Reference Wang, Lu, Waly, Ma, Zhang and Wang2017). The highest recovery was 24–72 h after feeding goats (ca 40 kg) in the study of Grande et al. (Reference Grande, Mancilla-Leytón, Vicente and Delgado-Pertíñez2016). Although the body mass of Korean water deer (~13 kg) is lighter than that of the sheep and goats, they had similar retention times. The gut passage time depends on several factors, such as body mass (Illius and Gordon, Reference Illius and Gordon1992). However, in some studies, there was no relationship between body mass and gut retention time (Schwarm et al., Reference Schwarm, Ortmann, Wolf, Jürgen Streich and Clauss2008; Steuer et al., Reference Steuer, Südekum, Müller, Franz, Kaandorp, Clauss and Hummel2011). As discussed by Picard et al. (Reference Picard, Papaïx, Gosselin, Picot, Bideau and Baltzinger2015), the ruminant feeding type is related to gut retention time, as they require minimal time to ingest and defaecate the seeds. The seed retention time also changes according to diet quality. A high-quality diet may result in fast digestion, but with a low-quality diet, the diet ingestion time will be much longer (Holand, Reference Holand1994). The Korean water deer is a browser, which mainly feeds on plant leaves in its natural habitat (Kim et al., Reference Kim, Lee and Lee2011). They are likely to feed on fresh leaves in spring/summer and wood twigs and bark during winter (Kim et al., Reference Kim, Lee and Lee2011; Kim, Reference Kim2015). This seasonal change in diet quality might affect the retention of seeds in the gut, as there is more high-quality food consumption in spring and summer than in winter.
After gut passage, the germination rate of seeds was not different from that of uningested seeds for A. mangostanus, C. album and P. oleracea. This was also in agreement with the general pattern after their passage through the gut of the family Cervidae, with non-significant lower seed germination rate (Torres et al., Reference Torres, Castano and Carranza-Quiceno2020). After passing the gut, seed recovery and germination rate decreased for most species. This conformed to the seed survival rate of C. album seeds fed to cattle, which reduced after the gut passage (D'hondt and Hoffmann, Reference D'hondt and Hoffmann2011). When we looked at the seed germination according to the time interval, the germination rate of A. mangostanus and C. album seeds in the 24–48 h interval increased after gut passage, but it was not statistically significant. The retention time in the animal gut is a factor affecting seed germination (Traveset et al., Reference Traveset, Robertson, Rodríguez-Pérez, Dennis, Schupp, Green and Westcott2007; Jaganathan et al., Reference Jaganathan, Yule and Liu2016). Seeds with impermeable coats (i.e. physically dormant seeds) can successfully survive gut passage by animals (Jaganathan et al., Reference Jaganathan, Yule and Liu2016). Overall, although it was not statistically significant, the seeds defaecated within the 24–48 h interval had the highest germination rate in this study. Jaganathan et al. (Reference Jaganathan, Yule and Liu2016) postulated that physically dormant seeds defaecated in an optimal time to break the dormancy could successfully germinate; however, some seeds can still be defaecated as dormant seeds, and those defaecated over the optimal time to break the dormancy become permeable, which results in seed death. These trends were found for C. album. However, with the gut passage, the germination rate of P. bisulcatum was hampered; non-dormant seeds or soft-coated seeds may have hampered the germination rate (Yu et al., Reference Yu, Xu, Wang, Shang and Long2012; Wang et al., Reference Wang, Lu, Waly, Ma, Zhang and Wang2017). For future studies, the type of seed dormancy should be considered to fully understand endozoochorous seed dispersal.
After the gut passage, several other factors induce seed mortality. The seeds sown in herbivore dung had a decreased germination rate (Milotić and Hoffmann, Reference Milotić and Hoffmann2016a). Seeds also encounter seedling competition within or between species (Milotić and Hoffmann, Reference Milotić and Hoffmann2017). Additionally, after endozoochorous seed dispersal, the germination in natural conditions might also affect the seed germination success (Milotić and Hoffmann, Reference Milotić and Hoffmann2016a; Karimi et al., Reference Karimi, Hemami, Esfahani and Baltzinger2020). Meanwhile, the seedlings had a higher increase in biomass and height, as dung can supply the nutrients (e.g. N and P) for their growth compared to the substrate of vermiculite or sand-compost (Traveset et al., Reference Traveset, Bermejo and Willson2001; Mancilla-Leytón et al., Reference Mancilla-Leytón, Fernández-Alés and Vicente2012; Milotić and Hoffmann, Reference Milotić and Hoffmann2016b). A study reported that the faeces of Korean water deer likely act as a fertilizer by providing nutrition for the growth of Zea mays (Park and Lee, Reference Park and Lee2014). This can relate to the potential advantage of endozoochorously dispersed seeds by deer. Thus, in this sense, a low percentage of successful germination after the gut passage can consequently benefit the plant species dispersed endozoochorously. Further studies on how other factors affect seedling mortality are needed to comprehensively understand seed dispersal effectiveness.
Overall, endozoochorous seed dispersal by Korean water deer seems unbeneficial and has a high cost for plant species, as the germination rate decreases after the gut passage. However, as the home range of the deer is reported to be 2.77 km2 (Kim and Lee, Reference Kim and Lee2011), as they move around, they can allow long-distance seed dispersal for the few seeds able to germinate. The deer inhabit the Korean peninsula with a growing population (Kim et al., Reference Kim, Lee and Kim2016; National Institute of Biological Resources, 2018). Lee and Lee (Reference Lee and Lee2020) showed that, on average, eight seeds germinated per 100-g dung (with an average dung mass of 6.3 g). Because of their abundance in Korea, they can disperse daily numerous seeds from different species. Through endozoochory, deer can affect vegetation dynamics by maintaining plant populations, contributing to plant communities and colonizing new habitats. Therefore, when planning to manage and conserve the deer population, their role as seed dispersal vector must be considered, as they can potentially consume and disperse seeds over long distances, even at the high cost of low seed recovery and germination rate.
Supplementary material
To view supplementary material for this article, please visit: https://doi.org/10.1017/S0960258521000246.
Acknowledgements
We thank the members of the Wildlife Rescue Center of Kangwon National University for helping us with the feeding experiment, Nayoung Ahn for seed preparation and Jaesang Jung for help with the preparation of the germination test. We also thank the three Korean water deer who ate the seeds for the experiment. This work was partly supported by the National Research Foundation of Korea (NRF) grant funded by the Korean government (MSIT) (No. 2017R1A2B4006761). The authors also wish to thank the anonymous referees for the constructive and helpful comments on the revision of this article.
Conflicts of interest
The authors declare no conflict of interest.