Introduction
Physical dormancy (PY) is caused by one or more water-impermeable palisade cell layer(s) in seed (or fruit) coats which prevents imbibition and, consequently, the onset of germination (Rolston, Reference Rolston1978; Baskin and Baskin, Reference Baskin and Baskin1998; Baskin et al., Reference Baskin, Baskin and Li2000; Baskin and Baskin, Reference Baskin and Baskin2004), due the compact structure and presence of hydrophobic substances (Rangaswamy and Nandakumar, Reference Rangaswamy and Nandakumar1985; Manning and Van Staden, Reference Manning and Van Staden1987; Kelly et al., Reference Kelly, Van Staden and Bell1992; Serrato-Valenti et al., Reference Serrato-Valenti, Cornara, Ghisellini and Ferrando1994; Venier et al., Reference Venier, Funes and García2012). PY enables seeds to remain viable for long periods of time and allows formation of soil seed banks, which can persist during unfavourable conditions for seedling establishment (Baskin and Baskin, Reference Baskin and Baskin1998). Under natural conditions, seed dormancy break may be influenced by temperature variation, associated with either seasonality (Van Assche et al., Reference Van Assche, Debucquoy and Rommens2003) or forest openings (Vázquez-Yanes and Orozco-Segovia, Reference Vázquez-Yanes and Orozco-Segovia1982), and by environmental disturbances, such as fire (Keeley and Fotheringham, Reference Keeley, Fotheringham and Fenner2000), due to stimulation of sites for water uptake in seeds.
Specialized sites where water enters the seed (water gaps) control seed dormancy, responding to environmental signals which allow the seeds to germinate only under specific conditions (Baskin et al., Reference Baskin, Baskin and Li2000; Baskin, Reference Baskin2003). Once opened, water gaps cannot be closed (Baskin, Reference Baskin2003), i.e. if water is available, it can reach the inner tissues of the seed at any time after opening. Water gaps vary in structure, determining various ways of action by which water enters the seed, as can be observed in species from Fabaceae and other families (Dell, Reference Dell1980; Hanna, Reference Hanna1984; Manning and Van Staden, Reference Manning and Van Staden1987; Lersten et al., Reference Lersten, Gunn and Brubaker1992; Baskin et al., Reference Baskin, Baskin and Li2000; Jayasuriya et al., Reference Jayasuriya, Baskin, Geneve and Baskin2007, Reference Jayasuriya, Baskin, Geneve, Baskin and Chien2008, Reference Jayasuriya, Baskin, Geneve and Baskin2009; Turner et al., Reference Turner, Cook, Baskin, Baskin, Tuckett, Steadman and Dixon2009; Gama-Arachchige et al., Reference Gama-Arachchige, Baskin, Geneve and Baskin2010, Reference Gama-Arachchige, Baskin, Geneve and Baskin2013). Thereby, Gama-Arachchige et al. (Reference Gama-Arachchige, Baskin, Geneve and Baskin2013) identified three basic types of water gaps according to their structure, which can be simple or compound, depending on the number of openings involved in initial water uptake, and the micropyle and hilum can act as a secondary opening. Another seed structure, the pleurogram or fracture line, is a specialized structure that can act as a hygroscopic valve (similar to the hilum) and which plays a role in the drying out of the seed at the end of seed maturation (Gunn, Reference Gunn, Polhill and Raven1981). In some species, a change in colour and texture occurs in the pleurogram region (Werker, Reference Werker1997), which may also be involved in seed imbibition.
The Fabaceae family is one of the most representative plant families, with great ecological and economic importance, and the occurrence of PY in this family is frequent. In leguminous seeds, the lens is one such specialized site for water uptake, in addition to the hilum and micropyle, which are also important for imbibition in seeds of some species (Hanna, Reference Hanna1984; Rangaswamy and Nandakumar, Reference Rangaswamy and Nandakumar1985; Manning and Van Staden, Reference Manning and Van Staden1987; Serrato-Valenti et al., Reference Serrato-Valenti, De Vries and Cornara1995; Baskin et al., Reference Baskin, Baskin and Li2000, De Paula et al. Reference De Paula, Delgado, Paulilo and Santos2012). However, the water gap of seeds with PY is still not well understood, and the lens may not be the main site of water entrance in seeds (Rolston, Reference Rolston1978; Lersten et al., Reference Lersten, Gunn and Brubaker1992; Morrison et al., Reference Morrison, McClay, Porter and Rish1998; De Paula et al. Reference De Paula, Delgado, Paulilo and Santos2012). Moreover, studies of species from the subfamily Caesalpinioideae are scarce and a greater understanding of the morphology and anatomy of the water gap is needed to identify the mechanism involved in seed dormancy.
Senna multijuga (Rich.) H.S. Irwin & Barn. (Fabaceae: Caesalpinioideae) is a widely distributed tree species in Brazil. It is classified as a pioneer species and can reach up to 10 m in height (Lorenzi, Reference Lorenzi1992; Carvalho, Reference Carvalho2004). Seeds are oblong and moss-green to greenish-brown in colour (Amorim et al., Reference Amorim, Davide, Ferreira and Chaves2008). S. multijuga has been used successfully in reforestation of degraded areas (Lorenzi, 1992) and can establish soil seed banks (Ferreira et al., Reference Ferreira, Davide and Motta2004). Due to the importance of understanding the biology of PY, the aims of this study were to: (1) characterize the PY break; (2) examine the role of different seed structures in water uptake; and (3) identify the water gap of S. multijuga seeds.
Materials and methods
Seed collection
Seeds were collected in September 2011 from S. multijuga trees at Universidade Federal de Lavras, Lavras, Brazil (21°14′S; 45°0′W, 900 m elevation). Ecologically, the region is characterized as a transition zone between Atlantic Forest and Cerrado. Seeds were manually separated by flotation in water, dried in a climate-controlled chamber [20°C, 60% relative humidity (RH)] for 2 d and stored in sealed semipermeable plastic bags in a cold chamber (5°C, 40% RH) until the beginning of the experiment. The average water content of seeds was 8.4% and experiments were initiated within 1 week from the collection date.
Dormancy breaking and germination
Intact seeds were subjected to two dormancy breaking treatments: (1) immersion in water at 80°C initial temperature for 12 h; and (2) immersion in water at 100°C initial temperature for 12 h. A group of control seeds was not subjected to any of the dormancy breaking treatments. Seeds were then placed into Petri dishes with moist germination paper and kept at 25°C under constant light for 30 d to evaluate germination. Germination percentage, germination speed index (GSI) (Maguire, Reference Maguire1962) and percentage of normal seedlings were determined. Four replicates of 25 seeds were utilized for each treatment. The criterion for assessing germination was protruded primary root ≥ 1 mm.
Imbibition curve
Water absorption by dormant and non-dormant seeds was quantified. Twenty replicates of one intact seed were placed into Petri dishes with moist germination paper at 25°C under constant light and weighed consecutively using a precision scale. Prior to each weighing, superficial water was removed from the seeds using absorbent paper. To evaluate the imbibition pattern of non-dormant seeds, twenty replicates of one non-dormant seed were placed into Petri dishes with moist germination paper at 25°C under constant light and weighed consecutively. Seed fresh mass was weighed until the primary root reached 3 mm in length (for non-dormant seeds). Dormancy breaking treatment involved immersion in water at 80°C and subsequent cooling to room temperature. Seeds remained submerged for 12 h during treatment and fresh mass was determined from the start of submersion in hot water.
Morphological changes in seeds during breaking of dormancy and germination
Seeds subjected to the dormancy breaking treatment (water at 80°C initial temperature for 12 h) and germination conditions (Petri dishes with moist germination paper at 25°C under constant light) were observed and documented at intervals of 1 h until 12 h of imbibition and every 12 h from 12 h until 48 h of imbibition, using a stereomicroscope (Olympus Micronal VMT; Olympus, São Paulo, Brazil) with a digital camera (Benq DC E1030; Benq, São Paulo, Brazil). Images were recorded of the hilar and extra-hilar regions. Morphological changes were recorded sequentially from the dormant stage until the protrusion of the primary root, to identify the water gap and changes during germination (Jayasuriya et al., Reference Jayasuriya, Baskin, Geneve and Baskin2007; Turner et al., Reference Turner, Cook, Baskin, Baskin, Tuckett, Steadman and Dixon2009; Gama-Arachchige et al., Reference Gama-Arachchige, Baskin, Geneve and Baskin2010).
Blocking of the water gap
Approximately 500 non-treated seeds were placed into Petri dishes with moist paper for 3 d (time needed to reach 100% imbibition or germination, as observed during germination tests) at 25°C, in order to identify the impermeable (dormant) seeds. To identify the water gap, those seeds were distributed into three treatment groups: blocking of lens (1), micropyle (2) or whole seed (3) using Super Bonder® instant adhesive (cyanoacrylate ester) (based on Jayasuriya et al., Reference Jayasuriya, Baskin, Geneve and Baskin2007; Turner et al., Reference Turner, Cook, Baskin, Baskin, Tuckett, Steadman and Dixon2009; Gama-Arachchige et al., Reference Gama-Arachchige, Baskin, Geneve and Baskin2010). Seeds were maintained at ambient conditions in the laboratory for 12 h to allow the adhesive to dry and then treated with hot water at 80°C initial temperature for 12 h. As a control, a fourth treatment was applied, with unblocked seeds, which were also subjected to the same dormancy breaking treatment. All seeds were placed into Petri dishes on moist paper at 25°C under constant light, with 4 replicates of 25 seeds per treatment. Imbibed seeds were counted in all treatments for 3 d. Seeds were considered imbibed when the seed coat swelled, resulting in a change in colour and size.
Water absorption by seed parts
Seeds were subjected to dormancy breaking treatment (water at 80°C initial temperature for 12 h), during which water absorption by their parts was evaluated (after 30 min, 1 h, 3 h, 6 h and 12 h). Seeds were then divided into three parts for quantifying water content (International Seed Testing Association, 2004): hilar region (comprising the hilum, micropyle and lens, up to the limit of the pleurogram); pleurogram region (and the area that it encloses); and distal region (the part of the seed opposite the hilar region, below the pleurogram) (Fig. 1). Four replicates of 20 seeds per treatment were used.

Figure 1 Schematic diagram of a Senna multijuga seed. Sections show the parts of the seed used to quantify water absorption by different seed parts. DR, distal region; HI, hilum; HR, hilar region; LE, lens; MI, micropyle; PL, pleurogram; PR, pleurogram region.
Seed coat structural characteristics
Non-dormant seeds (using the same method of dormancy breaking as the previous assay), were fixed with Karnovsky solution (Karnovsky, Reference Karnovsky1965) for 24 h and stored in 70% ethanol. Seeds were then further dehydrated in a graded ethanol series and then infiltrated with and embedded in hydroxyethyl methacrylate (Leica®, São Paulo, Brazil) according to the manufacturer's instructions. Seed material was sectioned (2–5 μm) transversally and longitudinally using a sliding microtome, stained with toluidine blue 0.05% (O'Brien and McCully, Reference O'Brien and McCully1981) and mounted in synthetic resin. Seed sections were observed and documented using an optical microscope (Carl Zeiss Primo Star; Carl Zeiss, São Paulo, Brazil), and images of the sections were recorded with a digital camera (Axiocam Erc 5S; Axiocam, São Paulo, Brazil). To evaluate morphological changes in seed structures over the dormancy breaking process, both dormant and non-dormant seeds were used. To avoid damage during sample preparation, samples were directly mounted on stubs and sputter-coated with gold (Bal-Tec, São Paulo, Brazil) and then observed with a scanning electron microscope (SEM) LEO EVO 40 XVP (São Paulo, Brazil).
Statistical analysis
The experimental design was completely randomized. Germination, normal seedling and GSI data were analysed using a generalized linear model (GLM) followed by a Tukey test (P ≤ 0.05). The data from the experiment of blocking the water gap were subjected to analysis of variance (ANOVA) followed by a Tukey test (P ≤ 0.05), performed on the arcsin√x/100 transformed data. R 2.12.0 (R Development Core Team, 2011) software was used for all these statistical tests. To analyse the data of the imbibition curve and water absorption by seed parts, a regression analysis was performed, and the fit of the model was evaluated using the coefficient of determination (R 2) (P ≤ 0.05) using SigmaPlot 11.0 software (Systat Software Inc., San José, California, USA).
Results
Dormancy breaking and germination
The use of water with an initial temperature of 80°C or 100°C was an efficient method to break dormancy of S. multijuga seeds. Germination mostly occurred up to the fifth day of evaluation, after which it stabilized (Fig. 2). The treatments did not cause damage to the embryo, thus enabling a high percentage of normal seedlings (Fig. 3A), and GSI values significantly higher than those of the control (Fig. 3B). The percentage of seeds that remained hard following the evaluation period was 90, 10 and 2% for the control treatment, and immersion in water at 80°C and 100°C, respectively.
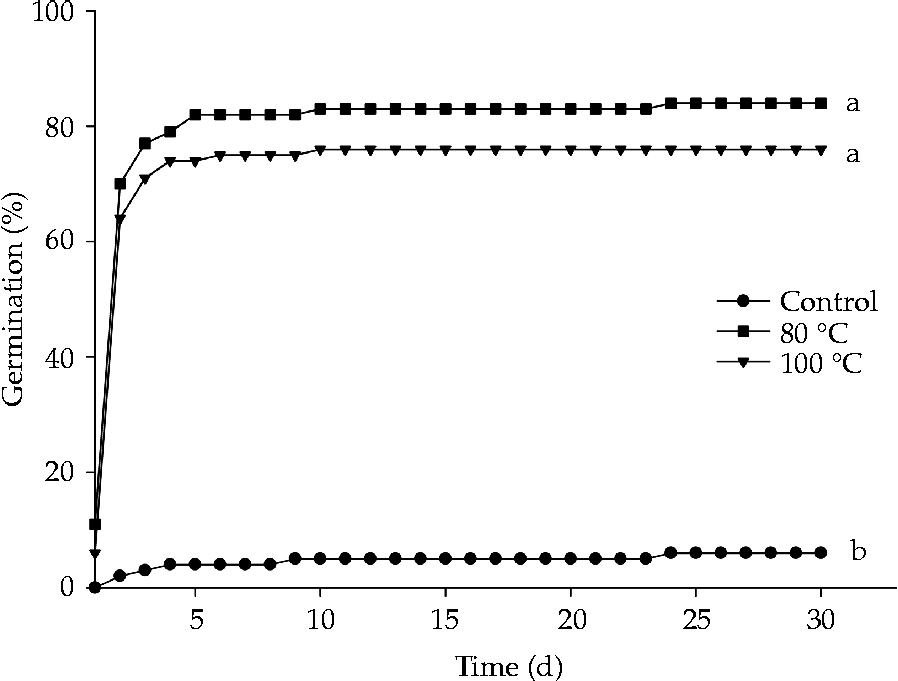
Figure 2 Effect of the dormancy breaking method on seed germination of Senna multijuga. The same letters indicate no significant differences, according to a Tukey test (P ≤ 0.05).

Figure 3 Effect of the dormancy breaking method on percentage of normal seedlings (A) and germination speed index (GSI) (B) for Senna multijuga seeds (average ± standard deviation). The same letters indicate no significant differences, according to a Tukey test (P ≤ 0.05).
Imbibition curve
No increase in fresh mass was observed in dormant seeds, which maintained approximately the same mass throughout the experiment. Imbibition of non-dormant seeds occurred in three phases: a rapid increase in water absorption until approximately 12 h of imbibition (Phase I), seed fresh mass stabilization until 32 h of imbibition (Phase II) and a second period of rapid increase in water absorption following primary root protrusion (Phase III) (Fig. 4).

Figure 4 Water absorption of dormant and non-dormant Senna multijuga seeds (average ± standard deviation). The arrow indicates 50% germination.
Morphological changes in seeds during dormancy breaking and germination
Dry seeds had a hard and opaque seed coat with a greenish-brown colour (Fig. 5A, F). After 3 h of imbibition, seeds changed colour and became orange-brown as a result of swelling of the seeds from dormancy breaking and imbibition. During this period, half of the seed showed characteristics of an imbibed seed and the other half showed characteristics of a dry seed, with pleurogram deformation (Fig. 5B, G). The water gap was located at the hilar region, close to the seed coat bulge formed by the positioning of the embryo axis. The hilar region showed a different colour than the rest of the seed coat when the seed was imbibed (Fig. 5B, C, D). After 12 h of imbibition, seeds were uniform in colour and had increased in size (Fig. 5C, D, H). The seeds maintained this appearance until the protrusion of the primary root, which occurred after 48 h of imbibition with the rupture of the bulge region (Fig. 5E, I).

Figure 5 (Colour online) Morphological changes in Senna multijuga seeds during germination. Frontal (A–E) and lateral view (F–I) of the seed. (A) Dry seed; (B) seed imbibed for 3 h, with arrow indicating the limit of swelling; (C) seed imbibed for 12 h, with arrow indicating the hilar region with a different colour from the extra-hilar region; (D) seed imbibed for 24 h, with arrow indicating the bulge region; (E) germinated seed, with arrow indicating the rupture of the bulge region; (F) hilar region of a dry seed; (G) hilar region of a seed imbibed for 3 h, with arrow indicating partial swelling; (H) hilar region of fully imbibed seed; (I) root protrusion with rupture of the bulge region. AR, areola; BU, bulge; HI, hilum; PL, pleurogram; PR, primary root). Scale bar = 1 mm.
Blocking of the water gap
The percentage of imbibed seeds was the same for both seeds blocked at the micropyle and unblocked seeds. After 1 d under germination conditions, the maximum percentage of imbibed seeds was reached, 94 and 96% for seeds with blocked micropyle and unblocked seeds, respectively. Blocking the lens prevented imbibition, with the percentage of imbibed seeds being the same for those with a blocked lens and the fully blocked seeds. The comparison of seeds with a blocked lens and seeds with a blocked micropyle clearly showed the role of the lens in water uptake in S. multijuga seeds (Fig. 6). The percentage of seeds with a blocked lens that imbibed after 3 d under germination conditions was only 12% and was equal to the percentage of fully blocked seeds that were imbibed (14%). Therefore, the imbibition of some seeds with a blocked lens was due to some failure of the blocking method for those seeds and not the absorption of water by other seed sites.
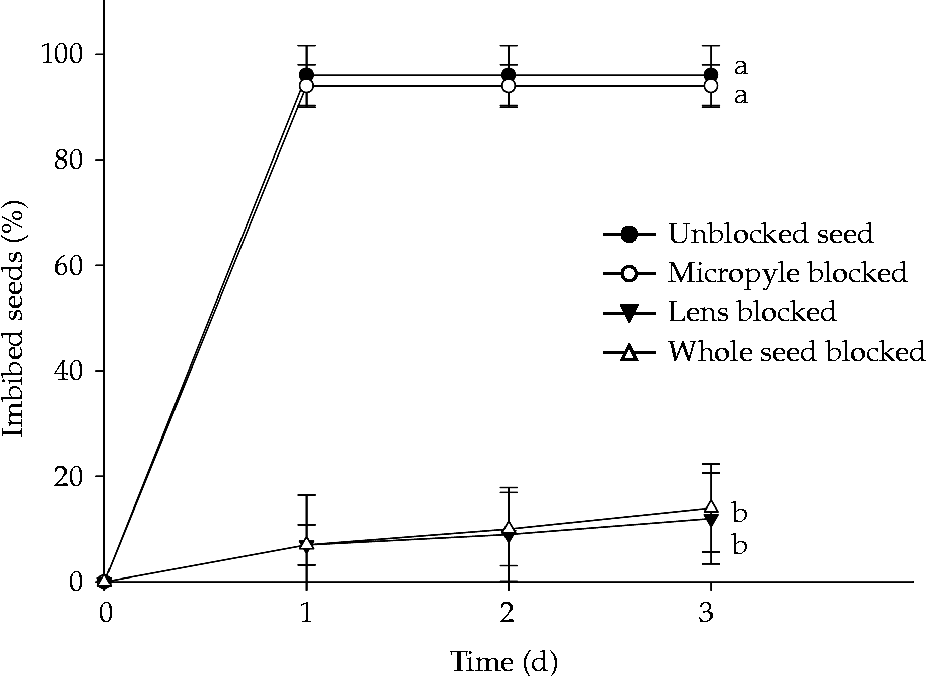
Figure 6 Percentage of imbibed Senna multijuga seeds (average ± standard deviation) for unblocked seeds and seeds with the micropyle, lens and whole seed blocked after 3 d under germination conditions at 25°C, following dormancy break.
Water absorption by seed parts
Dry seeds had a water content of about 10%. Following 30 min of imbibition, water content in the hilar region increased to approximately 15%, whereas in the other regions water content remained the same as that of the dry seeds. After 1 h of imbibition, water content increased to 28% in the hilar region and 13.3% in the pleurogram region, but there were no changes in water content of the distal region. After 3 h of imbibition, water content in the hilar and pleurogram regions was above 35%, and water content in the distal region began to increase (to approximately 13%). After 6 h of imbibition, all seed parts had a large increase in water content, reaching 54%, 55% and 39% water content in hilar, pleurogram and distal regions, respectively. After 12 h of imbibition, water content was similar for all seed parts (Fig. 7). Changes in water content of the seed parts corresponded with the observed morphological changes (Fig. 5). Water content was similar in whole seed and in pleurogram because this region comprises most of the seed. Whole-seed water content was 9.8, 10.6, 15.1, 34.6, 53 and 61.4% for the sequence of imbibition periods (Fig. 7).

Figure 7 Water absorption at different sites on Senna multijuga seeds following dormancy break (average ± standard deviation).
Seed coat structural characteristics
S. multijuga seeds have a testa with a linearly aligned micropyle, hilum and lens (Fig. 8A). The seed coat has a layer of macrosclereids with a thick cuticle (exotesta) and a mesotesta composed of parenchyma cell layers with thick walls, limited externally and internally by a layer of osteosclereids and more internally by an endotesta with a layer of cells pressed together with tegmen remains (Fig. 8B). At the mesotesta, there is a single vascular bundle irrigating the raphe–antiraphe region (Fig. 8A).

Figure 8 (Colour online) Cross-sections (B, D, E, G–I) and longitudinal sections (A, C, F) of Senna multijuga seeds. (A) Seed coat with linearly aligned lens, funiculus and micropyle, with arrows indicating the vascular bundle (white arrow) and osteosclereids (black arrow). (B) Detailed image of the seed coat and endosperm in the extra-hilar region. (C–E) Detail of the lens region. Arrows in (E) indicate the fragile region of the macrosclereid layer, where seed coat rupture occurs. (F) Seed showing the location of micropyle and funiculus. (G) Detailed image of the micropyle region, showing the opening that runs along the entire seed coat. (H) Seed coat at the pleurogram region, showing displacement at the height of the light line of macrosclereids and the cuticle. (I) Detailed image of the light line, shown by the arrow. CT, cuticle; EN, endosperm; FU, funiculus; LE, lens; LL, light line; MC, macrosclereid cells; MI, micropyle; OS, osteosclereids; PA, parenchyma; PL, pleurogram; VB, vascular bundle; WH, white cells. Scale bars = 500 μm (A), 200 μm (F, G), 100 μm (D), 50 μm (B, C, E), 40 μm (H), 20 μm (I).
The lens appears as a protuberance immediately below the hilum (Fig. 8A, C, D, E). In the hilar region, no osteosclereids are observed immediately under the macrosclereids (Fig. 8C, D, E). In this region, the seed coat has only one layer of osteosclereids, above the endotesta (Fig. 8A, D). A group of parenchyma cells with thickened walls and irregular shape (white cells) can be observed under the macrosclereid cells (Fig. 8C, D, E). In addition, a vascular bundle and typical parenchyma cells with thin walls and hyaline content are found under the white cells (Fig. 8C, D, E). The lens has two fragile regions comprised of shorter macrosclereids (Fig. 8D, E).
In addition to the rupture in the lens region, another seed coat discontinuous region is the micropyle (Fig. 8F, G). A cross-section of this region shows a radial elongation of the macrosclereids (exotesta), a mesotesta with thick-walled parenchyma cells and an endotesta with papilla-shaped cells bordering the opening that connects the external and internal tissues (Fig. 8G). The hilum is a scar on the seed coat left by the funiculus, with elongated macrosclereids and several layers of parenchyma cells with thickened walls underneath them (Fig. 8A, F). The pleurogram region has a structure similar to that of the rest of the seed coat and is characterized by displacement of a light line of macrosclereids (Fig. 8H, I).
After only 15 min of exposure to the treatment of dormancy breaking, there was a structural change in the lens that enabled the formation of the water gap, as observed by using SEM (Fig. 9). The lens lost its initial morphology and the internal tissues were exposed, allowing the absorption of water into the seed (Fig. 9A–D). There were no morphological changes in the hilum or micropyle (Fig. 9A, B, E, F). The hilar region showed superficial fissures due to the disruption of the cuticle (Fig. 9A, B), which does not allow water uptake. No other changes were observed in the seeds following 12 h of dormancy breaking. In dormant seeds, several cracks were observed across the pleurogram region (areola) (Fig. 9G), but there was no change in pleurogram morphology after dormancy break, with the pleurogram maintaining its structure (Fig. 9H). These cracks, as previously observed, do not allow the absorption of water (Figs 7 and 8H).

Figure 9 Scanning electron micrographs of dormant (A, C, E, G) and non-dormant (B, D, F, H) Senna multijuga seeds. (A) Hilar region of a dormant seed showing the lens, hilum and micropyle, with arrow indicating the cuticle. (B) Hilar region of a non-dormant seed showing the lens, hilum and micropyle, with changes in lens morphology following the breaking of dormancy, which created an opening for water uptake (arrows). (C) Dormant seed lens. (D) Non-dormant seed lens, showing the opening for water uptake. (E) Micropyle of a dormant seed. (F) Intact micropyle of seed following dormancy break. (G) Seed coat of a dormant seed, evidencing the location of the pleurogram region (areola) with cracks. (H) Seed coat of a non-dormant seed, showing that the pleurogram region remained unchanged, with the arrow indicating the rupture of the cuticle. AR, areola; HI, hilum; LE, lens; MI, micropyle. Scale bars = 200 μm (F, G), 100 μm (A), 20 μm (B, C, D, E).
Discussion
Several methods have been used to break seed PY. Among these methods, the use of hot water appears to be efficient for some species, e.g. Acacia melanoxylon (Burrows et al., Reference Burrows, Virgona and Heady2009), Parkia biglobosa (Aliero, Reference Aliero2004), Cuscuta australis (Jayasuriya et al., Reference Jayasuriya, Baskin, Geneve, Baskin and Chien2008) and Ipomoea lacunosa (Jayasuriya et al., Reference Jayasuriya, Baskin, Geneve and Baskin2007, Reference Jayasuriya, Baskin, Geneve and Baskin2009). According to Taylor (Reference Taylor1981), high temperatures may weaken the lens region, enabling later imbibition of seeds. However, exposure to high temperatures may also damage the seeds of some species, increasing seed mortality and causing the development of abnormal seedlings, as reported by Vari et al. (Reference Vari, Jethani, Sharma, Khanna and Barnwal2007) for Sesbania spp. PY in S. multijuga seeds is confirmed by the fact that high temperature released dormancy and untreated seeds did not imbibe, as reported by other authors (Lemos-Filho et al., Reference Lemos-Filho, Guerra, Lovato and Scotti1997; Carvalho, Reference Carvalho2004; Lacerda et al., Reference Lacerda, Lemos Filho, Goulart, Ribeiro and Lovato2004).
Breaking of dormancy in S. multijuga seeds by immersion in hot water showed that water enters the seeds at the hilar region as a result of stimulation of the lens, as reported by Baskin et al. (Reference Baskin, Baskin and Li2000) for Fabaceae species. De Paula et al. (Reference De Paula, Delgado, Paulilo and Santos2012) also reported the important role of the lens as the water gap in seeds of other species of Senna (S. macranthera). In the family Fabaceae, the palisade layer of the lens region shows anatomical differences from the rest of the seed coat (Dell, Reference Dell1980; Hanna, Reference Hanna1984; Serrato-Valenti et al., Reference Serrato-Valenti, De Vries and Cornara1995, De Souza et al., Reference De Souza, Voltolini, Santos and Paulilo2012), indicating the importance of this region for water absorption into seeds with PY. Structural differences in these regions were observed in S. multijuga seeds, with regions of fragility in the lens (Fig. 8E), forming water gaps.
Among these structural differences in the lens, decreased macrosclereid size in the lens region has been reported previously (Dell, Reference Dell1980; Serrato-Valenti et al., Reference Serrato-Valenti, De Vries and Cornara1995; De Souza et al., Reference De Souza, Voltolini, Santos and Paulilo2012) to result in a higher sensitivity to rupture in that region, which was observed in the fragile areas of lens in S. multijuga seeds. Furthermore, lighter-coloured parenchyma cells (white cells) observed below the lens region may play an important role in lifting the lens region, causing its opening. Serrato-Valenti et al. (Reference Serrato-Valenti, De Vries and Cornara1995) observed cells with hydrophobic substances below the lens region in Leucaena leucocephala seeds, which may influence the lens opening. These cells also showed a different colour than the surrounding cells and were referred to as ‘white cells’ by the authors. The absence of these parenchyma cells in the extra-hilar region may indicate their importance for absorption of water by the seed. Thereby, the role of the parenchyma cells in lifting the lens region and opening the water gap should be further examined to understand how these cells act in breaking PY. Parenchyma cells, instead of osteosclereids, immediately below the macrosclereids in the lens region were also reported by Dell (Reference Dell1980) and Serrato-Valenti et al. (Reference Serrato-Valenti, De Vries and Cornara1995) in seeds of Albizia lophantha and L. leucocephala, respectively.
The opening of the micropyle could enable the late entrance of water into the seed (Fig. 8F, G), but water uptake would be very restricted, and the hilum showed elongated macrosclereids, with no locations for water uptake. Morphological changes following dormancy break were only observed in the lens region, not in any other seed structures. In the rest of the seed coat, cells exhibited a compact structure that did not allow water uptake. The light line of the testa of seeds with PY is composed of hydrophobic substances that contain callose (Serrato-Valenti et al., Reference Serrato-Valenti, Cornara, Ghisellini and Ferrando1994, Reference Serrato-Valenti, De Vries and Cornara1995) and may also play a role in the impermeabilization of S. multijuga seeds, since it is present in the entire seed coat. Even though the pleurogram of S. multijuga seeds was disrupted during imbibition, water had not entered through it as it has a similar anatomy to that of the extra-hilar region. Another characteristic of the pleurogram is the presence of superficial cracks in the areola region, present even in dormant seeds, which do not allow water absorption. This region may play an important role in the drying of seeds during maturation (Gunn, Reference Gunn, Polhill and Raven1981), but does not play a role in water absorption during germination in S. multijuga seeds.
Hu et al. (Reference Hu, Wang, Wu and Baskin2009) reported difficulties in understanding the sites responsible for water absorption by seeds, especially in the identification of the primary sites where imbibition begins. These authors reported that the lens is responsible for initial water absorption in Sesbania sesban seeds, in contrast with Vigna oblongifolia, in which absorption occurs in the hilum region. However, seeds may respond differently to different types of dormancy breaking treatments, which may result in water uptake through different sites (Hu et al., Reference Hu, Wang, Wu and Baskin2009). The use of methods for breaking PY of seeds by stimulating water gaps, such as hot water in S. multijuga seeds, avoids physical damage to the embryo and controls the rate of water uptake, thereby preventing damage to internal tissues, leading to the formation of a healthy seedling.
It is concluded that 15 min of exposure to hot water treatment is sufficient for the formation of the water gap in S. multijuga seeds. No secondary opening is involved in initial water uptake, which was restricted to the lens. Further, a larger lens in comparison to the micropyle leads to a higher efficiency of water use, shortening the germination period.
Acknowledgements
The authors wish to thank Rodrigo A. de Melo for his contribution in preparing the anatomical slides.
Financial support
The authors thank the Laboratório de Microscopia Eletrônica e Análise Ultraestrutural da Universidade Federal de Lavras and FAPEMIG (Fundação de Amparo à Pesquisa do estado de Minas Gerais) for funding this laboratory. A.G.R.-J. wishes to thank CAPES (Coordenação de Aperfeiçoamento de Pessoal de Nível Superior) for his scholarship. T.A.A.V. wishes to thank FAPEMIG for her scholarship.
Conflicts of interest
None.