Introduction
In securing delayed germination of seeds [i.e. dormancy], plants are not limited to the dead monotony of one method. As one studies the problem more fully he wonders whether there is any conceivable method of securing delay not made use of in one plant or another.
(Crocker, Reference Crocker1916)More than a century ago, the American plant physiologist William Crocker (Reference Crocker1916; also see Crocker, Reference Crocker1948) proposed what appears to be one of the first classification systems for the various causes of seed dormancy (e.g. Nikolaeva, Reference Nikolaeva2001; Pozdova and Razumova, Reference Pozdova, Razumova and Batygina2006). Crocker (Reference Crocker1916, p. 104) stated that a classification scheme is ‘… very desirable at this time in clearing up a chaotic situation and in giving future experimentation direction and aim’. In 1957, the British plant population biologist John L. Harper (Reference Harper1957; also see Harper, Reference Harper1977) presented a three-category (innate, imposed and induced) seed dormancy classification system that especially has been used by plant ecologists. Harper's innate dormancy category includes most of the causes of dormancy in Crocker's scheme. The most thorough seed dormancy classification system, however, was devised by the Russian seed physiologist Marianna G. Nikolaeva in Reference Nikolaeva1967[1969], which she modified several times between 1967 and 2001 (see Baskin and Baskin, Reference Baskin and Baskin2008). Her system was the first one to use both names and symbols/formulae to describe the kinds of dormancy. It is based on causes of dormancy (exogenous factors such as water-impermeable vs water-permeable seed coat and germination inhibitors in fruit coat and endogenous factors such as hormone balance and underdeveloped vs fully developed embryo) and conditions for breaking dormancy such as by treatment with gibberellic acid (GA3) and warm and/or cold stratification.
Baskin and Baskin (Reference Baskin and Baskin2004) proposed a three-tier hierarchical (class, level and type) classification system for seed dormancy based on a modification of Nikolaeva's scheme without using her symbols/formulae that has been widely used by seed biologists. In the second edition of Seeds: ecology, biogeography and evolution of dormancy and germination, Baskin and Baskin (Reference Baskin and Baskin2014a) presented an expanded, six-tier word-formula hierarchical seed dormancy classification system that integrated their scheme with that of Nikolaeva (see Baskin and Baskin, Reference Baskin and Baskin2008). However, there have been several new developments/further insights into whole-seed dormancy since 2004 that were not included in the Baskin and Baskin (Reference Baskin and Baskin2014a) classification system. We think that further revision of the Nikolaeva–Baskin dormancy classification scheme is needed at this time.
The Baskin and Baskin (Reference Baskin and Baskin2004) paper has been cited numerous times in the literature, which indicates that the Nikolaeva–Baskin system is being widely used by seed biologists. Especially noteable is that this system has been used to determine (1) proportional distribution (dormancy profile) of the five classes of dormancy and of nondormancy in various vegetation regions (biomes) on earth and in different kinds of plant communities (Baskin and Baskin, Reference Baskin and Baskin1998, Reference Baskin and Baskin2005, Reference Baskin and Baskin2014a; Sautu et al., Reference Sautu, Baskin, Baskin, Deago and Condit2007; Torres, Reference Torres2008; Schwienbacher et al., Reference Schwienbacher, Navarro-Cano, Neuner and Erschbamer2011; Kos et al., Reference Kos, Baskin and Baskin2012; Sommerville et al., Reference Sommerville, Martyn and Offord2013; Souza et al., Reference Souza, Torres, Seiner and Paulilo2015; Carta, Reference Carta2016; Dayrell et al., Reference Dayrell, Garcia, Negreiros, Baskin, Baskin and Silveira2016; Lan et al., Reference Lan, Yin, He, Tan, Liu, Xia, Wen, Baskin and Baskin2018; Escobar et al., Reference Escobar, Silveira and Morellato2021; Fernández-Pascual et al., Reference Fernández-Pascual, Carta, Cavieres, Rosbakh, Venn, Satyanti, Guja, Briceño, Vandelook, Mattana, Saatkamp, Bu, Sommerville, Poschlod, Liu, Nicotra and Jiménez-Alfaro2021; Liu et al., Reference Liu, Baskin, Baskin, Yang, Cao and Wen2021; Zupo et al., Reference Zupo, Daibes, Pausas and Fidelis2021), (2) proportional distribution of desiccation-sensitive seeds in relation to dormancy class (Tweddle et al., Reference Tweddle, Dickie, Baskin and Baskin2003), (3) phylogenetic relationships/evolution of the five classes of seed dormancy (Willis et al., Reference Willis, Baskin, Baskin, Auld, Venable, Cavender-Bares, Donohue and Rubio de Casas2014), (4) class of seed dormancy in relation to life-history traits including dispersal (Sautu et al., Reference Sautu, Baskin, Baskin and Condit2006, Reference Sautu, Baskin, Baskin, Deago and Condit2007; Salazar et al., Reference Salazar, Goldstein, Franco and Miralles-Wilhelm2011; Sánchez et al., Reference Sánchez, Montejo, Gamboa, Albert-Puentes and Hernández2015; Souza et al., Reference Souza, Torres, Seiner and Paulilo2015; Vandvik et al., Reference Vandvik, Elven and Töpper2017; Escobar et al., Reference Escobar, Silveira and Morellato2018; Soltani et al., Reference Soltani, Baskin, Baskin, Heshmati and Mirfazeli2018b; Costea et al., Reference Costea, Miari, Laczkó, Fekete, Molnár, Lovas-Kiss and Green2019) and (5) the class of dormancy of seeds used in ecological restoration and conservation projects, as a guide to how to break dormancy (Silveira, Reference Silveira2013; Erickson et al., Reference Erickson, Muñoz-Rojas, Kildisheva, Stokes, White, Heyes, Dalziell, Lewandrowski, James, Madsen, Turner and Merritt2017; Kildisheva et al., Reference Kildisheva, Erickson, Madsen, Dixon and Merritt2019, Reference Kildisheva, Dixon, Silveira, Chapman, Sacco, Mondoni, Turner and Cross2020; Zanetti et al., Reference Zanetti, Dayrell, Vardil, Damasceno, Fernandes, Castilho, Santos and Silveira2020). Also, dormancy formulae have been used to show in detail the step-by-step changes that occur during dormancy break in seeds with deep simple epicotyl morphophysiological dormancy (MPD) (Copete et al., Reference Copete, Herranz, Ferrandis, Baskin and Baskin2011) and non-deep complex MPD (Copete et al., Reference Copete, Copete, Ferrandis and Herranz2020).
In this review, we discuss the changes that have been made in the system since it was first published in 2004. Thus, some of the changes discussed (in the present paper) were included in the Baskin and Baskin (Reference Baskin and Baskin2014a) scheme. Our purpose is to present to the international seed biology community an updated-revised and expanded Nikolaeva–Baskin scheme of dormancy classification at the whole-seed level. Notable changes/additions to the scheme are presented below, and some of the finer details regarding dormancy formulae are given in the extensive list of footnotes in Table 1. This review is based on information collected from the literature over the past two decades. The dormancy formulae for the new kinds of dormancy are based on our interpretation of the morphological and physiological characteristics/requirements for dormancy break and germination reported in word-form by various authors.
Table 1. An expanded Nikolaeva–Baskin word-formula hierarchical classification system for primary seed dormancy
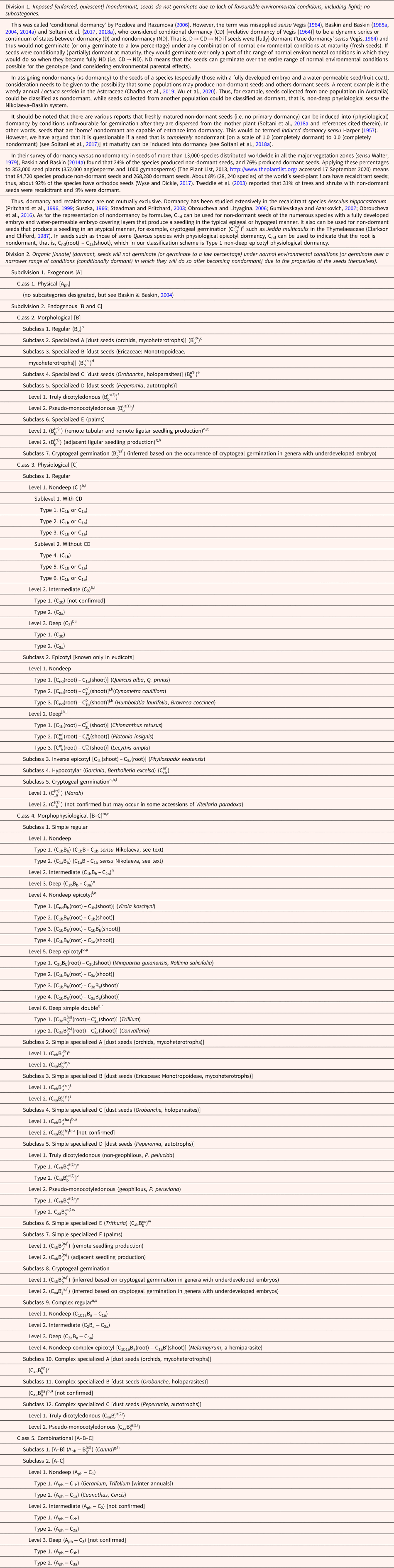
a (rs)′, root (r) and shoot (s) production from the cotyledonary petiole after its emergence from the diaspore of palms with remote tubular or remote ligular seedling production (superscript prime); and for cryptogeal germination in which the plumule is buried in the soil inside the cotyledonary petiole tube.
b Subscripts b and a, exposure to warm (b) or cold (a) temperatures is required for physiological dormancy (C) break (Cx a or Cx b); or embryo (B) grows during exposure to warm (Bb) or cold (Ba) temperatures. x indicates some unknown level (i.e. x = 1, 2 or 3) of physiological dormancy.
c Undifferentiated (superscript u) embryo (Bu) requiring warm temperatures (subscript b) to grow and form a protocorm (superscript p), that is, without differentiating into a plumular–radicular embryo axis. That is, a plumular–radicular embryo axis is never differentiated. Only a shoot meristem is differentiated by the protocorm. Roots arise adventitiously from the protocorm.
d Undifferentiated (superscript u′) embryo (Bu′) requiring warm temperatures (subscript b) to form a radicular pole germ tube (prime of u′) that differentiates a root apical meristem (superscript s′).
e Undifferentiated embryo (Bu′) requiring warm temperatures to grow (subscript b) to form a radicular pole germ tube (prime of u′) that gives rise to a haustorium (superscript h). That is, a radicle, which in most angiosperms gives rise to the primary root, is never differentiated. The part of the germ tube that remains outside the host forms a tubercule, which gives rise to the shoot.
f Undifferentiated embryo (Bu) requiring warm temperature (subscript b) to form a typical dicot embryo (inside seed), which upon germination gives rise to a dicotyledonous seedling with a root–shoot axis (superscript s, i.e. bipolar germination). Superscript (2) means that both cotyledons remain inside the seed and act as haustoria. Superscript (1) means that only one cotyledon acts as a haustorium, while the other one is free and photosynthetic (see footnote v for more details).
g For palms (and Canna), subscript b′ means that growth of the embryo (i.e. expansion of the cotyledonary haustorium) at warm temperatures inside the seed following emergence from the diaspore of the cotyledonary petiole with the embryo axis within it (germination). Although we indicate in our classification system that palms require exposure to warm temperatures for embryo growth/dormancy-break, Jaganathan et al. (Reference Jaganathan, Bayarkhuu, He, Liu, Li and Han2021) have reported that diaspores of Trachycarpus fortunei with remote germination require cold stratification for embryo growth and germination.
h (rs), root (r) and shoot (s) production from the cotyledonary petiole after its emergence from the diaspores of palms with adjacent ligular seedling production [non-prime on (rs)] and seedling production in Canna.
i Subscripts 1, 2 and 3 indicate non-deep, intermediate and deep physiological dormancy (C), respectively.
j Subscript nd indicates nondormant.
k Superscripts p and p′ indicate plumule dormant and nondormant, respectively, that is, delay and no delay of growth of plumule (inside seed), respectively, after root emergence.
l Embryo (hypocotyl) large but root and shoot meristems undifferentiated at seed maturity, and the root and shoot arise at germination from meristematic tissue at opposite ends of the hypocotyl. m means that there is a long delay in shoot emergence after root emergence, and m′ means that there is only a short or no delay in shoot emergence after root emergence.
m MPD also includes seeds with an undifferentiated embryo at seed maturity that differentiates into a typical underdeveloped embryo and becomes fully developed within the seed before germination occurs.
n In intermediate and deep simple MPD and in all four levels of complex MPD, the second component of the dormancy formula (Bb) indicates that further treatment is required to break dormancy of the embryo after it becomes fully developed.
o In non-deep simple and deep simple epicotyl MPD, a B′ (i.e. B′b and B′a) indicates that although growth of the underdeveloped embryo began in the first (warm) phase of dormancy break it continues in the second phase, in which exposure to warm (B′b) or cold (B′a) is required for the embryo to become fully developed.
p In deep simple epicotyl MPD, a B (i.e. without the prime) in both phases of dormancy break indicates that little (or no) embryo growth occurs in the first (warm) phase and that all or nearly all of it occurs during the second phase.
q Superscripts e and h indicate epigeal and hypogeal germination, respectively.
r For deep simple double MPD, subscripts b″ and b′ indicate that the embryo grows inside the seed before and after root emergence, respectively, in spring from seeds that have been exposed to cold temperatures (subscript a of C3a).
s Undifferentiated (organless) embryo (Bu) requiring a warm (Cx b)- or cold (Cx a)-temperature pretreatment to come out of dormancy grows into a protocorm (superscript p) from which a shoot (from apical meristem) and adventitious roots are produced. x indicates some unknown level (i.e. x = 1, 2 or 3) of physiological dormancy (C).
t Undifferentiated embryo (Bu′) requiring warm temperatures (subscript b) to form a germ tube that differentiates into a root apical meristem (superscript s′), after dormancy is broken by a warm (stratification or afterripening) treatment (Cx b) or by a cold (moist) stratification period (Cx a).
u Undifferentiated embryo (Bu′) requiring a warm temperature pretreatment [Cx b; or perhaps in some species a period of cold stratification (Cx a)] conditioning period to germinate in response to host exudate [e.g. the plant hormones strigolactones (Zwanenburg et al., Reference Zwanenburg, Pospišil and Zeljkovié2016)]. The embryo differentiates into a radicular pole and a germ tube, which gives rise to a haustorium (superscript h). That is, a radicle, which in most angiosperms gives rise to the primary root, is never differentiated. The part of the germ tube that remains outside the host forms a tubercle that gives rise to the shoot. x indicates some unknown level (i.e. x = 1, 2 or 3) of physiological dormancy (C).
v Undifferentiated embryo (Bu) requiring warm temperature (subscript b) to form a typical dicot embryo (inside seed), which upon germination gives rise to a dicotyledonous seedling with a root–shoot axis (superscript s, i.e. bipolar germination). Superscript (2) means that both cotyledons remain inside the seed and act as haustoria, after which they become free and photosynthetic, and superscript (1) means that only one cotyledon acts as a haustorium that remains permanently inside the seed (never becoming photosynthetic), while the other one is free and photosynthetic, never acting as a haustorium. Cx b means that warm (stratification or afterripening) temperatures are required for dormancy break, and Cx a means that cold (moist) stratification is required for dormancy break. x indicates some unknown level (i.e. x = 1, 2 or 3) of physiological dormancy (C).
w Seeds have PD (Cx b), and after germination [i.e. protrusion of undifferentiated embryo (Bex) to the outside of the seed coat (indicated by superscript ex, for external)] the embryo becomes differentiated into root and shoot. Thus, germination is bipolar. Subscript b of B is the same as it is in footnote b. Should seeds of a Trithuria species be documented to have no PD, the dormancy formula would be ${\rm B}_{\rm b}^{{\rm ex}} $. In which case, a specialized subclass of morphological dormancy would need to be added to our dormancy classification scheme.
x For non-deep complex regular MPD, subscript ‘1b1a’ indicates that the embryo requires warm followed by cold to grow.
y A cold pretreatment (Cx a) is required for the undifferentiated embryo (Bu) to develop into a plant [e.g. beginning with a protocorm (superscript p) in orchids] at warm temperatures (subscript b).
Results and discussion
Criteria for seed germination
Visible radicle emergence from the covering structures of the seed or other kinds of diaspores (dispersal/germination unit) is the commonly used criterion for germination (Bewley et al., Reference Bewley, Bradford, Hilhorst and Nonogaki2013). However, in evaluating the kinds of seed dormancy and germination morphologies across the seed plants, and especially the monocots, one needs to be aware that there is a considerable number of other definitions of (whole-seed) germination, that is, criteria for determining that a seed has germinated and thus that dormancy (if present) has been broken. In this paper, we have described various morphologies of germination that do not fit the ‘radicle emergence’ definition. These include cryptogeal germination that is represented in several eudicot families and the ‘unconventional’ germination morphologies of holoparasites (Orobanchaceae), mycoheterotrophs (orchids and monotropoids) and autotrophs (Peperomia, Trithuria and Utricularia) with ‘dust seeds’ and an undifferentiated embryo; Garcinia with a hypocotylar (macropodus) embryo; ‘liliaceous’ taxa such as Convallaria and Trillium with hypogeal and epigeal germination, respectively; palms; and seagrasses (e.g. Zostera marina). Taken together, taxa with unconventional morphologies of diaspore germination occur in many species. For example, around 28,000 species of orchids (Christenhusz and Byng, Reference Christenhusz and Byng2016; Givnish et al., Reference Givnish, Spalink, Ames, Lyon, Hunter, Zuluaga, Doucette, Caro, McDaniel, Clements, Arroyo, Endara, Kriebel, Williams and Cameron2016) alone, all of which have dust seeds with an undifferentiated embryo and monopolar germination, represent around 8% of the extant angiosperms. Other large groups, such as the grasses (10,500 species) and sedges (4,450 species) (Mabberley, Reference Mabberley2008), in which the coleorhiza and coleoptile, respectively, emerge first from the seed (caryopsis, achene) could be added to the list of species with unconventional germination, as could other monocot groups, in particular.
Hierarchical system
Although Nikolaeva's seed dormancy classification system is arranged in a hierarchical way, she did not assign names to the different tiers (layers) in her scheme. Baskin and Baskin (Reference Baskin and Baskin2004) used a three-layer hierarchical system – class, level and type – in ordering a modification of Nikolaeva's system. Baskin and Baskin (Reference Baskin and Baskin2014a) expanded the number of layers to six (division, subdivision, class, subclass, level and type), and these layers plus an additional one, namely sublevel, are used in the system presented in this review (Table 1).
It should be noted that kind of dormancy may be somewhat labile. For example, seeds of Frasera caroliniensis (Gentianaceae) mature in late summer, and the seeds are shed from the fruits of the mother plants over the following several months. Fresh seeds and those collected in early autumn have deep complex morphophysiological dormancy (MPD), while those collected in winter/early spring have non-deep complex MPD (Threadgill et al., Reference Threadgill, Baskin and Baskin1981; Baskin and Baskin, Reference Baskin and Baskin1986). Furthermore, the kind of dormancy may vary among fresh seeds within a population. For example, some fresh seeds of Aristolochia macrophylla (Aristolochiaceae) have morphological dormancy and others various degrees of non-deep simple MPD (Adams et al., Reference Adams, Baskin and Baskin2005). Other examples of the lability of the kind of dormancy in a taxon are discussed in the following pages.
Exclusion of chemical and mechanical dormancy
Nikolaeva recognized three kinds of exogenous dormancy (i.e. chemical, mechanical and physical) in her classification scheme (see Baskin and Baskin, Reference Baskin and Baskin2008). However, Baskin and Baskin (Reference Baskin and Baskin2004) did not include either chemical [germination inhibitor in embryo covering layer(s)] or mechanical [physical restriction to radicle emergence by embryo covering layer(s)] dormancy in their scheme. They argued that mechanical dormancy is a component of physiological dormancy (PD, low growth potential of embryo) and that the presence/significance of chemical dormancy in nature is yet to be proven. Nikolaeva (Reference Nikolaeva2004) agreed that the exclusion of chemical and mechanical dormancy from her system seems to be justified. Thus, neither chemical nor mechanical dormancy is included in the updated-revised scheme presented here (Table 1). Neither were they included in a recent translation (Russian to English) of the information on dormancy and germination of the nearly 3,000 species of seed plants in the book by Nikolaeva et al. (Reference Nikolaeva, Razumova, Gladkova and Danilova1985) entitled ‘Reference book on dormant seed germination’ (Rosbakh et al., Reference Rosbakh, Baskin and Baskin2020).
Two sublevels and six types of non-deep (regular) PD
Nikolaeva (Reference Nikolaeva2001) divided non-deep PD (C1) into subtype a (C1a) and subtype b (C1b). C1 indicates non-deep PD and subscripts a and b the requirement for a cold and warm pretreatment for seed dormancy break, respectively (Baskin and Baskin, Reference Baskin and Baskin2008). Baskin and Baskin (Reference Baskin and Baskin1998, Reference Baskin and Baskin2004, Reference Baskin and Baskin2014a) recognized five types of non-deep PD based on the temperature requirements for germination during and after dormancy break (see Figure 2 in Baskin and Baskin, Reference Baskin and Baskin2004). However, a sixth type of non-deep PD has been documented (Nur et al., Reference Nur, Baskin, Lu, Tan and Baskin2014). As in Types 4 and 5, fresh seeds of Type 6 do not germinate at any temperature, but with dormancy break, they germinate over the whole range of temperatures possible for the species. However, although there is no increase or decrease in the temperature requirements for germination during the dormancy-breaking process the germination percentage and/or rate increase(s). This pattern of response differs from that of Types 1, 2 and 3 in which there is a continuum of changes, for example gradual widening of the range of temperatures at which seeds will germinate as they come out of dormancy (see Soltani et al., Reference Soltani, Baskin and Baskin2017). In other words, seeds pass from the dormancy (D) state to the nondormancy (ND) state through various degrees of conditional dormancy (CD) [See Table 4.3 in Baskin and Baskin (Reference Baskin and Baskin2014a) for other continuous changes in physiological responses that seeds may undergo as they pass through the dormancy-breaking process, that is, D → CD → ND.] Our dormancy Types 1, 2, 3, 5 and 6 can be broken by either a warm or a cold (moist) pretreatment, depending on the species. Thus, the dormancy formula for each of these types is either C1b or C1a. However, to our knowledge, Type 4 has been shown to be broken only by a warm pretreatment, and thus, its formula is C1b (Baskin and Baskin, Reference Baskin and Baskin2014a). Based on these differences in the response of the seeds to temperature during the dormancy-breaking process, we created two sublevels of non-deep PD: Sublevel 1 (with CD) for Types 1, 2 and 3 and Sublevel 2 for Types 4, 5 and 6 (without CD) (see Soltani et al., Reference Soltani, Baskin and Baskin2017).
Physiological dormancy in Garcinia (Clusiaceae)
Garcinia is a genus of around 250–260 species of shrubs and trees that is widely distributed in tropical and subtropical areas of the world (Stevens, Reference Stevens and Kubitzki2007; Mabberley, Reference Mabberley2008; Sweeney, Reference Sweeney2008). The embryo consists primarily of a massive hypocotyl (‘hypocotyle tubercle’ of Sprecher, Reference Sprecher1919) with a central vascular cylinder (procambial strand) and meristematic tissue at the two poles of the seed; no endosperm is present and cotyledons are absent or rudimentary (Sprecher, Reference Sprecher1919; de Vogel, Reference de Vogel1979, Reference de Vogel1980; Onyekwelu, 1987; Joshi et al., Reference Joshi, Kumar, Gowda and Srinivasa2006; Asinelli et al., Reference Asinelli, Souza and Mourão2011; Cardoso et al., Reference Cardoso, Pereira, Pereira, Corrêa, Castro and Santos2013; Noor et al., Reference Noor, Aizat, Hussin and Rohani2016). During germination, the meristematic tissue produces a primary root at one end of the seed and a shoot at the other end. Subsequent to shoot emergence from the seed, an adventitious (secondary) root, which functions as the main root system of the plant, is produced at the junction between the shoot and hypocotyl. The primary root may persist for only a short period of time, or it may not be produced at all (de Vogel, Reference de Vogel1980; Ha et al., Reference Ha, Sands, Soepadmo and Jong1988; Joshi et al., Reference Joshi, Kumar, Gowda and Srinivasa2006; Kanmegne and Ndoumou Omokolo, Reference Kanmegne and Ndoumou Omokolo2007; Asomaning et al., Reference Asomaning, Olympio and Sacande2011; Noor et al., Reference Noor, Aizat, Hussin and Rohani2016). This morphological pattern of germination is known as the ‘Garcinia type’ (de Vogel, Reference de Vogel1979, Reference de Vogel1980).
Although they are recalcitrant (Lan et al., Reference Lan, Xia, Wang, Liu, Zhao and Tan2014; Subbiah et al., Reference Subbiah, Ramdhani, Pammenter, Macdonald and Sershen2019), the seeds of various species of Garcinia exhibit primary dormancy that can persist for several weeks or even months (Ng, Reference Ng1973, Reference Ng1980; Ng and Sanah, Reference Ng and Sanah1979; Miquel, Reference Miquel1987; Onyekwelu, Reference Onyekwelu1987; Raich and Khoon, Reference Raich and Khoon1990; Mathew and George, Reference Mathew and George1995; Chacko and Pillai, Reference Chacko and Pillai1997; Chen and Zhang, Reference Chen and Zhang1999; Chen et al., Reference Chen, Peng, Zhang and Zhao2002; Liu et al., Reference Liu, Qiu, Zhang and Chen2005; Joshi et al., Reference Joshi, Kumar, Gowda and Srinivasa2006, Reference Joshi, Phartyal and Arunkumar2017; Agyili et al., Reference Agyili, Sacande, Koffi and Peprah2007; Oboho and Nwaihu, Reference Oboho and Nwaihu2016; Rocha et al., Reference Rocha, Matos, Sena, Pachero and Ferreira2018). Seeds of G. cambogia with seed coat and without seed coat took about 1 year and 1 month, respectively, to reach maximum germination percentage. Seeds of several Garcinia species from a Malaysian rainforest took 6–8 months to reach maximum germination percentage (Ha et al., Reference Ha, Sands, Soepadmo and Jong1988). The number of days to the beginning of germination for the subtropical forest species (China) G. multiflora and G. oblongifolia was 101 and 175, respectively (Chen et al., Reference Chen, Peng, Zhang and Zhao2002). GA3 had no effect on seeds with or without seed coat (Mathew and George, Reference Mathew and George1995). The removal of the seed coat promotes germination (Mathew and George, Reference Mathew and George1995; Chacko and Pillai, Reference Chacko and Pillai1997; Liu et al., Reference Liu, Qiu, Zhang and Chen2005; Joshi et al., Reference Joshi, Kumar, Gowda and Srinivasa2006, Reference Joshi, Phartyal and Arunkumar2017).
We designate the kind of dormancy in Garcinia as a new subclass (Subclass 4. Hypocotylar) of PD (Table 1) with a general formula of ${\rm C}_{x{\rm b}}^{{\rm {m}^{\prime}}} $, where x is level 1, 2 or 3 of PD, subscript b indicates that warm temperatures are required to break PD and superscript m′ means the root and shoot arise (i.e. shoot is produced in a short period of time after the root is produced) at germination from meristems on opposite ends of the hypocotyl. However, seeds of some species of Garcinia have been reported to be nondormant (Malik et al., Reference Malik, Chaudhury and Abraham2005; Noor et al., Reference Noor, Aizat, Hussin and Rohani2016; Sánchez et al., Reference Sánchez, Pernús, Torres-Arias, Barrios and Dupuig2019), which we designate as
${\rm C}_{{\rm nd}}^{{\rm {m}^{\prime}}} $, where superscript ‘nd’ means nondormant. In the study by Agyili et al. (Reference Agyili, Sacande, Koffi and Peprah2007), seeds of G. kola seem to have epicotyl PD in which the shoot emerged 6 weeks after the onset of radicle protrusion. In which case, the dormancy formula for G. kola would be
${\rm C}_{1{\rm b}}^{\rm m} $(root) –
${\rm C}_{1{\rm b}}^{\rm m} $(shoot), where superscript m (without the prime) means that there is a long delay in shoot emergence after root emergence (see footnote a of Table 1 for more details). However, two other studies on the germination morphology of G. kola do not indicate that the seeds have epicotyl PD, that is, drawings in Onyekwelu (Reference Onyekwelu1987) and a photograph in Kanmegne and Ndoumou Omokolo (Reference Kanmegne and Ndoumou Omokolo2007) show that there is not much of a lag time between root and shoot emergence. Seeds of Garcinia species that take a long time to germinate may have the intermediate level of PD.
Embryo morphology/structure and germination phenology in the Clusiaceae genera Allanblackia (Delay and Mangenot, Reference Delay and Mangenot1960; Orwa et al., Reference Orwa, Mutua, Jamnadass and Simons2009; Ofori et al., Reference Ofori, Asomaning, Peprah, Agyeman, Anjarwalla, Tchoundjeu, Mowo and Jamnadass2015) and Symphonia (Maury-Lechon et al., Reference Maury-Lechon, Corbineau and Côme1980; Corbineau and Côme, Reference Corbineau and Côme1986) are the same as they are for Garcinia. Also, like seeds of Garcinia (Normah et al., Reference Normah, Ramiya and Gintangga1997; Pritchard et al., Reference Pritchard, Daws, Fletcher, Gaméné, Msanga and Omondi2004; Daws et al., Reference Daws, Garwood and Pritchard2005; Asomaning et al., Reference Asomaning, Olympio and Sacande2011; Anto et al., Reference Anto, Angala, Jothish and Anilkumar2019) those of Allanblackia (Mwaura and Munjuga, Reference Mwaura, Munjuga, van der Vosssen and Mkamilo2007; Orwa et al., Reference Orwa, Mutua, Jamnadass and Simons2009; FAO, 2013) and Symphonia (Corbineau and Côme, Reference Corbineau and Côme1986, Reference Corbineau and Côme1988; Daws et al., Reference Daws, Garwood and Pritchard2005) are recalcitrant. Ofori et al. (Reference Ofori, Peprah, Cobbinah, Atchwerebour, Osabutey, Tchoundjeu, Simons and Jamnadass2011) reported that seeds of A. parviflora were dormant, taking 7 months after sowing for germination to begin, and that removal of the seed coat promoted germination. Seeds of A. floribunda also are dormant (Ileleji et al., Reference Ileleji, Hamadina and Orluchukwu2015). However, the seeds of S. globulifera were reported by Corbineau and Côme (Reference Corbineau and Côme1986, Reference Corbineau and Côme1988) to be nondormant. Note in Table 1 that seeds of Platonia insignis (also in Clusiaceae) have Type 3 deep epicotyl PD.
Physiological dormancy in Brazil nut (Bertholletia excelsa, Lecythidaceae)
The Brazil nut, an economically important tree native to the Amazon Basin (Mori and Prance, Reference Mori and Prance1990), has an embryo similar to that of Garcinia (i.e. macropodial) (Prance and Mori, Reference Prance and Mori1978; Cunha et al., Reference Cunha, Prado, Carvalho and Góes1996). Seeds have intermediate storage behaviour (Figueirêdo and Carvalho, Reference Figueirêdo and Carvalho1994; Hong et al., Reference Hong, Linington and Ellis1998). Intact fresh seeds require 6–36 months to germinate (Watson, Reference Watson1901; Hill, Reference Hill1939; Müller, Reference Müller1981), and even seeds (embryos) with the seed coat removed may take a long time to germinate (Kainer et al., Reference Kainer, Duryea, Malavasi, Silva and Harrison1999a,Reference Kainer, Malavasi, Duryea and Silvab; Dionisio et al., Reference Dionisio, Auca, Schwartz, Bardales-Lozano, Agurto and Corvera-Gomringer2019). However, embryos isolated from seeds that were moist stored (warm stratified) for 5.5 months germinated to a higher percentage and rate (speed) than those from non-stored seeds (Kainer et al., Reference Kainer, Duryea, Malavasi, Silva and Harrison1999a). Thus, as for Garcinia, we assign B. excelsa to Subclass 4 (Hypocotylar) of PD with the formula of ${\rm C}_{x{\rm b}}^{{\rm {m}^{\prime}}} $. Cx b is a level of PD requiring warm temperatures to break dormancy, and superscript m′ means the root and shoot arise (i.e. shoot is produced in a short period of time after the root is produced) at germination from meristematic tissue at opposite ends of the hypocotyl. Since intact seeds of B. excelsa, as well as those with the seed coat removed, take a long time to germinate, warm moist storage increases the percentage and rate of germination and seedlings from embryos of fresh seeds grow normally, the level of PD in these seeds probably is intermediate, in which case
${\rm C}_{x{\rm b}}^{{\rm {m}^{\prime}}} $ is
${\rm C}_{2{\rm b}}^{{\rm {m}^{\prime}}} $. Note in Table 1 that seeds of Lecythis ampla (also in Lecythidaceae) have Type 3 of deep epicotyl PD.
Non-deep (regular) PD in tribe Myrteae (Myrtaceae)
This tribe in the large family Myrtaceae (subfamily Myrtoideae) consists of about 2400 species, and it has three basic types of embryos that, for the most part, correspond closely to the three subtribes of Myrteae: Myrciinae (myrcioid embryo); Eugeniiae (eugenioid embryo) and Myrtinae (myrtoid embryo) (Schmid, Reference Schmid1980; Landrum, Reference Landrum1981, Reference Landrum1986; Landrum and Stevenson, Reference Landrum and Stevenson1986). Landrum (Reference Landrum1986) subdivided Myrteae into the three subtribes and the genus Luma or just into three subtribes (Landrum and Kawaski, Reference Landrum and Kawaski1997) based on features of the embryo such as the hypocotyl (e.g. well developed or not), cotyledons (thin or fleshy) and their shape and relative size. Features of Luma embryos are more or less intermediate between the eugenioid and myrcioid types. Landrum (Reference Landrum1986) and Landrum and Stevenson (Reference Landrum and Stevenson1986) sorted out the Camponesia complex (several genera) from the rest of the subtribe Myrtinae based on the swollen hypocotyl and the presence of a central core of vascular tissue distinct from the surrounding parenchyma in the Camponesia complex. There is no evidence of a radicle in the embryo of tribe Myrteae before the seed germinates. During germination, the hypocotyl emerges from the seed, after which a radicle and shoot are produced from meristematic tissue at opposite ends of the hypocotyl (Landrum, Reference Landrum1986; Landrum and Stevenson, Reference Landrum and Stevenson1986; Justo et al., Reference Justo, Alvarenga, Alves, Guimarãea and Strassburg2007; Rego et al., Reference Rego, Cosmo, Gogsz, Kuniyoshi and Nogueira2011).
As for seed dormancy and germination in tribe Myrteae, the most extensively studied genus is Eugenia. The seeds of Eugenia are recalcitrant (desiccation sensitive) (e.g. Anjos and Ferraz, Reference Anjos and Ferraz1999; Andrade et al., Reference Andrade, Cunha, Souza, Reis and Almeida2003; Daws et al., Reference Daws, Garwood and Pritchard2005; Mayrinck et al., Reference Mayrinck, Vaz and Davide2016; Calvi et al., Reference Calvi, Anjos, Kranner, Pritchard and Ferraz2017a,Reference Calvi, Aud, Ferraz, Pritchard and Krannerb; Pelissari et al., Reference Pelissari, José, Fontes, Matos, Pereira and Faria2018; Subbiah et al., Reference Subbiah, Ramdhani, Pammenter, Macdonald and Sershen2019; Mattana et al., Reference Mattana, Peguero, Sacco, Agramonte, Castillo, Jiménez, Clase, Pritchard, Gómez-Barreiro, Castillo-Lorenzo, Encarnación, Way, Garcia and Ulian2020). However, while seeds of some species are nondormant at maturity, others are dormant (e.g. Rizzini, Reference Rizzini1970; Garwood, Reference Garwood1983; Anjos and Ferraz, Reference Anjos and Ferraz1999; Gentil and Ferreira, Reference Gentil and Ferreira1999; Santos et al., Reference Santos, Ferreira and Aquila2004; Scalon et al., Reference Scalon, Filho and Rigoni2004; Martinotto et al., Reference Martinotto, Paiva, Santos, Soares, Nogueira and Silva2007; Braz and Mattos, Reference Braz and Mattos2010; Mendes and Mendonca, Reference Mendes and Mendonca2012; Calvi et al., Reference Calvi, Anjos, Kranner, Pritchard and Ferraz2017a,Reference Calvi, Aud, Ferraz, Pritchard and Krannerb). We see no reason not to classify dormant seeds of Myrteae as having Subclass 4 (Hypocotylar) of class PD with a formula of ${\rm C}_{x{\rm b}}^{{\rm {m}^{\prime}}} $. However, it seems that seeds of many (most?) of about 950 species of Eugenia (Mabberley, Reference Mabberley2008) are nondormant (e.g. von Bülow et al., Reference von Bülow, Carmona and Parente1994; Maluf et al., Reference Maluf, Bilia and Barbedo2003; Silva et al., Reference Silva, Bilia, Maluf and Barbedo2003; Delgado and Barbedo, Reference Delgado and Barbedo2007, Reference Delgado and Barbedo2012; Teixeira and Barbedo, Reference Teixeira and Barbedo2012; Scalon et al., Reference Scalon, Neves, Maseto and Pereira2012; Lamarca et al., Reference Lamarca, Prataviera, Borges, Delgado, Teixeira, Camargo, Faria and Barbedo2013). In which case, the formula would be
${\rm C}_{{\rm nd}}^{{\rm {m}^{\prime}}} $.
In addition to seedling production from whole-seed germination, seeds of Eugenia are ‘cut-tolerant’. Thus, they can be cut into halves, fourths or even eighths, and each fragment may produce a root, shoot or both root and shoot (complete seedling), that is, totipotent germination. By this method, it is possible to produce two, three or even more seedlings from a single seed (e.g. Silva et al., Reference Silva, Bilia, Maluf and Barbedo2003; Delgado et al., Reference Delgado, Mello and Barbedo2010; Teixeira and Barbedo, Reference Teixeira and Barbedo2012; Prataviera et al., Reference Prataviera, Lamarca, Teixeira and Barbedo2015; Calvi et al., Reference Calvi, Anjos, Kranner, Pritchard and Ferraz2017a,Reference Calvi, Aud, Ferraz, Pritchard and Krannerb). This cutting of the seeds into fragments is a way to propagate the species when the number of seeds is limited, as apparently they often are in Eugenia (Silva and Pinheiro, Reference Silva and Pinheiro2009). Teixeira and Barbedo (Reference Teixeira and Barbedo2012) suggested that the ability of Eugenia to regenerate seedlings from seed fragments is an adaptation to the high level of seed predation by insects.
Other species with cut-tolerant seeds include Allanbackia parviflora (Ofori et al., Reference Ofori, Asomaning, Peprah, Agyeman, Anjarwalla, Tchoundjeu, Mowo and Jamnadass2015) and Garcinia species (Malik et al., Reference Malik, Chaudhury and Abraham2005; Joshi et al., Reference Joshi, Kumar, Gowda and Srinivasa2006; Noor et al., Reference Noor, Aizat, Hussin and Rohani2016) in the Clusiaceae. Joshi et al. (Reference Joshi, Kumar, Gowda and Srinivasa2006) speculated that the ability of seed fragments of Garcinia gummi-gutta to regenerate ‘… may have evolved to exploit mammalian frugivory for dispersal’. In Gustavia superba (Lecythidaceae), whose seeds are recalcitrant (Daws et al., Reference Daws, Garwood and Pritchard2005), small cotyledonary fragments and insect-infested seeds can germinate and produce seedlings (Harms et al., Reference Harms, Dalling and Aizprúa1997; Dalling and Harms, Reference Dalling and Harms1999). The recalcitrant diaspores of Idiospermum australiense (Calycanthaceae) are large (up to 225 g) and have two to six cotyledons (average four). Each cotyledon separated from a single diaspore is capable of producing an independent seedling (Edwards et al., Reference Edwards, Gadek, Weber and Worboys2001).
Epicotyl PD
Until relatively recently, the term epicotyl dormancy primarily has been used to describe dormancy in seeds with an underdeveloped embryo in which shoot emergence lags behind root emergence by a few to several weeks or even months. Although acorns of some temperate-zone oaks (Quercus species, Fagaceae) are recalcitrant and have fully developed embryos, they exhibit epicotyl dormancy, especially the white oak group, with the root emerging in autumn shortly after dispersal and the shoot the following spring (Lewis, Reference Lewis1911; Korstian, Reference Korstian1927; Wood, Reference Wood1938; Jones, Reference Jones1959; Allen and Farmer, Reference Allen and Farmer1977; Farmer, Reference Farmer1977; Fox, Reference Fox1982; Bonner and Vozzo, Reference Bonner and Vozzo1987; Wigston, Reference Wigston1987; Matsuda and McBridge, Reference Matsuda and McBridge1987; Thoreau, Reference Thoreau2000, pp. 183–186; Connor and Sowa, Reference Connor and Sowa2003; Steele et al., Reference Steele, Wauters, Larsen, Forget, Lambert, Hulme and VanderWall2005; Xia et al., Reference Xia, Daws, Hay, Chen, Zhou and Pritchard2012a,Reference Xia, Daws, Stuppy, Zhou and Pritchardb, Reference Xia, Hill, Li and Walters2014; Yi et al., Reference Yi, Yang, Curtis, Bartlow, Agosta and Steele2012, Reference Yi, Curtis, Bartlow, Agosta and Steele2013; McCartan et al., Reference McCartan, Jinks and Barsoum2015; Joët et al., Reference Joët, Ourcival, Capelli, Dussert and Morin2016; Leiva and Díaz-Maqueda, Reference Leiva and Díaz-Maqueda2016). In contrast, in members of the red oak group, both the root and shoot emerge in spring. Thus, the white oaks have epicotyl PD and the red oaks PD (Korstian, Reference Korstian1927).
For acorns of the white oak Q. robur, McCartan et al. (Reference McCartan, Jinks and Barsoum2015) showed that epicotyl dormancy (shoot emergence lagging behind root emergence, which they called germination) is due to differences in thermal time that needs to be accumulated for the two events (germination and shoot emergence) leading to the production of a seedling. Thus, more °C weeks are required for shoot than for root emergence, resulting in root emergence in autumn–winter and shoot emergence in spring–summer in southern England. It should be noted that the percentage of acorns with epicotyl PD may vary among maternal families, seed lots and populations/provenances of a species (Allen and Farmer, Reference Allen and Farmer1977; Farmer, Reference Farmer1977; Wigston, Reference Wigston1987; McCartan et al., Reference McCartan, Jinks and Barsoum2015). Yunnanopilia longistaminea (Opiliaceae), a recalcitrant species, seems to have a similar kind of epicotyl PD as Quercus (Yang et al., Reference Yang, Yang, Wang and Shen2017).
The general formula for epicotyl PD in Quercus acorns is Cx(root) − Cx ′(shoot), where C = PD, subscript x = nd (nondormant) and subscript x′ = 1a [or 1b (see below)]. Thus, Cnd(root) means a nondormant root and Cx ′(shoot) is a shoot with some level of physiological dormancy, that is, non-deep PD in which the shoot needs a winter cold period (subscript 1a) to come out of dormancy. However, although shoot emergence lags behind root emergence, shoot growth may occur at warm temperatures after an extended period of time, in which case, one could argue that subscript x′ should be 1b.
It should be noted that, in many oak species, the epicotyl is carried just outside the apex of the acorn via elongation of the cotyledonary petioles prior to shoot elongation (Rowlee and Hastings, Reference Rowlee and Hastings1898; Pammel and King, Reference Pammel and King1917; Korstian, Reference Korstian1927; Jones, Reference Jones1959; Molinas and Verdaguer, Reference Molinas and Verdaguer1993; Pascual et al., Reference Pascual, Molinas and Verdaguer2002; Sung et al., Reference Sung, Kormanik and Zarnoch2010; Yi et al., Reference Yi, Yang, Curtis, Bartlow, Agosta and Steele2012, Reference Yi, Curtis, Bartlow, Agosta and Steele2013, Reference Yi, Bartlow, Curtis and Agosta2019). Furthermore, in some species of Quercus, including Q. chungii (Sun et al., Reference Sun, Song, Ge, Dai and Kozlowski2021), Q. engelmannii (Snow, Reference Snow1991), S. semecarpifolia (Troup, Reference Troup1921; Shrestha, Reference Shrestha2003) and Q. virginiana (Engelmann, Reference Engelmann1880; Lewis, Reference Lewis1911; Coker, Reference Coker1912), the cotyledonary tube (formed by fusion of cotyledonary petioles) carries/pushes the embryonic axis up to several centimetres from the apex of the acorn. Troup (Reference Troup1921) says that in Q. semicarpifolia ‘… the tube may thus attain a length of as much as 4 in [10 cm] or even more’. This mode of germination in Q. engelmannii (Snow, Reference Snow1991) and Q. virginiana (Engelmann, Reference Engelmann1880) has been compared to that of cryptogeal germination of Marah oreganus, which is described below.
Epicotyl PD of a different kind than that in Quercus has been found in several woody species other than oaks: Brownea coccinea (Fabaceae, subfamily Caesalpinioideae) (Jayasuriya et al., Reference Jayasuriya, Wijetunga, Baskin and Baskin2012); Chionanthus retusus (Oleaceae) (Chien et al., Reference Chien, Kuo-Huang, Shen, Zhang, Chen, Yang and Pharis2004); Cynometra cauliflora (Fabaceae, subfamily Caesalpinioideae) (Jayasuriya et al., Reference Jayasuriya, Wijetunga, Baskin and Baskin2012); Humboldtia laurifolia (Fabaceae, subfamily Caesalpinioideae) (Jayasuriya et al., Reference Jayasuriya, Wijetunga, Baskin and Baskin2010); Lecythis ampla (Lecythidaceae) (Flores, Reference Flores and Vozzo2002); and Platonia insignis (Clusiaceae) (Mourão and Beltrati, Reference Mourão and Beltrati1995a,Reference Mourão and Beltratib,Reference Mourão and Beltratic; Carvălho et al., Reference Carvălho, Muller and Leao1998). Seeds of five of the species as well as those of Quercus are recalcitrant (desiccation sensitive) (Subbiah et al., Reference Subbiah, Ramdhani, Pammenter, Macdonald and Sershen2019); seeds of Chionanthus retusus are orthodox (Chien et al., Reference Chien, Kuo-Huang, Shen, Zhang, Chen, Yang and Pharis2004; Yang and Lin, Reference Yang and Lin2004). While, in Quercus, the epicotyl/plumule does not have to grow (elongate) inside the mature dispersed acorns (embryo fully developed) before the shoot emerges, in the other six genera it must grow (Brownea, Chionanthus, Cynometra and Humboldtia) or the meristem to differentiate (Lecythis and Platonia) inside the seed before the shoot emerges. Furthermore, depending on the species, there may or may not be a delay (lag time) in the initiation of growth of the epicotyl/plumule inside the seed following root emergence (see below).
In B. coccinea, C. retusus, C. cauliflora and H. laurifolia, the general formula for epicotyl PD is Cx(root) − ${\rm C}_{{x}^{\prime}}^{\rm p} $(shoot) [or Cx(root) −
${\rm C}_{{x}^{\prime}}^{{\rm {p}^{\prime}}} $(shoot) (see below)], where subscript x = nd (nondormant) or 1b, that is, non-deep PD that requires a warm period (subscript 1b) for dormancy break. Thus, the root is nondormant or has non-deep PD.
${\rm C}_{{x}^{\prime}}^{\rm p} $ indicates a level of PD (i.e.
${\rm C}_{{x}^{\prime}}$) in which the epicotyl/plumule (superscript p or p′, see below) needs to grow inside the seed before the shoot emerges.
${\rm C}_{{x}^{\prime}}$ is C1b for Humboldtia, Brownea and Cynometra and C1a for Quercus alba, Q. prinus and Q. robur and C3b for Chionanthus (Table 1). In seeds of some of these species with epicotyl PD in which the epicotyl/plumule has to grow before the shoot emerges, there is a delay of several weeks before the epicotyl/plumule begins to grow following root emergence (indicated by superscript p). However, in seeds of other species with this kind of epicotyl dormancy, there is no delay in the beginning of growth of the epicotyl/plumule following root emergence (indicated by superscript p′) (Jayasuriya et al., Reference Jayasuriya, Wijetunga, Baskin and Baskin2010, Reference Jayasuriya, Wijetunga, Baskin and Baskin2012). Sánchez et al. (Reference Sánchez, Pernús, Torres-Arias, Barrios and Dupuig2019) reported that seeds of Cynometra cubensis subsp. cubensis in Cuba were nondormant, but dormancy in this taxon needs further scrutiny to determine if the seeds have epicotyl rather than regular PD (J. Sánchez, personal communication).
In fresh seeds of Lecythis ampla (Prance and Mori, Reference Prance and Mori1978; Flores, Reference Flores and Vozzo2002) and Platonia insignis (Mourão and Beltrati, Reference Mourão and Beltrati1995a,Reference Mourão and Beltratib,Reference Mourão and Beltratic), the large embryo consists mainly of a massive hypocotyl, and the root and shoot are not differentiated into organs, that is, meristems only. The general formula for epicotyl dormancy in these two species is ${\rm C}_x^{\rm m} $(root) −
${\rm C}_{{x}^{\prime}}^{\rm m} $(shoot), where superscript m indicates undifferentiated root and shoot and x and x′ indicate the level of dormancy in root and shoot, respectively. Subscripts x and x′ for L. ampla are 1b and 3b, respectively, and for P. insignis nd and 3b, respectively. Thus, we designate the dormancy formula for L. ampla as
${\rm C}_{1{\rm b}}^{\rm m} $(root) −
${\rm C}_{3{\rm b}}^{\rm m} $(shoot) and that for P. insignis as
${\rm C}_{{\rm nd}}^{\rm m} $(root) −
${\rm C}_{3{\rm b}}^{\rm m} $(shoot), where superscript ‘m’ means that root and shoot in fresh ripe seeds are present only as meristems. In L. ampla, the root emerges from the seed in about 45 d and the shoot in about 240 d (Flores, Reference Flores, Ouédraogo, Poulsen and Stubsgaard1996). In P. insignis, root emergence occurs within 1 month after sowing and the shoot in approximately 6 months (Mourão and Beltrati, Reference Mourão and Beltrati1995c; Carvãlho et al., Reference Carvălho, Muller and Leao1998). Furthermore, it seems likely that several woody species of the cerrado (Brazilian savanna), including Andira laurifolia (Fabaceae), Pouteria torta (Sapotaceae) and Vochysia thyrsoidea (Vochysiaceae) have seeds with epicotyl PD (Rizzini, Reference Rizzini1965).
Seed dormancy and germination in seagrasses
The embryo in seagrasses is fully developed and occupies most of the space inside the seed coat; no endosperm is present in mature seeds. Most of the volume of the ‘macopodus’ embryo (sensu Arber, Reference Arber1920) consists of the hypocotyl. In most species, the seeds have non-deep PD, or they are nondormant (see Table 11.7 in Baskin and Baskin, Reference Baskin and Baskin2014a). According to Kuo and Kirkman (Reference Kuo, Kirkman, Kuo, Phillips, Walker and Kirkman1996), there are three categories of seed dormancy in seagrasses, that is seeds (1) with a membranous seed coat and no dormancy; (2) with a hard (not implying water impermeability) pericarp and/or distinct seed coat and a distinct dormancy period and (3) without a seed coat (seed is ategmic, i.e. without a seed coat) germinating on parent plant (vivipary) and no dormancy. The main nutrient reserves in categories 1 and 2 are starch and protein, which are stored in the hypocotyl and used during germination and seedling development. Seeds in category 3 do not store starch or protein, and seedlings obtain nutrients directly from the parent plant via transfer cells located at the interface between seedling and parent.
Germination in seagrasses often is defined as splitting of the seed coat and emergence of the cotyledon (Taylor, Reference Taylor1957; McMillan, Reference McMillan1981; McMillan et al., Reference McMillan, Bridges, Kock and Falanruw1982; Churchill, Reference Churchill1983; Vollebergh and Congdon, Reference Vollebergh and Congdon1986; Koch and Seeliger, Reference Koch and Seeliger1988; Harrison, Reference Harrison1991; Peterken and Conacher, Reference Peterken and Conacher1997; Probert and Brenchley, Reference Probert and Brenchley1999; Kaldy et al., Reference Kaldy, Shafer, Ailstock and Magoun2015; Xu et al., Reference Xu, Zhou, Wang, Wang, Zhang and Gu2016; Gu et al., Reference Gu, Zhou, Song, Xu, Zhang, Lin, Xu and Zhu2018). Following cotyledon emergence, adventitious roots emerge from the cotyledonary node, or a primary root (radicle) emerges from the primary root meristem. Thus, in some seagrasses (e.g. Zosteraceae), the embryo has a primary root (radicle) meristem, but it does not develop (Tillich, Reference Tillich, Rudall, Cribb, Cutler and Humphries1995). However, while in Halophila spinulosa (Birch, Reference Birch1981), H. ovalis (Kuo and Kirkman, Reference Kuo and Kirkman1992; Statton et al., Reference Statton, Sellers, Dixon, Kilminster, Merritt and Kendrick2017), H. tricostata (Kuo et al., Reference Kuo, Long and Coles1993) and Thalassia testudinum (Hydrocharitaceae) (Orpurt and Boral, Reference Orpurt and Boral1964), for example, a primary root is produced. Since there is no endosperm and the embryo cotyledon is small, most of the seed reserves are stored in the large hypocotyl. The first leaf of the seedling arises through a slit-like opening in the cotyledonary sheath. Xu et al. (Reference Xu, Zhou, Wang, Wang, Zhang and Gu2016) divided the germination/seedling development of Zostera marina into six stages following a pre-germination stage, which they called stage 0: (0) seeds ripe, plump and intact, (1) rupture of seed coat and emergence and growth of cotyledon (germination per se); (2) cotyledonary sheath, cotyledonary blade and adventitious roots elongated from basal hypocotyl (primary root does not develop); (3) first true leaf emerged from base of cotyledonary blade; (4) adventitious roots emerged from base of hypocotyl; (5) seedling with second true leaf that emerged from base of cotyledonary blade and (6) seedling with two true leaves (cotyledonary blade has withered away).
An unusual kind of germination morphophysiology has been described by Kuo et al. (Reference Kuo, Iizumi, Nilsen and Aioi1990) for the Japanese seagrass Phyllospadix iwatensis (Zosteraceae). The sequence of dormancy break (here defined as both root and shoot emergence from diaspore) in this species is the reverse (shoot before root) of that of physiological epicotyl and of morphophysiological epicotyl and double MPD (root before shoot). In which case, we designate seed dormancy in this species with a fully developed embryo to a new subclass of PD, that is, ‘inverse’ (Table 1). Seeds of P. iwatensis are dormant and require several months to complete germination, that is, both shoot and root emergence; the shoot emerges well in advance of the root. The emergence of the shoot (cotyledon–plumule) began in about 6 weeks, and 53.5% of the seeds had germinated after 6 months, at which time roots had not emerged from any germinated seeds; no primary root is produced. The onset of (adventitious) root emergence from the shoot began about 8 months after seed collection, by which time the plumule had given rise to several grass-like leaves.
Shoot emergence in P. iwatensis began after the seeds had been exposed to warm-stratifying temperatures (15–19°C), while root emergence did not begin until after the seeds also had been exposed to cold-stratifying temperatures (4–10°C) for several months (Kuo et al., Reference Kuo, Iizumi, Nilsen and Aioi1990). Based on this information, we suggest the formula C1b(shoot)–C3a(root) for dormancy in this species. We are puzzled by the assignment of nondormant to seeds of this species by Orth et al. (Reference Orth, Harwell, Bailey, Bartholomew, Jawad, Lombana, Moore, Rhode and Woods2000). A western North American species of Phyllospadix (specific epithet not given) exhibits the same or a very similar morphological sequence during germination as does P. iwatensis, that is, shoot emergence followed by adventitious root emergence (Gibbs, Reference Gibbs1902). However, no mention was made of how long shoot emergence was delayed after seed maturity and dispersal or how long root emergence was delayed after shoot emergence.
Taylor (Reference Taylor1957) described a pattern of seedling production similar to that of P. iwatensis for Zostera marina from Prince Edward Island, Canada, another seagrass in the Zosteraceae. He cold-stratified seeds of Z. marina in seawater in a refrigerator at 4°C for 4 months. Upon removal of seeds from the refrigerator to room conditions, the emergence of hypocotyl, cotyledon and plumule occurred within a few days and of emergence of two (adventitious) roots (from the cotyledon node) within less than 3 weeks. Thus, although root emergence followed shoot emergence in Z. marina, the time interval was much shorter than that in P. iwatensis.
Tutin (Reference Tutin1938) described this pattern of germination morphology in which shoot emergence precedes (adventitious) root emergence for Z. marina in England, and also for Z. hornemenniana. Most of the seeds of both species that germinated did so only after several months of incubation, and most of those that had not germinated after about 6 months rotted. These data indicate that at least the shoot is dormant in these two species of Zostera from England. Montes-Recinas et al. (Reference Montes-Recinas, Márquez-Guzmán and Orozco-Segovia2012) have described what appears to be a similar germination phenology in seeds of Tillandsia recurvata (Bromeliaceae), which their Figure 1d shows a seed with a fully developed embryo and no endosperm. Thirty to forty days after the seed coat splits and the embryo turns green the first true leaf emerges from the seed; the root appears several months later.
Many studies have been done on the seed germination of Z. maritima [syn. Z. marina] (e.g. see Appendix 1 in Orth et al., Reference Orth, Harwell, Bailey, Bartholomew, Jawad, Lombana, Moore, Rhode and Woods2000; Xu et al., Reference Xu, Zhou, Wang, Wang, Zhang and Gu2016 and many references cited therein), a geographically widely distributed species in the North Atlantic and North Pacific oceans, where it is the dominant seagrass (den Hartog, Reference den Hartog1970). After a thorough study of the effect of temperature, salinity and substrate oxygen content (i.e. anaerobic vs aerobic conditions) on the germination of seeds of plants of this species from the UK, Probert and Brenchley (Reference Probert and Brenchley1999) concluded that the seeds were nondormant. However, the lengths of the incubation periods required for the seeds of Z. marina to germinate in the Probert and Brenchly study, even under optimum conditions, were much longer than the 30-d limit. Baskin and Baskin (Reference Baskin and Baskin2014a) used to assign seeds (fruits) with a fully developed embryo and water-permeable covering layers (such as Z. marina) to nondormancy. Thus, we conclude that the seeds of Z. marina in the study by Probert and Brenchley (Reference Probert and Brenchley1999) were dormant. Nevertheless, a significant contribution of the Probert and Brenchley (Reference Probert and Brenchley1999) study is the demonstration that seeds of Z. marina germinate much better under anaerobic than under aerobic conditions, a factor that most of the many studies on this species have not considered. Both dormancy and nondormancy have been reported in this geographically widespread species (Orth et al., Reference Orth, Harwell, Bailey, Bartholomew, Jawad, Lombana, Moore, Rhode and Woods2000). Thus, a thorough evaluation of dormancy versus nondormancy of Z. marina seeds collected throughout the range of this species is needed.
Do tropical/subtropical species of Fagaceae have epicotyl PD?
The Fagaceae is a Northern Hemisphere woody plant family (Manos and Stanford, Reference Manos and Stanford2001) of nine genera and more than 900 species, 97% of which belong to Castanopsis (around 150 species), Lithocarpus (around 300) and Quercus (around 435) (Cannon et al., Reference Cannon, Brendel, Deng, Hipp, Kremer, Kua, Plomion, Romero-Severson and Sork2018). Acorns of temperate oaks with epicotyl PD sometimes are referred to as being nondormant since the root emerges soon after dispersal in autumn (e.g. Pammel and King, Reference Pammel and King1917; Korstian, Reference Korstian1927; Olson and Boyce, Reference Olson and Boyce1971; Barnett, Reference Barnett1977; Steele et al., Reference Steele, Wauters, Larsen, Forget, Lambert, Hulme and VanderWall2005; Bonner, Reference Bonner, Bonner and Karrfalt2008; Yi et al., Reference Yi, Yang, Curtis, Bartlow, Agosta and Steele2012). Center et al. (Reference Center, Etterson, Deacon and Cavender-Bares2016) said that acorns of Q. oleoides, a live oak of New World tropical dry forests, were nondormant. However, although the root of Q. oleoides can emerge immediately after maturity, even sometimes while still attached to the parent tree (Klemens et al., Reference Klemens, Deacon, Cavender-Bares, Dirzo, Young, Mooney and Ceballos2011), shoot emergence was delayed about 50–150 d after planting (Center et al., Reference Center, Etterson, Deacon and Cavender-Bares2016). Thus, it seems likely that the acorns of this species have epicotyl PD. Recently, Sun et al. (Reference Sun, Song, Ge, Dai and Kozlowski2021) have clearly demonstrated that seeds of Quercus chungii from subtropical southern China have epicotyl PD.
For four species of Castanopsis and five of Lithocarpus, the mean minimum time from seed sowing to germination (production of a seedling) in Kuala Lumpur, Malaysia, was 102 and 101 d, respectively, and the mean maximum length of time was 513 and 190 d, respectively (Ng, Reference Ng1991). In two species of Lithocarpus from Thailand, the median length of dormancy (sowing to seedling emergence) was 143 and 219 d; however, for two species of Castonopsis, it was only 16 and 31 d and for two species of Quercus 14 and 18 d (Blakesley et al., Reference Blakesley, Elliott, Kuarak, Navakitbumrung, Zangkum and Anusarnsunthorn2002). Thus, it appears that the two species of Castanopsis and the two species of Quercus from Thailand have non-dormant seeds. Considering the long time taken for germination in Castanopsis and Lithocarpus in Malaysia and Lithocarpus in Thailand, it would not be surprising to learn that embryos of these species had both root and shoot dormancy, for example, C. foxworthyi and L. cyclophorus with germination (apparently seedling emergence) periods of 227–432 and 151–255 d, respectively (Ng, Reference Ng1991).
In diaspores of the temperate species of Quercus with epicotyl PD, the root is nondormant [Cnd(root)], and the shoot has non-deep PD [C1a(shoot)] (Table 1). However, in the case of the dormant seeds of Castanopsis and Lithocarpus, the general dormancy formula would be Cx(root) = Cx ′(shoot), as above for temperate Quercus species, but with both subscripts x and x′ representing the appropriate levels of PD (C1b, C2b or C3b); in case the root is nondormant, x = nd. Furthermore, while it is unlikely that diaspores of any temperate-zone oak with epicotyl dormancy will be found in which the embryo has a dormant radicle, it seems likely that the epicotyl could have intermediate [C2a(shoot)], in addition to non-deep [C1a(shoot)] PD.
Cryptogeal germination (‘plumule burying’ or ‘plumule protecting’)
A somewhat similar germination morphology to that of palms (see below) that produce remote tubular and remote ligular seedlings (also referred to as remote hypogeal or cryptogeal; Tahir et al., Reference Tahir, Mu'azu, Khan and Iortsuun2007; Fisher, Reference Fisher2008) has been described for species in several eudicot families, for example, Apiaceae (Holm, Reference Holm1901; Galil, Reference Galil1970; Haines and Lye, Reference Haines and Lye1979), Berberidaceae (Dickson, Reference Dickson1882; Holm, Reference Holm1899; Galil, Reference Galil1970), Combretaceae (Jackson, Reference Jackson1974), Cucurbitaceae (Hill, Reference Hill1916; Stocking, Reference Stocking1955; Schlising, Reference Schlising1969), Fabaceae (Hofmeyr, Reference Hofmeyr1921; van der Schijff and Snyman, Reference Van der Schijff and Snyman1970; Jackson, Reference Jackson1974), Fagaceae (Engelmann, Reference Engelmann1880; Troup, Reference Troup1921; Snow, Reference Snow1991), Malpighiaceae (Barbosa et al., Reference Barbosa, Mendonça and Rodrigues2014), Ochnaceae (Jackson, Reference Jackson1974), Ranunculaceae (Holm, Reference Holm1891; Sargant, Reference Sargant1904; Haines and Lye, Reference Haines and Lye1979), Rubiaceae (Jackson, Reference Jackson1974), Sapotaceae (Jackson, Reference Jackson1968, Reference Jackson1974; Ugese et al., Reference Ugese, Ojo and Bello2005, Reference Ugese, Baiyeri and Mbah2010) and Thymelaeaceae (Clarkson and Clifford, Reference Clarkson and Clifford1987); the magnoliid family Piperaceae (Hill, Reference Hill1906; Haines and Lye, Reference Haines and Lye1979); and the gymnosperm families Araucariaceae (Burrows et al., Reference Burrows, Boag and Stockey1992) and Zamiaceae (Fisher, Reference Fisher2008). In these and other cryptogeal (‘plumule burying’) species, the cotyledonary petioles are fused, forming a hollow tube in which the whole embryonic axis or (only) the plumule is located. Upon germination, the tube elongates and breaks through the seed coat, carrying the embryonic axis into the soil. In some species, the cotyledons remain in the seed, while, in others, they do not. The plumule breaks through the cotyledonary tube in some species, while, in others, it grows up the tube, that is, inside the tube. Furthermore, the cotyledon tube is planted relatively deeply in the soil in some species, while, in others, it is planted shallowly (Jackson, Reference Jackson1974; also see Burrows et al., Reference Burrows, Boag and Stockey1992). Clarkson and Clifford (Reference Clarkson and Clifford1987) think that Jackson's (Reference Jackson1974) characterization of the term ‘cryptogeal germination’ is weakened by including species in which plumule burying is minimal. Burtt (Reference Burtt1972) has pointed out that ‘It [plumular protection] is characteristic of the monocotyleodonous seedling, whether germination is hypogeal or epigeal, that the primary seedling axis, with plume and radicle, is carried out of the seed into the soil by the growth of the cotyledonary sheath’.
Perhaps the dictyledonous genus with this kind of seed germination morphology that has attracted the most attention is the western North American genus Marah (syn. Megarrhiza) in the Cucurbitaceae (Gray, Reference Gray1877; Darwin, Reference Darwin1880[1888]; Hill, Reference Hill1916; Stocking, Reference Stocking1955; Schlising, Reference Schlising1969; Borchert, Reference Borchert2004, Reference Borchert2006). A thorough autecological study of Marah oreganus has been done by Schlising (Reference Schlising1969). Seeds germinate after dispersal and burial in the soil by rodents (seed predators). Upon germination (in the natural habitat of the species), the cotyledonary tube elongates and breaks through the seed coat, carrying the embryonic axis (i.e. epicotyl, hypocotyl and radicle) inside it several centimetres deeper into the soil, where the root emerges into the soil and the shoot (whose early growth is inside the tube) emerges from the tube and (eventually) elongates to aboveground. In the meantime, the hypocotyl grows into a swollen underground tuber. The two fleshy cotyledon blades remain inside the buried seed. Like other Cucurbitaceae species, the seeds of Marah have little or no endosperm, and the cotyledons are large (Mabberley, Reference Mabberley2008). In Marah, they occupy most of the space within the fresh seed (Schlising, Reference Schlising1969). Thus, unlike palms (see below) in which the haustorium (cotyledon blade) remains inside the seed following emergence of the cotyledonary petiole (germination), the embryo of Marah does not grow inside the seed following emergence of the cotyledonary tube. In the field in California, seeds of M. oreganus are dispersed in May or June and germinate with the onset of winter rains in November or December in this area with a Mediterranean climate (Schlising, Reference Schlising1969). By mid-January, the epicotyl is growing up the cotyledonary tube, and by early March the first-year shoot is aboveground. According to Stocking (Reference Stocking1955), the seeds of Marah species are dormant at maturity, and dormancy can be broken by cold stratification.
We have assigned cryptogeal germination to the nondormancy (see under Division I in Table 1) and to hierarchical categories in three of the five classes of dormancy, that is, morphological, physiological and morphophysiological (see under Division 2 in Table 1). Here, we will explain briefly the basis for assignment of the dormancy formula to the genus Marah. Seeds of this genus have non-deep (regular) PD (C1) broken by low temperatures (subscript a of C1a; see Stocking, Reference Stocking1955), and the root (r) and shoot (s) emerge from the cotyledonary tube following its emergence from the seed (rs)′. That is, superscript (rs)′ means that the embryonic axis is buried in the soil inside the cotyledonary tube and the root and shoot emerge from the tube after the tube emerges from the seed [prime of (rs)′]. Thus, the formula for this kind of cryptogeal dormancy is ${\rm C}_{1{\rm a}}^{( {\rm rs}{) }^{\prime}} $. Note that the assignment of cryptogeal germination to classes morphological and morphophysiological dormancy (Table 1) are inferred based on an underdeveloped embryo in seeds of Apiaceae and Ranunculaceae species (Baskin and Baskin, Reference Baskin and Baskin2014a).
Two types of deep PD
Until relatively recently, deep PD had been applied to seeds that require a long period of cold stratification to come out of a dormancy that cannot be broken by gibberellic acid (GA3) and whose isolated embryo (in fresh seeds) does not grow, or if it does grow a dwarf plant is produced. However, the case has been made for deep PD in seeds of the Hawaiian montane shrub Leptocophylla tameiameiae (Ericaceae) that need a long warm stratification period to break dormancy, which cannot be overcome by GA3 (Baskin et al., Reference Baskin, Baskin, Yoshinaga and Thompson2005). In addition, seeds of a number of tropical evergreen rainforest trees in Malaysia with fully developed embryos needed many weeks to germinate under near-natural conditions in Kuala Lumpur (Ng, Reference Ng1991, Reference Ng1992), and thus we suspect that they also have deep PD broken by a long warm stratification period. To accommodate this additional kind of deep PD, Nikolaeva's symbol for deep PD, C3, was subdivided. Thus, we suggest that the current designation for it, C3, be subdivided into C3b and C3a to describe deep PD of seeds that require a long warm period and a long cold period, respectively, to come out of dormancy: C3b would be Type 1 and C3a Type 2 of deep PD (Table 1). This word-symbol designation would be consistent with the way it is used for types of non-deep PD, that is, subscripts 1b and 1a for seeds needing warm stratification and cold stratification, respectively, to break dormancy.
Temperature requirements for embryo growth
Nikolaeva (Reference Nikolaeva and Khan1977, Reference Nikolaeva2001) used uppercase ‘B’ as the symbol for the underdeveloped embryo in morphological dormancy (MD) (B only), and she used it in combination with symbols for several kinds of PD to represent formulae for morphophysiological dormancy (MPD). In simple MPD (and also in specialized MPD, see below), the embryo grows at warm temperatures and in complex MPD at cold temperatures. Thus, we have added subscripts b and a to B to distinguish between warm (Bb) and cold (Ba) temperature requirements for embryo growth. These additions to B will allow one to readily distinguish, by use of formulae only, whether seeds have a kind of simple or of complex MPD. We also have added subscript b to B to show that seeds with MD require only warm temperatures (Bb) for embryo growth.
Dust seeds of mycoheterotrophs and holoparasites with undifferentiated (organless) embryos
Species in nine families [Burmaniaceae, Corsiaceae, Ericaceae, Gentianaceae, Iridaceae (including Geosiridaceae), Orchidaceae, Petrosaviaceae, Thismiaceae and Triuridaceae (including Lacadoniaceae)] of mycoheterotrophic (MH) and ten families (Apodanthaceae, Balanophoraceae, Cynomoriaceae, Cytinaceae, Hydnoraceae, Lennoaceae, Mitrastemonaceae, Mystropetalaceae, Orobanchaceae and Rafflesiaceae) of holoparasitic (HP) angiosperms (no gymnosperms) are known to produce extremely small seeds (‘dust seeds’, typically <1 mm in greatest dimension) with an undifferentiated (organless) embryo (Baskin and Baskin, Reference Baskin and Baskin2021a,Reference Baskin and Baskinb). Baskin and Baskin (Reference Baskin and Baskin2021a,Reference Baskin and Baskinb) recently reviewed the literature on seed dormancy and germination in these 19 families. They found that other than the mycoheterotrophic orchids and Ericaceae subfamily Montropoideae (sensu Kron et al., Reference Kron, Judd, Stevens, Crayn, Anderberg, Gadek, Quinn and Luteyn2002) and the holoparasitic Orobanchaceae (sensu McNeal et al., Reference McNeal, Bennett, Wolfe and Mathews2013) little (or nothing) is known about germination morphology and physiology in these two trophic groups of plants. In both MH and HP with an undifferentiated embryo, only the radicular pole of the embryo participates in germination, except in orchids (MH) in which only the plumular pole (of the protocorm) participates in germination (see below). Thus, germination in MH and HP is monopolar, with meristem differentiation and organ formation occurring only during seed germination and not during embryogenesis (Rangaswamy, Reference Rangaswamy1967).
Germination in orchids is unique among seed plants. The germination process in orchids usually is divided into several stages, the number of which varies among authors. It typically includes development from the non-germinated seed → germinated seed → protocorm → seedling (plantlet). More specifically, primarily following Arditti (Reference Arditti1967), the germination process includes the following stages: (0) unimbibed seed; (1) embryo swollen (imbibed) and green or white but still inside the testa; (2) embryo more swollen, seed coat broken and lost; (3) early protocorm [spherical tuber-like body formed by germinating orchid seed (Arditti and Ernest, Reference Arditti, Ernest and Arditti1993)] with a pointed vegetative apex; (4) discoid late protocorm with ‘leaflets’; (5) seedling (plantlet) with two ‘leaflets’ and (6) seedling (plantlet) with two or more ‘leaflets’ as well as one or more (adventitious) roots. Germination (or a germinated seed) per se has been variously defined as an imbibed (swollen) seed (Gogoi et al., Reference Gogoi, Kumaria and Tandon2012; Lesar et al., Reference Lesar, Čeranič, Kastelec and Luthar2012; Huang et al., Reference Huang, Zi, Lin and Gao2018) to protocorm formation and leaf production (Thornhill and Koopowitz, Reference Thornhill and Koopowitz1992; Huynh and McLean, Reference Huynh and McLean2002; Shao et al., Reference Shao, Wang, Beng, Zhao and Jacquemyn2020). However, germination is frequently defined as ‘emergence of embryo from seed coat’ (e.g. Whigham et al., Reference Whigham, O'Neill, Rasmussen, Caldwell and McCormick2006; Yamazaki and Miyoshi, Reference Yamazaki and Miyoshi2006; Chen et al., Reference Chen, Liu, Kohler, Yan, Luo, Chen and Guo2017; Li et al., Reference Li, Guo and Lee2020) or as ‘rupture of seed coat by enlargement of the embryo’ (e.g. Gale et al., Reference Gale, Yamazaki, Hutchings, Yukawa and Miyoshi2010; Hynson et al., Reference Hynson, Madsen, Selosse, Roy, Gebauer and Merckx2013a; Zeng et al., Reference Zeng, Zhang, Teixeira da Silva, Wu, Zhang and Duan2014; Dulić et al., Reference Dulić, Ljubojević, Ornjanov, Barać and Dulić2019; Durán-López et al., Reference Durán-López, Caroca-Cáceres, Jahreis, Narváez-Vera, Ansoloni and Cazar2019), that is, stage 2 above.
Nikolaeva (Reference Nikolaeva1999) stated that the dust seeds of Orchidaceae ‘… exhibit a special pattern of germination ….’, and Baskin and Baskin (2014) referred to dust seeds with an undifferentiated embryo as having a ‘Specialized type of MD or MPD’ in their key to the classes of seed dormancy. However, the present paper is the first attempt to fit dust seeds with an undifferentiated embryo into a word-formula scheme for dormancy classification. Thus, we have created subclasses under classes MD and MPD to accommodate the specialized kinds of dormancy in dust seeds of monotropoids (MH), orchids (MH) and broomrapes (Orobanche spp., HP) with an undifferentiated embryo and monopolar germination (Table 1). It has been stated/proposed/implied that the seed coat of mature seeds of some orchid species is water-impermeable (e.g. Meekers et al., Reference Meekers, Hutchings, Honnay and Jacquemyn2012; Custódio et al., Reference Custódio, Marks, Pritchard, Hosomi and Machado-Neto2016; Yeh et al., Reference Yeh, Chen and Lee2021), but this needs further study. In any case, coat anatomy of water-impermeable orchid seeds is not the same (e.g. Yeh et al., Reference Yeh, Chen and Lee2021) as that of diaspores (fruit or seeds) of species in the 18 families of angiosperms reported to have physical dormancy (Baskin and Baskin, Reference Baskin and Baskin2014a).
The germination of dust seeds of MH species in Ericaceae subfamily Monotropoideae with an undifferentiated embryo also may be described by a sequence of stages from seed to ‘seedling’. For example, in a buried-seed study of six species of tribe Monotropeae and two species of tribe Pterosporeae, Bidartondo and Bruns (Reference Bidartondo and Bruns2005) divided the sequence into the following four stages: non-germinated seed → seed coat cracked → embryo/‘hypocotyl’ emerging mycorrhized → seedling branching. In a buried-seed study of two species of tribe Pyroleae, Hynson et al. (Reference Hynson, Weiß, Preiss, Gebauer and Treseder2013b) defined germination as follows: ‘A seed was considered to be germinated when the testa was broken and the embryo was enlarged or emerging’.
As in MH species with dust seeds and an undifferentiated embryo, the seed germination process in HP can be divided into several stages. For example, the following stages can be distinguished for Orobanche (Kadry and Tewfic, Reference Kadry and Tewfic1956a,Reference Kadry and Tewficb; also see Teryokhin, Reference Teryokhin1997): (1) swelling of micropylar (‘radicular’) region of embryo in intact seed; (2) emergence of germ tube with apical meristematic cells at ‘radicular’ end; (3) growth of germ tube and contact of its tip with host root; (4) formation of haustorium and penetration into host root; (5) enlargement of tip of germ tube at its point of attachment to host root, forming a tubercle and (6) endogenous production of a single stem apex and numerous root-like structures from the tubercle.
Dust seeds with undifferentiated (organless) embryos in the carnivorous plant genus Utricularia (Lentibulariaceae)
In the evolution of Lentibulariaceae, the embryo has undergone reduction from one or two cotyledons, radicle and meristematic shoot apex in the most primitive genus Pinguicula to two cotyledons and an aborted root in Genlisea to organless in most species of Utricularia (Kausik, Reference Kausik1938; Khan, Reference Khan1954; Farooq, Reference Farooq1964; Kumazawa, Reference Kumazawa1967; Kondo et al., Reference Kondo, Segawa and Nehira1978; Siddiqui, Reference Siddiqui1978; Degtjareva et al., Reference Degtjareva, Casper, Hellwig and Sokoloff2004, Reference Degtjareva, Casper, Hellwig, Schmidt, Steiger and Sokoloff2006; Płachno and Świątek, Reference Płachno and Świątek2010). The dust seeds of Utricularia (Abraham and Subramanyam, Reference Abraham and Subramanyam1965; Dwyer, Reference Dwyer1983; Menezes et al., Reference Menezes, Gasparino, Baleeiro and Miranda2014) consist of an epidermis, meristematic tissue at the apex and storage parenchyma (Kausik, Reference Kausik1938; Khan, Reference Khan1954; Farooq, Reference Farooq1964; Siddiqui, Reference Siddiqui1978; Płachno and Świątek, Reference Płachno and Świątek2010). Swamy and Mohan Ram (Reference Swamy and Mohan Ram1969) described the germination of Utricularia inflexa as the emergence of a mound of cells at the plumular pole (proximal to micropyle), which had several papillate (cotyledon-like) outgrowths. Then, a shoot was produced, but there was no root formation. Little is known about seed dormancy in Utricularia but based on the organless embryo. Baskin and Baskin (Reference Baskin and Baskin2014a) concluded that the seeds have a specialized kind of MD or MPD. Research is needed to determine if fresh seeds have physiological dormancy (or not) in addition to the organless embryo. Furthermore, not much is known about seed dormancy in the Lentibulariaceae generally.
Seven subclasses of MD
Heretofore, the class MD has not been subdivided (Baskin and Baskin, Reference Baskin and Baskin2014a). Dust-size seeds with an undifferentiated embryo not only occur in MH and HP but also in fully autotrophic plants with bipolar (plumular–radicular) germination, for example in the genus Peperomia (Piperaceae). Thus, specialized subclasses 2, 3, 4 and 5 of MD have been added to the Nikolaeva–Baskin seed dormancy classification scheme to accommodate MH, HP and autotrophs with dust seeds and an undifferentiated embryo.
Diaspore (fruit with seed inside it) structure and germination morphology of species in the large genus Peperomia [around 1600 species (Mabberley, Reference Mabberley2008)] were described in detail over a century ago (Johnson, Reference Johnson1900, Reference Johnson1902; Hill, Reference Hill1906; also see Hill, Reference Hill1939). The undifferentiated embryo (about 15 cells in P. pellucida) in the ripe diaspore (0.85 long × 0.60 mm wide in P. pellucida) is located at the micropylar end of the seed and is enclosed by a small endosperm. Most of the interior of the ripe seed is occupied by perisperm (nucellar tissue). During germination, the endosperm enlarges and breaks through the seed covering layers (seed coat + pericarp). Meanwhile, the rounded (organless) embryo enlarges and differentiates into a typical dicotyledonous embryo with radicle while still enclosed by the endosperm, which protrudes to the outside of the diaspore covering layers. Then, the radicle of the fully differentiated embryo breaks through the surrounding endosperm (radicle emergence). From this point on, Peperomia species exhibit two distinct germination morphologies. One, which apparently occurs in most species of the genus (non-geophilous), produces a typical epigeal dicotyledonous seedling with each of the two cotyledons acting as a haustorium until the food supply (endosperm and perisperm) of the seed is exhausted, after which the seed covering layers are shed and the cotyledons become assimilating (photosynthetic) organs. The germination morphology of Saururaceae is similar to that of Piperaceae (Baskin and Baskin, Reference Baskin and Baskin2018). Two, in the relatively few geophilous (i.e. bulb, corm or tuberous rhizome produced from a short hypocotyl), species of Peperomia, on the other hand, one of the two cotyledons is epigeal and the other hypogeal, remaining (permanently) inside the seed and acting as a haustorium. The seedlings resemble those of some monocots, for example, Arisaema and Arum of the Araceae (Scott and Sargent, Reference Scott and Sargant1898; Hill, Reference Hill1906; Pritchard et al., Reference Pritchard, Wood and Manger1993; Pickett, Reference Pickett1913; Tillich, Reference Tillich2003, Reference Tillich2014) and have been said to have a ‘pseudo-monocotyledonous’ mode of germination (Hill, Reference Hill1906, Reference Hill1939).
We have created a new subclass of morphological dormancy (i.e. Subclass 5) to accommodate autotrophic Peperomia species with dust seeds and an undifferentiated embryo without PD (Table 1). Furthermore, Subclass 5 has been divided into two levels, one for truly dicotyledonous seedlings and the other for ‘pseudo-monocotyledonous’ seedlings. The assumption for creation of this subclass is that in some species of Peperomia, there is no physiological component of dormancy break, only a morphological one. In other species, however, we have assumed that there also is a physiological component of dormancy and thus have created a new subclass to accommodate this possibility (see Subclass 5 of Class Morphophysiological Dormancy).
Are seeds of Bromeliaceae nondormant, or do they have MD?
The Bromeliaceae is a monocot family with about 3140 species that is primarily restricted to the Neotropics, and many of the species are epiphytes (Benzing, Reference Benzing2000; Givnish et al., Reference Givnish, Barfuss, Van Ee, Riina, Schulte, Horres, Gonsiska, Jabaily, Crayn, Smith, Winter, Brown, Evans, Holst, Luther, Till, Zizka, Berry and Sytsma2014). Seeds of most of the species whose germination has been studied are reported to be nondormant (Smith and Downs, Reference Smith and Downs1974; Tarré et al., Reference Tarré, Pires, Guinarães and Mansur2007; Mantovani and Iglesias, Reference Mantovani and Iglesias2008; Pereira et al., Reference Pereira, Pereira, Rodrigues and Andrade2008, Reference Pereira, Andrade, Pereira, Forzza and Rodrigues2009; Silva and Scatena, Reference Silva and Scatena2011; Sosa-Luría et al., Reference Sosa-Luría, Chávez-Servia, Mondragón, Estrada-Gómez and Ramírez-Vallejo2012; Correa and Zotz, Reference Correa and Zotz2014; Marques et al., Reference Marques, Atman, Silveira and Lemos-Filho2014; Rodrigues et al., Reference Rodrigues, Forzza and Andrade2014; Dayrell et al., Reference Dayrell, Garcia, Negreiros, Baskin, Baskin and Silveira2016; Müller et al., Reference Müller, Albach and Zotz2017; Garcia et al., Reference Garcia, Barreto and Bicalho2020; de Andrade et al., Reference de Andrade, Sánchez-Tapia and de Andrade2021; Zupo et al., Reference Zupo, Daibes, Pausas and Fidelis2021). In many species, the embryo is small, with a low embryo volume:seed volume ratio and a low embryo length:seed length ratio (Smith and Downs, Reference Smith and Downs1974; Benzing, Reference Benzing2000; Baskin and Baskin, unpublished embryo database). According to Benzing (Reference Benzing2000), the embryo typically occupies one-fourth to one-third of the volume of the seed. The question is whether or not the seeds are nondormant or have morphological dormancy. That is, does the embryo need to undergo a period of growth (afterripening, sensu Eames, Reference Eames1961) before the germination process begins. If it does, then the embryo is underdeveloped, and the seeds that have been said to be nondormant would have morphological dormancy. If, on the other hand, the embryo does not grow prior to germination or if it grows only during the germination process, then the seeds that have been said to be nondormant would be truly nondormant. We have not found any publications that deal specifically with trying to answer the question about whether seeds are nondormant or have morphological dormancy. However, the germination process for Dyckia geohringii as illustrated by Duarte et al. (Reference Duarte, Carneiro and Rezende2009) shows that the embryo grows during the germination process, which took place in 4–5 d. A caveat concerning calling seeds of Bromeliaceae nondormant is that, in some studies, the seeds were stored before they were tested for germination, during which time any physiological dormancy may have been broken.
Twelve subclasses of MPD
Heretofore, two main categories of MPD, that is, simple and complex, have been recognized (Baskin and Baskin, Reference Baskin and Baskin2008), and they were given subclass status by Baskin and Baskin (Reference Baskin and Baskin2014a). Here, we increase the number of subclasses of MPD to 12 to accommodate other kinds of MPD, including those in the mycoheterotrophic orchids and Ericaceae (subfamily Monotropoideae); holoparasitic Orobanchaceae (Cistanche, Orobanche); and autotrophic Peperomia, Hydatellaceae (Trithuria), palms and (hemiparasitic) Melampyrum. Subclasses 5 and 6 of MPD were created to accommodate autotrophic species with dust seeds and an undifferentiated embryo with PD (Table 1). Furthermore, each of these subclasses has been divided into two levels, one for truly dicotyledonous seedlings and the other for pseudo-monocotyledonous seedlings.
A new kind of ‘specialized MPD’ was reported for (dust-size) seeds of the autotrophic ANA (Amborellales–Nymphaeales–Austrobaileyales) grade angiosperm Trithuria, a monotypic genus in the Hydatellaceae (Nymphaeales) in which the embryo is undifferentiated or nearly so (Rudall et al., Reference Rudall, Eldridge, Tratt, Ramsay, Tuckett, Smith, Collinson, Remizowa and Sokoloff2009; Tuckett et al., Reference Tuckett, Merritt, Rudall, Hay, Hopper, Baskin, Baskin, Tratt and Dixon2010; Friedman et al., Reference Friedman, Bachelier and Hormaza2012; Sokoloff et al., Reference Sokoloff, Remizowa, Conran, Macfarlane, Ramsay and Rudall2014). At seed maturity, the embryo, which was reported to be undifferentiated by Rudall et al. (Reference Rudall, Eldridge, Tratt, Ramsay, Tuckett, Smith, Collinson, Remizowa and Sokoloff2009) and Tuckett et al. (Reference Tuckett, Merritt, Rudall, Hay, Hopper, Baskin, Baskin, Tratt and Dixon2010), has a protoderm and two cotyledon primordia, but the procambial and ground meristem cells are undifferentiated (Friedman et al., Reference Friedman, Bachelier and Hormaza2012). In Trithuria, the embryo exhibits an increase in the number of cells, the embryo length, the embryo length (E):seed length (S) ratio and the E:S (area) ratio inside the seed prior to germination (Tuckett et al., Reference Tuckett, Merritt, Rudall, Hay, Hopper, Baskin, Baskin, Tratt and Dixon2010). The expansion of the embryonic cotyledons causes displacement of the operculum and pushes the embryo (capped by a portion of the single-layered endosperm; most stored food is perisperm) to the outside of the seed coat (germination), where most of the growth and the differentiation of the radicle and first leaf occur (Rudall et al., Reference Rudall, Eldridge, Tratt, Ramsay, Tuckett, Smith, Collinson, Remizowa and Sokoloff2009; Tuckett et al., Reference Tuckett, Merritt, Rudall, Hay, Hopper, Baskin, Baskin, Tratt and Dixon2010; Friedman et al., Reference Friedman, Bachelier and Hormaza2012; Sokoloff et al., Reference Sokoloff, Remizowa, Beer, Yadav, Macfarland, Ramsey and Rudall2013, Reference Sokoloff, Remizowa, Conran, Macfarlane, Ramsay and Rudall2014). Tuckett et al. (Reference Tuckett, Merritt, Rudall, Hay, Hopper, Baskin, Baskin, Tratt and Dixon2010) found that fresh seeds of T. austinensis, T. bibracteata and T. submersa took 50, 35 and 37 d, respectively, to reach 50% of total germination (their TG50) and 119, 85 and 77–88 d, respectively, to reach maximum germination percentage. Thus, it was concluded that Trithuria species have a special kind of MPD (Tuckett et al., Reference Tuckett, Merritt, Rudall, Hay, Hopper, Baskin, Baskin, Tratt and Dixon2010). Some pre-germination growth and cell division of the embryo occur inside the seed. However, differentiation of shoot and root occurs outside the seed, after the embryo (enclosed by the thin endosperm) ruptures the seed coat, displacing the operculum and emerging from the seed (germination) (Rudall et al., Reference Rudall, Eldridge, Tratt, Ramsay, Tuckett, Smith, Collinson, Remizowa and Sokoloff2009; Tuckett et al., Reference Tuckett, Merritt, Rudall, Hay, Hopper, Baskin, Baskin, Tratt and Dixon2010).
A similar embryo and germination morphology as described for Trithuria occurs in the autotrophic monocot families Eriocaulaceae, Mayacaceae and Xyridaceae (Baskin and Baskin, Reference Baskin and Baskin2018). Fresh seeds of various tropical species of Eriocaulaceae and Xyridaceae from the cerrado of Brazil have been reported to be nondormant, that is, no primary dormancy (Garcia et al., Reference Garcia, Barreto and Bicalho2020; Gonçalves-Magalhães et al., Reference Gonçalves-Magalhães, de Santana and Ribeiro-Oliveira2020; Oliveira et al., Reference Oliveira, Duarte and Diamantino2021; Zupo et al., Reference Zupo, Daibes, Pausas and Fidelis2021). However, seeds of Eriocaulon septangulare from temperate eastern North America were dormant and required cold stratification (1–3°C submerged in water) for germination; they did not come out of dormancy when stored wet (in water) or dry at laboratory temperatures or when stored dry at 1–3°C (Muenscher, Reference Muenscher1936). Seeds of Xyris lacera (Merritt et al., Reference Merritt, Turner, Clarke and Dixon2007), X. lanata, X. maxima (Cochrane, Reference Cochrane2020) from Australia and X. tennesseensis (Baskin and Baskin, Reference Baskin and Baskin2003) from the eastern USA exhibited some physiological dormancy (see below). Since seeds of Eriocaulaceae and Xyridaceae have broad undifferentiated (organless) embryos, they would not be considered to be nondormant following the classification scheme of seed dormancy presented in Table 1. Fresh seeds of the tropical Brazilian species would have a specialized kind of MD and fresh seeds of the temperate species a specialized kind of MPD. Furthermore, the so-called nondormant, freshly matured seeds of Eriocaulon and Xyris from the cerrado (Brazilian savanna) were reported to be induced into dormancy and then cycle between dormancy and nondormancy. However, since the seeds of Eriocaulon and Xyris have an undifferentiated (organless) embryo at seed maturity (Baskin and Baskin, Reference Baskin and Baskin2018), according to our classification scheme (Table 1), they would have a specialized kind of MD and cycle between MD and MPD: sMD ↔ sMPD.
Baskin and Baskin (Reference Baskin and Baskin2007) concluded that although small (low E:S ratio), the cupulate embryo in Nymphaea (Baskin and Baskin, Reference Baskin and Baskin2018; broad embryo sensu Martin, Reference Martin1946) did not exhibit a significant amount of growth prior to radicle (actually hypocotyl, see below) emergence and thus is fully developed. A cupulate embryo has two thick hemispherical fleshy cotyledons and embryonic leaves and/or leaf primordia inside. Based on these results, the Nymphaeales would be the only clade in the basal (ANA: A, Amborellales; N, Nymphaeacles; A, Austrobaileyeales) grade of angiosperms that does not have an underdeveloped embryo (Baskin and Baskin, Reference Baskin and Baskin2007). Since the publication of the Baskin and Baskin (Reference Baskin and Baskin2007) paper, however, a specialized kind of MPD (see above) has been described for Trithuria (Hydatellaceae, Nymphaeales) (Tuckett et al., Reference Tuckett, Merritt, Rudall, Hay, Hopper, Baskin, Baskin, Tratt and Dixon2010, see above), and apparently this same kind of embryo also occurs in Nymphaea lotus [see Figure 10 in Rudall et al. (Reference Rudall, Eldridge, Tratt, Ramsay, Tuckett, Smith, Collinson, Remizowa and Sokoloff2009)].
Furthermore, in her PhD thesis, Dalziell (Reference Dalziell2016) reported that three of her six study species of Nymphaea (N. macrocarpa, N. odinea and N. violaceae) from the seasonally wet tropics of northern Australia have MPD; the embryos in these three species increased in length by 21, 37 and 32%, respectively, prior to hypocotyl emergence (germination). In agreement with the results of studies on pre-germination growth of embryos of five Nymphaea species by Baskin and Baskin (Reference Baskin and Baskin2007), Dalziell et al. (Reference Dalziell, Baskin, Baskin, Young, Dixon and Merritt2019) found that the embryo in two of the six species of Nymphaea (N. immutabilis and N. violacea) grew in length to only 8% and 10%, respectively. Thus, these authors did not consider the embryo to be underdeveloped, that is, they have a small, fully developed cupulate embryo, which they called a broad embryo. For the other Nymphaea species in their study (N. pubescens), there was no embryo growth or germination at all, although the seeds were viable.
Philomena and Shah (Reference Philomena and Shah1985) defined germination in Nymphaea as emergence of the coleoptile, Baskin and Baskin (Reference Baskin and Baskin2007) as emergence of the radicle and Dalziell et al. (Reference Dalziell, Baskin, Baskin, Young, Dixon and Merritt2019) as emergence of the hypocotyl. Based on drawings in Haines (Reference Haines1975) and Tillich (Reference Tillich1990), it can be seen that, in Nymphaeaceae, the hypocotyl (within which the radicle is enclosed) is the first thing to emerge from the seed coat during germination. The hypocotyl is pushed out of the seed (germination) via elongation of the two cotyledon lobes (Haines, Reference Haines1975; Sokoloff et al., Reference Sokoloff, Remizowa, Conran, Macfarlane, Ramsay and Rudall2014). Subsequently, the radicle emerges from the hypocotyl, the ends of the cotyledon lobes enlarge and fuse to form the haustorium, which remains inside the seed, and a ‘shoot’ consisting of the coleoptile and the first leaf elongates from the cavity between the cotyledon lobes.
Thus, is the cupulate embryo in some Nymphaeaceae species underdeveloped? In the case of this family, growth of the embryo appears to lead directly to germination and to occur concurrently with activation of the whole-seed germination process. In other words, embryo growth is part of the germination process per se and not afterripening, that is, growth of embryo inside the seed between seed maturity and the onset of germination (sensu Eames, Reference Eames1961). Sokoloff et al. (Reference Sokoloff, Remizowa, Conran, Macfarlane, Ramsay and Rudall2014) stated that ‘Trithuria, like Nymphaeaceae, exhibits asymmetric intercalary growth of cotyledon bases during release of the embryo from the seed coat …’. Trithuria is in the Hydatellaceae and has a broad undifferentiated embryo with cotyledon initials and protoderm. In contrast, in the typical underdeveloped embryo that occurs in numerous families of seed plants (Baskin and Baskin, Reference Baskin and Baskin2014a), growth of the embryo occurs in advance of the beginning of the germination process, and after embryo growth is completed the seed germinates. As such, then, embryo growth in the Nymphaeaceae is not the same as it is in a seed with MD or MPD that does not have epicotyl or double dormancy, in which the embryo must grow inside the seed (i.e. afterripening sensu Eames, Reference Eames1961) before the germination process begins. Neither is it like the underdeveloped embryo of palms (see below), in which growth of the haustorium (cotyledon blade) occurs after the cotyledonary petiole (with plumular–radicular axis inside it) emerges from the diaspore (germination) (Baskin and Baskin, Reference Baskin and Baskin2014b). Thus, we suggest that the cupulate embryo of Nymphaea is not underdeveloped sensu stricto. In which case, perhaps with a few exceptions, for example, Nymphaea lotus [see Figure 10 in Rudall et al. (Reference Rudall, Eldridge, Tratt, Ramsay, Tuckett, Smith, Collinson, Remizowa and Sokoloff2009)], the seeds of Nymphaeaceae species either have PD or they are nondormant.
Two types of non-deep simple MPD
While Nikolaeva (Reference Nikolaeva2001, see Baskin and Baskin, Reference Baskin and Baskin2008) recognized only a single kind of non-deep simple MPD, two kinds are now recognized (Baskin and Baskin, Reference Baskin and Baskin2014a; Table 1). Her formula for non-deep simple MPD is C1bB − C1b. However, Baskin and Baskin (Reference Baskin and Baskin2008) argued that C1bB is the more appropriate formula for this kind of MPD, since the fully developed embryo is not physiologically dormant. That is, for example, if the seed of Chaerophyllum tainturieri is in light after PD (C1b) is broken, the underdeveloped embryo (B) grows to full size and germinates in a continuous process without further pretreatment (Baskin and Baskin, Reference Baskin and Baskin1990). The fully developed embryo is not physiologically dormant as might be interpreted by Nikolaeva's second −C1b in her formula. C1bBb is Type 1 non-deep simple MPD in our scheme.
Baskin and Baskin (Reference Baskin and Baskin2014a) recognized a second kind of non-deep simple MPD, in which cold stratification is required to break PD of the embryo, and then, the embryo grows and the seed germinates at warm temperatures. The fully developed embryo is not physiologically dormant (see Baskin and Baskin, Reference Baskin and Baskin2008). Thus, the formula for this second kind (Type 2) of non-deep simple MPD is C1aBb (Table 1).
A second level of simple epicotyl MPD
Nikolaeva (see Baskin and Baskin, Reference Baskin and Baskin2008) included only one kind (level) of epicotyl MPD, that is, deep simple epicotyl MPD, in her classification scheme, as did Baskin and Baskin (Reference Baskin and Baskin2004) in their scheme. However, although Nikolaeva (Reference Nikolaeva2001) was of the opinion that epicotyl MPD is deep in seeds of most species with this kind of dormancy, she also stated that ‘… epicotyl dormancy may be intermediate or even non-deep as evidenced by response of seeds (e.g. Paeonia suffruticosa and Viburnum trilobum) to GA3’. Indeed, Karlsson et al. (Reference Karlsson, Hidayati, Walck and Milberg2005) found non-deep simple epicotyl MPD (although they did not apply this name to it) in Viburnum tinus (Adoxaceae) from Spain, and Baskin et al. (Reference Baskin, Chien, Chen and Baskin2008) identified it in V. odoratissimum from Taiwan. However, more detailed studies are needed to verify the occurrence of intermediate simple epicotyl MPD. It would not be surprising to find intermediate epicotyl MPD in seeds of tropical species in which shoot dormancy is broken by relatively long periods of exposure to warm temperatures, that is, [C2b(shoot)]. Seeds of the Neotropical forest species Minquartia guianensis (Olacaceae) already have been identified as requiring a long period of exposure to warm temperatures both to break dormancy of the root and of the shoot (Flores, Reference Flores and Vozzo2002); thus, they have Type 1 deep simple epicotyl MPD (Table 1). Our updated, revised scheme (Table 1) includes two levels and eight types of epicotyl MPD.
In seeds with epicotyl MPD that are dispersed in spring or early summer, root dormancy is broken in summer, and the root emerges in autumn and the shoot the following spring after epicotyl dormancy is broken by cold-stratifying temperatures in winter (e.g. Baskin and Baskin, Reference Baskin and Baskin1985b). However, in species with epicotyl MPD such as Viburnum acerifolium (Hidayati et al., Reference Hidayati, Baskin and Baskin2005), V. opulus (Walck et al., Reference Walck, Karlsson, Milberg, Hidayati and Kondo2012) and Paeonia corsica (Porceddu et al., Reference Porceddu, Mattana, Prtichard and Bacchetta2016, Reference Porceddu, Pritchard, Mattana and Bacchetta2020), the root of seeds dispersed late in the warm growing season does not emerge until the following growing season, after seeds are exposed to a warm dormancy-breaking period. Thus, the shoots would not emerge until the second spring, after the seeds with an emerged root are cold-stratified during winter.
Two types of deep simple double MPD
The first structure to emerge from seeds during germination of many monocots, including Convallaria and Trillium, is the cotyledonary petiole/sheath with the embryonic root–shoot axis inside it (Boyd, Reference Boyd1932; Barton and Schroeder, Reference Barton and Schroeder1942; Tillich, Reference Tillich, Rudall, Cribb, Cutler and Humphries1995, Reference Tillich2007). Following emergence of the cotyledonary petiole in seeds of both Convallaria and Trillium, the primary root emerges from the base of the root–shoot axis of the embryo. Following root emergence in Trillium, the cotyledon elongates and turns green. However, in Convallaria, the cotyledon remains inside the seed and does not elongate or turn green. Following root emergence in Convallaria, a bud is formed from the apical portion of the embryonic root–shoot axis, and from it a true leaf is produced (Kondo et al., Reference Kondo, Narita, Phartyal, Hidayati, Walck, Baskin and Baskin2015). In both Convallaria and Trillium, the first phase of germination, that is, emergence of the cotyledonary petiole/primary root, will be referred to as root emergence, and the second phase, that is, elongation of the cotyledon in Trillium and of the first true leaf in Convallaria, will be referred to as shoot emergence (see below).
The seed dormancy classification system of Nikolaeva (see Baskin and Baskin, Reference Baskin and Baskin2008) and of Baskin and Baskin (Reference Baskin and Baskin2004) lists only one kind (level sensu Baskin and Baskin, Reference Baskin and Baskin2004, Reference Baskin and Baskin2014a) of double MPD, that is, deep simple double MPD (see Baskin and Baskin, Reference Baskin and Baskin2008). Our revised scheme recognizes two types under this level (Baskin and Baskin, Reference Baskin and Baskin2014a; Table 1). In both types, embryo growth and root emergence occur the first spring following seed maturity/dispersal the previous growing season. However, in one type, the embryo grows before root emergence and germination is epigeal (e.g. Trillium), and, in the other type, the embryo grows after root emergence and germination is hypogeal (e.g. Convallaria) (Kondo et al., Reference Kondo, Narita, Phartyal, Hidayati, Walck, Baskin and Baskin2015, Reference Kondo, Walck and Hidayati2019). In monocots, which include most of the known examples of double dormancy, we follow Tillich (Reference Tillich2007) in defining epigeal and hypogeal germination. Thus, while in epigeal germination in eudicots, the seed is raised above the soil surface by elongation of the hypocotyl, in monocots it is raised above the soil surface by elongation of the cotyledon. Although there are differences in hypogeal germination in eudicots and monocots, in neither is the seed raised above the soil surface (Tillich, Reference Tillich, Rudall, Cribb, Cutler and Humphries1995, Reference Tillich2007).
In monocot species with double dormancy and epigeal germination, for example Trillium, the second phase of dormancy break [${\rm C}_{3{\rm a}}^{\rm e} $(shoot)] involves elongation of the cotyledon. On the other hand, in species with double dormancy and hypogeal germination, for example Convallaria, Polygonatum and Smilacina, the second phase of dormancy break [
${\rm C}_{3{\rm a}}^{\rm h} $(shoot)] involves elongation of the first non-cotyledonous leaf that arises from a bud formed by growth of the embryonic shoot apex. In Trillium, the first non-cotyledonous leaf is produced from the embryonic shoot apex (see Vogt, Reference Vogt1915; Barton and Schroeder, Reference Barton and Schroeder1942; Barton, Reference Barton1944; Whigham, Reference Whigham1974; Tillich, Reference Tillich, Rudall, Cribb, Cutler and Humphries1995; Suzuki and Kawano, Reference Suzuki and Kawano2010; Kondo et al., Reference Kondo, Mikubo, Yamada, Walck and Hidayati2011).
Since the small embryo in Convallaria keiskei does not grow until after the cotyledonary petiole emerges from the seed, that is, until after the seed germinates (Kondo et al., Reference Kondo, Narita, Phartyal, Hidayati, Walck, Baskin and Baskin2015), it does not fit the standard definition of being underdeveloped, that is, growth inside the seeds before the root emerges (Baskin and Baskin, Reference Baskin and Baskin2014a). In which case, following that definition, strictly speaking, the seeds would not have MPD. Thus, for seeds with epicotyl and double dormancy, we propose to redefine an underdeveloped embryo as one that completes growth before and/or after root emergence (or emergence of cotyledonary petiole) but in either case before shoot emergence. In fact, in seeds with these two types of MPD, a germinated seed probably should be defined as one in which both root and shoot have emerged; only then is germination completed for these kinds of seed dormancy.
Need for additional double MPD categories
As noted above, Nikolaeva recognized only one kind (level sensu Baskin and Baskin, Reference Baskin and Baskin2004, Reference Baskin and Baskin2014a) of double dormancy, that is, deep simple double MPD. However, she (Nikolaeva et al., Reference Nikolaeva, Razumova, Gladkova and Danilova1985) assigned deep simple double MPD to seeds of six species among and within which the seed physiologist Lela Barton found considerable variation in depth of root and shoot dormancy (Barton and Schroeder, Reference Barton and Schroeder1942; Barton, Reference Barton1944). For example, Barton (Reference Barton1944) found that the roots and shoots of Trillium grandiflorum seeds were more dormant than those of Polygonatum commutatum seeds and that shoot dormancy in T. grandiflorum seeds was deeper than that in T. erectum seeds. Barton (Reference Barton1944) also found that a portion of the seeds of Sanguinaria canadensis (apparently collected at Yonkers, New York) behaved as if they had epicotyl dormancy (i.e. did not require cold stratification for root emergence), while others had double dormancy (i.e. seeds required cold stratification for root emergence); in both cases, exposure to a period of low temperature was required to break shoot dormancy. Thus, some seeds in the seed lot had epicotyl dormancy and others double dormancy, and we have found the same thing for seeds of S. canadensis collected in central Kentucky (Baskin and Baskin, Reference Baskin and Baskin1998). Kondo et al. (Reference Kondo, Walck and Hidayati2019) found that although most of the seeds T. camschatcense exhibited double MPD, a small percentage of them had epicotyl MPD.
All of this indicates that levels and types need to be created to accommodate the diversity of the kinds of double MPD in seeds. Clearly, one formula does not fit all seeds with this kind of dormancy. Thus, much research remains to be done to sort out the details of the diversity of double MPD. We suggest that the Asian/Himalayan–North American–continental disjunct genus Trillium (Melanthiaceae) would be a good study taxon to use to help sort out this diversity. Thus, it seems that there is a gradient of kind and degree of dormancy in this genus that might extend through an epicotyl MPD → double MPD spectrum (Barton, Reference Barton1944; Baskin and Baskin, Reference Baskin and Baskin1998; Suzuki and Kawano, Reference Suzuki and Kawano2010; Kondo et al., Reference Kondo, Mikubo, Yamada, Walck and Hidayati2011, Reference Kondo, Walck and Hidayati2019; Thompson and Leege, Reference Thompson and Leege2011). It would be interesting to determine how such variation sorts out phylogenetically and geographically within the genus and what role genetics (G), maternal environment (E) and G × E play in determining the proportions of seeds with MD and MPD.
The term ‘double dormancy’ has been applied to seeds of temperate-zone species with an underdeveloped embryo in which the root emerges the first year post-dispersal, and the shoot emerges the second year. That is, the root emerges after the seeds have been exposed to low (cold-stratifying) temperatures during the first winter following dispersal, and the shoot emerges after the seeds with an emerged root are exposed to low (cold-stratifying) temperatures during the second winter following dispersal. A long lag time also occurs between dispersal and root emergence and between root and shoot emergence in the germination process of seeds of tropical species with an underdeveloped embryo, for example, Minquartia guianensis (Olacaceae, Table 1) and Rollinia salicifolia (Annonaceae) (Franceschini, Reference Franceschini2004). Thus, the root emerges after the seeds have been exposed to a long period of non-cold-stratifying temperatures, and the shoot emerges after the seeds with roots emerged are exposed to a second long period of non-cold-stratifying temperatures. In which case, it could be argued that seeds of such species have double dormancy. However, in this review, we have listed these species as having epicotyl MPD.
Epicotyl MPD in the hemiparasitic genus Melampyrum (Orobanchaceae)
Typically, in seeds of species with epicotyl MPD, the root emerges in autumn of the year of seed maturity at relatively warm temperatures, and the shoot emerges in spring following a period of cold temperatures during winter (Baskin and Baskin, Reference Baskin and Baskin2014a). However, seeds of Melampyrum have a type of epicotyl dormancy in which root and shoot each require a separate period of cold-stratifying temperatures (in succession) to germinate. Roots emerge during several weeks of incubation at about 0–10 [−12]°C, and then, an additional period at low temperatures is required for epicotyl growth in seeds with an emerged root. Pretreatment with GA3 or storage in the field or laboratory enhances germination at the low temperatures (Cantlon et al., Reference Cantlon, Curtis and Malcolm1963; Horrill, Reference Horrill1972; Oesau, Reference Oesau1973, Reference Oesau1975; Masselink, Reference Masselink1980). Storage of fresh seeds of M. pratense in the field for 1 or 2 months in mid to late summer in The Netherlands increased ‘activation values’ (rate × %) for germination and also widened the temperature range for germination. Furthermore, GA3 substituted for the low temperature requirement for breaking epicotyl dormancy in this species (Masselink, Reference Masselink1980). Thus, in the field, the roots of these summer annuals emerge in autumn–early winter and the shoots in spring. Although root and hypocotyl may continue to develop during winter, the epicotyl does not emerge until spring.
Relative to the size of the seed and endosperm, the embryo of Melampyrum is small, but well differentiated (Martin, Reference Martin1946; Arekal, Reference Arekal1963; Tiaga, Reference Tiaga1965). However, it is underdeveloped at seed maturity and thus grows inside the seed (at low temperatures) before the radicle emerges (Curtis and Cantlon, Reference Curtis and Cantlon1963, Reference Curtis and Cantlon1965, Reference Curtis and Cantlon1968). For M. lineare, embryo length is up to 40% of that of the seed and occupies 10% of its volume. The embryo length is less than 80% of that of the seed at radicle emergence, but following radicle emergence the cotyledons continue to grow slowly until they occupy all of the space inside the seed. Based on information in the publications cited above, we suggest that seeds of Melampyrum have non-deep complex epicotyl MPD: C1b1aBa(root) – C1aB′(shoot), that is, Level 4, Subclass 9 of Class Morphophysiological Dormancy (Table 1).
Like seeds with deep simple double MPD, two cold periods are required to break dormancy in seeds of Melampyrum, that is, to produce a seedling with both a root and a shoot. However, unlike seeds with double MPD, those of Melampyrum do not require that the two cold periods be separated by a warm period, during which the root system continues to grow and develop, in order to germinate. Thus, in nature seeds with double MPD like those of the polycarpic perennials, Convallaria, Polygonatum, Smillacina and Trillium require two winters and a summer to complete germination (root and shoot emergence), while those of the annual Melampyrum require only one successive autumn-to-spring period to do so.
Seed dormancy classification with particular reference to the monocots palms and Canna
There are about 2600 known species of palms (Baker and Dransfield, Reference Baker and Dransfield2016), about two-thirds of which occur within the Indomalayan and Neotropical regions (Kissling et al., Reference Kissling, Baker, Balslev, Barfod, Borchsenius, Dransfield, Govaerta and Svenning2012). The morphoanatomy of the germination process (seed → seedling) in monocots is complex (Arber, Reference Arber1925; Boyd, Reference Boyd1932; Kaul, Reference Kaul1978; Tillich, Reference Tillich, Rudall, Cribb, Cutler and Humphries1995, Reference Tillich2007). For example, germination in palms (Arecaceae) usually is defined as protrusion of the cotyledonary petiole (tube) through the opening created by displacements of the micropylar endosperm–seed coat–endocarp operculum (Perez et al., Reference Perez, Criley and Baskin2008; Neves et al., Reference Neves, Ribeiro, Gomez da Cunha, Silva Pimento, Mercadante-Simões and Nascimento Lopes2013; Oliveira et al., Reference Oliveira, Lopes, Ribeiro, Mercandante-Simões, Oliveira and Silvério2013; Baskin and Baskin, Reference Baskin and Baskin2014b; Silva et al., Reference Silva, Ribeiro, Mercadante-Simões, Nunes and Lopes2014; Chandra et al., Reference Chandra, Salin and Puthur2015; Rodrigues-Junior et al., Reference Rodrigues-Junior, Oliveira, de Souza and Riberio2016; Rajapakshe et al., Reference Rajapakshe, Jayasuriya, Rajapakse and Peramunugama2017; Dias et al., Reference Dias, Ribeiro, Lopes, Munné-Bosch and Garcia2017, Reference Dias, Ribeiro, Lopes, Melo, Müller and Munné-Bosch2018). In species with adjacent ligular seedling production, the cotyledonary petiole elongates very little and forms a protuberance (germination button) just outside the fruit (Moura et al., Reference Moura, Ribeiro, Mazzottini-dos-Santos, Mercadante-Simőes and Nunes2019). Root and shoot do not emerge until after the plumular–radicular axis of the small, differentiated embryo is carried outside the diaspore (seed enclosed by endocarp) by elongation of the cotyledonary petiole (tube), within which it is located (Tomlinson, Reference Tomlinson1960; Henderson, Reference Henderson2006). The cotyledonary blade remains inside the seed following germination and root and shoot emergence from the cotyledonary tube, and it acts as a haustorium and grows to fill the cavity created by breakdown of the massive endosperm (Yampolsky, Reference Yampolsky1922; Anonymous, 1955; Hussey, Reference Hussey1958; DeMason, Reference DeMason1984, Reference DeMason1985; Alang et al., Reference Alang, Moir and Jones1988; Hartley, Reference Hartley1988; Sugimuma and Murakami, Reference Sugimuma and Murakami1990; Panza et al., Reference Panza, Lainez and Maldonado2004; Oliveira et al., Reference Oliveira, Lopes, Ribeiro, Mercandante-Simões, Oliveira and Silvério2013; Chandra et al., Reference Chandra, Salin and Puthur2015; Rajapakshe et al., Reference Rajapakshe, Jayasuriya, Rajapakse and Peramunugama2017; Dias et al., Reference Dias, Ribeiro, Lopes, Melo, Müller and Munné-Bosch2018).
Storage behaviour of palm seeds can be orthodox or non-orthodox (intermediate or recalcitrant) (Baskin and Baskin, Reference Baskin and Baskin2014b and references cited therein; Subbiah et al., Reference Subbiah, Ramdhani, Pammenter, Macdonald and Sershen2019; Mattana et al., Reference Mattana, Peguero, Sacco, Agramonte, Castillo, Jiménez, Clase, Pritchard, Gómez-Barreiro, Castillo-Lorenzo, Encarnación, Way, Garcia and Ulian2020; Jaganathan, Reference Jaganathan2021), and the intact diaspores of most palms take a long time to germinate (Orozco-Segovia et al., Reference Orozco-Segovia, Batis, Rojas-Arechiga and Mendoza2003). They have both a morphological, that is, embryo not fully grown (underdeveloped) at seed maturity, and a physiological, that is, require a period of afterripening or warm stratification to germinate, component of dormancy. Thus, the diaspores have some kind of MPD (Perez et al., Reference Perez, Criley and Baskin2008; Baskin and Baskin, Reference Baskin and Baskin2014b; Rajapakshe et al., Reference Rajapakshe, Jayasuriya, Rajapakse and Peramunugama2017; Jaganathan, Reference Jaganathan2020, Reference Jaganathan2021; Jaganathan et al., Reference Jaganathan, Bayarkhuu, He, Liu, Li and Han2021). For palms with the remote tubular and remote ligular types of seedling formation (see Baskin and Baskin, Reference Baskin and Baskin2014b), we suggest the formula ${\rm C}_{x{\rm b}}{\rm B}_{{\rm {b}^{\prime}}}^{( {\rm rs}{) }^{\prime}} $. Cx b indicates non-deep (C1b), intermediate (C2b) or deep (C3b) physiological dormancy that is broken by warm temperatures (subscript b). For
${\rm B}_{{\rm {b}^{\prime}}}^{( {\rm rs}{) }^{\prime}} $, B indicates an underdeveloped embryo (i.e. growth of haustorial cotyledon after germination), subscript b′ growth of the embryo (i.e. expansion of the haustorium) at warm temperatures inside the seed following emergence of the cotyledonary petiole from the diaspore (prime of b′) and superscripts (rs)′ root (r) and shoot (s) production from the cotyledonary petiole after its emergence from the diaspore and elongation away from the plant [the prime of (rs)′], that is, in palms with remote tubular and remote ligular types of seedling production (see Baskin and Baskin, Reference Baskin and Baskin2014b). The formula would be the same for palms with adjacent ligular type of seedling formation, except for the absence of the prime on the superscript on (rs)′, that is,
${\rm C}_{x{\rm b}}{\rm B}_{{\rm {b}^{\prime}}}^{( {\rm rs}) } $.
However, the diaspores of some palms germinate in a short period of time, for example, <30 d (Orozco-Segovia et al., Reference Orozco-Segovia, Batis, Rojas-Arechiga and Mendoza2003), indicating the absence of physiological dormancy and thus that the diaspores have MD. In which case, the dormancy formula may be written as ${\rm B}_{{\rm {b}^{\prime}}}^{( {\rm rs}{) }^{\prime}} $ for palms with remote tubular and remote ligular and as
${\rm B}_{{\rm {b}^{\prime}}}^{( {\rm rs}) } $ for those with adjacent ligular seedling formation. Thus, for palms, we suggest a new subclass of morphological dormancy, that is, Subclass 6 simple specialized E, and a new subclass of morphophysiological dormancy, that is, Subclass 7: simple specialized F (Table 1).
Based on drawings of young seedlings of species in many monocot families (Arber, Reference Arber1925; Boyd, Reference Boyd1932; Tillich, Reference Tillich, Rudall, Cribb, Cutler and Humphries1995, Reference Tillich2007), we suggest that the diaspore formulae described above may serve as ‘working models’ for studies on dormancy/germination of diaspores of quite a lot of monocots. For example, the germination morphology of Ensete venticosum (Musaceae) is similar to that of palms. Thus, the scutellum (cotyledon) remains inside the seed, acts as a haustorium and grows to fill the cavity created by digestion of the endosperm (Karlsson et al., Reference Karlsson, Tamado, Dalbato and Mikias2013) after the cotyledonary petiole with the plumular–radicular axis of the embryo inside it emerges through the opercular opening (Bezunch, Reference Bezunch1971). Furthermore, the seed is physiologically dormant as indicated by the mean time of 8.5–13 weeks to reach 50% and 90% of final germination, respectively (Karlsson et al., Reference Karlsson, Tamado, Dalbato and Mikias2013). The general formula for MPD in palm diaspores would seem to be appropriate for those of E. ventricosum.
Eighteen families of angiosperms and no gymnosperms are known to contain species whose diaspores have a water-impermeable seed (or fruit) coat (physical dormancy, PY): 17 in the eudicots and one in the monocots. Canna, the only genus in the Cannaceae, is the only monocot genus with diaspores known to have a water-impermeable seed coat (Baskin and Baskin, Reference Baskin and Baskin2014a). Furthermore, unlike many genera in the 18 families with PY/(PY + PD), in which the embryo fills most of the volume of the seed, seeds of Canna species contain a substantial amount of storage material [perisperm (Humphrey, Reference Humphrey1896)], and thus, the embryo does not fill most of the volume of the seed (e.g. Benedict et al., Reference Benedict, Smith, Specht, Collinson, Leong-Šorničková, Parkinson and Marone2016).
According to Baskin and Baskin (Reference Baskin and Baskin2004, Reference Baskin and Baskin2014a), no diaspores with PY have an underdeveloped embryo, and this is true in that in none of the 18 families with PY/PY + PD does the embryo grow prior to emergence of root or cotyledonary petiole (germination). However, like for the palms discussed above, the haustorium of the Canna embryo grows considerably following emergence of the cotyledonary petiole (Gatin, Reference Gatin1908). Thus, considering that in addition to PY Canna seeds also have an underdeveloped embryo, they have a kind of combinational dormancy, that is, A [exogenous (physical)] × B [endogenous (morphological)]. For this combined (i.e. exogenous × endogenous, sensu Nikolaeva, Reference Nikolaeva1967[1969]) category, we suggest that the formula ${\rm A}_{{\rm ph}}-{\rm B}_{{\rm {b}^{\prime}}}^{( {\rm rs}) } $ (terms defined above) and that it be at the rank of subclass. The second part of the formula is the same as that of palm diaspores with morphological dormancy and adjacent ligular seedling production. The only other category of combinational dormancy in our revised classification scheme is A [exogenous (physical)] × C [endogenous (physiological)], which we suggest also should be ranked as a subclass. Thus, combinational dormancy consists of two subclasses: Subclasse A–B and Subclasse A–C.
As we learn more about the morphology and physiology of the germination process of monocot seeds, undoubtedly it will be necessary to add even more subclasses, levels and/or types to the Nikolaeva–Baskin seed dormancy classification system. In this review, we have touched on the diversity of the dormancy/germination process in monocots in discussing the germination morphology of such taxa as Arecaceae, Canna, Convallaria, Trillium and Zosteraceae. However, certainly, our classification system does not cover all of the considerable variation in the whole-seed morphology and physiology of the dormancy/germination process that exists among the monocots. Tillich (Reference Tillich2007) stated that, ‘The evolution of the monocotyledonous embryo/seedling required a profound reorganization of the plant body’. Thus, it should not be surprising that the morphoanatomy of the seed dormancy/germination processes in monocots differs considerably from that in other seed plants, with perhaps Nymphaeales, including Trithuria [previously classified as a monocot (Saarela et al., Reference Saarela, Rai, Doyle, Endress, Mathews, Marchant, Briggs and Graham2007)], in the ANA grade basal angiosperms being an exception (Tillich et al., Reference Tillich, Tuckett and Facher2004).
Types of PD in seeds with (PY + PD)
Nikolaeva et al. [Reference Nikolaeva, Razumova, Gladkova and Danilova1985, see Rosbakh et al. (Reference Rosbakh, Baskin and Baskin2020) for English translation] listed species with each of the three levels of PD (their B1, B2 and B3) in combination with PY (their AΦ). Thus, species of Astragalus, Geranium, Koelreuteria, Lathyrus and Trifolium were indicated to have PY + non-deep PD (AΦ + B1); those of Ceanothus, Cercis and Cladrastis PY + intermediate PD (AΦ + B2) and those of Cotinus, Rhus, Tilia and Toxicodendron PY + deep PD (AΦ + B3). However, we are not aware of any studies in which intermediate or deep PD have been documented for seeds that also have PY. Thus, it appears that, to date, the level of PD in all seeds in Subclass [A–C] of combinational dormancy is non-deep (C1a or C1b). Furthermore, it seems possible that, in some seeds in this subclass, the physiological component may be at the intermediate level (C2b or C2a) and, in others, at the deep level (C3b or C3a). However, we are somewhat skeptical that seeds of a species with a water-impermeable seed or fruit coat also could have an embryo with deep PD. Thus, we suggest the scheme shown in Table 1 for the levels and types of dormancy in the combined class. We know that level 1 with two types of PD, that is, seeds requiring either warm (C1b) or cold (C1a) pretreatment to break PD, depending on the species, exists. However, levels 2 and 3 are yet to be documented convincingly. We suggest that the primarily western North American genus Ceanothus (Rhamnaceae) and the North American–Mediterranean–Asian disjunct genus Cercis (Fabaceae), both of which contain several species whose seeds have been shown to have (PY + PD) (Baskin and Baskin, Reference Baskin and Baskin2014a), are good candidates for study taxa on levels of PD in seeds with combinational dormancy.
Are seeds of the ‘morphologically misfit’ riverweed family Podostemaceae dormant or nondormant?
The Podostemaceae have been labelled a ‘morphological misfit’ family because the vegetative growth/architectural body plan does not fit the classical root–shoot (CR-S) model typical of angiosperms (see Rutishauser, Reference Rutishauser2016); the basic vegetative body plan is thalloid (Mohan Ram and Sehgal, Reference Mohan Ram and Sehgal1992, Reference Mohan Ram, Sehgal and Rangaswamy2001; Sehgal et al., Reference Sehgal, Mohan Ram and Bhatt1993; Uniyal and Mohan Ram, Reference Uniyal and Mohan Ram1996, Reference Uniyal and Mohan Ram2001; Rutishauser, Reference Rutishauser2016). The mature embryo ranges from that of a typical eudicot in the most phylogenetically primitive taxa to that of a embryo with two well-developed cotyledons [one cotyledon in Hydrodiscus koyamase (Koi and Kato, Reference Koi and Kato2010)] and hypocotyl, that is, no embryo root or shoot meristem in the most phylogenetically advanced taxa (Mohan Ram and Sehgal, Reference Mohan Ram, Sehgal and Rangaswamy2001; Uniyal and Mohan Ram, Reference Uniyal and Mohan Ram2001; Katayama et al., Reference Katayama, Kato, Nishiuchi and Yamada2011; Kita and Kato, Reference Kita and Kato2005; Koi and Kato, Reference Koi and Kato2010; Koi et al., Reference Koi, Werukamkul, Ampornpan and Kato2012). This family of riverweeds contains about 50 genera and 270 species, and its habitat typically is swift-flowing streams where it attaches to rocks and other solid objects (Philbrick and Novelo, Reference Philbrick and Novelo2004). The seeds germinate only when they are submerged under water (Philbrick, Reference Philbrick1984; Philbrick and Novelo, Reference Philbrick and Novelo1997). Mohan Ram and Sehgal (Reference Uniyal and Mohan Ram2001) reported that seeds of Indotristicha ramossima are dispersed between November and February in India and ‘… lie on rocks until the next monsoon and germinate with the first showers’. Does this mean that the seeds came out of dormancy during the dry period or that they were nondormant at dispersal and remained in enforced dormancy (quiescent) until onset of the monsoon?
A problem often encountered in attempting to determine from the literature if fresh seeds of a taxon are dormant or nondormant is that they are stored dry (a dormancy-breaking treatment for seeds of many species) before being tested for germination. In many such studies, if seeds germinate following storage, they are said to be nondormant. A case in point is the Podostemaceae in which seeds of various species have been reported to be nondormant (or it could be inferred from the data on germination that the seeds were nondormant) (Philbrick, Reference Philbrick1984; Vidyashankari and Mohan Ram, Reference Vidyashankari and Mohan Ram1987; Sehgal et al., Reference Sehgal, Mohan Ram and Bhatt1993; Philbrick and Novelo, Reference Philbrick and Novelo1994, Reference Philbrick and Novelo1997; Uniyal and Mohan Ram, Reference Uniyal and Mohan Ram1996, Reference Uniyal and Mohan Ram2001; Kita and Kato, Reference Kita and Kato2005; Reyes-Ortega et al., Reference Reyes-Ortega, Sánchez-Coronado and Orozco-Segovia2009; Koi and Kato, Reference Koi and Kato2010; Luna et al., Reference Luna, Guzmán, Núñez-Farfán, Philbrick, Collazo-Ortega and Márquez-Guzmán2012; Koi et al., Reference Koi, Werukamkul, Ampornpan and Kato2012; Castillo et al., Reference Castillo, Marquez-Guzmán and Collazo-Ortega2013; Flores-Enríquez et al., Reference Flores-Enríquez, Castillo and Collazo-Ortega2019). However, a possible problem with some of the studies reporting that the seeds were nondormant is that the seeds were stored for 40 d to >1.5 years (maximum 9 years) before they were tested for germination (e.g. Philbrick, Reference Philbrick1984; Philbrick and Novelo, Reference Philbrick and Novelo1994; Luna et al., Reference Luna, Guzmán, Núñez-Farfán, Philbrick, Collazo-Ortega and Márquez-Guzmán2012; Castillo et al., Reference Castillo, Marquez-Guzmán and Collazo-Ortega2013; Flores-Enríquez et al., Reference Flores-Enríquez, Castillo and Collazo-Ortega2019), thus raising the possibility that the fresh seeds were dormant and afterripened during storage. Interestingly, Philbrick and Novelo (Reference Philbrick and Novelo1994) reported high germination percentages for seeds of five species of Mexican Podostemaceae stored for 6–18 months, but they state in their discussion that ‘… preliminary experiments indicate that seed of the five species studied herein will germinate as soon as they are mature, as long as they are wet and in the light’. Although there is a caveat concerning some of the studies with regard to procedures for determining if fresh seeds had primary dormancy, that is, seeds tested only after a period of storage during which they possibly could have afterripened, we suggest that fresh seeds of Podostemaceae are nondormant, as reported by Philbrick and Novelo (Reference Philbrick and Novelo1994) and Reyes-Ortega et al. (Reference Reyes-Ortega, Sánchez-Coronado and Orozco-Segovia2009).
Closing remarks
Crocker (Reference Crocker1916) stated that, ‘I believe that failure to grasp the variety of methods and the counter attempts to explain all by one or at most two methods is the main source of controversy, error and confusion that have prevailed in this field [seed dormancy]’. In our opinion, for the most part, this problem Crocker recognized in the early part of the twentieth century still exists in the early part of the twenty-first century. In particular, most scientists working with seeds tend to ignore (or are unaware of) the great diversity in the mechanics of dormancy. We suggest that the ‘chaotic situation’, with regard to seed dormancy that Crocker referred to in 1916, can be cleared up by use of a detailed word-formula system for seed dormancy classification, initiated by Nikolaeva more than 50 years ago, that is adhered to by the international community of seed scientists.
Certainly, a well-founded, universally acceptable whole-seed dormancy classification system is essential for meaningful studies on the phylogenetics/evolution and biogeography of seed dormancy (Baskin and Baskin, Reference Baskin and Baskin2014a; Willis et al., Reference Willis, Baskin, Baskin, Auld, Venable, Cavender-Bares, Donohue and Rubio de Casas2014). The following example illustrates the utility of the detailed formula system in describing the evolution of one level of MPD from another one in Osmorhiza (Apiaceae). Deep complex MPD is plesiomorphic in seeds (mericarps) of Osmorhiza, and non-deep simple MPD is the derived condition (Wen et al., Reference Wen, Lowry, Walck and Yoo2002 and references therein), that is, C3aBa − C3a → C1b1aBa − C3a. Thus, following migration of the genus from eastern Asia, where it originated, across the Bering Land Bridge and on to eastern North America, the requirement for a long period of cold stratification (C3a) for the underdeveloped embryo (Ba) to become fully developed changed to a period of warm followed by a period of cold stratification (C1b1a). The requirement for a cold period for embryo growth (Ba) and the breaking of PD in seeds with a fully developed embryo (−C3a) in the Asian ancestor has been retained in the two eastern North American species with the derived seed dormancy condition. In contrast, the kind of dormancy in the western North American species remained the same as that in the ancestral Asian species, that is, C3aBa − C3a → C3aBa − C3a. It would be interesting to work out the dormancy formula for the Osmorhiza taxa that migrated to South America from North America.