Introduction
Seed traits determine to a large extent the time and place of establishment of a plant and as such the expression of post-germination traits (Donohue et al., Reference Donohue, Rubio de Casas, Burghardt, Kovach and Willis2010; de Casas et al., Reference De Casas, Donohue, Venable and Cheptou2015). Among different seed traits, dormancy is an important biological trait that synchronizes the germination event with the favourable time for seedling establishment (Leverett et al., Reference Leverett, Schieder and Donohue2018), while seed desiccation tolerance/sensitivity is important in determining the ability of the species to produce a soil seed bank (Tweddle et al., Reference Tweddle, Dickie, Baskin and Baskin2003).
Seeds that do not germinate under otherwise favourable environmental conditions are termed as dormant, and those that germinate readily in a wide range of environmental conditions are termed as non-dormant seeds. As seed dormancy is an important mechanism to synchronize seed germination with the favourable time for seedling development (Wagmann et al., Reference Wagmann, Hautekèete, Piquot, Meunier, Schmitt and Van Dijk2012; Baskin and Baskin, Reference Baskin and Baskin2005, Reference Baskin and Baskin2014), species producing dormant seeds are very common in seasonal ecosystems. On the other hand, species producing seeds with non-dormancy (ND) are more frequent in more aseasonal ecosystems (Pammenter and Berjak, Reference Pammenter and Berjak1999, Reference Pammenter and Berjak2000). Seeds can be classified as recalcitrant, intermediate or orthodox based on the sensitivity of seeds to desiccation and on storability (Hong et al., Reference Hong, Linington and Ellis1996). Further, recalcitrant storage behaviour has been identified as an adaptation to germinate quickly (Pammenter and Berjak, Reference Pammenter and Berjak1999; Daws et al., Reference Daws, Garwood and Pritchard2005) under environmental conditions favourable for seed pathogens and predators (Pammenter and Berjak, Reference Pammenter and Berjak2000; Daws et al., Reference Daws, Garwood and Pritchard2005; Baskin, and Baskin, Reference Baskin and Baskin2014).
Studying seed dormancy and seed desiccation sensitivity traits of taxonomically closely related species in different ecosystems enable understanding the relative importance of different kinds of seed dormancy (Baskin and Baskin, Reference Baskin and Baskin2014) and desiccation (Subbiah et al., Reference Subbiah, Woodenberg, Varghese and Pammenter2019) for these ecosystems. Such a study also facilitates the investigation of evolutionary origin and relationship of the five dormancy classes and ND (Baskin and Baskin, Reference Baskin and Baskin2014). There are few studies on tropical species conducted on seed germination of taxonomically related species (Jayasuriya et al., Reference Jayasuriya, Wijetunga, Baskin and Baskin2013; Xu et al., Reference Xu, Li, Zhang, Liu and Du2014). Strychnos is an ecologically and medicinally important genus consisting of species distributed in different ecosystems of Sri Lanka (Jayaweera, Reference Jayaweera1981), providing a venue to study the radiation of seed germination traits in different ecosystems.
Strychnos nux-vomica and Strychnos potatorum are two important woody medicinal plants, of which bark, leaves, roots, seeds and fruits have been used in traditional medicinal preparations for a long period of time in many Asian countries (Behpour et al., Reference Behpour, Ghoreishi, Khayatkashani and Motaghedifard2012; Yadav et al., Reference Yadav, Kadam, Patel and Patil2014; Patel et al., Reference Patel, Laloo, Singh, Gadewar and Patel2017). Furthermore, S. nux-vomica and S. potatorum are ecologically important species in the dry mixed evergreen forests, while Strychnos benthamii grows in lowland evergreen forests of Sri Lanka (Jayaweera, Reference Jayaweera1981). Many Sri Lankan lowland dry forests that have poor natural regeneration (Perera, Reference Perera2001) as well as lowland evergreen forests (Gunatilleke et al., Reference Gunatilleke, Pethiyagoda and Gunatilleke2008; Perera, Reference Perera, Weerakoon and Wijesundara2012) are in a degraded state. To reinstate the biodiversity of these forests and to retain continuity of the ecosystem services, these forests have to be rehabilitated. However, lack of information on the propagation of important native species such as S. nux-vomica and S. potatorum is a drawback in the rehabilitation process of these forests. Thus, information gathered in this research would also facilitate the rehabilitation of these forests.
Seeds of S. nux-vomica and S. potatorum have been reported to have physiological dormancy (PD) by many researchers (Elliott et al., Reference Elliott, Anbusarnsunthorn, Kopachon, Maxwell, Blakesley and Garwood1996; Pearson, Reference Pearson2005; Sivakumar et al; Reference Sivakumar, Anandalakshmi, Warrier, Tigabu, Odén, Vijayachandran, Geetha and Singh2006). In contrast, Warrier et al. (Reference Warrier, Anandalakshmi, Sivakumar and Singh2017) has reported that seeds of S. potatorum have morphophysiological dormancy (MPD). However, there is no sufficient information provided on either embryo morphology or embryo development that is essential to make conclusions on the morphological component of the dormancy (Baskin and Baskin, Reference Baskin and Baskin2007). Martin (Reference Martin1946) has identified species in Loganiaceae are producing dwarf seeds. Nevertheless, not all the species of Loganiaceae produce dwarf seeds (Baskin and Baskin, Reference Baskin and Baskin2014). Moreover, some Loganiaceae species were reported to have seeds with no dormancy (Anthocleista nobilis (Baskin and Baskin, Reference Baskin and Baskin2014), A. zambesiaca (Msanga, Reference Msanga1998)), while other species are reported to have seeds with PD (Logania buxifolia and L. vaginalis (Kullmann, Reference Kullmann1981) and Genlostoma rupestre (Burrows, Reference Burrows1997, Reference Burrows1999)). Thus, according to the available information, it could be suggested that seeds of the Strynchnos species have ND, PD or MPD.
Thus, the main aim of this research was to clarify the ambiguities of seed dormancy and storage behaviour of this important and understudied genus, Strychnos, using the three above-mentioned species that inhabit seasonal and aseasonal habitats in Sri Lanka. We hypothesized that Strychnos spp. growing in tropical seasonal habitats in Sri Lanka produce seeds with dormancy and desiccation-tolerant behaviour, while those in aseasonal habitats have non-dormant desiccation-sensitive seeds. Thus, we conducted experiments to determine the seed dormancy and storage behaviour of three Strychnos species inhabiting tropical dry mixed evergreen and tropical wet evergreen forests in Sri Lanka.
Materials and methods
Study organisms
The three study species, S. nux-vomica L., S. potatorum L.f. and S. benthamii C.B.Clarke (Loganiaceae), are ecologically and medicinally important species occurring in Sri Lanka (Philcox, Reference Philcox, Dassanayake, Fosberg and Clayton1996; Senaratne, Reference Senaratne2001). S. nux-vomica and S. potatorum are tree species listed as vulnerable in the National Red List of Sri Lanka, while S. benthamii is a small tree or shrub which is an endemic, near-threatened species to Sri Lanka (MOE, 2012). S. nux-vomica is distributed in India, Sri Lanka, China and Southeast Asia, while S. potatorum occurs in India, Sri Lanka, Myanmar and tropical Africa (Jayaweera, Reference Jayaweera1981). S. nux-vomica and S. potatorum are mainly confined to the lowland dry zone of Sri Lanka, while S. benthamii occurs in tropical montane forests to tropical lowland rainforests (Jayaweera, Reference Jayaweera1981; Philcox, Reference Philcox, Dassanayake, Fosberg and Clayton1996).
Seed collection
Ripened yellow and dark purple-coloured fruits of S. nux-vomica and S. potatorum, respectively, and purple-coloured fruits of S. benthamii were collected from at least five individual trees in their respective locations during their peak fruiting season (Table 1). Fruits were stored in polythene bags and brought to the Seed Biology laboratory, University of Peradeniya, where the experiments were conducted within 1–3 d after the collection. Fresh fruits were soaked in water for a few hours to remove the pulp. Seeds were sorted to discard visibly deficient seeds and air-dried.
Table 1. Information about seed collection sites and collection period

Standard germination test
This experiment was conducted to determine if seeds are dormant or not. Four samples of at least six seeds each from each species were incubated on moistened tissue (Flora paper serviette, four times folded thickness) papers in 9 cm diameter Petri dishes at 25°C under light/dark conditions (12 h/12 h) in incubators. S. nux-vomica and S. potatorum seed germination tests were also performed at 32°C in a 12 h photoperiod. Germination tests for S. benthamii were performed only at 25°C under light/dark conditions (12 h/12 h) due to the scarcity of seeds. Seeds were checked at weekly intervals until all the seeds germinated. Radicle emergence was the criterion for germination.
Seed storage behaviour
The seed storage behaviour of the three study species was identified using the 100 seed method as proposed by Pritchard et al. (Reference Pritchard, Wood, Hodges and Vautier2004). Moisture content (MC) of ten seeds was measured individually with the oven-dry method. Initial germination was determined at a temperature (25°C) considered optimal for the three species. To evaluate the effect of desiccation on germination, 32 seeds were kept under desiccated conditions maintained by an equal weight (to the mass of 32 seeds) of silica gel inside a zip-lock bag at 25°C. At 3-d intervals, silica gel was replaced and seeds were weighed until they reached a constant weight. For the moist-control, another sample of 32 seeds was stored at 25°C at 100% RH in a sealed Petri dish. RH in the Petri dish was maintained using cotton wool moistened with water. The Petri dish was opened to aerate the seeds in 3-d intervals. After the storage period, six seeds each were used to determine MC and then two samples from desiccated and moist-stored control samples each were incubated at 25°C. Germination was checked every day until all the seeds germinated. Seed germination percentage was plotted against the incubation time for the three test conditions for three Strychnos spp. as described above. Standard curves were fitted to distinguish between desiccation-tolerant and desiccation-sensitive behaviour of seeds.
Dormancy class of the study species
A series of experiments were performed to determine the class of seed dormancy of the three Strychnos species.
Imbibition of seeds
This experiment was conducted to determine if the seed coats of Strychnos species were impermeable to water. Ten fresh seeds of each species were weighed individually using a digital chemical balance to the nearest 0.001 g. Seeds were placed on tissue papers moistened with distilled water in Petri dishes and retrieved after 1 and 6 h and then after 24 h intervals for 10 d. Retrieved seeds were surface blotted, reweighed individually and returned to the Petri dishes.
Seed coat anatomy
Anatomical studies were conducted to confirm the presence/absence of a water-impermeable seed coat. Water-impermeable seed coats contain a palisade layer with a light line. Transverse hand sections (~30 μm) of the seed coat away from the hilum were taken and observed under a light microscope. Micrographs were taken with a Nikon D3300 digital camera.
Gibberellic acid treatment
Sixteen samples from S. nux-vomica and S. potatorum containing six seeds each were placed on tissue paper moistened with 100 or 500 ppm gibberellic acid (GA3) solutions. Four samples of seeds placed on 100 ppm GA3 from each species were incubated at 25°C and the other four at 32°C. Four samples of seeds of each species placed on 500 ppm GA3 were also incubated at 25 and 32°C. Four samples of six S. benthamii seeds were treated with 100 and 500 ppm GA3 and incubated at 25°C. This test was initiated simultaneously with the standard germination tests described above, which was considered as the control test.
Embryo type, embryo to seed length ratio (E:S ratio)
The embryo characteristics were examined visually to determine if freshly matured seeds of the three tested species are dispersed with underdeveloped or fully developed embryos. Embryo types in seeds of the three study species were categorized according to Baskin and Baskin (Reference Baskin and Baskin2007). Forty-eight seeds each from each species were incubated on tissue papers moistened with distilled water at 25°C. Six seeds were retrieved in every 2-week intervals until the shoot begin to emerge in all the seeds. Seeds were cut into two equal halves using a razor blade without damaging the embryo. Seed length and embryo length were measured using a ruler to the nearest 0.1 mm. The embryo length was taken excluding the length of radicle protruded outside the seed coat in seeds measured after radicle emergence. E:S ratio was calculated using the above measurements. Then, embryo development was calculated using the following formula (Athugala et al., Reference Athugala, Jayasuriya, Gunaratne and Baskin2016):

Epicotyl dormancy
Thirty-six samples (12 samples for each of the three species) containing six radicle emerged seeds of S. nux-vomica, S. potatorum and S. benthamii were incubated on tissue papers moistened with distilled water in 1000 ml beakers. Four samples from each species kept in a plant house while the other eight samples each from S. nux-vomica and S. potatorum were incubated at 25 and 32°C, while the four samples of S. benthamii were incubated only at 25°C. Seed samples were observed for seed coat rupture and shoot emergence of individual seeds. Time taken for seed coat rupture and shoot emergence from radicle emergence was determined for each seed.
Data analysis
All the experiments were performed in a completely randomized design. One-way analysis of variance was used in data analysis. Data were arcsine transformed before analyzing them in parametric methods. Germination data were analyzed with Minitab 17.0 statistical software. A logistic four parameters, the sigmoidal curve was fitted for germination percentages against incubation time period and graphs were obtained by the Sigma plot 10.0 statistical software. Time taken to reach 50% germination (T 50) was determined from the fitted curve.
Results
Standard germination tests
Seeds of S. nux-vomica and S. potatorum showed a considerable delay in germination as they took >30 d in all the tested temperatures to initiate germination. After 60 d, S. nux-vomica germinated to ~58%, while very few seeds of S. potatorum germinated during this time. In contrast, S. benthamii showed the first germination within only 11 d. T 50 of S. nux-vomica and S. potatorum at 32 and 25°C was >30 d, while that of S. benthamii at 25°C was 16 d (Table 2).
Table 2. Germination parameters (mean ± SD) calculated for fresh non-treated Strychnos nux-vomica, Strychnos potatorum and Strychnos benthamii seeds germinated at 25 and 32°C in light/dark conditions.

a Days to acquire the highest germination percentage indicates the number of days when at least one sample reached 100% of germination.
b Final cumulative germination percentage indicates the germination percentage reached at the end of the experiment (at the respective time, in days, shown in the parenthesis).
Seed storage behaviour
Seed MC of the three study species at dispersal ranged from 31.6% for S. potatorum to 45.9% for S. benthamii (Table 3). Seeds of non-treated S. nux-vomica, S. potatorum and S. benthamii germinated >90% within 129, 119 and 13 d, respectively. Seeds of both S. nux-vomica and S. potatorum germinated >80% even after desiccation storage. The non-germinated seeds rotted and died during the incubation period. When seeds of S. potatorum and S. nux-vomica reached a constant weight during desiccation, they had a MC of 2.4 to 2.9%, respectively (Table 3). None of the S. benthamii seeds germinated after desiccation storage to a MC of 5.1 ± 3.0%, and they rotted soon after the incubation began. Moist-stored S. nux-vomica, S. potatorum and S. benthamii seeds germinated >90% after 46, 98 and 13 d, respectively (Fig. 1A, B). The MC of seeds after moist storage was significantly lower than the initial moisture contents of each species (Table 3).

Fig. 1. Germination progression curves using the 100 seed method for fresh, moist-stored control and desiccated seeds of (A) Strychnos nux-vomica, (B) S. potatorum and (C) S. benthamii. Logistic 4 parameter sigmoidal curves were fitted to determine the germination pattern ($R_{S. nux-vomica}^2 = 0.94$,
$R_{S. potatorum}^2 = 0.98$,
$R_{S. benthamii}^2 = 0.97$).
Table 3. Moisture contents (mean ± SD) of fresh, moist-stored and desiccated seed samples of Strychnos nux -vomica, Strychnos potatorum and Strychnos benthamii

Dormancy class of the study species
Imbibition of seeds
All the seeds gradually increased in mass during imbibition. After 10 d, the mass increase of seeds of S. nux-vomica, S. potatorum and S. benthamii incubated on tissue papers moistened with distilled water at 25°C was 59.3 ± 7.0, 80.9 ± 7.8 and 69.1 ± 11.1%, respectively.
Seed coat anatomy
No palisade layer was observed in the seed coats of S. nux-vomica, S. potatorum (Data not shown). Trichomes developed from the uppermost layer of the seed coat were observed in both species. The uppermost layer was a single-layered columnar-shaped epidermis. In S. nux-vomica seeds, the epidermal layer seems to be disrupted. Below the epidermal layer, several layers of thick-walled sclerenchyma cells were present in seed coats of S. nux-vomica and S. potatorum.
Gibberellic acid treatment
A significantly higher germination percentage was observed when S. nux-vomica and S. potatorum seeds were incubated at 32°C on GA3 moistened tissue papers as compared to the non-treated controls [F = 23.64, P < 0.001 for S. nux-vomica, F = 45.18, P < 0.001 for S. potatorum (Fig. 2) ]. However, S. benthamii seeds incubated on tissue papers moistened with GA3 solutions germinated to a similar percentage as the non-treated seeds incubated on distilled water.

Fig. 2. Cumulative germination percentage of Strychnos nux-vomica, Strychnos potatorum and Strychnos benthamii seeds incubated on tissue paper moistened with 100 and 500 ppm gibberellic acid (GA3) and with distilled water (control) at different temperatures in light/dark (12 h/12 h) conditions after 60 d. Error bars are + SD. Different uppercase letters indicate significant differences between species within the same treatment (F = 23.64, P < 0.001 for S. nux-vomica, F = 45.18, P < 0.001 for S. potatorum, F = 1.09 P = 0.300 for S. benthamii).
Embryo type and Embryo to Seed length ratio (E: S ratio)
At dispersal, seeds of S. nux-vomica and S. potatorum possessed a spatulate underdeveloped embryo with an E:S ratio 0.34 and 0.29, respectively (Table 4). S. benthamii seeds also had a spatulate underdeveloped embryo with an E:S ratio equal to 0.51 ± 0.08. Even soon after radicle emergence, E:S ratio of both S. nux-vomica and S. potatorum remained below 0.5, while that of S. benthamii reached 0.64 ± 0.04.
Table 4. Embryo to seed length ratio (E:S ratio) (mean ± SD and percentages) of Strychnos nux-vomica, Strychnos potatorum and Strychnos benthamii of each stage of fresh seeds and, seeds soon after radicle emergence and soon before the shoot emergence

Epicotyl dormancy
Radicle-emerged seeds of the three study species required more than 30 d for the shoot to emerge at 25 or 32°C. On average, S. nux-vomica seeds took 76 ± 8 and 69 ± 20 d for shoot emergence to occur at 25 and 32°C, respectively. Moreover, S. potatorum seeds with emerged radicles kept in the beakers in the laboratory were viable for 71 d (Fig. 3, up to stage B4), after which they became infected with fungi that killed them before shoot emergence occurred at either 25 or 32°C. However, when seeds with emerged radicles were transferred to the plant house, they successfully emerged shoots after 74 ± 14 d. S. benthamii seeds with emerged radicles incubated at 25°C took 45 ± 3 d for shoots to emerge, and they required a similar time period for shoots to emerge when they were kept in the noncontrolled plant house.
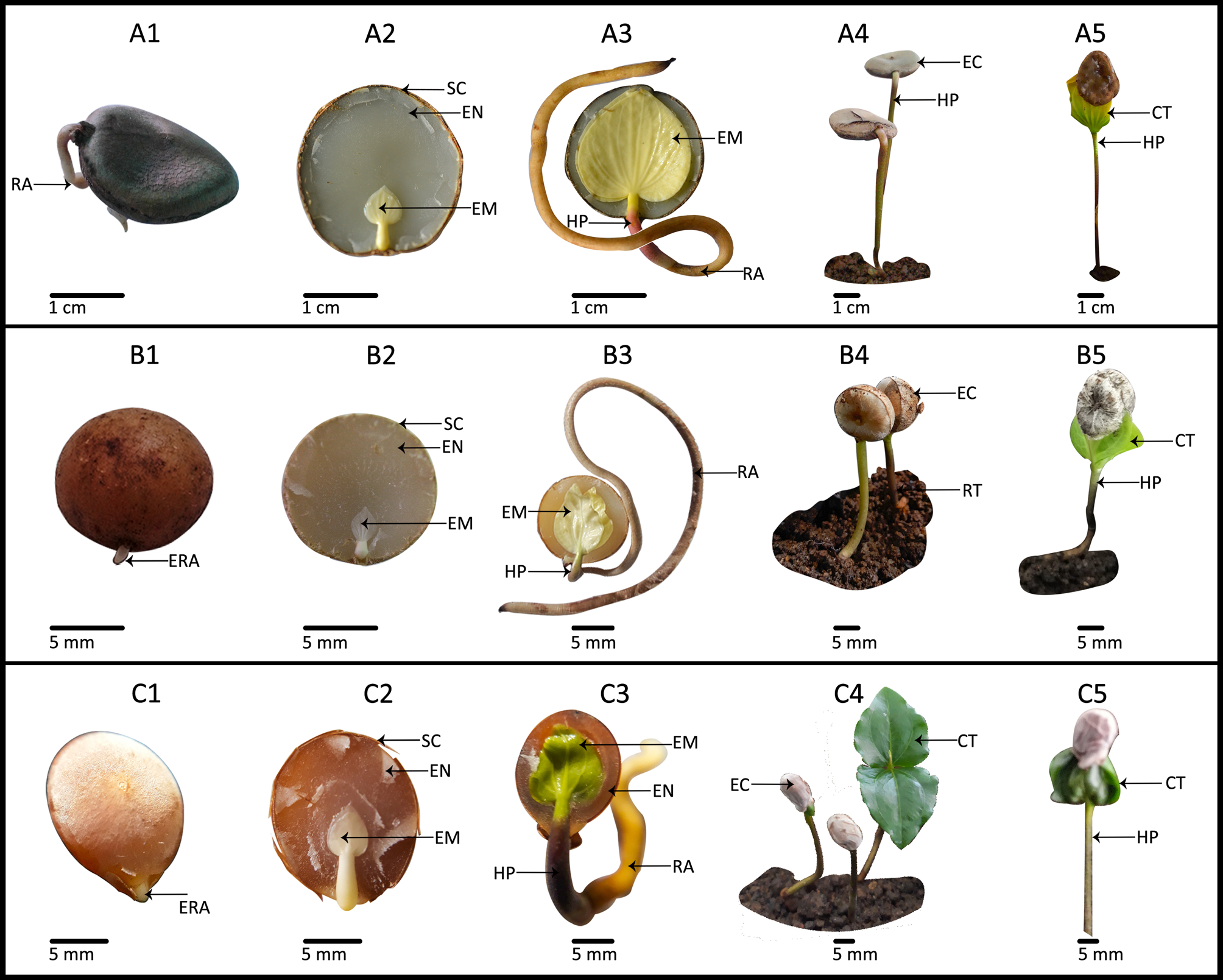
Fig. 3. Developmental stages of (A) Strychnos nux-vomica, (B) Strychnos potatorum and (C) Strychnos benthamii showing radicle emergence (A1, B1 and C1), transverse sections of fresh, mature seeds before radicle emergence showing spatulate underdeveloped embryo (A2, B2 and C2), embryo before shoot emergence (A3, B3 and C3), epicotyl dormant stage (A4, B4 and C4) and shoot emergence of S. nux-vomica, S. potatorum and S. benthamii (A5, B5 and C5). EN, endosperm; EM, embryo; SC, seed coat; HP, hypocotyl; ERA, emerging radicle; RA, radicle; EC, epicotyl dormant stage; CT, cotyledons; RT, root.
Discussion
The major finding of our study was that the three Strychnos spp. have epicotyl morphophysiological dormancy (eMPD), which is the first record of epicotyl dormancy in Loganiaceae. Further, our results indicated that S. nux-vomica and S. potatorum, two species that inhabit dry mixed evergreen forests, which is a relatively seasonal ecosystem, have seeds with orthodox storage behaviour. Moreover, S. benthamii inhabits wet evergreen forests, which is an aseasonal-wet ecosystem and has seeds with recalcitrant storage behaviour. The results of our experiments partially supported our hypothesis.
Moisture contents (MC) of S. nux-vomica, S. potatorum and S. benthamii at dispersal were within the MC range of recalcitrant seeds as well as orthodox seeds as reported by Hong and Ellis (Reference Hong, Ellis, Engels and Toll1996). However, the germination progress curves of S. nux-vomica and S. potatorum seeds resemble those of desiccation-tolerant seeds (see Pritchard et al., Reference Pritchard, Wood, Hodges and Vautier2004 for details of germination progress curves for recalcitrant and orthodox seeds). In contrast, S. benthamii seeds had germination progress curves similar to those of desiccation-sensitive seeds. Thus, we concluded that S. nux-vomica and S. potatorum seeds are desiccation-tolerant and those of S. benthamii are desiccation-sensitive. Lan et al. (Reference Lan, Xia, Wang, Liu, Zhao and Tan2014) and Sivakumar et al. (Reference Sivakumar, Anandalakshmi, Warrier, Tigabu, Odén, Vijayachandran, Geetha and Singh2006) reported that seeds of S. nux-vomica are desiccation-tolerant, while Warrier et al. (Reference Warrier, Anandalakshmi, Sivakumar and Singh2017) reported that seeds of S. potatorum are recalcitrant. These previous conclusions were based on the decrease in germination percentage of desiccated seeds. However, our experiments showed that S. potatorum seeds are desiccation-tolerant. These differences between our conclusion on the seed storage behaviour of S. potatorum and other previous reports could be due to the intra-specific differences since these reports are from two geographical regions in the world or due to procedural differences. However, there is no information available on the seed storage of S. benthamii. Nevertheless, results of our experiments indicated the presence of both desiccation-tolerant and -sensitive traits within the same genus of Strychnos. There are only a few genera that contain species producing seeds with a varying amount of desiccation tolerance (Hong et al., Reference Hong, Linington and Ellis1996). However, there are other reports on seeds of Strychnos spp. having different storage behaviour types (S. cocculoides seeds with intermediate storage behaviour (Msanga, Reference Msanga1998; Mkonda et al., Reference Mkonda, Akinnifesi and Mafongoya2001), while those of S. pseudoquina are desiccation-tolerant (Silva et al., Reference Silva, Sales, Silva, Campos, Branquinho and Silva2012)).
Strychnos nux-vomica and S. potatorum are dry zone species, while S. benthamii is a wet zone species. Desiccation tolerance in seeds of S. nux-vomica and S. potatorum is likely an ecological adaptation to their habitat, since the tropical dry mixed evergreen forest habitats undergo dry spells (Perera, Reference Perera, Weerakoon and Wijesundara2012) that last for about 3–5 months (Jayamaha, Reference Jayamaha1975; Gunda et al., Reference Gunda, Hornberger and Gilligan2016); recalcitrant behaviour may not be viable. Thus, these seeds may have evolved orthodox behaviour and dispersal during the dry season (Daws et al., Reference Daws, Garwood and Pritchard2005; Baskin, and Baskin, Reference Baskin and Baskin2014), which are viable adaptations for unfavourable environmental conditions in the dry zone. Especially, during the fruit dispersal season of S. nux-vomica and S. potatorum, the climatic conditions are dry and as such an orthodox storage behaviour allows seeds to remain viable. A similar conclusion was made by Singh et al. (Reference Singh, Phartyal and Rosbakh2017) on the basis of seed desiccation behaviour of tree species in Indian Himalayas. They concluded that seed desiccation sensitivity depends on the seed shedding and germination time in relation to the monsoonal season. They categorized species as monsoonal synchronized, partially monsoonal synchronized and monsoonal desynchronized based on the occurrence of dispersal and germination within or outside the monsoonal season. They found that 45% of the species producing recalcitrant seeds were from the monsoonal synchronized category, and seeds were dispersed and germinated within the monsoon season, which was the most favourable period for plant regeneration in the seasonal Himalayan ecosystem. However, when the species are desynchronized with the monsoonal season, they observed a very high percentage of orthodox seeds.
Strychnos benthamii seeds took only 11 d for the radicle to emerge, and it can be concluded that there is no PD in the radicle of this species. Thus, S. benthamii seeds should have either ND or morphological dormancy (MD). In contrast, seeds of S. nux-vomica and S. potatorum do show PD at the time of dispersal as none of the non-treated seeds had emerged radicles within 30 d when incubated at 25 or 32°C. The mean length of germination (MLG) of these two species is higher than 30 d, which indicates the presence of seed dormancy. All seeds of the latter two species showed signs of considerable imbibition without scarification of the seed coat, and we can conclude that seeds of the two species have no physical dormancy.
Embryos of seeds of all three study species were spatulate, and the E:S ratio at dispersal maturity was 0.29 for S. potatorum and 0.34 for S. nux-vomica. In contrast, E:S ratio of S. benthamii seeds was ~0.5. However, by the time of radicle emergence, the E:S ratio has increased by 38.2, 34.5 and 25.5% in seeds of S. nux-vomica, S. potatorum and S. benthamii, respectively, indicating that embryos of these three species were underdeveloped. However, seeds of these species contained fully differentiated embryos with distinct embryo axis, cotyledons and leaf primordia, indicating that they are differentiated. Thus, the embryo type of the three species could be identified as an underdeveloped differentiated spatulate embryo according to the modified Martin's (Reference Martin1946) seed classification system (Baskin and Baskin, Reference Baskin and Baskin2007). Furthermore, the seed dormancy of S. nux-vomica and S. potatorum can be classified as MPD, as they have an underdeveloped embryo and their MLG is >30 d. Three levels of PD have been described by Baskin and Baskin (Reference Baskin and Baskin2004, Reference Baskin and Baskin2014) as deep, intermediate and non-deep PD. Non-deep PD could be alleviated by GA3, dry storage and warm stratification. Radicle emergence of S. nux-vomica and S. potatorum was enhanced by GA3 treatment, dry storage (conducted in the 100 seed method experiments) and warm stratification. Thus, it can be concluded that S. nux-vomica and S. potatorum seeds have non-deep PD, in addition to an underdeveloped embryo.
According to our observations, S. benthamii seeds have no radicle dormancy. Production of recalcitrant seeds with no radicle dormancy is an ecological adaptation to an aseasonal-wet climate (Sautu et al., Reference Sautu, Baskin, Baskin, Deago and Condit2007). Throughout the year conditions in aseasonal-wet climates are not only favourable for seed germination but also favourable for pathogens and predators. Thus, pathogenic and predatory pressure is high on seeds in wet climates especially on large seeds with high nutrient content (Foster, Reference Foster1986; Saatkamp et al., Reference Saatkamp, Poschlod, Venable and Fenner2014). Thus, faster germination is one of the strategies to avoid predation and infection of seeds in wet climates (Dalling et al., Reference Dalling, Davis, Schutte and Elizabeth2011). Recalcitrant seeds are always prepared for germination, and thus, it is a good trait for a fast germinating seed in a wet climate (Pammenter and Berjak, Reference Pammenter and Berjak1999; Daws et al., Reference Daws, Garwood and Pritchard2005). Thus, having 11 d MLG and recalcitrant behaviour is a good adaptation of S. benthamii for its habitat. On the other hand, fast germination may be less favourable for the species if they may germinate in close proximity to the mother plant. However, the S. benthamii seeds in purple colour fruits are dispersed efficiently by the frugivorous birds (personal observations). Further, as S. benthamii seeds rely on fast germination to escape from pathogens and predators they do not rely on chemical defense (according to Dalling et al., Reference Dalling, Davis, Schutte and Elizabeth2011, seed defense theory). In contrast, seeds of S. nux-vomica and S. potatorum have PD, and thus, at 25°C, they had an MLG of 40 and 55, respectively, showing that they are slower germinating seeds than those of S. benthamii. Furthermore, they have orthodox storage behaviour. Thus, they remain in the soil until they receive the environmental signal for germination. These two species inhabit the dry zone and disperse seeds from February to early April which is within the minor dry season in the Sri Lankan dry zone. Thus, being desiccation-tolerant they could survive the dry season. However, seeds of these two species have a high nutrient content and are large in size. Thus, they have to have a strategy to escape predators and pathogens during their stay in the dry season. That may be the reason why seeds of these two species have poisonous chemicals, which are a chemical defense against predators and pathogens (see Dalling et al., Reference Dalling, Davis, Schutte and Elizabeth2011 for details on seed defense theory). S. nux-vomica seeds have strychnine which is a toxic alkaloid (Frédérich et al., Reference Frédérich, Choi and Verpoorte2003; Choi et al., Reference Choi, Sohn, Kim, Oh and Kim2004), while S. potatorum seeds are armed with diaboline hydrochloride, a small amount of strychnine (compared to S. nux-vomica), loganin and brucine (Singh and Dhar, Reference Singh and Dhar1977; Yadav et al., Reference Yadav, Kadam, Patel and Patil2014). Thus, we can conclude that the dormancy and storage behaviour traits of the three study species are adaptations to their habitats.
There was a considerable delay between radicle and shoot emergence for seeds of the three study species. It took more than 30 d to complete seedling development indicating a presence of eMPD (Baskin and Baskin, Reference Baskin and Baskin2014). Shoot emergence experiments were also conducted in pots in a plant house for all three species. It took a minimum of 85, 74 and 46 d to shoot emergence from radicle emergence of S. nux-vomica, S. potatorum and S. benthamii seeds, respectively. We assume that these are the minimum number of days to complete the germination of these species under favourable conditions. eMPD has been recorded in several tropical montane species; P. gardneri, P. nigra and P. zeylanica (Athugala et al., Reference Athugala, Jayasuriya, Gunaratne and Baskin2016); Bheza nitidissima and Gaetnera walkeri (Athugala et al., Reference Athugala, Jayasuriya, Gunaratne and Baskin2016, Reference Athugala, Jayasuriya, Gunarathne and Baskin2018). Athugala et al. (Reference Athugala, Jayasuriya, Gunaratne and Baskin2016) related the occurrence of epicotyl dormancy in P. nigra and P. zeylanica seeds to their peak dispersal period of mid-June to July and December to February, respectively, and to the prevailing habitat conditions at the dispersal in submontane forests in Sri Lanka. Further, these studies found that the radicle emergence occurs at the beginning of the rainy season, while the shoot emergence is delayed until the environment is favourable for the seedling establishment. There are no seasonal temperature changes in the tropical dry zone but the arrival of monsoon rain to the dry zone of Sri Lanka could be a reason for having epicotyl dormancy in the species studied. The distinct wet and dry season in the dry zone could influence the seed germination phenology, dormancy and epicotyl dormancy. Baskin and Baskin (Reference Baskin and Baskin2014) described the occurrence of epicotyl dormancy in species, especially herbs and shrubs inhabiting the temperate region. In most of these species, root emergence occurs during autumn and shoot emergence in following spring when favourable temperature conditions occur for seedling growth. In these seeds, epicotyl dormancy is alleviated by the cold stratification that they are exposed to during the winter. However, there are a few species with seeds requiring a considerably short period of warm stratification to alleviate epicotyl dormancy which is categorized as those with non-deep simple epicotyl MPD (Baskin and Baskin, Reference Baskin and Baskin2014). Furthermore, there are species with seeds requiring long periods of warm stratification to alleviate eMPD, which were categorized as seeds with deep simple epicotyl MPD. It could be suggested that tropical species fall under these two categories. Moreover, although many species have been reported to have seeds with eMPD (Hidayati et al., Reference Hidayati, Baskin and Baskin2005; Baskin et al., Reference Baskin, Chien, Chen and Baskin2009; Chien et al., Reference Chien, Chen, Chien, Baskin and Baskin2011; Dhyani et al., Reference Dhyani, Phartyal, Nautiyal and Nautiyal2013; Athugala et al., Reference Athugala, Jayasuriya, Gunarathne and Baskin2018), heretofore, eMPD was not reported in species in Loganiaceae nor in species from tropical dry mixed evergreen forests. Therefore, our report of eMPD in S. nux-vomica, S. potatorum and S. benthamii is the first record of eMPD in Loganiaceae and from tropical dry mixed evergreen forests. Athugala et al., (Reference Athugala, Jayasuriya, Gunarathne and Baskin2018) showed the occurrence of eMPD in Rubiaceae family belonging to the order Gentianales. With our current report of eMPD in Loganiaceae, a new plant family was added to the list of 19 families (Athugala et al., Reference Athugala, Jayasuriya, Gunarathne and Baskin2018) with species producing eMPD seeds.