Introduction
Understanding the mechanisms of desiccation tolerance and storability has long been a focus of seed research. In any study involving comparisons across genotypes of seed traits such as longevity or vigour, consideration of the timing of peak physiological maturity and post-harvest factors are extremely important (Hay and Probert, Reference Hay and Probert1995, Reference Hay and Probert2013; Probert et al., Reference Probert, Adams, Coneybeer, Crawford and Hay2007; Hay et al., Reference Hay, Timple and van Duijn2015; Whitehouse et al., Reference Whitehouse, Hay and Ellis2015). However, these traits themselves, the timing of harvest maturity when seed quality (including longevity) is maximal, or the response to drying, may also vary between genotypes and due to genotype × environment interaction. It is perhaps partly for this reason that identifying the mechanisms involved in maintaining seed viability during storage is still a difficult topic to study.
Among various theories, studies have shown that free radical scavenging by secondary metabolites and dynamic metabolic pathways such as the glutathione-ascorbate cycle, reduces damage to lipid membranes and leads to greater seed longevity (Noctor and Foyer, Reference Noctor and Foyer1998; Lehner et al., Reference Lehner, Mamadou, Poels, Côme, Bailly and Corbineau2008; Hameed et al., Reference Hameed, Rasheed, Gul and Khan2014). In particular, vitamin E (tocols) is known to scavenge lipid peroxy radicals because of their unique chromanol ring structure (Liebler, Reference Liebler1993). Arabidopsis mutants with a disrupted tocopherol-cycle were vitamin E deficient, and had significantly reduced seed longevity due to failure in defence against lipid peroxidation (Sattler et al., Reference Sattler, Gilliland, Magallanes-Lundback, Pollard and DellaPenna2004). A recent rice study using transgenic lines with constitutively silenced tocopherol-cycle genes reported similar results (Chen et al., Reference Chen, Li, Fang, Shi and Chen2015). However, a genome-wide association study using diverse barley accessions concluded that there was no such correlation between tocols and seed longevity (Nagel et al., Reference Nagel, Kranner, Neumann, Rolletschek, Seal, Colville, Fernández-Marín and Börner2015). This apparent conflict could be the result of different experimental methods or multiple longevity strategies among various species. There is also a possibility that intraspecific variation in genomic structure affects overall correlation results.
In the case of rice, population structure analysis has distinguished five variety groups: Indica, Aus/Boro, Basmati/Sadri, tropical Japonica and temperate Japonica (The 3,000 Rice Genomes Project, 2014). Based on the results of experimental storage at elevated moisture content and temperature, significant differences in seed longevity parameters were observed among rice variety groups (Hay et al., Reference Hay, Timple and van Duijn2015). Kim et al. (Reference Kim, Kwak, Baik, Yoon, Lee, Yoon and Kim2015) reported that tocopherol composition in brown rice was quite different between a Japonica and an Indica variety. This could be due to significant heterogeneity in the genetic architecture associated with the groups (Zhao et al., Reference Zhao, Tung, Eizenga, Wright, Ali, Price, Norton, Islam, Reynolds, Mezey, Mcclung, Bustamante and McCouch2011), thus the ability to tolerate long periods in air-dry conditions and strategies to reduce and/or overcome seed deterioration may differ among variety groups. The aims of this study were to determine the tocols composition in rice variety groups and evaluate correlation between tocols ratio and seed longevity.
Materials and methods
Seed lots
Seed lots and longevity data used in this study are as described in Hay et al. (Reference Hay, Timple and van Duijn2015). Briefly, seeds of 20 Oryza sativa accessions held in the T. T. Chang Genetic Resources Center, representing Indica (eight accessions), Aus/Boro (four accessions), tropical Japonica (three accessions), temperate Japonica (four accessions) and Basmati/Sadri (one accession) groups (Table 1) were grown on the Zeigler Experimental Station of the International Rice Research Institute in the 2013 dry season. Seed lots were harvested at four different maturity stages (24, 31, 38 and 45 days after peak flowering) and used for storage experiments at 45°C after equilibration to 60% relative humidity at room temperature (approximately 22°C). Samples were taken at regular intervals and tested for ability to germinate. Some samples were also used for moisture content determination. For each accession, the maturity stage with the greatest longevity (time for viability to fall to 50%; p 50) was chosen for this study.
Table 1. Information on 20 rice seed lots used in this study
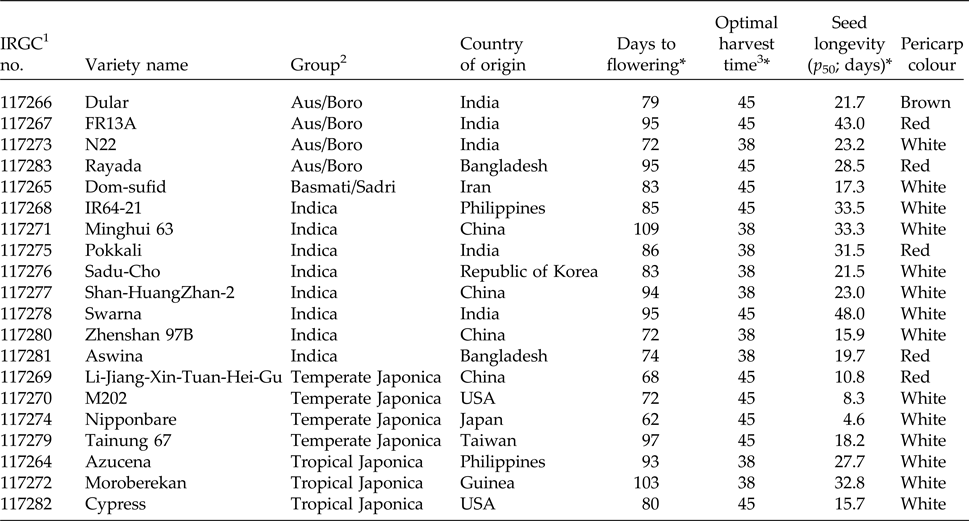
1 The international rice genebank collection (IRGC).
2 Groups based on population structure (McNally et al., Reference McNally, Childs, Bohnert, Davidson, Zhao, Ulat, Zeller, Clark, Hoen, Bureau, Stokowski, Ballinger, Frazer, Cox, Padhukasahasram, Bustamante, Weigel, Mackill, Bruskiewich, Ratsch, Buell, Leung and Leach2009).
3 Days after flowering (DAF). All accessions were harvested at 31, 38 and 45 DAF; the optimal harvest time is the seed lot which gave the highest p 50 from those three harvest times.
* Data taken from Hay et al. (Reference Hay, Timple and van Duijn2015).
Tocols analysis
Tocopherol isomers in lipid extracts of brown rice (caryopses) were analysed following Ko et al. (Reference Ko, Kim, Kim, Kim, Chung, Tae and Kim2003). Seeds were de-hulled (palea and lemma removed to leave the caryopsis) by a rubber roller husker (SY88-TH, Ssangyong Ltd, Incheon, Korea). Ground brown rice tissue was mixed with 300 ml n-hexane for 2 h, then concentrated by evaporating hexane using nitrogen gas. About 0.5 g of lipid extracts was mixed with 2 ml 5% pyrogallol solution in ethanol plus 20 ml ethanol in a flat-bottomed flask and incubated at 70°C on a hot plate. After boiling, 1 ml of 50% aqueous KOH was added. After 5 min saponification, the flask was cooled and the mixture transferred to a 250 ml separatory funnel. Each sample was extracted twice using 50 ml diethyl ether. The pooled diethyl ether layers were washed five times with 20 ml distilled water, filtered through anhydrous sodium sulphate, and then evaporated at 30°C. The residue was diluted with 10 ml n-hexane and filtered through a Millipore 0.2 µm membrane. Individual tocopherol isomers were determined by ultra performance liquid chromatography (UPLC, H-Class system, Waters, USA) at 298 nm excitation and 325 nm emission with a Lichrospher Si-60 column (250 × 4.6 mm i.d.; Merck Co., Germany). The mobile phase was n-hexane:isopropanol (99:1, v/v) at 1.0 ml min–1. Tocopherol isomers were quantified based on retention time and amounts of standard tocopherols (Darmstadt, Germany) and tocotrienols (Helios, Singapore).
Statistical analysis
Two subsets from each seed lot were used for tocols analysis and standard error between replicates was monitored (supplementary Table S1). All analyses were carried out using STAR v2.0.1 (International Rice Research Institute). Data were analysed by ANOVA and means were tested for significant differences using least significant difference (LSD; P < 0.05). Correlations between seed longevity (p 50, as given in Hay et al., Reference Hay, Timple and van Duijn2015; Table 1) value and proportion of tocols were determined.
Association analysis
To examine interactions between DNA sequences and phenotype, we utilized the 700 K high-density single-nucleotide polymorphism (SNP) marker data described by McCouch et al. (Reference McCouch, Wright, Tung, Maron, McNally, Fitzgerald, Singh, DeClerck, Agosto-Perez, Korniliev, Greenberg, Naredo, Mercado, Harrington, Shi, Branchini, Kuser-Falcaõ, Leung, Ebana, Yano, Eizenga, McClung and Mezey2016). From the data set, SNP markers in the region of tocols-related genes were extracted and effects of haplotype on the phenotype in each marker determined.
Results
Variation in tocols between variety groups
The proportion of lipids (location of tocols) in the brown rice (fresh weight) ranged between 2.11 (IR64-21) and 3.22% (Minghui 63) with a mean across the genotypes of 2.46% (Table 2). Eight types of tocopherol homologues were quantified by UPLC analysis. In general, α- and γ-types were predominant, whereas β- and δ-types were rarely detected. Temperate Japonica varieties showed much higher total tocopherol content (11.70 mg kg–1) compared with Indica (7.15 mg kg–1), Aus/Boro (7.49 mg kg–1) and tropical Japonica (8.22 mg kg–1) varieties. However, total tocotrienol content in the temperate Japonica group was lower (13.49 mg kg–1) than that of other groups (ranging from 14.10 to 16.01 mg kg–1). Among the eight homologues, large variation between variety groups was observed for α-tocopherol, α-tocotrienol and γ-tocotrienol contents. Temperate Japonica varieties were most abundant in α-tocopherol and α-tocotrienol, with 8.79 and 5.07 mg kg–1, respectively. In contrast, the γ-tocotrienol content of the temperate Japonica group was significantly lower (7.60 mg kg–1) than the Indica (13.14 mg kg–1), Aus/Boro (9.70 mg kg–1) and tropical Japonica (8.89 mg kg–1) groups. Overall, the ratio of α- to γ-tocols was most different between Indica and temperate Japonica groups, while Aus/Boro and tropical Japonica groups showed intermediate trends.
Table 2. Tocols in the brown rice of 20 varieties from different variety groups.
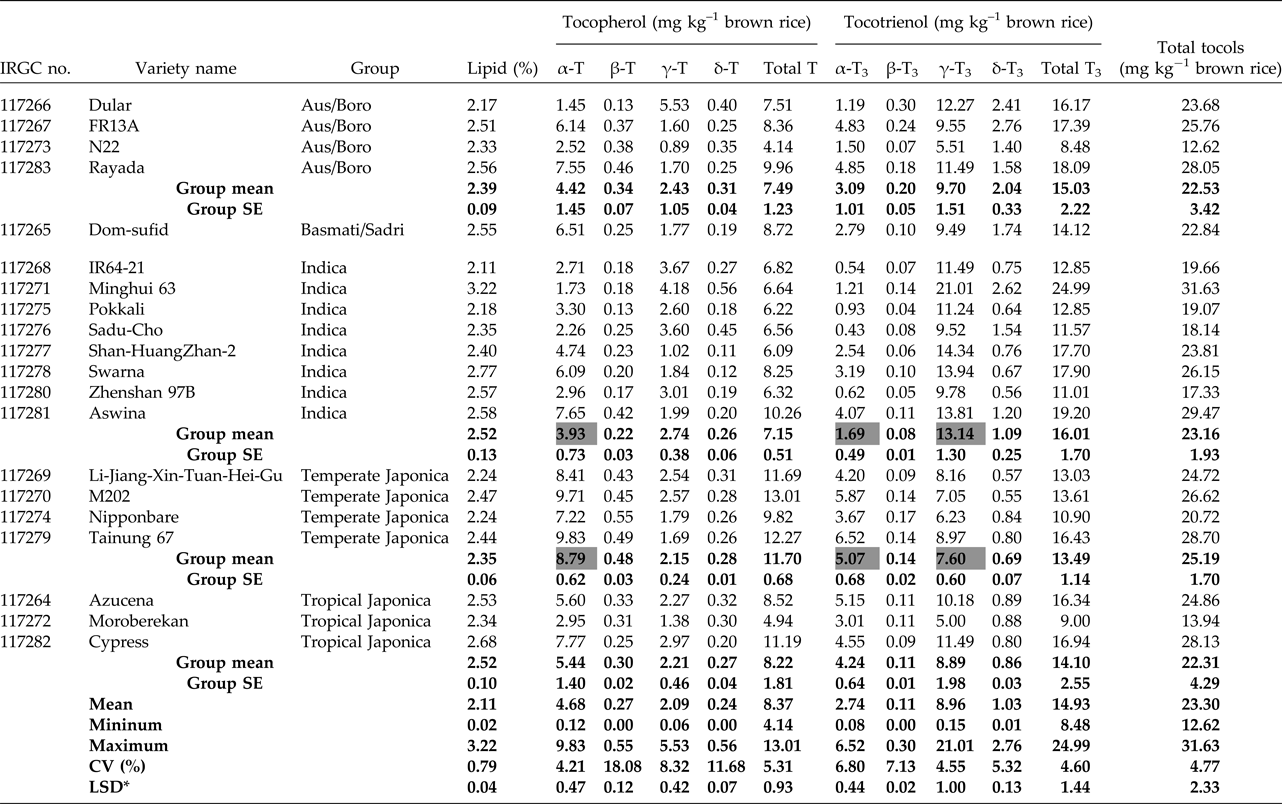
* LSD (P < 0.05) for the comparison of genotypic means. Shaded cells highlight the difference between Indica and temperate Japonica groups in the tocols that vary between varieties.
Correlation with seed longevity
Positive correlations were observed between log10 p 50 and γ- and δ-tocols while negative correlations were found between log10 p 50 and α- and β-types (Table 3). Seed longevity was significantly positively correlated with γ-tocotrienol (absolute value 0.546; P < 0.05) and significantly negatively correlated with α-tocopherol (absolute value –0.581; P < 0.01). For example, Swarna with great longevity (p 50: 48.0 days) possessed twofold higher γ-tocotrienol but lower (0.6-fold) α-tocopherol than M202, one of the poorest longevity accessions (p 50: 8.3 days) (Fig. 1; Tables 1 and 2). High longevity accessions (p 50 > 25 days) showed a tendency for higher γ-tocotrienol content with the exception of two accessions, FR13A (Aus/Boro) and Moroberekan (tropical Japonica) (supplementary Fig. S1). FR13A had great longevity (p 50: 43.0 days) but relatively low γ-tocotrienol content (9.55 mg kg–1). In spite of high longevity (p 50: 32.8 days), Moroberekan recorded the lowest γ-tocotrienol content (5.00 mg kg–1). Excluding two exceptional accessions, correlation between log10 p 50 and the proportion of γ-tocotrienol increased from 0.546 to 0.709 (Table 3). Furthermore, the correlation with α- and β-tocopherols was also stronger (–0.626 and –0.557, respectively).

Figure 1. Comparison of tocol homologue chromatograms between Swarna (relatively high seed longevity) and M202 (relatively poor seed longevity).
Table 3. Correlation coefficients between seed longevity (log10 p 50) and proportion of tocols for 20 diverse genotypes of rice

*Significant at P < 0.05; **significant at P < 0.01; n.s., not significant (P > 0.05). aAll accessions; n = 20.
b Excluding two exceptional accessions, FR13A and Moroberekan; n = 18.
Discussion
While previous studies have considered total tocols (or α-tocopherol) content in relation to seed longevity (Sattler et al., Reference Sattler, Gilliland, Magallanes-Lundback, Pollard and DellaPenna2004; Chen et al., Reference Chen, Li, Fang, Shi and Chen2015), we have focused on the proportions of individual tocol homologues in different variety groups of rice that have recently been defined by population structure analysis (The 3,000 Rice Genomes Project, 2014; McCouch et al., Reference McCouch, Wright, Tung, Maron, McNally, Fitzgerald, Singh, DeClerck, Agosto-Perez, Korniliev, Greenberg, Naredo, Mercado, Harrington, Shi, Branchini, Kuser-Falcaõ, Leung, Ebana, Yano, Eizenga, McClung and Mezey2016). There were clear variations in the ratio of α- to γ-tocols. DNA haplotype–phenotype interaction may explain the variation in γ-tocotrienol proportion, which tended to be higher in high-longevity accessions. Among eight known genes associated with the tocopherol-cycle (The Rice Annotation Project database: http://rice.plantbiology.msu.edu; Rice SNP-Seek Database: http://snp-seek.irri.org), clear allelic variations were observed in the region of three genes: Os01g0264700 (α-tocopherol transporter) on chromosome 1, and Os02g0276500 (tocopherol cyclase) and Os02g0701600 (γ-tocopherol methyltransferase) on chromosome 2 (Fig. 2). Accessions (mostly Indica) having positive minor haplotypes showed higher γ-tocotrienol proportion compared with negative major haplotypes. In particular, one non-synonymous SNP variant in the coding region (at position 28,895,665) of Os02g0701600, which is known to facilitate γ-tocotrienol conversion process into α-tocopherol (Sattler et al., Reference Sattler, Cahoon, Coughlan and DellaPenna2003), enhanced γ-tocotrienol proportion by 41.5% compared with the negative haplotype. This indicates that a non-synonymous mutation regulates the efficiency of conversion process, i.e. the positive haplotype slowed down conversion process resulting in less α-tocopherol but high γ-tocotrienol (Fig. 3). Wang et al. (Reference Wang, Song and Li2013) also reported similar results that α-tocopherol level significantly increased in Japonica varieties having certain haplotypes on Os02g0701600. Since this association analysis was performed with a low number of accessions, from a statistical point of view this result needs to be validated through further appropriate genome-wide association analysis using many more accessions. The other limitation of this study was that tocols were extracted from the whole grains rather than isolated embryos. This is because of the small size of rice embryos and hence the difficulty in isolating embryos.

Figure 2. Haplotype analysis in comparison with positive minor haplotypes (green shading) for three genes associated with the tocopherol-cycle. Three accessions, IRGC 117270, 117273 and 117277, were not included in the high-density SNP data set.

Figure 3. Seed longevity maintenance via tocopherol biosynthetic pathway-induced anti-oxidant activity (Sattler et al., Reference Sattler, Cahoon, Coughlan and DellaPenna2003; Lushchak and Semchuk, Reference Lushchak and Semchuk2012).
Although many biochemical studies demonstrated α-tocopherol as a strong anti-oxidant, temperate Japonica accessions containing high amount of α-tocopherol showed poor seed longevity (Tables 1 and 2; Fig. 1). On the other hand, Indica accessions containing high γ-tocotrienol had good seed longevity. Vitamin E is a chain-breaking anti-oxidant and tocotrienols possess three additional double bonds on the isoprenoid side chain (Traber and Stevens, Reference Traber and Stevens2011). Furthermore, tocotrienols with an unsaturated side chain can be more uniformly distributed in the lipid bi-layer as scavenging lipid peroxyl radicals (Aggarwal et al., Reference Aggarwal, Sundaram, Prasad and Kannappan2010). While previous studies reported the strongest anti-oxidant capacity of α-tocols (Kamal-Eldin and Appelqvist, Reference Kamal-Eldin and Appelqvist1996), several recent experiments demonstrated that γ- and δ-tocols have more efficient and synergistic anti-oxidant activity than α-type (Kadoma et al., Reference Kadoma, Ishihara, Okada and Fujisawa2006, Jiang, Reference Jiang2014; Kim, Reference Kim2014).
Correlation between the ratio of tocols (in particular α-tocopherol, α-tocotrienol and γ-tocotrienol) and seed longevity were highly significant (Table 3). Nevertheless, the absolute values were not enough to conclude that tocols ratio per se decided the seed longevity. Therefore, other biochemical factors besides tocols should be examined in further studies. Coloured rice varieties are known to contain high amounts of various anti-oxidants such as phenolic acid, flavonoids, coumarins, tannins and anthocyanins (Goufo and Trindade, Reference Goufo and Trindade2013). Such anti-oxidants, present in the red-coloured pericarp of FR13A (Table 1), in spite of its low ratio of α- to γ-tocols, might contribute to prolonging seed longevity during storage. In the temperate Japonica group, one coloured rice variety, Li-Jiang-Xin-Tuan-Hei-Gu, recorded greater seed longevity than two of the three white varieties (Table 1). Moroberekan (tropical Japonica) is a white rice variety and recorded the lowest γ-tocotrienol content as well as total tocols contents (Table 2). However, the longevity of Moroberekan seeds was very high (p 50: 32.8 days), thus this accession must have a different mechanism to extend seed longevity rather than a tocols-related strategy or high anti-oxidant composition.
Acknowledgements
We would like to thank Dmytro Chebotarov (IRRI) for technical advice on genotype data.
Financial support
This research was funded by a grant from Rural Development Administration, Korea (RDA) as RDA-IRRI cooperative research project (grant reference PJ012723).
Conflicts of interest
None.
Supplementary material
Fig. S1: Correlation between seed longevity and tocols contents. Square boxes indicates two unique varieties: FR13A (Aus/Boro) and Moroberekan (tropical Japonica). FR13A: high longevity with high α-tocopherol content; Moroberekan: high longevity with the lowest γ-tocotrienol content. Table S1: Contents (mg g−1 oil) of tocols in 20 varieties of rice from the International Rice Genebank Collection (IRGC).
To view supplementary material for this article, please visit https://doi.org/10.1017/S0960258517000265.