Introduction
As the price of conventional fertilizer soars and interest in alternative sources of crop fertilizer growsReference Adeli, Tewolde, Sistani and Rowe1, there is an increasing demand for on-farm sources of soil fertilityReference Watson, Atkinson, Gosling, Jackson and Rayns2. Integrating livestock and crop production is one channel by which agricultural practitioners can enhance soil fertility using on-farm resources of animal manure and pasture plantsReference Acosta-Martinez, Zobeck and Allen3–Reference Hilimire5. Farmer interest in utilizing pasture-raised chickens (Gallus gallus domesticus), in particular, to improve soil fertility and enhance crop performance has grown in recent years, as has consumer demand for pastured poultry productsReference Burbaugh, Toro and Gernat6–Reference Hilimire8. In pastured production, poultry live outdoors and consume pasture plants and grainReference Ponte, Prates, Crespo, Crespo, Mourao, Alves, Bessa, Chaveiro-Soares, Gama, Ferreira and Fontes9, Reference Karsten, Patterson, Stout and Crews10. Relatively few peer-reviewed articles have been published regarding soil fertility and crop growth under pastured poultry management, yet inferences about the role of poultry on soil fertility can be made from the substantial literature on the fertilizer content values of poultry manure and litterReference Adeli, Tewolde, Sistani and Rowe1, Reference Sainju, Senwo, Nyakatawa, Tazisong and Reddy11–Reference Edmeades13.
Poultry manure and litter are known effective plant fertilizers. Various estimates give the nutrient concentrations of poultry manure at an average of 5.1% nitrogen (N), 1.9% phosphorus (P) and 2.6% potassium (K)Reference Sims and Wolf14–Reference Edwards and Daniel16. Many field studies suggest that poultry manure and/or litter application to crop soils can enhance chemical, biological and physical quality, and plant growth. Sainju et al.Reference Sainju, Senwo, Nyakatawa, Tazisong and Reddy11 found broiler litter increased soil carbon (C) and N sequestration in intensive cropping systems at a depth of 0–10cm. Adeli et al.Reference Adeli, Tewolde, Sistani and Rowe1 reported that broiler litter application to cropping systems linearly increased extractable soil P, K, soil cation exchange capacity and soil total C at 0–15cm in a 3-year study. Land application of poultry litter has also been found comparable to inorganic fertilizer for supplying soil P and increasing forage yieldReference McGrath, Maguire, Tracy and Fike17.
Poultry manure and litter have been widely studied because the poultry industry generates high quantities that are frequently applied to crop or pasture fieldsReference Sainju, Senwo, Nyakatawa, Tazisong and Reddy11, Reference Kelleher, Leahy, Henihan, O'Dwyer, Sutton and Leahy18. Less is known about the effect of utilizing live birds for soil fertilization. Manure deposited by live birds is surface-applied and not tractor-incorporated; although Kratz et al.Reference Kratz, Rogasik and Schnug19 reported that fecal N and P inputs by free-ranging birds corresponded with increased soil N and P levels. Studies such as these suggest that integrating live poultry into crop agroecosystems may effect significant changes to soil fertility and yield.
The objectives of this study were to: (1) determine whether soil from poultry pasture (PPAS) enhanced soil fertility in comparison with typical organic non-animal based management (control); and (2) identify whether crops grown in soil from PPAS fields achieved equivalent growth parameters as crops grown in control soils. These effects were assessed for two agroecosystems: (1) a mixed annual/berry system where crops were planted subsequent to removal of birds from pasture; and (2) an orchard setting where birds were temporally and spatially integrated with crop production.
Methods
Study region and experiments conducted
Research was conducted between 2009 and 2011 in the Central Coast region of California at two working farms. The region is characterized by a nearly year-round agricultural growing season with a mild Mediterranean climate. The rainy, cooler season is from November to April, and the dry, warm season is from May to October. Three assessments were conducted: for objective 1, soil fertility parameters were compared from adjacent plots on two farms, and spatial variation in soil fertility was assessed relative to location of poultry coops. For objective 2, crop plants were grown in a replicated greenhouse experiment using soils from plots with a recent history of pastured poultry and soils from adjacent cropped areas without a history of pastured poultry.
Site selection
This research was conducted on working farms, making site selection a critical aspect of experimental design. The advantage of agroecological studies based on working farms is that they reflect real-world farming conditions and generate data based on actual management practices. The disadvantage is a reduced ability to replicate treatments and choose ideal controls. In choosing sites for this research, we sought farms with management practices representative of integrated pastured poultry/crop production. Field histories and farmer interviews were used to determine that there were no a priori reasons for poultry placement related to soil fertility and that plots were similar enough to deduce whether differences were due to the presence or absence of poultry and pasture. Two farms were selected that met all requirements. At each farm we established two adjacent plots, one with a history of crop production and one with a history of pastured poultry. Adjacent plots had similar cropping histories and identical soil types and slopes.
Research sites
Farm A was used as an exemplar of PPAS in a mixed annual/berry agroecosystem. Soil is a Lockwood series (fine-loamy, mixed, Thermic and Pachic Argixerolls). Texture of the topsoil is clay (sand: 28.4%, silt: 28.4% and clay: 43.2%). At this site, hens were the integrated animals, and plots were alternately dedicated to crop and pasture production. Plot sizes were 24.5m×7m (Fig. 1).

Figure 1. Experimental design of soil fertility study showing transects (bold lines), distance between transects, and buffer zones at both sites.
At this site, the pasture plot had a 2-year history of poultry grazing (2470birds per ha). Pasture fields were seeded with fescue (Festuca arundinacea), orchardgrass (Dactylis glomerata), ryegrass (Lolium spp.), clover (Trifolium spp.) and trefoil (Lotus corniculatus), and intermittently ranged by hens for 2 years before being converted back to crop production. Prior to pasture, the treatment field was planted with popcorn (Zea mays averta) in 2007 and pumpkins (Cucurbita spp.) in 2006. During crop sequences, plots were planted with cover crops [bell beans (Vicia faba), purple vetch (Vicia benghalensis), peas (Pisum sativum) and oats (Avena sativa)] during the winter season. While managed as pasture, overhead irrigation was used, and during crop rotations, drip irrigation was employed.
The control field had a 2-year history of wheat (Triticum sp.) and popcorn (Zea mays averta) during the time the treatment field was planted as pasture and grazed by poultry. Previously, the control field was planted with wheat in 2007 and popcorn in 2006. Winter cover crops, as described above, were planted into these crop rotations. Compost and lime were added to all crop sequences biennially, both at a rate of 2.4Mgha−1. Both fields were converted to strawberry (Fragaria sp.) production subsequent to the first day of data collection. During strawberry cultivation, winter cover crops were not utilized.
Farm B was used as an exemplar of PPAS in an orchard system. Soil is a Sorrento series (fine-loamy, mixed, Thermic and Calcic Haploxerolls). Texture of the topsoil is sandy clay loam (sand: 51.4%, silt: 26.4%, and clay: 22.2%). At this site, meat chickens were integrated into a portion of walnut (Juglans regia) orchard on pasture seeded as above. Plot size was 68m×50m (Fig. 1). An equally sized section of the orchard with no prior history of poultry was used as the control, and both plots had equivalent walnut tree density (100trees per ha). Chickens grazed the PPAS orchard from April to September annually for the previous 7 years (2470birds per ha). The control orchard had no history of poultry and was planted with the same cover crop mix described above. No other amendments were added to either plot. Both plots were irrigated on the same schedule, receiving water three times per year for 12h.
Data collection for soil fertility
This assessment was conducted from 2009 to 2011. Using a stratified composite sampling designReference Quinn and Keough20, eight composite samples per plot were gathered. Each composite sample was composed of 15 thoroughly combined sub-samples. Samples were gathered from two depths (0–15 and 15–30cm) along eight permanent transects per site in two plots (Fig. 1), and sub-samples of each composite sample were used for analysis. Field history and farmer interviews were obtained to determine that no a priori reasons existed for delineation of field margins (e.g., soil-type differences or productivity), and transects were placed so that the distance between transects was greater than the distance between treatments to rule out stochastic spatial variation. At Farm A, transects were not true replicates because the treatment field was grazed by a single flock of hens, meaning that all data collected represent the effect of one flock. For this reason, we present data from this site as repeated measures. At Farm B, transects were true replicates because meat chickens were housed in discrete pens, and each transect represents the effect of a distinct flock. Data from this site are presented as means across time.
Inorganic N was measured four times per year (n=256)Reference Allan, Killorn, Doran and Jones21, analyzed for ammonium–nitrogen (NH4+–N) and nitrate–nitrogen (NO3−–N) on a flow injection analyzer (Lachat QuikChem 8000, Milwaukee, WI)22 at the University of California, Santa Cruz (UCSC). Total C and total N were measured twice yearly (n=64) and assessed on the VarioMAX CNS analyzer (Elementar, Mt. Laurel, NJ)23 at UCSC. Calcic properties of Farm B soils were limited to >50cm depth, making inorganic C removal from soils prior to total C analysis unnecessary because sample soils were collected from surface depth increments of 0–15 and 15–30cm. Soil electrical conductivityReference Smith, Doran, Doran and Jones24 was determined twice yearly (n=31, missing one sample, topsoil only) as a saturated paste using a conductivity meter (Denver Instrument, Model 250) at UCSC. Extractable sodium bicarbonate P (Olsen P), exchangeable K (ex. K), pH (saturated paste), cation exchange capacity and soil organic matter as determined by weight loss-on-ignition were assessed four times per year (n=128)Reference Ankerman and Large25 at A&L Laboratories (Modesto, CA).
In a sub-study conducted in February 2010, spatial variation in soil fertility was assessed for the coop-based system at Farm A. Hens, unlike the meat chickens at Farm B, ranged within their fields during the day, frequently seeking shelter under the coop. This study was conducted to determine whether soil fertility values changed with distance from the coop. Samples were taken every 4m on four 16-m transects at two coops (n=20). Soil samples were collected from a depth of 0–15cm and analyzed for inorganic N, Olsen P, ex. K, pH, cation exchange capacity and organic matter, using the methods described above.
Greenhouse trial
To address the question of crop growth response to PPAS independent of farmer management, a series of greenhouse experiments were conducted using soil from PPAS and control plots. From each plot, five gallons of topsoil (0–15cm depth) were collected from 20 random sub-samples. Soil was then air-dried, mixed well and crushed to approximately 2mm diameter aggregates. Two bean seeds (Phaseolus vulgaris L. var. Hystyle) were planted on November 5, 2009 with Farm A soil and thinned to one shoot on November 11, 2009 (n=33). In the same manner, sunflowers (Helianthus annuus L.) were planted on April 23, 2010 with soil from Farms A and B and thinned on April 29, 2010 (n=174). Containers were arranged in a random formation, misted daily and grown at daytime temperatures of 22.8–23.9 °C for 30 days in the UCSC greenhouse. At 30 days from seeding, plants were clipped at the soil level and measured for height. Plants were then oven-dried at 60 °C for 36h and the biomass weighed.
Data analysis
True replication of treatment within site at Farm A was not viable due to the scale of the treatment, and management practices between the two farms differed too greatly to warrant statistical comparison across farms. Instead, we present results as two distinct case studies. Two-tailed Student's t-tests were conducted for most data analysis with the exception of the spatial variation study, where simple linear regressions were used to determine the effect of distance from coop on soil fertility parameters. JMP, version 8 was used for all statistical tests26 with the alpha level set at 0.05. The following data with non-normal distributions were transformed to meet assumptions of normality: log10 of total C at Farm A (15–30cm) and Farm B (both depths); total N at Farm B (15–30cm); NH4+–N at Farm A (15–30cm) and Farm B (both depths); NO3−–N for all tests; Olsen P at Farm A (0–15cm) and Farm B (both depths); ex. K at Farm B (15–30cm); and organic matter at Farm B (15–30cm). For the spatial variation data, NH4+–N, NO3−–N and Olsen P were log10 transformed, and ex. K data were square root transformed. However, original means and standard errors are reported and graphically displayed hereafter for ease of interpretation.
Results
Effects on soil fertility
At Farm A, PPAS plots had elevated soil pH, total N, organic matter and electrical conductivity levels relative to the control at a depth of 0–15cm at day 0, the day birds were removed from pasture and pre-conversion to strawberries (Table 1). Subsequent to removal of birds from pasture and conversion to strawberries, pH values remained significantly higher. Soil inorganic N differences were statistically significant for NH4+–N at 98 days after conversion at the 0–15cm depth and at 51 days for the 15–30cm depth. Soil NO3−–N differences differed significantly at 51 and 98 days after conversion at the 15–30cm depth. No other significant differences were found at the 15–30cm depth.
Table 1. Farm A soil fertility results for the 0–15cm depth except where indicated.

Figures in parentheses are standard errors.
Results are shown from time zero until the sampling date after which all differences were neutral.
‘dir.’ indicates direction of change for significant results of PPAS relative to the control.
* indicates statistically significant differences.
At Farm B, PPAS plots had decreased soil pH and elevated total C, total N, NH4+–N, NO3−–N, Olsen P, ex. K, organic matter, cation exchange capacity and electrical conductivity levels relative to the control (Table 2). These results were consistent at the 15–30cm depth with the exception of soil organic matter.
Table 2. Farm B soil fertility results.

Figures in parentheses are standard errors.
‘dir.’ indicates direction of change for significant results of PPAS relative to the control.
* indicates statistically significant differences.
Spatial variation in soil fertility results
Certain soil fertility parameters varied strongly along spatial gradients within PPAS fields where free-ranging hens lived. Regression analysis showed that soil NH4+–N, NO3−–N, Olsen P and ex. K all significantly decreased with distance from coop at a depth of 0–15cm (Fig. 2). Soil pH, organic matter and cation exchange capacity were unaffected. Correlations of the following parameters and distance from coop were significant: NH4+–N (r 2=0.42, F 1,18=13.27, P=0.0019); NO3−–N (r 2=0.79, F 1,18=68.28, P<0.0001); Olsen P (r 2=0.06, F 1,18=1.15, P<0.0001); ex. K (r 2=0.33, F 1,18=8.88, P=0.008).

Figure 2. Regressions of soil ammonium–nitrogen (NH4+–N), nitrate–nitrogen (NO3−–N), extractable sodium bicarbonate P (Olsen P) and exchangeable K (ex. K) showing significant correlations to distance from coop.
Greenhouse trial
PPAS soil improved crop growth relative to the control for both biomass and plant height, showing a neutral effect only for sunflower biomass at one site. PPAS soil enhanced average bean biomass by a factor of 1.48 in Farm A soil (Fig. 3, t(30.48)=3.31, P=0.0024; Farm B soil not tested). Biomass results for sunflowers varied by farm. At Farm A, PPAS soil enhanced average sunflower biomass by a factor of 1.08 (Fig. 4, t(54.94)=3.56, P=0.0008). At Farm B, there was no significant difference in average biomass between plants grown in PPAS and control soils (Fig. 4, t(49.28)=1.51, P=0.1373). PPAS soil enhanced sunflower height relative to the control group by a factor of 1.11 at Farm A (Fig. 5, t(57.92)=2.09, P=0.041) and by a factor of 1.06 at Farm B (Fig. 5, t(108.17)=2.30, P=0.023).

Figure 3. Biomass (grams/plant) of beans grown in soils from the control and PPAS with standard errors.
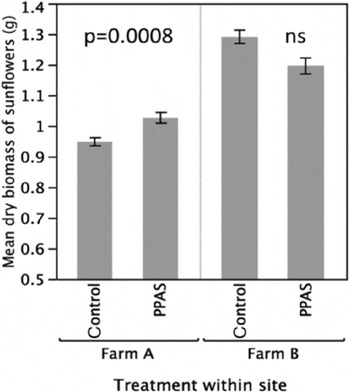
Figure 4. Biomass of sunflowers (grams/plant) grown in soils from the control and PPAS within farm with standard errors.

Figure 5. Height of sunflowers (cm/plant) grown in soils from the control and PPAS within farm with standard errors.
Discussion
PPAS plots had elevated soil fertility parameters of soil total C, total N, NH4+–N, NO3−–N, Olsen P, ex. K, organic matter and cation exchange capacity levels relative to the control. These changes conferred greater biomass and height to plants grown in these soils in the greenhouse in most cases. Some of these soil fertility parameters varied along spatial gradients within the PPAS fields where free-ranging hens lived. PPAS plots had highly increased soil Olsen P levels relative to the control at Farm B, with implications for environmental contamination of adjacent waterways. These results suggest that, despite the fact that in pasture-based systems manure is applied gradually by poultry rather than as a one-time mechanically incorporated application, PPAS does significantly affect soil fertility for multiple parameters, although it is not clear whether this effect is due to poultry manure or pasture plants.
Soil pH was raised relative to the control at Farm A, which is consistent with other studies of the effect of poultry manure and litter on soil acidityReference Adeli, Tewolde, Sistani and Rowe1, Reference Amiri and Fallahi27, Reference Kingery, Wood, Delaney, Williams and Mullins28. Hen poultry manure tends to increase soil pH because poultry feed contains calcium carbonate (CaCO3) as an additiveReference Mokolobate and Haynes29. Feeds designated for hens contain more CaCO3 than meat chicken feed to aid in shell production. Contrary to this phenomenon, manure in general can have a soil acidifying effect; when NH4+ is converted to NO3− and H+ ions are released to the soil, pH is loweredReference Sommer and Hutchings30. At Farm B, PPAS decreased soil pH. At this site, meat chickens were the integrated animal, which means that substantially less CaCO3 was present in the feed, and the liming effect of the feed was apparently not greater than the acidifying effect of the manure. Also having an effect on soil pH at these sites was the buffering capacity of the soil, as determined by cation exchange capacity. At Farm A, soil cation exchange capacity was higher than Farm B soil cation exchange capacity, meaning that more CaCO3 would be required to increase the pH of the soil than at Farm B. With hen feed containing more CaCO3 than meat chicken feed, this effect was achieved. These inorganic C additions were likely minor sources of increased total C measured in PPAS soils. Rather, soil total C increases are attributed largely to significant increases in organic matter.
PPAS plots showed elevated soil total C relative to the control at Farm B, the site with a 7-year history of PPAS. Similarly, other studies of manure have found a relationship between long-term manure applications and soil total C increaseReference Adeli, Tewolde, Sistani and Rowe1, Reference Bulluck, Brosius, Evanylo and Ristaino31. Soil total N was also increased by PPAS at both sites. Total N in poultry manure comprised 40–70% uric acid (C5H4N4O3), which is readily converted to ammonia (NH3) in soilReference Kratz, Rogasik and Schnug19, Reference Kirchmann and Witter32. NH3 is then either subject to volatilization or conversion to NH4+, depending on soil pH, and eventually NO3− by nitrification. NH4+–N and NO3−–N were increased in PPAS plots at both sites. Soil ex. K was also enhanced in PPAS plots at Farm B.
In addition to these chemical changes, soil organic matter was raised relative to the control in PPAS plots at the 0–15cm depth for both sites. High soil organic matter is associated with high soil nutrient-holding capacity, low penetration resistance and a biologically active soil ecosystemReference Edmeades13, Reference Haynes and Naidu33, Reference Goulding, Jarvis and Whitmore34. Soil cation exchange capacity was also raised in PPAS plots relative to the control at Farm B but not Farm A, which is explained by the soil type of each site. Farm B had a sandy clay loam with 22% clay content, while Farm A had a clay soil with 43% clay content. At Farm A, the control soil had a substantially higher cation exchange capacity than the control Farm B, which is consistent with cation exchange properties of clay. At Farm B, PPAS plots has significantly elevated soil organic matter levels, which would lead to an increase in measureable cation exchange capacity for a soil not dominated by clay. Soil electrical conductivity was also increased in PPAS plots at both sites, although not beyond established threshold values for salt-sensitive cropsReference Maas35.
Soil P levels were highly increased in PPAS plots relative to the control at Farm B. Phosphate is an additive to poultry feed, and excessive P accumulation associated with the use of poultry manure as a crop fertilizer is a well-known phenomenonReference Maguire, Mullins and Brosius36–Reference Sharpley and Moyer38. Crops utilize approximately six times more N than P, yet N:P ratios are typically closer to 1:1 or 2:1 in manureReference Cooperband and Good37. Growers often apply manure to meet crop growth goals or N levels, resulting in P excess. Excessive soil P is lost primarily to overland runoff of P-enriched sediment and manure particlesReference Vadas, Kleinman and Sharpley39. Loss of P to adjacent waterways can lead to eutrophicationReference Sharpley, Chapra, Wedepohl, Sims, Daniel and Reddy40. Whether soil P levels associated with pastured poultry pose an environmental risk is unique to each farm, as chemical and hydrological factors determine P retention and movementReference Maguire and Sims41. However, suggested threshold values of soil Olsen P for environmental quality are approximately 30–40mgkg−1Reference Sheffield, Brown, Chahine, de Haro Marti and Falen42, Reference do Carmo Horta43. Farm B levels exceeded this threshold for the PPAS treatment, and Farm A levels were close to the lower bound of this threshold in the PPAS treatment. Growers should be aware of this issue and conduct regular soil tests. When soil P reaches unacceptable levels for local conditions, birds should be relocated.
Finally, another issue of concern was the spatial variation in soil NH4+–N, NO3−–N, Olsen P and ex. K within PPAS fields where free-ranging hens lived. Hens tend to spend more time near or in the coop than toward the field margins, with the obvious result that they deposit more manure near the coop. Kratz et al.Reference Kratz, Rogasik and Schnug19 found similar hot spots in free-range meat chicken runs. This issue can be ameliorated with more frequent relocation of portable coops. Research is required to determine how often coops should be moved to maximize nutrient deposition.
The relevance of these values to plant growth and yield depends on the type of crop grown with or subsequent to PPAS. In the case of this study, PPAS levels were at least as effective as the input methods growers were using for control soil preparation as shown by the growth results in the greenhouse trial. At the study sites reported on in this paper, the equivalent quantity of dry manure applied to a plot was approximately 8.4Mgha−1yr−1 for layers and 10.9Mgha−1yr−1 for broilers. Studies using comparable application rates have found that 10Mgha−1 of dry poultry manure resulted in higher N uptake by barley than inorganic N fertilizer alone at comparable rates of inorganic N applicationReference Rees, Yan and Ferguson44; a litter application rate of 3.7Mgha−1 produced lint yields equivalent to maximum achievable with inorganic fertilizer, while 8.7Mgha−1 maximized lint yieldReference Tewolde, Adeli, Sistani, Rowe and Johnson12; and litter application for cotton at a rate of 6.7Mgha−1 maximized plant N utilization, while at rates of 10.1 and 13.4Mgha−1, bulk density was improved relative to inorganic N treated soilsReference Adeli, Tewolde, Sistani and Rowe1.
Conclusions and future research
Innovative sources of soil fertility enhancement are of utmost importance to growers in light of rising fertilizer costs and environmental concerns. This study demonstrates the utility of pastured poultry as a technique for soil fertility enhancement on intensively managed organic farms. We found increased levels of soil total C, total N, NH4+–N, NO3−–N, ex. K, organic matter and cation exchange capacity in PPAS plots. These soil fertility changes conferred greater biomass and height to sunflowers and beans grown in these soils. This management practice is not without drawbacks, however, and future research is necessary to mitigate nutrient management issues.
Particular attention is needed regarding soil N and P dynamics. This study suggests that 7 years of PPAS leads to excessively high Olsen P levels (Farm B). Research should be directed at (1) determining the amount of time birds should be withheld from a field before reintroducing a pasture rotation; and/or (2) a stocking density that optimizes soil P levels. At optimal P levels, N levels will likely be inadequate. To add more N, leguminous winter cover crops could be used. N-scavenging cover crops could also be used to minimize N loss and to recycle inorganic N. Radish (Raphanus sativus), mustard (Brassica hirta and Brassica alba), phacelia (Phacelia tanacetifolia), rye (Secale cereale) and annual ryegrass (Lolium multiflorum) are all known nitrogen scavengersReference Jackson, Wyland and Stivers45, Reference Wyland, Jackson, Chaney, Klonsky, Koike and Kimple46 that could function to recycle N from poultry manure. Finally, altering supplements added to poultry feed may impact water-soluble soil P levels and is an area of ongoing researchReference Vadas, Meisinger, Sikora, McMurtry and Sefton47. Movement of birds within a field also needs to be researched in order to optimize nutrient management. Hot spots can be addressed by determining how long a coop can remain in one location before leading to excessive nutrient accumulations.
Investigations into nutrient management and hot spots are imminent needs of future research that will directly impact management. Additional areas of research will serve to elucidate mechanism, adding to a theoretical understanding of integrated crop/livestock systems, and potentially influencing management. This research does not distinguish between the roles of manure and pasture plants in determining soil fertility changes. Although poultry manure undoubtedly determines certain soil-quality changes in pastured poultry/crop systems, pasture plants are also likely to play a role. Maintaining a field with a pasture mix of grass and legumes is functionally similar to cultivating a cover cropReference Clark48, and the effects of cover crops on nutrient cycling, organic matter and physical soil quality are well knownReference Watson, Atkinson, Gosling, Jackson and Rayns2, Reference Cherr, Scholberg and McSorley49. Additionally, pasture is tilled less frequently than crop fields, potentially leading to decreased erosion and higher carbon storageReference Cannell and Hawes50, Reference Fornara and Tilman51. In order to develop a mechanistic understanding of the relative contribution of manure versus pasture plants on soil quality, further research utilizing chicken exclosures is necessary.
Integrating pastured livestock with crop production is an emerging phenomenon on the Central Coast of California. Our study demonstrates that integrated pastured poultry systems appear to be an effective practice for managing soil fertility and crop growth. When managed with care, integrated pastured poultry/crop agroecosystems have the potential to contribute to the sustainable production of food via recycling of manure, bolstering of soil fertility and reduction of purchased fertility inputs.
Acknowledgements
We would like to express our deepest thanks to the farmers who contributed to developing the research objectives for this study and participated in its execution. We thank Erika Zavaleta for advising aspects of this research and for the field help from Scott Roberts, Devon Sampson, Blair McGlaughlin and Sara Pfeifer. This research was made possible through support from the following institutions: Western Sustainable Agriculture Research and Education Program (GW11-001); Center for Agroecology and Sustainable Food Systems; Annie's Sustainable Agriculture Scholarship; Organic Crop Improvement Association; David Gaines Award; Earthbound farm award; the Ruth and Alfred Heller Chair in Agroecology; and the department of Environmental Studies at the UCSC.