Introduction of Nitrogen Use Efficiency (NUE) in Conventional and Organic Agriculture
Nitrogen (N) fertilization is integral to the steady increase in global cereal production since the Green Revolution. The global demand for N fertilizer was 115 million metric tons (MMT) in 2015, and the fertilizer use is projected to increase to 236 MMT by 2050 to meet global demands (Pathak et al., Reference Pathak, Lochab, Raghuram and Moo-Young2011; Food and Agriculture Organization of the United Nations [FAO], 2015). Despite increased application of N fertilizer, a large amount is lost and/or unavailable to crop plants in most current agricultural systems. The estimated N recovery in crops during the first year of fertilizer application ranges from 25 to 50%. Applied N losses include loss into ground water, gaseous loss through volatilization and denitrification, immobilization within the soil system, or limits of plant uptake capacity (Fig. 1) (Robertson, Reference Robertson and Jackson1997; Raun and Johnson, Reference Raun and Johnson1999; Pathak et al., Reference Pathak, Lochab, Raghuram and Moo-Young2011). These N losses into the environment result in serious problems such as water pollution, increased greenhouse gas emissions, altered global N cycles and consequent vegetative alteration (Frink et al., Reference Frink, Waggoner and Ausubel1999; Horrigan et al., Reference Horrigan, Lawrence and Walker2002). Also, the production of synthetic N fertilizer requires a significant amount of non-renewable natural gas (Vance, Reference Vance2001). Transportation and application of the synthetic fertilizer add extra non-renewable energy consumption. Additionally, the use of synthetic fertilizer on the soil over a longer period of time may affect its ability to sustain healthy plant growth and crop production (Lim et al., Reference Lim, Wu, Lim and Shak2015). Therefore, our continuing overdependence on synthetic N for cereal production is not sustainable.
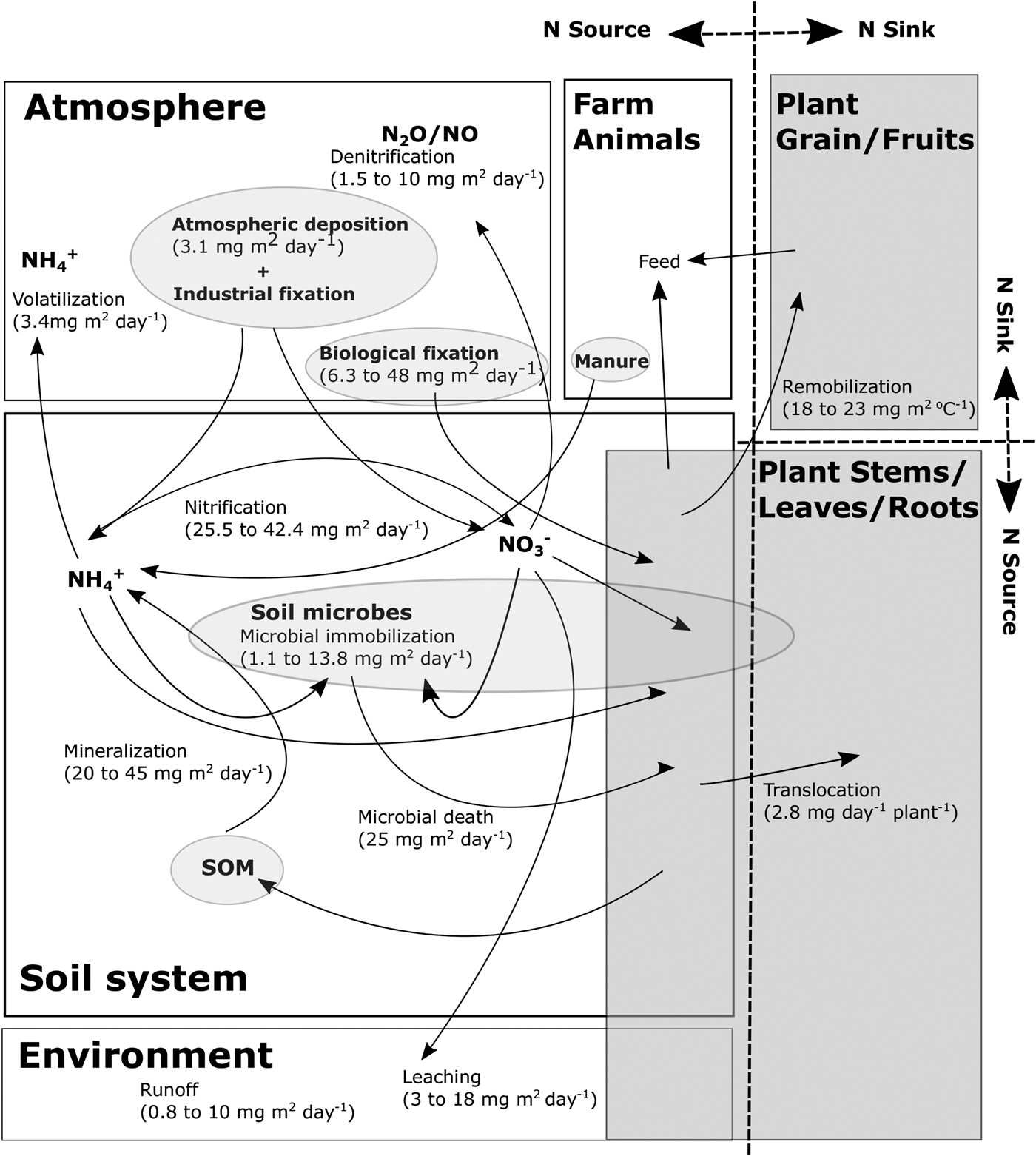
Figure 1. Schematic diagram of nitrogen dynamics in agroecosystems. An example of the amount of nitrogen dynamics in each nitrogen reserve is indicated in parentheses. Boxes with white color indicate environment, whereas dark gray ones refer to plant. Example values are obtained from previous studies (Simpson et al., Reference Simpson, Lambers and Dalling1982; Wu et al., Reference Wu, Bernardo, Mapp, Geleta, Teague, Watkins, Sabbagh, Elliott and Stone1997; Cabrera et al., Reference Cabrera, Kissel and Vigil2005; Gooding et al., Reference Gooding, Gregory, Ford and Pepler2005; Herridge et al., Reference Herridge, Peoples and Boddey2008; Dijkstra et al., Reference Dijkstra, Bader, Johnson and Cheng2009; Zhao et al., Reference Zhao, Hörmann, Fohrer, Li, Gao and Tian2011).
Accordingly, there is a growing interest in improving nitrogen use efficiency (NUE) in modern agriculture to reduce associated problems without adversely affecting grain yield. Increased NUE would also benefit primary producers as N fertilization is one of the major costs in crop production, especially in developing countries (Masclaux-Daubresse et al., Reference Masclaux-Daubresse, Daniel-Vedele, Dechorgnat, Chardon, Gaufichon and Suzuki2010). According to an estimate, a 1% improvement in cereal NUE could save more than US$200 million in N fertilizer costs globally (Raun and Johnson, Reference Raun and Johnson1999). Organic agriculture has become a major food production system over the last few decades partly because of the perceived environmental and health concerns related to high-input conventional agricultural practices. Organic agriculture is ‘a production system that sustains the health of soils, ecosystems and people. It relies on ecological processes, biodiversity and cycles adapted to local conditions, rather than the use of inputs with adverse effects’ (International Federation of Organic Agriculture Movement [IFOAM], 2008). Therefore, organic systems primarily rely on nutrition supplied from natural agroecosystems, which are highly variable N supply capability and dynamic. Organic soils often have low N levels, despite high N retention capability on a long-term basis due to higher soil organic matter (SOM) and soil microbial diversity, than soils in conventional systems (Mäder et al., Reference Mäder, Fliessbach, Dubois, Gunst, Fried and Niggli2002; Pimentel et al., Reference Pimentel, Hepperly, Hanson, Douds and Seidel2005).
One of the overall goals of NUE improvement in grain production is to increase grain yield per unit of N in soil. Variation of grain yield associated with NUE may be altered through genotypic selection (Van Sanford and MacKown, Reference Van Sanford and Mackown1986; Huggins and Pan, Reference Huggins and Pan1993; Sinebo et al., Reference Sinebo, Gretzmacher and Edelbauer2004; Muurinen et al., Reference Muurinen, Kleemola and Peltonen-Sainio2007), agricultural management (Spargo et al., Reference Spargo, Alley, Follett and Wallace2008; Khalil et al., Reference Khalil, Buegger, Schraml, Gutser, Richards and Schmidhalter2009a; Sainju et al., Reference Sainju, Caesar-Tonthat, Lenssen, Evans and Kolberg2009), rates, kinds and timing of N applied (Ortiz-Monasterio et al., Reference Ortiz-Monasterio, Sayre, Rajaram and Mcmahon1997), climate conditions (Sinebo et al., Reference Sinebo, Gretzmacher and Edelbauer2004; Muurinen et al., Reference Muurinen, Kleemola and Peltonen-Sainio2007) and soil properties (Meisinger et al., Reference Meisinger, Bandel, Stanford and Legg1985; Spargo et al., Reference Spargo, Alley, Follett and Wallace2008). Some studies suggest that NUE can be improved to obtain higher grain yield in both conventional and organic systems. Several reviews on different aspects of NUE have been published (Raun and Johnson, Reference Raun and Johnson1999; Bassirirad, Reference Bassirirad2006; Dawson et al., Reference Dawson, Huggins and Jones2008; Pathak et al., Reference Pathak, Lochab, Raghuram and Moo-Young2011).
Despite the significant contribution of NUE to crop productivity and the environment, understanding of the detailed mechanisms to improve NUE is relatively limited due to inherent complexity (Basra and Goyal, Reference Basra and Goyal2002). Especially, there is limited knowledge on the comparison of various aspects of NUE in conventional and organic production systems. Therefore, the purpose of this review is to present an overall examination of genetic, physiological and agronomical aspects of NUE and related traits under conventional and organic systems. We also propose a possible integrated approach of breeding and agronomic practices to improve NUE in both systems.
Definition and Concept of NUE
The N cycle in agricultural systems is affected by many factors such as soil N concentration, soil chemical, physical and biological properties, crop species and climatic conditions. The manipulation of N movement between N sinks and sources within a plant and an entire ecosystem is a critical component of NUE improvement. Moll et al. (Reference Moll, Kamprath and Jackson1982) defined the basic concept of NUE as the plant's ability to take up N efficiently from the soil and to partition absorbed N for grain production. Thus, NUE is expressed as the ratio of grain dry matter (Gw) to all N supplied from all available N sources (Ns) (Moll et al., Reference Moll, Kamprath and Jackson1982). Applied N fertilizer is often used as Ns because of the difficulties associated with measuring plant available N from mineralization through soil microbial activities. The amount of N loss is also often not accounted for in the calculation. The notion of N source and sink is interchangeable depending on research areas on NUE improvement. For instance, considering soil systems as N sources and crops as N sinks negates the non-linear reality that crops eventually become N sources, etcetera. In addition, a soil system itself could simultaneously become both sink and source; soil microbial immobilization as N sink and inorganic N release from SOM as N source (Havlin et al., Reference Havlin, Tisdale, Nelson and Beaton2014). Nitrogen exchange between N sink and source in the individual plant also exists in N metabolic processes according to plant growth (Fig. 1).
Owing to the involvement of complex traits in the concept of NUE, several parameters, which support the explanation of NUE, have been proposed (Table 1). First, NUE has been further divided into two primary components to illustrate the plant's ability to absorb available soil N and to partition N (Moll et al., Reference Moll, Kamprath and Jackson1982). Nitrogen uptake efficiency (NupE) reflects a crop's ability to absorb N and is defined as the ratio of total aboveground N (Nt) in the plant at maturity to supplied N (Ns) during the growing season. Nitrogen utilization efficiency (NutE) is an index of the N partitioning ability in the plant, defined as the ratio of grain yield (weight) (Gw) to total N (Nt) in the plant (Moll et al., Reference Moll, Kamprath and Jackson1982). For these parameters, the N sink is the entire plant for NupE and grain for NutE, whereas the N source is all plant available N, including applied fertilizer and SOM degradation for both NupE and NutE.
Table 1. Common parameters used for describing nitrogen (N) use efficiency.
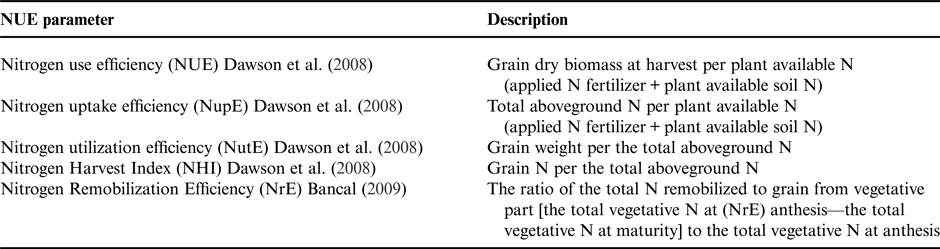
Variation in NupE is caused by genetic, soil, climatic and several agronomic factors (e.g. tillage, irrigation, timing and placement of N fertilizer) and their interaction (Huggins and Pan, Reference Huggins and Pan2003; Sinebo et al., Reference Sinebo, Gretzmacher and Edelbauer2004). Huggins and Pan (Reference Huggins and Pan1993) reported the importance of applied soil N levels to NupE. For instance, the contribution of applied N to grain N yield became less as the amount of the applied N increased, even though NupE increased with increased N application. Huggins and Pan (Reference Huggins and Pan2003) reviewed results of several previous studies and concluded that the decreased contribution of increased N use to NupE was unsynchronized crop N demand and N supply of N fertilizer and N mineralization. In other words, when N is applied over the soil storing capacity, N is lost from the system unless N demand of crop is high and synchronized with crop N demand to utilize an optimum quantity of applied N.
The NutE is another important parameter describing NUE. In a broader sense, NutE explains the plants’ ability to produce grain per unit of the total N in a plant. Optimum NutE is ideal for cereal crops grown in low fertility where plant-available N is limited. Several studies report that the contribution of NutE to overall NUE is not well understood and may not be as significant as NupE in wheat, barley, oats and maize (Presterl et al., Reference Presterl, Groh, Landbeck, Seitz, Schmidt and Geiger2002; Sinebo et al., Reference Sinebo, Gretzmacher and Edelbauer2004; Muurinen et al., Reference Muurinen, Slafer and Peltonen-Sainio2006; Sylvester-Bradley and Kindred, Reference Sylvester-Bradley and Kindred2009). In general, a crop species or cultivar has a unique N optimum utilization threshold, and crop internal N and grain yield do not increase in direct proportion to applied N (Sylvester-Bradley and Kindred, Reference Sylvester-Bradley and Kindred2009). In addition, quality traits such as grain protein are very important for wheat and barley producers. Increased NutE may lead to lower grain protein concentration due to N dilution effects when nitrogen harvest index (NHI: N in grain/N in total biomass) remains the same (Huggins and Pan, Reference Huggins and Pan2003). Isfan (Reference Isfan1993) suggested that increased NutE may be an important trait to consider when developing high NUE cultivars for limited N conditions typically found on organic farms. Greater NutE might be achieved through either improvement of N remobilization efficiency (NrE) or maintaining active photosynthesis (Masclaux-Daubresse et al., Reference Masclaux-Daubresse, Daniel-Vedele, Dechorgnat, Chardon, Gaufichon and Suzuki2010). Fertilization rates and regimes also affect wheat NrE, which is affected by specific enzymatic activity (Habash et al., Reference Habash, Massiah, Rong, Wallsgrove and Leigh2001; Miflin and Habash, Reference Miflin and Habash2002; Forde and Lea, Reference Forde and Lea2007; Bancal, Reference Bancal2009). Therefore, the effects of fertilizer managements on NutE cannot be ignored. Similar to NutE, NHI represents the translocation efficiency of acquired N for grain protein accumulation (Dawson et al., Reference Dawson, Huggins and Jones2008). This parameter is of particular importance in choosing parental cultivars for wheat breeding because the protein content in wheat grain directly affects grain price, and is of great importance to producers. High NHI levels indicate an efficient N utilization/translocation; thus cultivars with high NHI requires less N to produce similar yield as those with low NHI (Spiertz and De Vos, Reference Spiertz and De Vos1983).
Improvement of NUE for Mitigating Environmental Issues
The highly productive cereal production systems have negatively impacted environment through exploitation of non-renewable resources, ground fresh water pollution and eutrophication (Glibert et al., Reference Glibert, Harrison, Heil and Seitzinger2006). The relationship between yield and consumption of N fertilizer is non-linear (Sowers et al., Reference Sowers, Pan, Miller and Smith1994). Thus, the challenge in most of the agricultural systems is to find the means to improve efficiency of N fertilizer for sustainable food production. Mitigating the inefficiency of N fertilization has also a potential to slow down climate change through reducing emission of major greenhouse gases. Among these, nitrous oxide (N2O) emission from soil is mainly related to the excess amount of N fertilizer (Fig. 1); therefore, improved NUE in agriculture would reduce N2O emission. Liu et al. (Reference Liu, Cutforth, Chai and Gan2016) wrote a comprehensive review on the sustainable farming practices that could impede climate change through reducing carbon (C) footprints. In a study, direct and indirect N2O emission from cereal production was found to be 12 times higher in 2011 compared with 1970, while NUE decreased by approximately 74% in that period in Brazil (Amanullah et al., Reference Amanullah, Iqbal, Ali, Fahad and Parmar2016). The authors attributed these results to increased consumption of N fertilizer and predicted that an increase in NUE by 2.4% from current figure would reduce use of N fertilizer worth US$21 million, resulting in reduced N2O emission (Amanullah et al., Reference Amanullah, Iqbal, Ali, Fahad and Parmar2016). A meta-analysis of 19 published studies indicated that N2O emission per unit of harvested product, called yield-scaled N2O emission, reduced 12.7–7.1 g N2O-N kg−1 N uptake with an increase of NUE from 19 to 75% (Sowers et al., Reference Sowers, Pan, Miller and Smith1994). It is challenging to breed genotypes with 50% greater NUE than current genotypes in a short time; however, it may be realistic to improve crop NUE up to 50% with minimizing yield loss as NUE is largely controlled by phenotypic variations (Table 2).
Table 2. Examples of nitrogen use efficiency (NUE)1, uptake (NupE) and utilization efficiency (NutE) in cereals.

1 These NUE values are obtained from only studies in which NUE was calculated as Gw/Na (N applied + /or initial soil N).
2 The values are obtained as the ratio between the total aboveground DM and N in the DM.
Inorganic fertilizer or manure application is prone to leaching or runoff, resulting in the environmental issues, when not properly applied under water and tillage managements. In a semi-arid irrigation practice, increasing N fertilization over 150 kg ha−1 did not increase maize yield due to reduction in NUE, and increased the risk of N leaching under irrigation (Gholamhoseini et al., Reference Gholamhoseini, Aghaalikhani, Sanavy and Mirlatifi2013). All kinds of N inputs are vulnerable to loss in the environment when not incorporated into the soil (Eghball and Gilley, Reference Eghball and Gilley1999).
NUE in Conventional and Organic Agriculture
Physiology and genetics of NUE
To overcome the complex and quantitative manner of endogenous and exogenous mechanisms in NUE-related traits, understanding the genetic basis of N metabolism is essential for improving NUE. All components involved in NUE-related gene expression and metabolic pathways, controlling N uptake, utilization, partitioning and recycling are the subject of physiology, genetic and molecular studies. Most grain crops store a large pool of absorbed N during the vegetative stage and recycle pooled N during the reproductive stage when post-anthesis N uptake declines. In wheat, 50–90% of N in grain is derived from pooled N in stems and leaves (Spiertz and De Vos, Reference Spiertz and De Vos1983; Kichey et al., Reference Kichey, Hirel, Heumez, Dubois and Le Gouis2007). Therefore, systems in the pathways of N assimilation, remobilization and re-assimilation must be regulated according to external and internal N status to improve NUE. These metabolic pathways are controlled by complex genomic and consequent physiological steps in association with environmental factors that result in phenotypic characteristics. While N availability is mostly determined by environmental conditions and agronomic practices, the quantity of N intake into the root cytoplasm is regulated by passive and active uptake mechanisms controlled by several plant genes (Schenk, Reference Schenk1996; Tinker and Nye, Reference Tinker and Nye2000). Passive absorption usually refers to mass flow and diffusion, occurring along transpiration and energy gradients, respectively (Havlin et al., Reference Havlin, Tisdale, Nelson and Beaton2014). Active uptake occurs against concentration gradients through energy-required enzymatically driven actions. Thus, attempts to improve NupE through modifying root kinetics have been proposed (Bassirirad, Reference Bassirirad2006). The nature of uptake kinetics is mediated by genetically regulated active membrane transporters. Nitrate transporters (NRT) from the NRF and NRT2 families, and ammonium transporters have been identified for absorption and translocation of nitrate and ammonium (Dechorgnat et al., Reference Dechorgnat, Nguyen, Armengaud, Jossier, Diatloff, Filleur and Daniel-Vedele2011; Nacry et al., Reference Nacry, Bouguyon and Gojon2013; Léran et al., Reference Léran, Varala, Boyer, Chiurazzi, Crawford, Daniel-Vedele, David, Dickstein, Fernandez and Forde2014). Plants selectively use these transporter systems to absorb nitrate and ammonium, depending on their concentration in soil solutions. A high-affinity transporter system (HATS) works when nitrate and ammonium concentrations are lower than 1 mM, while a low-affinity transporter system (LATS) becomes predominant when concentrations are higher than 1 mM (Dechorgnat et al., Reference Dechorgnat, Nguyen, Armengaud, Jossier, Diatloff, Filleur and Daniel-Vedele2011; Nacry et al., Reference Nacry, Bouguyon and Gojon2013). Extensive studies have been conducted to characterize genes involved in these transporter systems as well as the functions of those genes (Vidmar et al., Reference Vidmar, Zhuo, Siddiqi, Schjoerring, Touraine and Glass2000; Orsel et al., Reference Orsel, Filleur, Fraisier and Daniel-Vedele2002; Plett et al., Reference Plett, Toubia, Garnett, Tester, Kaiser and Baumann2010; Léran et al., Reference Léran, Varala, Boyer, Chiurazzi, Crawford, Daniel-Vedele, David, Dickstein, Fernandez and Forde2014). Exploring functional mechanism of gene families NRF and NRT2, which are responsible for the activities of LATS and HATS, respectively, is the first step toward improving N uptake and specifically nitrate uptake in plants. The HATS system is further divided into constitutive HATS (cHATS) and inducible HATS (iHATS) (Behl et al., Reference Behl, Tischner and Raschke1988; Aslam et al., Reference Aslam, Travis and Huffaker1992). Attempts to increase N uptake through overexpressing these gene families resulted in improved nitrate influx under some conditions, but failed to improve NUE in Arabidopsis thaliana and tobacco plants (Fraisier et al., Reference Fraisier, Gojon, Tillard and Daniel-Vedele2000; Pathak et al., Reference Pathak, Ahmad, Lochab and Raghuram2008). This may suggest that improvement of NUE is not always source limited and improved N uptake can be successful to improve NUE when polygenetic functions involved in N metabolism pathways are taken into account for NUE enforcement.
During the vegetative stage, a large pool of absorbed nitrate is assimilated into roots and shoots. During this process of assimilation, nitrate is firstly reduced to nitrite in the cytoplasm by an enzyme called nitrate reductase (NR), and then further reduced to ammonium by nitrite reductase (NiR) in the chloroplast (Dechorgnat et al., Reference Dechorgnat, Nguyen, Armengaud, Jossier, Diatloff, Filleur and Daniel-Vedele2011). The ammonium produced by NiR and ammonium from soil solutions and photorespiration is finally transported through phloem sap in the phloem and stored in each part of the plant's body as different forms of amino acids. The amount and the place for storing these amino acids depend on the plant species and the amount of available nitrate in soil solutions (Christophe et al., Reference Christophe, Jean-Christophe, Annabelle, Alain, Marion and Anne-Sophie2011). Assimilated N is stored as some form of amino acid or protein in plant organelles, which later becomes a source for N remobilization. Because NR is the first enzyme involved in N assimilation where nitrate is the N source, it was thought that the variability of NR activity is rate limiting and a key to increasing NUE in maize and growth of tobacco (Sherrard et al., Reference Sherrard, Lambert, Below, Dunand, Messmer, Willman, Winkels and Hageman1986; Quilleré et al., Reference Quilleré, Dufossé, Roux, Foyer, Caboche and Morot-Gaudry1994; Hirel et al., Reference Hirel, Bertin, Quilleré, Bourdoncle, Attagnant, Dellay, Gouy, Cadiou, Retailliau and Falque2001; Masclaux et al., Reference Masclaux, Quilleré, Gallais and Hirel2001). In maize, a high variability of NR activity was observed and was used to increase grain yield through traditional breeding techniques (Sherrard et al., Reference Sherrard, Lambert, Below, Dunand, Messmer, Willman, Winkels and Hageman1986). In transformed Nicotiana plumbaginifolia, which overexpresses NR, NR activity in leaves increased 25–150% than that of the wild-type, while there was no difference in total N protein and dry matter production between the two types (Quilleré et al., Reference Quilleré, Dufossé, Roux, Foyer, Caboche and Morot-Gaudry1994). Since NR is just responsible for the first step of N, increased expression of NR genes in plants with little uptake or storage pool of nitrate may not be an ideal strategy. The enzymes involved in the further assimilation process need to be considered to improve nitrate assimilation.
The second candidate enzyme in the process of nitrate assimilation is glutamine synthetase (GS) (Andrews et al., Reference Andrews, Lea, Raven and Lindsey2004). Ammonium derived from nitrite reduction and photorespiration is finally synthesized into glutamine by GS. Together with 2-oxoglutarate, glutamine is further catalyzed by the glutamine 2-oxoglutarate aminotransferase (GOGAT) to form glutamate in the plastid and cytoplasm (Masclaux-Daubresse et al., Reference Masclaux-Daubresse, Daniel-Vedele, Dechorgnat, Chardon, Gaufichon and Suzuki2010). In major cereal crops, assimilation and remobilisation of N have been widely studied in relation to GS activity (Miflin and Habash, Reference Miflin and Habash2002; Masclaux-Daubresse et al., Reference Masclaux-Daubresse, Daniel-Vedele, Dechorgnat, Chardon, Gaufichon and Suzuki2010; Bao et al., Reference Bao, Zhao, Ding, Shi, Xu and Cai2015). Since the failure of improving crop yield through single enzyme overexpression of NR/NiR (Quilleré et al., Reference Quilleré, Dufossé, Roux, Foyer, Caboche and Morot-Gaudry1994; Hirel et al., Reference Hirel, Bertin, Quilleré, Bourdoncle, Attagnant, Dellay, Gouy, Cadiou, Retailliau and Falque2001; Masclaux et al., Reference Masclaux, Quilleré, Gallais and Hirel2001), GS has been studied to understand the entire enzymatic involvement for improving NUE in cereal crops. Together with glutamate synthetase, GS forms a GS–GOGAT cycle that is responsible for the central role of ammonium assimilation and remobilization from vegetative parts to developing grain in cereal crops (Miflin and Lea, Reference Miflin and Lea1977; Miflin and Habash, Reference Miflin and Habash2002). Multiple isoforms of GS exist in plants. The number of genes encoding cytosolic GS isoforms varies in wheat (TaGS1a, TaGS1b and TaGS1c for GS1;1, TAgsR1 and TaGSr2 for GS1;2 or GSr, TaGSe1, and TaGSe2 for GS1;3 or GSe) (Bernard et al., Reference Bernard, Møller, Dionisio, Kichey, Jahn, Dubois, Baudo, Lopes, Tercé-Laforgue and Foyer2008), rice (OsGS1;1, OsGS1;2 and OsGS1;3) (Tabuchi et al., Reference Tabuchi, Sugiyama, Ishiyama, Inoue, Sato, Takahashi and Yamaya2005) and maize (Gln1-1, Gln1-2, Gln1-3, Gln1-4 and Gln1-5) (Martin et al., Reference Martin, Lee, Kichey, Gerentes, Zivy, Tatout, Dubois, Balliau, Valot and Davanture2006), while a gene encoding chloroplast GS has been found in wheat (TaGS2a, TaGS2b and TaGS2c for GS2) (Bernard et al., Reference Bernard, Møller, Dionisio, Kichey, Jahn, Dubois, Baudo, Lopes, Tercé-Laforgue and Foyer2008; Wang et al., Reference Wang, Wei, Shi, Ma and Theg2015), maize (Gln2) (Martin et al., Reference Martin, Lee, Kichey, Gerentes, Zivy, Tatout, Dubois, Balliau, Valot and Davanture2006) and rice (OsGS2) (Tabuchi et al., Reference Tabuchi, Sugiyama, Ishiyama, Inoue, Sato, Takahashi and Yamaya2005). Improvement of reverse genetic techniques and availability of mutant plants have led to our increased knowledge of the specific gene functions related to the GS isoform family (Martin et al., Reference Martin, Lee, Kichey, Gerentes, Zivy, Tatout, Dubois, Balliau, Valot and Davanture2006). In maize, double- and single-mutants of Gln1-3 and Gln1-4 reduced the expression of GS mRNA in leaves, kernel number per ear and kernel weight, respectively, while not exhibiting a significant reduction of vegetative biomass (Martin et al., Reference Martin, Lee, Kichey, Gerentes, Zivy, Tatout, Dubois, Balliau, Valot and Davanture2006). This implies that these two GS isoforms are specifically responsible for grain development but not vegetative growth (Martin et al., Reference Martin, Lee, Kichey, Gerentes, Zivy, Tatout, Dubois, Balliau, Valot and Davanture2006). The knockout mutant of OsGS1;1 showed a severe decline in the total growth of a rice stand and grain filling (Tabuchi et al., Reference Tabuchi, Sugiyama, Ishiyama, Inoue, Sato, Takahashi and Yamaya2005). Although the presence of other GS1 genes OsGS1;2 and OsGS1;3 in the mutant did not compliment this phenotypic result, re-introduction of OsGS1;1 cDNA in the mutant led to complementation of these phenotypes (Tabuchi et al., Reference Tabuchi, Sugiyama, Ishiyama, Inoue, Sato, Takahashi and Yamaya2005). Therefore OsGS1;1 could be a pivotal gene for the assimilation of ammonium and the translocation of glutamine to developing rice grains.
Nitrogen remobilization occurs in the process of senescence. During this process, photosynthetic cellular components (e.g. chloroplast) and enzymes (e.g. Rubisco) are mainly dismantled into amino acids, amides and ammonium (Hörtensteiner and Feller, Reference Hörtensteiner and Feller2002; Gooding et al., Reference Gooding, Gregory, Ford and Pepler2005). Degraded ammonium is reduced by GS into glutamine. Glutamine is the major amino acid exported through both phloem and xylem to developing grain in barley and wheat. Therefore, the GS gene family plays a critical role in remobilizing necessary N to grain development during leaf senescence in cereal crops (Habash et al., Reference Habash, Massiah, Rong, Wallsgrove and Leigh2001; Miflin and Habash, Reference Miflin and Habash2002; Forde and Lea, Reference Forde and Lea2007). At this growth stage, leaves become an N source for protein synthesis in grain while N uptake from roots gradually reduces (Masclaux-Daubresse et al., Reference Masclaux-Daubresse, Daniel-Vedele, Dechorgnat, Chardon, Gaufichon and Suzuki2010). Therefore, efficient N recycling in leaves influences N yield in final harvest products. A positive correlation between GS activity in leaves and grain protein content has been reported in both rice and wheat (Yang et al., Reference Yang, Xiang, Ren and Wang2005; Habash et al., Reference Habash, Bernard, Schondelmaier, Weyen and Quarrie2007). Therefore, cytosolic GS1 and chloroplast GS2 activities in the flag leaf are considered useful characteristics to evaluate when selecting potential parents for breeding (Obara et al., Reference Obara, Kajiura, Fukuta, Yano, Hayashi, Yamaya and Sato2001; Kichey et al., Reference Kichey, Hirel, Heumez, Dubois and Le Gouis2007; Fontaine et al., Reference Fontaine, Ravel, Pageau, Heumez, Dubois, Hirel and Le Gouis2009). These three studies suggest that enzymes involved in N assimilation pathways (i.e. NR, NiR, GS and GOGAT) are all coordinated, and a single approach will not efficiently enhance NUE and final yield. However, GS activity appears to play a pivotal role during leaf senescence contributing to increasing NUE (Jain et al., Reference Jain, Khetarpal, Das and Abrol2011).
Aspects of Management Systems
Strategies to reduce N losses in conventional agriculture
Nitrogen fertilization is the primary source of N leaching and atmospheric nitrogen oxides (NOx) emissions and is estimated to exceed 50% of the global NOx budget by 2025 (Yienger and Levy, Reference Yienger and Levy1995). Understanding the proper application of N fertilizer is, therefore, critical to reducing its environmental loading and to improve NUE in the system (Yienger and Levy, Reference Yienger and Levy1995). To achieve optimum results, a fertilization strategy, also called the 4Rs of N nutrient management or Best Management Practices (BMPs) for N fertilization, has been proposed (Griffith and Murphy, Reference Griffith and Murphy1991; Matson et al., Reference Matson, Naylor and Ortiz-Monasterio1998; Alva et al., Reference Alva, Fan, Qing, Rosen and Ren2011; Ruidisch et al., Reference Ruidisch, Bartsch, Kettering, Huwe and Frei2013). The BMPs is a combination of four different concepts of fertilization practices. The practices emphasize a fertilization regime with the right rate and the right timing from the right sources at the right place (Griffith and Murphy, Reference Griffith and Murphy1991; Alva et al., Reference Alva, Fan, Qing, Rosen and Ren2011). Nitrogen dynamics vary with production systems such as organic and conventional, or dry and humid environments. Thus, operation of BMPs requires substantial understanding of on-site N mineralization, immobilization, cycling and N requirement of growing crops (Dawson et al., Reference Dawson, Huggins and Jones2008). Recent studies demonstrate that precision agricultural technologies based on BMPs offer a promising approach to improve NUE (Khosla et al., Reference Khosla, Fleming, Delgado, Shaver and Westfall2002; Zebarth et al., Reference Zebarth, Drury, Tremblay and Cambouris2009; Ahrens et al., Reference Ahrens, Lobell, Ortiz-Monasterio, Li and Matson2010). A study at a site-specific N management indicated that the key for successful on-farm precision N management is the right choice and timing of N application while taking into account on-farm residual N (Ahrens et al., Reference Ahrens, Lobell, Ortiz-Monasterio, Li and Matson2010).
Selection and rate of N fertilizers, placement depth (Khalil et al., Reference Khalil, Buegger, Schraml, Gutser, Richards and Schmidhalter2009a) and timing of application (Malhi et al., Reference Malhi, Soon, Grant, Lemke and Lupwayi2010) all influence N losses from soil. Different forms of inorganic N fertilizer cause different rate of N loss (Table 3). Urea is one of the most widely applied N fertilizers accounting for 55% of global N fertilizer consumption due to minimum risk of explosion under storage conditions, easy handling and low cost (Glibert et al., Reference Glibert, Harrison, Heil and Seitzinger2006; International Fertilizer Industry Association [IFA], 2011). The share of urea consumption to total N consumption is higher in developing countries than in developed countries (IFA, 2011). Urea is also responsible for a greater amount of ammonia and N2O emissions than other N fertilizer because of its rapid and easily hydrolyzed nature after application (Khalil et al., Reference Khalil, Rosenani, Van Cleemput, Boeckx, Shamshuddin and Fauziah2002; San Francisco et al., Reference San Francisco, Urrutia, Martin, Peristeropoulos and Garcia-Mina2011). This characteristic causes a significant amount of ammonia to be volatilized and a percentage of nitrate from the ammonia to be denitrified. Application of urea over large physical areas also results in severe N loss.
Table 3. Estimation of nitrogen loss by volatilization for various N source and application methods [Cai et al. (Reference Cai, Chen, Ding, Pacholski, Fan and Zhu2002) and Havlin et al. (Reference Havlin, Tisdale, Nelson and Beaton2014)].

Nitrogen sources affect crop morphological root development differently. For instance, nitrate promotes elongation of lateral roots, whereas ammonium promotes initiation of lateral roots (Zhou et al., Reference Zhou, Cai, Guo and Gan2009; Lima et al., Reference Lima, Kojima, Takahashi and Von Wirén2010). Due to this differential response, plants can extend specific roots and efficiently explore different N sources, which selectively promote root development in a large volume of soil. Wang and Below (Reference Wang and Below1992) studied the effect of mixture of nitrate and ammonium fertilizers on morphology of two wheat cultivars. Although no causal relationship of treatment-induced differences was found, they observed a substantial increase in vegetative dry weight and the number of tillers of cultivars with the mixture of these fertilizers (i.e. 75/25, 50/50 and 25/75, NO3/NH4), compared with that of cultivars receiving only one form. The two wheat cultivars also responded differently to the different forms of N fertilizer for tiller development; one cultivar produced more tillers with nitrate, whereas the other produced more tillers with ammonium (Wang and Below, Reference Wang and Below1992). Similarly, yield, development of leaf area, chlorophyll and plant height differed with N sources in maize (Abbasi et al., Reference Abbasi, Tahir and Rahim2013; Amanullah et al., Reference Amanullah, Iqbal, Ali, Fahad and Parmar2016). Yield and the growth response of these traits were greater with application of either calcium ammonium nitrate or ammonium sulfate than with urea. These studies indicate that crops and N sources interact to cause various growth responses, thus further studies are needed to take full advantage of choice of N fertilizers for achieving better NUE.
Critical periods of N demand vary in crops and adjusting fertilization timing according to crop N requirements may reduce the risk of N loss and excessive N fertilizer application (Fageria and Baligar, Reference Fageria and Baligar2005). For instance, the critical timing of N demand in spring wheat is between double ridge to terminal spikelet phases (between 20 and 45 days after seeding) (Limon-Ortega and Villaseñor-Mir, Reference Limon-Ortega and Villaseñor-Mir2006), while in maize it ranges from 35 to 45 days after seeding (Richie et al., Reference Richie, Hanway and Benson1986). In malting barley, adjusting the amount of split-N application is necessary to ensure good malting quality (Baethgen et al., Reference Baethgen, Christianson and Lamothe1995). López-Bellido et al. (Reference López-Bellido, López-Bellido and Redondo2005) reported that recovery of split-15N fertilizer application in wheat was, on average, 55% in three different N rates when fertilizer was applied as topdressing at terminal spikelet and stem-elongation stages; compared with 14% recovery for one-time application at pre-sowing. Under a winter wheat cultivation system, the plant recovery of 15N-labeled N at booting was greater when applied with split-N fertilization than with one-time fall application (57.4 and 46%, respectively) (Sowers et al., Reference Sowers, Pan, Miller and Smith1994). In many cereal production areas, N application practices are based on soil N analysis at seeding. Although one-time application of all required N at seeding is a common and relatively effective practice for winter wheat production in drier areas (McKenzie et al., Reference Mckenzie, Middleton, Pfiffner and Bremer2010), several studies indicated that split-application of N fertilizer (e.g. at seeding, tillering and stem elongation) improved NUE, the total N yield and grain yield in various growing conditions (Spiertz and De Vos, Reference Spiertz and De Vos1983; Limaux et al., Reference Limaux, Recous, Meynard and Guckert1999; López-Bellido et al., Reference López-Bellido, López-Bellido and Redondo2005). These results clearly show that the extent of plant N uptake is associated with N demands at different plant growth stages where N is used for cell division and growth, and thus the timing of fertilizer application needs to be synchronized with the timing of crop N requirement.
Besides split-fertilization, application of polymer coated N fertilizers is another useful strategy to synchronize N supply and demand, and reduce N losses (Yanai et al., Reference Yanai, Kosaki, Nakano and Kyuma1997; Khalil et al., Reference Khalil, Gutser and Schmidhalter2009b; Malhi et al., Reference Malhi, Soon, Grant, Lemke and Lupwayi2010). These fertilizers are covered with different types of polymer coats such as controlled-release compounds or urease and nitrification inhibitors (Havlin et al., Reference Havlin, Tisdale, Nelson and Beaton2014). Several studies reported the positive effects of polymer-coated controlled-release N (CRN) on grain yield, NUE (McKenzie et al., Reference Mckenzie, Bremer, Middleton, Pfiffner and Dowbenko2007; Malhi et al., Reference Malhi, Soon, Grant, Lemke and Lupwayi2010) and N leaching (Yanai et al., Reference Yanai, Kosaki, Nakano and Kyuma1997). The release timing of CRN is designed to synchronize N requirement in the plant (Malhi et al., Reference Malhi, Soon, Grant, Lemke and Lupwayi2010), thereby minimizing the risk of N leaching under normal conditions. For instance, urea is nitrified within 2 weeks under favorable conditions, whereas anhydrous ammonia is converted to nitrate slower than urea (Nielsen, Reference Nielsen2006). The critical timing for N requirement in winter wheat is at the double ridge stage and from tillering to stem elongation, which occurs at the beginning of the spring. Therefore, regular fertilization at the time of seeding does not provide the appropriate amount of N at the proper time in winter wheat. Moreover, a considerable amount of N is lost to the environment during the winter. Therefore, the application of CRN at seeding is especially effective in winter wheat to prevent N loss under wet winter conditions, which tend to result in increased denitrification and N leaching (Malhi et al., Reference Malhi, Soon, Grant, Lemke and Lupwayi2010). Nevertheless, negative or negligible influence of CRN on NUE has also been reported in the literature (McKenzie et al., Reference Mckenzie, Middleton, Pfiffner and Bremer2010). Such inconsistent responses may be caused by year-to-year variation in weather (Malhi et al., Reference Malhi, Soon, Grant, Lemke and Lupwayi2010; McKenzie et al., Reference Mckenzie, Middleton, Pfiffner and Bremer2010).
Split-banded application of urea in spring wheat and barley (half each at seeding and tillering) and canola (at bolting stages) was as effective as the spring-banded application of CRN as a single dose (Malhi et al., Reference Malhi, Soon, Grant, Lemke and Lupwayi2010). Use of CRN to pots with and without crop reduced the concentration of nitrate in soil solutions at all sampling times, indicating that CRN reduces N leaching (Yanai et al., Reference Yanai, Kosaki, Nakano and Kyuma1997). These results suggest that CRN and split applications of fertilizer may have theoretically similar effects of reducing N loss and, therefore, improving NUE.
To mitigate soil enzymatic factors affected by environmental variation, the combination of urease and nitrification inhibitors has been tested (Gioacchini et al., Reference Gioacchini, Nastri, Marzadori, Giovannini, Antisari and Gessa2002; Boeckx et al., Reference Boeckx, Xu and Van Cleemput2005). These types of inhibitors are responsible for limiting the enzymatic activities of urea hydrolysis and ammonium nitrification. The use of urease inhibitors leads to increased urea content in the soil, and the use of a nitrification inhibitor increases soil ammonium content (San Francisco et al., Reference San Francisco, Urrutia, Martin, Peristeropoulos and Garcia-Mina2011). In general, nitrate is more readily lost in the environment than ammonium. Usage of several urease and nitrification inhibitors resulted in some suppression of N losses (Weber et al., Reference Weber, Gutser, Michel, Wozniak, Chen, Xu, Niclas and Weiske2004). In general, these inhibitors are more effective in reducing N loss when the fertilizers along with inhibitors are applied, under conditions that are prone to volatilization, denitrification and N leaching (Barth et al., Reference Barth, Von Tucher and Schmidhalter2001). Therefore, results depend on soil and environmental variation (Gioacchini et al., Reference Gioacchini, Nastri, Marzadori, Giovannini, Antisari and Gessa2002; Boeckx et al., Reference Boeckx, Xu and Van Cleemput2005; Khalil et al., Reference Khalil, Gutser and Schmidhalter2009b; San Francisco et al., Reference San Francisco, Urrutia, Martin, Peristeropoulos and Garcia-Mina2011).
In addition to fertilization regimes, the diversification of crops/cropping practices in crop rotation plays a significant role in improving NUE in conventional systems. Diversified crop rotations with leguminous crops take biologically fixed N into the N cycle in situ, resulting in the reduction of N fertilizer use. When legume crops were included as preceding crops in a no-till crop rotation, N fertilizer accounted for 42–55% of the total N input in wheat and canola compared with 52–60% of total N input derived from N fertilizer when preceding crops were non-legumes (Luce et al., Reference Luce, Grant, Ziadi, Zebarth, O'donovan, Blackshaw, Harker, Johnson, Gan and Lafond2016). Nitrogen derived from the decomposition of preceding leguminous crops compensated for a portion of the N fertilizer input. When a large volume of N is required for cereal crops, selection of N-fixing crops as preceding crops could reduce N fertilizer application amount, minimizing the risk of N loss in the environment. When succeeding crops were fertilized with the recommended amount of N, increased yield of the following crops are greater than those following non-leguminous crops (Badaruddin and Meyer, Reference Badaruddin and Meyer1994; Yadav et al., Reference Yadav, Singh, Dwivedi and Shukla2003; O'Donovan et al., Reference O'donovan, Grant, Blackshaw, Harker, Johnson, Gan, Lafond, May, Turkington and Lupwayi2014; Luce et al., Reference Luce, Grant, Zebarth, Ziadi, O'donovan, Blackshaw, Harker, Johnson, Gan and Lafond2015). The amount and timing of available N in legumes depends on factors such as the C/N ratio, tillage practices, soil microbial diversity and climate. Greater care is needed when legumes are incorporated in rotations for reducing N fertilizer.
The inclusion of post-harvest cover crops in crop rotations has been successfully practised throughout the world. Cover crops maintain favorable soil conditions for improving NUE such as adding and conserving N, optimizing the C/N ratio of residues and preventing soil erosion (Dabney et al., Reference Dabney, Delgado, Meisinger, Schomberg, Liebig, Kaspar, Mitchell, Reeves, Delgado and Follett2010). In summer crop farming regions, the use of two winter cover crops affected yield and N uptake of the following maize crop (Gabriel et al., Reference Gabriel, Alonso-Ayuso, García-González, Hontoria and Quemada2016). Barley as a winter cover crop grew faster than vetch; however, vetch finally covered more ground than barley in winter, resulting in high N content in yield biomass (Gabriel et al., Reference Gabriel, Alonso-Ayuso, García-González, Hontoria and Quemada2016). Thus, barley may be used as a means of N conservation in this system as it minimizes the risk of post-harvest N loss and soil erosion. On the other hand, vetch has a greater N contribution to the following crop than barley. A meta-analysis of N dynamics between the diversified rotations and the conventional simple rotations with bare fallow demonstrated that legume-based crop rotations reduced nitrate leaching up to 40% relative to conventional fertilizer-based rotations, indicating that the replacement of bare fallow with legume cover crops provide dual benefits of conserving and adding N (Tonitto et al., Reference Tonitto, David and Drinkwater2006). The authors also reported that inclusion of cover crops prevented post-harvest N volatilization and denitrification. In the Northern Plain semiarid area, summer fallow used to be a common practice to store sufficient soil moisture for succeeding crop germination despite the risk of soil erosion and denitrification (Aulakh et al., Reference Aulakh, Rennie and Paul1982). Studies of crop diversification in rotations in semiarid regions reported that diversification with pulse/green manure in rotation had the additional benefit of increased water availability for succeeding crops (Gan et al., Reference Gan, Hamel, O'donovan, Cutforth, Zentner, Campbell, Niu and Poppy2015, Reference Gan, Mooleki, Lemke, Zentner and Ruan2016). Such a finding provides farmers an alternative practice to conserve soil moisture, replacing summer fallow. Although, long-term studies investigating the direct effect of diversified crop rotations on NUE are few, these studies suggest the importance of crop sequences and diversification of crops in rotations for potential improvement of NUE.
Strategies to Reduce N Loss in Organic Agriculture
Organic agriculture is based on using the existing ecological system and recycling processes. In the absence of synthetic N fertilizer, the primary approach for improving NUE in organic agriculture is to maintain existing residual N, potential N mineralization reserves in the soil and to recycle on-farm materials through green manure, and incorporating plant residues from previous crops (Spiertz and De Vos, Reference Spiertz and De Vos1983; Drinkwater, Reference Drinkwater, Mosier, Syers and Freney2004; IFOAM, 2008). Diverse knowledge, techniques and strategies are required to optimize the utilization of various N reservoirs. Sources of N are mainly derived from on-farm materials, and can be limited, especially in extensive organic farming systems. Therefore, proper management of these reserves in soils is a key management strategy to provide the necessary amount of N to crops. This can be achieved through a combination of crop rotation, incorporation of legume crops as a source of biologically fixed N in the rotation, tillage practices and use of catch crops (Lupwayi et al., Reference Lupwayi, Clayton, O'donovan, Harker, Turkington and Soon2006; Constantin et al., Reference Constantin, Mary, Laurent, Aubrion, Fontaine, Kerveillant and Beaudoin2010; Snyder and Spaner, Reference Snyder and Spaner2010; Doltra and Olesen, Reference Doltra and Olesen2013). Although these practices are also observed in conventional systems, the contribution of these practices toward NUE is greater in organic systems than in conventional systems (Huggins and Pan, Reference Huggins and Pan1993; Drinkwater, Reference Drinkwater, Mosier, Syers and Freney2004).
Organic practices tend to cause less N loss than conventional systems; a combined result attributed to inherent low concentrations of plant available inorganic N, the incorporation of legumes and catch crops and diverse crop rotations (Stopes et al., Reference Stopes, Lord, Philipps and Woodward2002; Syswerda et al., Reference Syswerda, Basso, Hamilton, Tausig and Robertson2012). For example, annual nitrate loss under organic management was 19 kg N ha−1 yr−1 compared with 62 kg N ha−1 yr−1 in conventional systems in one study (Syswerda et al., Reference Syswerda, Basso, Hamilton, Tausig and Robertson2012), and 36 kg N ha−1 yr−1 in organic systems versus 57 kg N ha−1 yr−1 in conventional systems in another (Stopes et al., Reference Stopes, Lord, Philipps and Woodward2002). It may, therefore, be more difficult to increase NUE in organic systems than in conventional.
This suggests that it is even more important to understand the mechanisms contributing to increased NUE in organic systems.
Crop rotation is one of the most important management practices for organic farmers to maintain soil fertility (López-Bellido and López-Bellido, Reference López-Bellido and López-Bellido2001; Eriksen et al., Reference Eriksen, Askegaard and Kristensen2004). In western Canada, the typical organic rotation includes legumes and pulses in a several-year rotation that appears to maintain soil N levels due to N fixation of these crops (Degenhardt et al., Reference Degenhardt, Martin and Spaner2005; Snyder and Spaner, Reference Snyder and Spaner2010). The choice of crops in rotation depends on weather, soil condition, plant adaptation and market demands but the inclusion of legumes is obviously beneficial to maintain soil N levels. The relationship between crop rotation and NUE/N loss has been extensively studied (Porter et al., Reference Porter, Follett and Halvorson1996; López-Bellido and López-Bellido, Reference López-Bellido and López-Bellido2001; Sainju et al., Reference Sainju, Caesar-Tonthat, Lenssen, Evans and Kolberg2009; Askegaard et al., Reference Askegaard, Olesen, Rasmussen and Kristensen2011).
Legumes and their associated N-fixing bacteria are critical components of N supply in organic crop rotation systems. Inclusion of leguminous crops provides atmospheric N to the host plants which eventually store N as SOM for long term. Several studies have reported improvement of soil N status, including potentially mineralizable N, total N and plant available N in legume-based rotation practices (Marriott and Wander, Reference Marriott and Wander2006; Kayser et al., Reference Kayser, Müller and Isselstein2010). Ammonia fixed through biological means such as legume and N-fixing bacteria provides an important portion of plant required N, partially obviating the need for industrial fertilizers. For instance, endophytic N-fixing bacteria can provide 10–25% of required N in maize and rice (Okon and Labandera-Gonzalez, Reference Okon and Labandera-Gonzalez1994; Mano and Morisaki, Reference Mano and Morisaki2008; Figueiredo et al., Reference Figueiredo, Seldin, De Araujo and Mariano2010; Amiri and Rafiee, Reference Amiri and Rafiee2013). Moreover, biologically fixed N is less susceptible to loss than synthetic N fertilizer on a short-term basis due to direct translocation of ammonia from bacteria to their host plants (Ondersteijn et al., Reference Ondersteijn, Beldman, Daatselaar, Giesen and Huirne2002). The fixed N is immediately converted into organic forms as amino acids, which assimilate into plant cells and become stable. A study reported that unfertilized wheat following a grain legume crop yields almost the same as wheat with 75 kg N ha−1 fertilization although grain N concentration varies according to weather conditions (Badaruddin and Meyer, Reference Badaruddin and Meyer1994). Wheat NUE, NupE and NutE were greater after cowpea compared with rice (Yadav et al., Reference Yadav, Singh, Dwivedi and Shukla2003). This was caused by the better root growth and crop establishment of wheat coupled with the increase of the soil organic C and available N in the cowpea–wheat rotation. These results demonstrated that including legumes in rotation ensures N availability to succeeding crops. Although availability in legume residual N in rotation is unpredictable and difficult to manage; plough-in legumes or other plant residues are eventually decomposed by soil microorganisms, and released N from these residues is prone to loss when N supply and crop demand are asynchronous (Crews and Peoples, Reference Crews and Peoples2005). A key for the rate of N mineralization in plowed legumes is the C/N ratio, weather and soil microbial conditions. Thus timing of plowing, which provides favorable conditions to decomposers, must be considered (Herridge et al., Reference Herridge, Peoples and Boddey2008; Kayser et al., Reference Kayser, Müller and Isselstein2010). Much of the N pool of grain legumes is removed during harvesting, whereas green manure or cover crop legumes are not grown for grain and inclusion of these non-grain legumes is a better choice for keeping all fixed N within the system. Planting legumes with overlapping life cycle of main crops, also called relay intercropping, is found to be an agronomically and ecologically feasible practice for improving NUE (Jeranyama et al., Reference Jeranyama, Hesterman and Sheaffer1998). Although competition between legumes and winter wheat for resources caused reduction of grain protein in wheat grain after relay intercropping, the yield of subsequent crops increased due to leguminous soil N enrichment (Amossé et al., Reference Amossé, Jeuffroy and David2013, Reference Amossé, Jeuffroy, Mary and David2014).
Tillage remains necessary in organic systems especially for weed control. Tillage provides necessary aeration and optimum moisture and is, therefore, very important to the microbial decomposition of SOM. However, tillage may cause severe N losses through N leaching or soil erosion (Kessavalou et al., Reference Kessavalou, Mosier, Doran, Drijber, Lyon and Heinemeyer1998; Sainju et al., Reference Sainju, Caesar-Tonthat, Lenssen, Evans and Kolberg2009). Due, in part, to N conservation awareness and increased fuel costs, no-till has been widely implemented in conventional systems to conserve N pools (Porter et al., Reference Porter, Follett and Halvorson1996; Spargo et al., Reference Spargo, Alley, Follett and Wallace2008). Organic matter derived from plant residues accumulates on the surface. Plant available N is immobilized into organic forms by diverse soil microorganisms around the surface soil under no-till managements (Doran et al., Reference Doran, Elliott and Paustian1998), whereas an increase in N loss through leaching, volatilization and denitrification was observed as the frequency and intensity of tillage increased (Sainju et al., Reference Sainju, Caesar-Tonthat, Lenssen, Evans and Kolberg2009). Another study noted that no-till maintained a larger amount of soil nitrate concentration than the conventional tillage system (Lupwayi et al., Reference Lupwayi, Clayton, O'donovan, Harker, Turkington and Soon2006). This may be because long-term conservation (reduced or no) tillage systems preserve habitat for soil microbial communities and promote accumulation of soil enzymes responsible for N dynamics. Since most soil enzymes are of microbial origin (Gianfreda et al., Reference Gianfreda, Rao, Mora, Huang, Li and Sumner2011), maintaining soil microbial communities in no-till systems has a great impact on soil enzyme activities, thus affecting N mineralization (Muruganandam et al., Reference Muruganandam, Israel and Robarge2009). Nitrate and ammonium produced through microbial mineralization may simultaneously immobilize due to the rapid turnover of microbial biomass under the high C/N ratio in soil (Muruganandam et al., Reference Muruganandam, Israel and Robarge2010). Immobilization of N caused by no-till is in balance with N mineralization; thus no-tillage practice may be more efficient from a sustainable standpoint (House et al., Reference House, Stinner, Crossley, Odum and Langdale1984; Muruganandam et al., Reference Muruganandam, Israel and Robarge2010). Franzluebbers (Reference Franzluebbers, Magdoff and Weil2004) estimated the amount of soil organic N in several tillage treatments in the previous studies and found that the total organic N in no-till managements is 23 kg N ha−1 yr−1 higher compared with plow tillage. However, the effect of no-till on limiting N losses is mostly controlled by soil and weather conditions (Hansen and Djurhuus, Reference Hansen and Djurhuus1997), and may be less effective in limiting N loss than catch crops or timing of tillage (Constantin et al., Reference Constantin, Mary, Laurent, Aubrion, Fontaine, Kerveillant and Beaudoin2010). Finding mechanisms to incorporate no or limited tillage systems into organic management strategies is needed.
Depending on soil fertilization regimes, the rate of N uptake during the crop growing season is generally higher than the rate of N mineralization in organic fields and the risk of N loss is low in most cases (Watkins and Barraclough, Reference Watkins and Barraclough1996; Masunga et al., Reference Masunga, Uzokwe, Mlay, Odeh, Singh, Buchan and De Neve2016). However, a high risk of N leaching could become a problem in bare fields after harvest, especially when the temperature in a fallow season is relatively high and the field is left uncropped (Stopes et al., Reference Stopes, Lord, Philipps and Woodward2002; Eriksen et al., Reference Eriksen, Askegaard and Kristensen2004). This leaching is due to a combination of increased activity of decomposers and the absence of crop to trap N. The use of a catch crop after the main crop harvest has a potential role in reducing the risk of N leaching during the off-season (i.e. fall and winter). Catch crops absorb N in the soil and store N, thus preventing N leaching. The stored N as SOM is eventually decomposed in the next crop season. A long-term study was conducted to evaluate the effect of cover crop on the reduction of soil N loss from the system (Constantin et al., Reference Constantin, Mary, Laurent, Aubrion, Fontaine, Kerveillant and Beaudoin2010). Using cover crops in the off-season resulted in a reduction of 36–62% of N loss from the system compared to no-cover crop in three different locations. However, the large variability in the reduction of N loss by cover crops in three different locations also indicated that other factors such as precipitation, soil types and crop rotation also affect the amount of N leaching (Constantin et al., Reference Constantin, Mary, Laurent, Aubrion, Fontaine, Kerveillant and Beaudoin2010). To test the effectiveness of cover crops in reducing N loss under different cropping practices, Lemaire and Meynard (Reference Lemaire, Meynard and Lemaire1997) investigated 6-year average annual N leaching loads among three cultivation systems. The conventional system received an appropriate amount of N fertilizer and two organic systems treated with and without animal manure. Mean N leaching loads between the three systems were not statistically significant when catch crops were grown. They concluded that the use of catch crop after the main crop had a potential role in reducing the risk of N leaching during off-season under different amount and quality of external inputs. Similar results were reported by Stopes et al. (Reference Stopes, Lord, Philipps and Woodward2002) . Catch crops absorb N in the soil and store N, thus preventing N leaching. Therefore, the ability to store a large quantity of N and the turnover rate of the stored N in catch crops, may be important subjects to consider for the selection of catch crops. These studies highlight the importance of cover crops for reduction of N loss between growing seasons for both organic and conventional cultivation systems.
One of the distinctive differences of organically managed soils from conventionally managed soils is the diversity and quantity of functional microbial population (Mäder et al., Reference Mäder, Fliessbach, Dubois, Gunst, Fried and Niggli2002; Pimentel et al., Reference Pimentel, Hepperly, Hanson, Douds and Seidel2005). Besides N-fixation, all other N-cycling processes are governed by the quality and quantity of substrate and microbial activity. Organic farming, which relies on ecosystem service, essentially has the potential to increase crop NUE through its cultivation practices (Veresoglou et al., Reference Veresoglou, Chen and Rillig2012). For instance, the rapidly available fraction of SOM, particulate organic matter (POM), is 30–40% higher in organic systems than in conventional system and functional soil microbial community that decompose the POM may be more active and diverse in species and abundance (Lavelle and Spain, Reference Lavelle and Spain2001; Marriott and Wander, Reference Marriott and Wander2006; Reilly et al., Reference Reilly, Cullen, Lola-Luz, Stone, Valverde, Gaffney, Brunton, Grant and Griffiths2013). Microbial contribution to plant N acquisition is estimated to be 0–20% or more through N-fixing bacteria including free-living bacteria, and 0–80% through mycorrhizal symbiosis (Van Der Heijden et al., Reference Van Der Heijden, Bardgett and Van Straalen2008). Ubiquitous soil symbiotic fungi, arbuscular mycorrhizal fungi (AMF) are able to transport N from soil to the host plant, although this does not appear to contribute to increasing plant biomass or total plant N content (Hawkins and George, Reference Hawkins and George1999; Hawkins et al., Reference Hawkins, Johansen and George2000). That study indicated that the hyphae of AMF uptake both inorganic (NO3− and NH4+) and organic (glycine and glutamic acid) N. The quantity of acquired N through AMF varied depending on AMF isolated and species, but the organically managed field had higher ability to uptake organic N than conventional (Hamilton III and Frank, Reference Hamilton Iii and Frank2001). A comprehensive review of the mechanism of N acquisition through AMF is elsewhere (Jin et al., Reference Jin, Liu, Liu and Huang2012; Veresoglou et al., Reference Veresoglou, Chen and Rillig2012). Recent studies suggest that the degree of N mineralization in mixed crop–livestock farming systems is partly controlled by C excretes from plant roots, which stimulate activities of the soil microbial community (De Nobili et al., Reference De Nobili, Contin, Mondini and Brookes2001; Hamilton III and Frank, Reference Hamilton Iii and Frank2001). Promoted C exudates by glazed grass stimulate microbial activity, resulting in increase of soil inorganic N as well as plant N uptake (Hamilton III and Frank, Reference Hamilton Iii and Frank2001).
Predicting N Recovery from Soil Organic Matter
Unpredictable rate and timing of mineralization of N source (i.e. green manure, farmyard manure, compost, residual SOM) also impede synchronization of N sink and source in both conventional and organic agriculture. Organic N materials tend to release a form of plant available N in various manners depending on the materials, environment and agronomical practices. Nitrogen recovery in crops from SOM varies due to environment and characteristics of SOM (Amlinger et al., Reference Amlinger, Götz, Dreher, Geszti and Weissteiner2003; Crews and Peoples, Reference Crews and Peoples2005). The recovery rates from different SOM are variable and are generally <30% of the total recovery at first year of SOM incorporation and several percent in subsequent years (Amlinger et al., Reference Amlinger, Götz, Dreher, Geszti and Weissteiner2003; Crews and Peoples, Reference Crews and Peoples2005). Nitrogen mineralization models from various SOM provides rough estimates of SOM mineralization and may help to optimize N sink/source synchrony in both systems. The details of several proposed models are reviewed by Benbi and Richter (Reference Benbi and Richter2002). Mineralization during crop growth has been estimated through non-linear equations using the separated or the total active SOM (Benbi and Richter, Reference Benbi and Richter2002). The common limitation of the N models under organic systems is that the active organic N pools are larger in size and variety than the inorganic pools, thus causing a significant error variance in the models (Tanji et al., Reference Tanji, Broadbent, Mehran and Fried1979; Camargo et al., Reference Camargo, Gianello and Vidor1997). However, meta-analyses of accumulated laboratory data have proven that exponential models are useful to predict N dynamics when site-specific calibration is possible. These models, however, do not take into account the mineralization/immobilization reverse cycles occurring simultaneously in various N pools (Benbi and Richter, Reference Benbi and Richter2002; Dijkstra et al., Reference Dijkstra, Bader, Johnson and Cheng2009).
Exponential models are based on mathematical fitting techniques to predict N mineralization as a function of time (Benbi and Richter, Reference Benbi and Richter2002). The models consider existing N pools as separated fractions of soil N pools and estimate the total N mineralization from each fraction with different mineralization rates. Benbi and Richter (Reference Benbi and Richter2002) suggested that multiple N fraction models provide better estimations of N mineralization. Therefore, the double-N-pool model (i.e. labile and recalcitrant SOM) would be the best to calculate parameters for N mineralization modeling compared with other N pool models (Benbi and Richter, Reference Benbi and Richter2002). By using data sets from several previous studies, Gilmour and Mauromoustakos (Reference Gilmour and Mauromoustakos2011) found that the rate of N mineralization of labile N pool and accumulated N from labile N pool are correlated with the rate of labile N mineralization during the first week. These labile N mineralization rate and accumulated N could be estimated using total soil N, CO2 release during first 3-day incubation, clay content in soils, soil temperature and moisture (Gilmour and Mauromoustakos, Reference Gilmour and Mauromoustakos2011). The environmental factors affecting N mineralization vary with the C/N ratio and biochemical quality of organic residue, soil moisture and temperature and the frequency of soil rewetting events (Cabrera et al., Reference Cabrera, Kissel and Vigil2005); therefore, calibration and validation of the models are necessary for reliable prediction (Cabrera et al., Reference Cabrera, Kissel and Vigil2005; Zhao et al., Reference Zhao, Hörmann, Fohrer, Li, Gao and Tian2011).
Breeding for Integration of Physiological and Agronomic Strategies to Improve NUE
Genetic improvement to maximize NUE for target conditions
Genetic improvement in NUE through breeding relies on the availability of genetic variation and heritability of the morphological and physiological traits associated with NUE. Genetic variability in N uptake and utilization has been reported in several grain crops (Table 2) including wheat (Ortiz-Monasterio et al., Reference Ortiz-Monasterio, Sayre, Rajaram and Mcmahon1997; Gaju et al., Reference Gaju, Allard, Martre, Snape, Heumez, Legouis, Moreau, Bogard, Griffiths and Orford2011; Swain et al., Reference Swain, Rempelos, Orr, Hall, Chapman, Almadni, Stockdale, Kidd, Leifert and Cooper2014), maize (Barbieri et al., Reference Barbieri, Echeverría, Saínz Rozas and Andrade2008; Kalinova et al., Reference Kalinova, Kostadinova and Hristoskov2014; Li et al., Reference Li, Chen, Cai, Liu, Pan, Liu, Gu, Mi, Zhang and Yuan2015), rice (Cassman et al., Reference Cassman, Gines, Dizon, Samson and Alcantara1996; Sun et al., Reference Sun, Ma, Sun, Xu, Yang, Liu, Jia and Zheng2012) and barley (Sinebo et al., Reference Sinebo, Gretzmacher and Edelbauer2004; Anbessa et al., Reference Anbessa, Juskiw, Good, Nyachiro and Helm2009). Existing variation in grain/total N concentration in different genotypes under the same amount of fertilization indicate the potential genotypic improvement of both NupE and NutE through breeding. Many of these studies did not focus on root traits. Roots are an interface where crops absorb N and are essential functional traits for synchrony of N supply and demand. Several positively correlated overlapping quantitative trait loci (QTLs) for root traits and N uptake have been reported (Table 4).
Table 4. Co-localized QTL for GS or root traits and NUE-related traits in three major cereals.

1 The numbers in parentheses indicate chromosome numbers.
2 Doubled haploid lines.
3 Glutamine synthetase.
4 Recombinant inbred lines.
5 Backcross inbred lines.
Functional root traits responsible for optimizing N uptake are key subjects to improve NUE. Since roots have multiple roles, improvement of a single trait in the root function is not likely to enhance N uptake and NUE (Lynch, Reference Lynch1995). Positive correlations among QTL for root traits, some physiological traits and N uptake in maize, wheat and rice have been reported (Gallais and Hirel, Reference Gallais and Hirel2004; An et al., Reference An, Su, Liu, Zhu, Tong, Li, Jing, Li and Li2006; Coque et al., Reference Coque, Martin, Veyrieras, Hirel and Gallais2008; Namai et al., Reference Namai, Toriyama and Fukuta2009; Gaju et al., Reference Gaju, Allard, Martre, Snape, Heumez, Legouis, Moreau, Bogard, Griffiths and Orford2011). For instance, QTLs for NupE and root dry weight under low N conditions were reported linked in a Chinese doubled haploid wheat population (An et al., Reference An, Su, Liu, Zhu, Tong, Li, Jing, Li and Li2006). In a QTL meta-analysis, QTLs for NupE, traits of root architecture (root depth, surface area, diameter) and leaf stay-green positively coexisted in eight clusters of QTLs, while QTLs for N remobilization coincided with QTLs for leaf senescence in maize (Coque et al., Reference Coque, Martin, Veyrieras, Hirel and Gallais2008). While referring to the results in the meta-analysis, the authors hypothesized that NupE and N remobilization (or NutE) are independently inherited traits, and traits associated with these two NUE sub-components may, therefore, be combined through breeding to further improve NUE (Dawson et al., Reference Dawson, Huggins and Jones2008).
Although the final goal of breeding for both organic and conventional agriculture converges at increasing productivity through improvement of crop potential, biotic and abiotic resistance, in terms of root function for improved NUE, breeding approaches depend on soil N levels where intended bred cultivars grow. Root traits for conventional agriculture require an early vigorous development of roots. Such roots are robust and uptake N when and as available depending on whether applied as conventional, slow-released or split fertilizer regime. In contrast, proliferation of root system and root biomass could be more important under organic or low available N conditions. Wheat breeding lines with early vigorous root branching resulted in a higher N uptake and biomass production in sandy soil where nitrate leaching and runoff are major problems (Wu et al., Reference Wu, Bernardo, Mapp, Geleta, Teague, Watkins, Sabbagh, Elliott and Stone1997; Liao et al., Reference Liao, Fillery and Palta2004, Reference Liao, Palta and Fillery2006). As a consequence, the final amount of N per plant was greater in these vigorous wheat lines than in conventional cultivars (Liao et al., Reference Liao, Palta and Fillery2006). This trait can, therefore, reduce N leaching into the environment when fertilizer is applied at seeding as a single dose. The authors also highlighted the significance of the root-branching pattern for early N uptake. The contribution of branched root system in the upper and middle soil profile (0–0.7 m) to N fertilizer recovery was greater than the deeper root system. This greater contribution was related to larger root length density at shallower soil profiles, although nitrate is soluble and easily moves into roots by mass flow in transpiration and diffusion, so that roots do not need to have a physical interface with N (Liao et al., Reference Liao, Palta and Fillery2006). Similar results were observed in maize in another study (Wiesler and Horst, Reference Wiesler and Horst1994). Cultivars with this trait may be more versatile for any cultivation strategies to reduce N loss if the capacity of N storage in their vegetative parts is high.
For crops grown in limited N condition, the proliferation of roots is advantageous where there is irregular and unpredictable soil N availability. Increased proliferation of roots is considered an adaptability to the spatial and temporal heterogeneity of soil N concentration in several crops, including maize, wheat and barley in response to the localized high N availability (Drew et al., Reference Drew, Saker and Ashley1973; Drew and Saker, Reference Drew and Saker1975; Hong-Bo et al., Reference Hong-Bo, Zhang and Jian-Bo2012; Jing et al., Reference Jing, Zhang, Rengel and Shen2012; Shen et al., Reference Shen, Li, Mi, Li, Yuan, Jiang and Zhang2013). The proliferation of lateral roots in high N concentration patch and consequent increased N inflow into crops logically increase NUE under soil conditions of uneven SOM, which is characteristic of organic fields. The benefit of lateral root proliferation can be expected not only in organic, but also conventional fields with band placement of N fertilizer. Although there appears to be a small contribution of the root proliferation to total N uptake in some studies (Wiesler and Horst, Reference Wiesler and Horst1994; Van Vuuren et al., Reference Van Vuuren, Robinson and Griffiths1996), the mechanism underlying this root proliferation within the soil zone of localized high N concentration has been only validated on the basis of single plant inter-C/N economy (Robinson, Reference Robinson2001). In the plant inter-specific competition model, the author found that the extra C required for the proliferation of roots was just 0.2% of daily C gain from photosynthesis under 12 h photoperiod, which is much less than that for establishment of AM symbiosis (e.g. 4–20%) (Douds et al., Reference Douds, Pfeffer, Shachar-Hill, Kapulnik and Douds2000; Graham, Reference Graham, Podila and Douds2000; Robinson, Reference Robinson2001). The ability of C assimilation and allocation that is governed by the gradient of labile C in shoots and leaves is assumed to have an influence on root biomass production (Andrews et al., Reference Andrews, Raven and Sprent2001; Chun et al., Reference Chun, Mi, Li, Chen and Zhang2005). Therefore, as long as the sacrifice of C to promote root proliferation is at a reasonable cost and compensates the benefit of extra N inflow, it can be beneficial to choose any means of fertilization that promote root proliferation. The gene responsible for this reaction is well-described in a review (Walch-Liu et al., Reference Walch-Liu, Ivanov, Filleur, Gan, Remans and Forde2006a).
An investigation of the genetic relationship between the height reducing Rht genes and root traits using doubled-haploid and near-isogenic lines in wheat found coincidence of QTLs for plant height and root traits (Bai et al., Reference Bai, Liang and Hawkesford2013). Dwarfing genes (Rht-B1c, Rht-D1c and Rht12) reduced root-related traits, including root length, total root surface area, root dry weight and some Rht genes controlled both height and root traits (Bai et al., Reference Bai, Liang and Hawkesford2013). We may need to take into account this relationship between Rht genes and root traits because the introduction of Rht dwarfing genes may have a negative impact on root proliferation traits that can be useful characteristics for improving NUE (Bai et al., Reference Bai, Liang and Hawkesford2013; Hawkesford, Reference Hawkesford2014).
Walch-Liu et al. (Reference Walch-Liu, Liu, Remans, Tester and Forde2006b) proposed a novel theory that the presence of L-glutamate in organic N-rich soil induces shorter but branched root system in Arabidopsis to efficiently acquire N, while inhibiting primary root growth. They observed natural variation for L-glutamate sensitivity among different ecotypes, suggesting that this initiation of root branching is genotype-dependent. This finding suggests that breeders might take advantage of this trait to develop new cultivars with branched roots. This trait may be more advantageous for crops grown in organic fields because, in general, soil under organic management contains more SOM thus is likely to have more L-glutamate than in conventional management. Further detailed studies of genes involved in the response of L-glutamate are needed. As a result of significant interaction between genotypic profiles and soil N availability, selection for elite NUE traits adapting given N levels should take place at several levels of soil N concentrations in order to devise their potentials toward appropriate agronomic practices (Poorter and Nagel, Reference Poorter and Nagel2000; Trachsel et al., Reference Trachsel, Kaeppler, Brown and Lynch2013; Obara et al., Reference Obara, Ishimaru, Abiko, Fujita, Kobayashi, Yanagihara and Fukuta2014).
Optimization of N supply and demand
The observed genetic variation for NUE in various grain crops under different spatial and temporal soil N availability suggests a possibility to optimize NUE by filling the gaps in these environmental- and cultivar-specific variations (Scharf et al., Reference Scharf, Kitchen, Sudduth, Davis, Hubbard and Lory2005; Coque et al., Reference Coque, Martin, Veyrieras, Hirel and Gallais2008; Anbessa et al., Reference Anbessa, Juskiw, Good, Nyachiro and Helm2009; Bancal, Reference Bancal2009; Cui et al., Reference Cui, Zhang, Mi, Chen, Li, Chen, Li and Shi2009; Gaju et al., Reference Gaju, Allard, Martre, Snape, Heumez, Legouis, Moreau, Bogard, Griffiths and Orford2011).
The breeding strategy for improved morphological and physiological N efficiency is mainly based on knowledge of functions of crop organs and enzymatic activities involved in N metabolism of absorption, assimilation, translocation and remobilization by considering the crop itself or cereals as N sink (Fig. 1) (Simpson et al., Reference Simpson, Lambers and Dalling1982; Cormier et al., Reference Cormier, Faure, Dubreuil, Heumez, Beauchêne, Lafarge, Praud and Le Gouis2013). Thus, the characterization of related traits needs to be explored. Contrarily, agronomic strategies for better NUE are based on strategies that reduce N loss from soil systems and efficiently supply N according to crop demand. Although theoretical mechanisms of each NUE component have been studied for decades, improvement of a single crop trait or cultivation technique does not necessarily result in increased crop NUE (Fraisier et al., Reference Fraisier, Gojon, Tillard and Daniel-Vedele2000; Pathak et al., Reference Pathak, Ahmad, Lochab and Raghuram2008). Several NUE studies emphasize the importance of synchrony between N supply from N sources and plant N demand as an N sink (Sandhu et al., Reference Sandhu, Arora, Chand, Sandhu and Khera2000; Crews and Peoples, Reference Crews and Peoples2005; Shanahan et al., Reference Shanahan, Kitchen, Raun and Schepers2008). Asynchronous events could happen spatially and temporally when N supply exceeds plant demands or when N supply is insufficient to meet crop demands (Crews and Peoples, Reference Crews and Peoples2005). Although, the former often occurs in conventional systems and the latter is more prominent in many low input/organic production systems, improvement of synchrony between N demand and supply, and reduction of N loss from agricultural systems, maybe achieved through a combination of several different approaches (Table 5). The integration of knowledge from breeding and agronomic strategies, therefore, may provide useful information to improve NUE although the lack of on-farm studies about optimization of N supply and demand will require long-term commitments.
Table 5. Factors controlling synchrony between nitrogen supply and crop demand in conventional and organic agriculture (Crews and Peoples, Reference Crews and Peoples2005).

As a result of significant interaction between genotypic profiles and soil N availability, selection for elite NUE traits should take place at several levels of soil N concentrations in order to devise appropriate agronomic practices (Poorter and Nagel, Reference Poorter and Nagel2000; Trachsel et al., Reference Trachsel, Kaeppler, Brown and Lynch2013; Obara et al., Reference Obara, Ishimaru, Abiko, Fujita, Kobayashi, Yanagihara and Fukuta2014). The scarcity of N in the soil solution results in greater biomass partitioning in roots than in shoots (Poorter and Nagel, Reference Poorter and Nagel2000; Chun et al., Reference Chun, Mi, Li, Chen and Zhang2005). In this situation, a cultivar with improved stay-green trait may not display its ability toward NUE. It has also been reported that root growth angles of maize inbred lines become steeper (i.e. deeper) in N deficit condition (Trachsel et al., Reference Trachsel, Kaeppler, Brown and Lynch2013). The total root length in rice increases in high soil ammonium concentration (Obara et al., Reference Obara, Ishimaru, Abiko, Fujita, Kobayashi, Yanagihara and Fukuta2014). Through these responses to soil N concentration, crops modify their root systems. It has been demonstrated that the relative contribution of NupE and NutE on NUE depends upon the soil N levels (Moll et al., Reference Moll, Kamprath and Jackson1982; Le Gouis et al., Reference Le Gouis, Béghin, Heumez and Pluchard2000). The contribution of NupE toward NUE is greater than that of NutE in low soil N concentrations in spring wheat (Ortiz-Monasterio et al., Reference Ortiz-Monasterio, Sayre, Rajaram and Mcmahon1997; Le Gouis et al., Reference Le Gouis, Béghin, Heumez and Pluchard2000; Muurinen et al., Reference Muurinen, Slafer and Peltonen-Sainio2006). The contributions of both NupE and NutE are same at moderate soil N concentrations, and that of NutE is greater than NupE under high soil N levels (Ortiz-Monasterio et al., Reference Ortiz-Monasterio, Sayre, Rajaram and Mcmahon1997). In a study of genetic variability among ancestral and modern cultivated wheat, Gorny and Garczyński (Reference Gorny and Garczyński2008) found that under low soil N level, the primitive polyploid species had higher NupE, whereas the modern cultivated hexaploid species had better NutE. Generally, a low soil N level decreases biomass and grain yield, but improves NutE in wheat (Gorny and Garczyński, Reference Gorny and Garczyński2008). Therefore, selection from a wide range of germplasm under low soil N levels may be useful in breeding cultivars for different management systems (Reid et al., Reference Reid, Yang, Salmon and Spaner2009). Although results of these studies varied, they did indicate genetic variability with differences in soil N concentration. Therefore, performance of new cultivars may not meet breeders’ expectation when grown under conditions different than those where breeding was practised. In addition, heritability of NUE-related traits seems to vary with N levels, which complicates selection for these traits (Presterl et al., Reference Presterl, Groh, Landbeck, Seitz, Schmidt and Geiger2002; Laperche et al., Reference Laperche, Brancourt-Hulmel, Heumez, Gardet and Le Gouis2006; Coque et al., Reference Coque, Martin, Veyrieras, Hirel and Gallais2008; Reid et al., Reference Reid, Yang, Salmon and Spaner2009). Therefore, it is useful to have more information about the behavior of important NUE-related traits under different soil N concentration to breed ideal cultivars for specific N management practices.
The concept of N dilution curve has been studied as a crop N status indicator. This curve is a plant-based diagnostic tool providing an estimation of the crop total N requirement under given N conditions for optimum biomass production (Greenwood et al., Reference Greenwood, Lemaire, Gosse, Cruz, Draycott and Neeteson1990). Nitrogen concentration in crop aboveground biomass declines according to growth stages even under optimal soil N levels (Justes et al., Reference Justes, Mary, Meynard, Machet and Thelier-Huché1994; Hoogmoed, Reference Hoogmoed, AcuñA, Moeller, Parsons and Harrison2015). This N dilution phenomenon is commonly shared in any species and genotypes of crops grown in any climatic conditions and is related to plant aboveground biomass production (Lemaire and Meynard, Reference Lemaire, Meynard and Lemaire1997; Lemaire et al., Reference Lemaire, Van Oosterom, Sheehy, Jeuffroy, Massignam and Rossato2007). The curve is, therefore, a useful decision-making tool for N fertilization. Crop-specific nitrogen dilution curves have been proposed in wheat (Justes et al., Reference Justes, Mary, Meynard, Machet and Thelier-Huché1994; Ziadi et al., Reference Ziadi, Bélanger, Claessens, Lefebvre, Cambouris, Tremblay, Nolin and Parent2010; Hoogmoed, Reference Hoogmoed, AcuñA, Moeller, Parsons and Harrison2015), rice (Sheehy et al., Reference Sheehy, Dionora, Mitchell, Peng, Cassman, Lemaire and Williams1998; Ata-Ul-Karim et al., Reference Ata-Ul-Karim, Yao, Liu, Cao and Zhu2014a, Reference Ata-Ul-Karim, Zhu, Yao and Caob) and maize (Herrmann and Taube, Reference Herrmann and Taube2004). Utilization of the curve to evaluate a critical point of the best balance between crop N concentration and biomass may be another approach for improving crop NUE.
Application of NUE knowledge is more challenging in organic production systems due to a number of uncontrollable biotic- and abiotic factors, which result in significant phenotypic variation. Therefore, synchronization in N sink and source in organic fields is more challenging. From sink's perspective, for instance, the prediction of expected genetic gain in spring wheat by direct selection in an organic field is difficult because genetic parameters cannot be precisely estimated due to variability in some agronomic traits (Reid et al., Reference Reid, Yang, Salmon and Spaner2009). A low heritability for grain protein content is reported in organically grown wheat, while others reported high heritability for NupE, NutE and NrE in wheat and maize under organic conditions (Presterl et al., Reference Presterl, Groh, Landbeck, Seitz, Schmidt and Geiger2002; Coque and Gallais, Reference Coque and Gallais2007; Reid et al., Reference Reid, Yang, Salmon and Spaner2009). Struik and Yin (Reference Struik, Yin, Østergård, Lammerts Van Bueren and Bouwman-Smiths2009) concluded that polygenic control, environmental variation (e.g. plant available N concentration, timing and amount of mineralization, weed and disease pressure) and their interaction with NUE-related traits cause inconsistencies and hinder improvement of crop NUE through conventional breeding. Therefore, QTL mapping and marker-assisted selection for traits associated with NUE and the interaction of these genes/QTLs with environment and management practices may provide breeders with tools to improve NUE for target management systems (Struik and Yin, Reference Struik, Yin, Østergård, Lammerts Van Bueren and Bouwman-Smiths2009; Van Bueren et al., Reference Van Bueren, Backes, De Vriend and Østergård2010).
Conclusion
NUE is a complex trait to improve with many potential interactions and trade-offs with other factors controlling final yield. Key genotypic factors for improving crop NUE seem to be common traits between conventional and organic agriculture. Those traits capture and utilize N when it is available for grain production. However, those regulations and responses vary according to genotypes, environmental and agronomic regimes. Therefore, a genetic, environmental and N-level interaction should be taken into consideration in breeding programs for intended production systems. The agronomic approach requires considering ways to synchronize N supply with crop N demand besides optimizing N utilization in a system. Implementation of BMPs for N fertilization has great potential to fill the gap between optimum and the actual current practices, and thus in the improvement of NUE in conventional production systems. Organic practices put more emphasis on incorporation of legumes in crop rotation and diversification of cropping practices. Factors controlling crop NUE and the efficiency in crop production management are intertwined in both organic and conventional systems. The challenge of maintaining crop yield and improving NUE needs emphasis globally. While theoretical approaches to improving NUE by altering single traits related to N efficiency are necessary, studies covering holistic approaches will lead to improvement of NUE within the entire agroecosystem.
Acknowledgements
This research was financed by grants to the University of Alberta wheat breeding program from the Alberta Crop Industry Development Fund, Organic Agriculture Cluster grant from Agriculture and Agri-Food Canada, Western Grains Research Foundation Endowment and Core Funds, the Alberta Wheat Commission and an NSERC Discovery Grant to D. Spaner.