INTRODUCTION
Skeletal remains, both faunal and human, are frequently encountered at archaeological sites and are vitally important for reconstructing environment, evolution, and chronology. The organic fraction, collagen, is by far the most frequently radiocarbon-dated material, while the mineral fraction was long seen as unreliable and affected by contamination issues (Zazzo and Saliège Reference Zazzo and Saliège2011). This stands in contrast to research on stable isotopes where dental enamel is considered especially reliable (Lee-Thorp and van der Merwe Reference Lee-Thorp and van der Merwe1991).
It is only recently that interest in 14C dating of bioapatites has increased again (for an overview see Zazzo and Saliège Reference Zazzo and Saliège2011). While work on calcined bone shows reliable results (e.g. Lanting et al. Reference Lanting, Aerts-Bijma and van der Plicht2001; Naysmith et al. Reference Naysmith, Scott, Cook, Heinemeier, van der Plicht, Van Strydonck, Bronk Ramsey, Grootes and Freeman2007), methodological attempts at understanding dental enamel alteration and resulting pretreatment effects remain rare (e.g. Hedges et al. Reference Hedges, Lee-Thorp and Tuorss1995; Zazzo Reference Zazzo2014).
Research has shown that in tropical and arid climates, collagen rapidly deteriorates (Saliège et al. Reference Saliège, Person and Paris1995; Zazzo and Saliège Reference Zazzo and Saliège2011) and many Paleolithic skeletal remains show very low to no collagen yield (Weiner and Bar-Yosef Reference Weiner and Bar-Yosef1990; Pinhasi et al. Reference Pinhasi, Nioradze, Tushabramishvili, Lordkipanidze, Pleurdeau, Moncel, Adler, Stringer and Higham2012). Dating bioapatite would therefore tremendously increase the application range of 14C dating. Furthermore, teeth are often considered to be better preserved (though see Zazzo Reference Zazzo2014) and allow for easier species identification than small bone fragments.
14C dates of dental enamel have a long history of being too young. In 1961, before the introduction of chemical contamination removal steps, Olson and Broecker (Reference Olson and Broecker1961) suggested that apatite dates would continue to be too young “as a consequence of ground-water-carbonate contamination.” Accuracy improved with the introduction of HCl and acetic acid purification steps (Berger et al. Reference Berger, Horney and Libby1964; Haynes Reference Haynes1968) and later with fractional hydrolysis (Hassan et al. Reference Hassan, Termine and Haynes1977) and step heating of fossil apatite (Haas and Banewicz Reference Haas and Banewicz1980). Nonetheless, the unreliability of the dates and results from the first enamel specific study by Hedges et al. (Reference Hedges, Lee-Thorp and Tuorss1995) seemed to prove Olson and Broecker right.
In the light of the improved method for extracting collagen developed by Longin (Reference Longin1971), the focus turned towards collagen purification methodology as a means to increase accuracy (see e.g. Long et al. Reference Long, Wilson, Ernst, Gore and Hare1989; Higham et al. Reference Higham, Jacobi and Bronk Ramsey2006; Marom et al. Reference Marom, McCullagh, Higham, Sinitsyn and Hedges2012).
The attitude towards bioapatite started shifting when Surovell (Reference Surovell2000) managed to obtain the first accurate dental enamel date as old as 10,810±40 BP. More recently, two enamel samples from Gobero (Niger) corresponded well with 14C dates obtained from bone apatite, charcoal, bone artifacts, sediments, and mollusks (Sereno et al. Reference Sereno, Garcea, Jousse, Stojanowski, Saliège, Maga, Ide, Knudson, Mercuri, Stafford, Kaye, Giraudi, N’Siala, Cocca, Moots, Dutheil and Stivers2008), and Cherkinsky (Reference Cherkinsky2009) published comparable collagen and enamel dates for a llama and a sheep. In Zazzo (Reference Zazzo2014), several comparisons between bone apatite and enamel fractions (using acetic acid leaching under weak vacuum) and a collagen reference age are presented. Also here, accurate dates were only obtained on samples from the Holocene, though large variation meant that enamel dates remain unreliable and have to be considered a terminus ante quem. With age, the disparity between the bioapatite date and the collagen reference increases to around 20,000 14C yr for a rhino specimen 40,000 14C yr old from Kent’s Cavern (Hedges et al. Reference Hedges, Lee-Thorp and Tuorss1995).
Enamel mineral is an impure carbonate-containing apatite (bioapatite). Its exact chemical composition and structure is difficult to determine as there is large variation, due to environmental and biological influences. In structure, it is similar to hydroxyapatite [Ca10(PO4)6(OH)2] with the phosphate (B-site) and hydroxyl group (A-site) positions partially occupied by carbonates (LeGeros et al. Reference LeGeros, Trautz, Klein and LeGeros1969; Skinner Reference Skinner2005: 667–78). Bioapatite can be summarized as follows, with potential vacancies represented by [] (LeGeros Reference LeGeros1991; LeGeros et al. Reference LeGeros, Balmain and Bonel1986; Skinner Reference Skinner2005: 667–78):

While bioapatite can also refer to the mineral component of bone, dental enamel contains significantly less carbonates and shows higher crystallinity. Furthermore, after maturation, enamel—unlike bone apatite—is assumed to be chemically and structurally invariant. This, combined with very low porosity, suggests that enamel has a higher resistance to diagenesis than bone apatite (Krueger Reference Krueger1991; Lee-Thorp and van der Merwe Reference Lee-Thorp and van der Merwe1991; Fraser et al. Reference Fraser, Grün, Privat and Gagan2008). However, overall carbonate concentration in enamel is very low (about 0.5–0.8 wt%) (Hedges and Law Reference Hedges and Law1989; Sydney-Zax et al. Reference Sydney-Zax, Mayer and Deutsch1991). Consequently, even with modern accelerator mass spectrometry (AMS) dating methods, large samples are required and small amounts of modern contamination may severely influence dating accuracy. A recent study by Zazzo (Reference Zazzo2014) suggests that the 14C signal in enamel is not necessarily better preserved than in bone apatite.
Biogenic carbon does not differ chemically from the most common forms of carbonate contamination. The main source of contamination is expected to be (ground)water carbonates, an assumption consistent with erroneous enamel dates being too young rather than too old, even when buried in a 14C-depleted environment (Zazzo Reference Zazzo2014). Research has shown that exogenous carbonates do not restrict themselves to the outer enamel surface, as finely ground enamel powder gave better pretreatment results than using larger enamel chunks (Zazzo Reference Zazzo2014). It is therefore expected that contaminants are also to be found at crystal grain boundaries, but whether they replace their position in the crystal grain remains unclear. In case of contamination forming part of the labile, more reactive component of the structure, adequate acid pretreatment should be able to counteract the process, thus improving dating validity. It is hoped that this pilot study will provide further data on dental enamel contamination and 14C dating.
METHODS
Samples
Three specimens covering the broad dating range of the 14C method were analyzed (see Figure 1). Two archaeological samples were chosen from a temperate climate with a high likelihood of exposure to water carbonates, one from the Pleistocene and one from the Holocene: an Upper Paleolithic woolly rhino molar (UP) from a paleochannel excavated in Sutton Courtenay (Oxfordshire, UK), and a Late Roman cattle molar (R) from the well fillings of a settlement at Tiddington (Warwickshire, UK). A modern unburied sample consisting of four equine incisors (M) from an individual specimen from Wakefield (Yorkshire, UK) was added as a control. The teeth were extracted at the Equine Veterinary Centre in Doncaster, after the specimen died in 2013 aged 25.5 yr.

Figure 1 Specimens used in this study: Upper Paleolithic woolly rhino molar (top left), Late Roman cattle 2nd molar (right), modern equine incisors (bottom left).
Sample Preparation
All specimens were cleaned with an air-abrader using aluminum oxide powder at 40 psi and minimum powder flow to prevent heating. Subsequently, all residue powder was removed with an air duster and cavities that were difficult to reach were emptied with the help of slight vibrations from a diamond drill. For further information on equipment used at ORAU, see Brock et al. (Reference Brock, Higham, Ditchfield and Bronk Ramsey2010).
The enamel was sampled using a diamond drill with rotation below 3000 rpm, once again to avoid heating. The enamel powder was collected in aluminum foil and homogenized in a glass beaker to prevent inhomogeneous distribution of carbon distorting the results. Dental enamel from the four modern lower incisors was combined and homogenized in order to obtain a sufficient amount of sample for the experiment. This homogeneous fraction is used as a reference to observe if any pretreatment has an adverse effect on the date. If after pretreatment, all ages (including the non-acid-treated fraction) are statistically identical, then the pretreatments do not add ancient carbon to the sample, though the addition of modern carbon cannot be detected.
Pretreatment
Each homogenized sample was divided into five fractions of 200 mg. Fraction 1 was used as a control and left untreated. Fractions 2 to 5 were pretreated with 23 mL of acid solution of various strengths (see Table 1). For technical reasons, this could not be done under weak vacuum as implemented by others (Cherkinsky Reference Cherkinsky2009; Balter and Zazzo Reference Balter and Zazzo2014; Zazzo Reference Zazzo2014).
Table 1 Initial pretreatment used on each of the five sample fractions (F1–F5).

Commonly used acids for removal of adsorbed and diagenetic carbonates present in bioapatites are HCl (e.g. Beech et al. Reference Beech, Mashkour, Hüls and Zazzo2009; Van Strydonck et al. Reference Van Strydonck, Boudin and De Mulder2009) and acetic acid (e.g. Brock et al. Reference Brock, Higham, Ditchfield and Bronk Ramsey2010; Zazzo Reference Zazzo2014). For this study, we decided to focus on HCl as the main acid as it is inorganic and might reduce recrystallization due to fast reaction (for recrystallization see Koch et al. Reference Koch, Tuross and Fogel1997; Lee-Thorp and van der Merwe Reference Lee-Thorp and van der Merwe1991). If the diagenetic carbon is mainly found in the labile component of the enamel, acid pretreatment should preferentially dissolve components high in contamination. If recrystallization occurs during the treatment, diagenetic or exogenous carbon could be (re-)incorporated.
Hydrochloric acid (HCl) concentrations were chosen as a consequence of preliminary tests on enamel from Roman cattle molars. They indicated that 0.05M HCl was the highest concentration of solution that guaranteed enough remaining dental enamel for producing graphite targets of 0.8 mg C from a 200-mg sample. In case of unexpected sample loss, this would still allow for the production of graphite targets of 0.4 mg C, the smallest targets currently processed at ORAU. Additionally, HCl solutions of less than 0.01M used in preliminary tests have caused only minimal sample loss, suggesting that there might not be an observable difference in the 14C date. Furthermore, previous research suggests that stronger and shorter treatments give better results, though the majority of that data stems from samples pretreated with acetic acid solutions (for a list see Zazzo Reference Zazzo2014).
Preliminary tests also showed a difference in FTIR spectra for fractions that were treated with identical solution for 2 hr and 4 hr, respectively. Consequently, fraction 3 was treated with the same HCl solution as fraction 2, but with an increased treatment duration.
We also used a 2M acetic acid pretreatment for 4 hr. The concentration of the acetic acid solution was selected to reflect a pH closer to 0.05M HCl (fraction 5). As previous research indicates better results with stronger acid treatments, it was felt that an increase from 1M to 2M acetic acid should not have an adverse effect.
One batch of solution (HCl and acetic acid, respectively) was prepared in order to make sure that variability in pH did not affect the experiment. That is, identically treated fractions of all three samples (M, R, and UP) were treated with identical solutions.
The samples were left to react in a fridge at 4°C and subsequently rinsed three times with Milli-Q™ water. Between each step, the vessels were centrifuged at 2150 rpm for 5 min. The treated enamel was frozen overnight before freeze-drying for 48 hr.
Radiocarbon Dating
For FTIR analysis, 3 mg of each fraction were set aside. The remaining part was treated alongside IAEA-C1 marble standards following standard protocol for shell carbonates at ORAU (Brock et al. Reference Brock, Higham, Ditchfield and Bronk Ramsey2010: 109), using phosphoric acid (3 mL, 85 %) in vacuo in a two-armed Pyrex® reaction vessel for CO2 extraction. CO2 was recycled using an in-house gas collection system, a Carlo Erba elemental analyzer, and a Sercon stable isotope mass spectrometer. The small graphite targets (0.8 mg C) were dated using the HVEE AMS system at ORAU as described by Bronk Ramsey et al. (Reference Bronk Ramsey, Higham and Leach2004).
The results were compared as fraction modern (F14C), as well as in conventional 14C ages (BP) and calibrated 14C ages (cal BC/AD). The first is applied to detect small changes in 14C content, the latter to see how the variation would impact archaeological dating. The calibrated calendar ages were preferred over the more commonly used cal BP values, in order to have all three specimens, including the post-1950 one, on the same timeline. The uncalibrated ages are necessary to make our data more readily comparable using future calibration curves.
The 14C dates were calibrated using OxCal v 4.2 (Bronk Ramsey Reference Bronk Ramsey2009) with IntCal13 (Reimer et al. Reference Reimer, Bard, Bayliss, Beck, Blackwell, Bronk Ramsey, Buck, Cheng, Edwards, Friedrich, Grootes, Guilderson, Haflidason, Hajdas, Hatté, Heaton, Hoffmann, Hogg, Hughen, Kaiser, Kromer, Manning, Niu, Reimer, Richards, Scott, Southon, Staff, Turney and van der Plicht2013) for the archaeological samples and the Northern Hemisphere Zone 1 bomb curve (Hua et al. Reference Hua, Barbetti and Rakowski2013) for the modern sample.
Fourier Transform Infrared Spectroscopy (FTIR)
FTIR has been successfully used in various studies to assess alterations in dental enamel (e.g. Rink and Schwarcz Reference Rink and Schwarcz1995; Sponheimer and Lee-Thorp Reference Sponheimer and Lee-Thorp1999; Roche et al. Reference Roche, Ségalen, Balan and Delattre2010). Sample fractions were analyzed before and after the experimental pretreatment step with a FTIR in attenuated total reflectance (ATR) mode. Unlike in the transmission mode, there was no need for pressing the powdered dental enamel into pellets, allowing for rapid measurement.
An Agilent Technologies Cary 640 FTIR with GladiATRTM from Pike Technologies with a diamond crystal was used for analysis. Analysis procedures as described by Snoeck et al. (Reference Snoeck, Lee-Thorp and Schulting2014) were followed. There are several indices used for assessing bioapatites. For this study, the infrared splitting factor (IRSF), the type B carbonate to phosphate index (BPI), and the carbonate to carbonate ratio (C/C) were calculated. The IRSF is calculated according to Weiner and Bar-Yosef (Reference Weiner and Bar-Yosef1990) and is indicative of the crystallinity of a sample. BPI assesses the relative proportions of B carbonates. It was calculated using the phosphate peak at 605 cm−1, and carbonate peak at 1415 cm−1 (LeGeros and LeGeros Reference LeGeros and LeGeros1983). The C/C ratio provides information on the change in proportions between carbonates of type A and B (Snoeck et al. Reference Snoeck, Lee-Thorp and Schulting2014).
To estimate the error on the FTIR indexes used in this study, the standard deviation for each triplicate was calculated and subsequently averaged over all samples analyzed (a similar approach can be found in Lebon et al. Reference Lebon, Zazzo and Reiche2014). The results are reported in Table 2 and depicted graphically in Figure 2.

Figure 2 Infrared spectra of modern equine dental enamel (M-F1: untreated; M-F4: pretreated with 0.05M HCl M-F4) with peaks for carbonates, phosphates, and water/amide highlighted. A: shows a decrease in carbonates content after pretreatment with 0.05M HCl. B: highlights the absorbance peaks used to measure the IRSF, indicator of crystallinity. The deeper valley between the two peaks indicated an increase in crystallinity after HCl pretreatment.
Table 2 Description of FTIR indexes used for this study (B = height of a band; V = valley).

Reference Age
The reference age of the two archaeological samples was obtained by 14C dating their dental collagen (see R-Ref and UP-Ref in Table 3). The procedure followed standard ORAU protocol as described by Brock et al. (Reference Brock, Higham, Ditchfield and Bronk Ramsey2010) and contained an additional solvent extraction step for the woolly rhino tooth.
Table 3 Results for all five fractions of the modern equine incisors (M), the late Roman cattle molar (R) and the Upper Paleolithic woolly rhino molar (UP): weight lost during first acid treatment in % (wt loss), δ13C value after treatment, fraction modern carbon (F14C), with conventional (BP) and IntCal13 calibrated 14C age, FTIR indexes after initial pretreatment step and their differences compared to the untreated sample fraction (∆%). Dentine reference age for R and UP added for convenience.

It was deemed unnecessary to use archaeological samples with a non-14C-established known age. The extra precision gained on the reference age would not be translated into a better understanding of dating accuracy, considering the sometimes large errors observed on enamel 14C dates. Such samples shall be reserved for later studies where high precision and accuracy become more relevant. Comparing dentine collagen to enamel bioapatite dates at this stage of research increases the amount of possible samples available, while providing high enough resolution for interpreting the results.
Statistical Analysis
To test whether two F14C values are statistically indistinguishable, we used a two-tailed Z test, where we have assumed the F14C value to be the mean of a normal distribution, with a standard deviation equal to the F14C value’s error. If Z is the difference of the two F14C values, we can test whether P(Z>0)<0.025 or P(Z<0)>0.975 using the Normal cumulative distribution function (Rice Reference Rice2007). We rejected the null hypothesis that two values are statistically indistinguishable at a confidence level of 0.05, and conclude that they are different. The calculations were performed in R (R Core Team 2015). The command Combine() in OxCal v 4.2 was used to perform a χ2 test on the calibrated 14C age probability distributions.
RESULTS
The results for the individual samples are listed in Table 3. Three sample fractions (M-F5, R-F3, R-F5) could not be dated. Fraction 5 of both the modern and the Roman specimen had too little sample material left after pretreatment with 2M acetic acid. The reaction vessel of R-F3 leaked during the CO2 extraction process, leading to contamination with atmospheric carbon. As there was only enough dental enamel left for one more fraction, it was decided to re-attempt the fraction 3 treatment. As a consequence, R-F3-2 was not treated with the identical batch of HCl solution as the original sample fractions M-F3, R-F3, and UP-F3. This was considered unlikely to distort the results as the FTIR analyses indicated R-F3 and R-F2-3 to be comparable. FTIR analysis showed no formation of brushite in any of the samples analyzed.
As expected, all reactions with HCl solution ran to completion (indicated by a neutral pH after treatment) and the acetic acid solution remained acidic even after 4 hr (pH = 4.5). Protocol rinsing was still applied irrespective of pH to keep sample loss as a consequence of rinsing comparable and make sure that all dissolved carbonate was removed.
For the modern equine specimen, all fractions analyzed lie within the error (see Table 3 and Figure 3). Pretreatment with higher concentration of HCl solution produced F14C values closer to the reference for the archaeological samples R and UP (see Figure 3). For both R and UP, fraction 3 shows a slightly higher value than fraction 2. However, they remain statistically indistinguishable. This reflects expectations, as reactions with 0.01M HCl run to completion in both cases (indicated by neutral pH after 2 hr, respective 4 hr). The results for R-F4, albeit slightly higher, are also statistically indistinguishable from R-Ref. Although none of the UP fractions reach a F14C value close to the reference, UP-F5 (the only acetic-acid-treated fraction that survived pretreatment) has a higher F14C value than UP-F4. The difference is statistically significant.
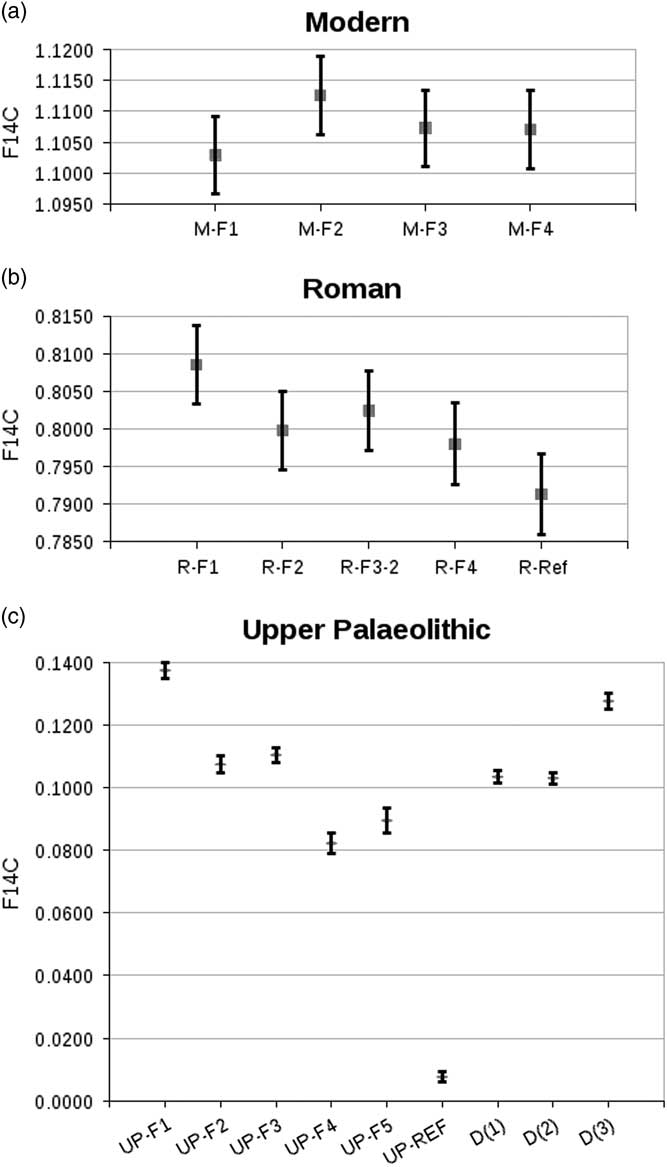
Figure 3 Comparison of F14C values obtained from the dated fractions of sample M (top), sample R (middle), and sample UP (bottom). Results obtained by P Ditchfield for sample UP added for convenience: D(1) = OxA-2392-50, D(2) = OxA-2392-51, D(3) = OxA-2409-7 (see Discussion for details). Error bars at 2σ.
In terms of 14C dating, this translates into calibrated 14C ages for all modern samples that are statistically indistinguishable. The same is true for fraction 2 and 3 of each of the two archaeological specimens. While the calibrated 14C age for the Roman specimen is statistically indistinguishable from the reference, it shows a distribution including younger years than the reference age. This is as a result of a short plateau on the calibration curve. For the woolly rhino specimen, there remains a nearly 20,000-yr gap between the oldest enamel date and the collagen reference.
DISCUSSION
The C/C index may indicate preferential attack of the B-type carbonate with increasing acid concentration. However, this interpretation is not supported by the BPI index and Roche et al. (Reference Roche, Ségalen, Balan and Delattre2010) has suggested that the spectral band at 1455 cm−1 used for C/C might be connected to carbonates absorbed on the bioapatite surface. This could explain the difference in F14C observed in all treated fractions compared to the untreated fraction, but does not match the lower value obtained with 0.05M HCl compared to 2M acetic acid treatment. An increase in IRSF, as observed from F1 to F5 in all three samples, is either indicative of removal of smaller less crystalline crystallites or recrystallization. The lower increase in IRSF combined with the improvement of 14C dating observed for the HCl-treated fractions indicates preferred dissolution of diagenetic carbonates through removal of the more soluble fraction of the sample. In contrast, the results for the 2M acetic-acid-treated fraction F5 (both higher F14C and IRSF values) might suggest recrystallization during the pretreatment process, though brushite was not observed in the FTIR spectra. In any case, there could be a potential for (re-)incorporation of diagenetic or exogenous carbon, which could be avoided by treating the samples under weak vacuum (Zazzo Reference Zazzo2014). It should be stressed that this is a tentative explanation based on the observation of a single sample, as R and M did not survive the 2M acetic acid treatment. Further experiments are necessary to confirm the effect.
UP-F4 shows F14C values closest to the woolly rhino reference compared with the other enamel fractions analyzed in this study as well as with results obtained by Peter Ditchfield in 2009/2010 (unpublished data and see Table 4) using 1.4M NaOCl followed by ORAU standard shell carbonate protocol (Brock et al. Reference Brock, Higham, Ditchfield and Bronk Ramsey2010). However, the result remains 19,130 14C yr too young compared to the collagen age. Furthermore, Ditchfield’s data suggest that 0.01M HCl may give results comparable with simple bleaching, which has theoretically no impact on the carbonate fraction of bioapatites. It is worth noting that the only enamel sample of comparable age found in the literature is a rhino tooth from Kent’s Cavern (UK) analyzed by Hedges et al. (Reference Hedges, Lee-Thorp and Tuorss1995). In their study, the result closest to the reference age was obtained by bleaching powdered enamel (particle size 0.5 mm) and subsequently treating with 1M HCl for 5 min. The 14C age of 24,570±310 BP remains nearly 15,000 14C yr younger than the collagen age of 39,630±1420 BP. At the same time, they also observed that the 1M acetic-acid-treated fraction (duration: overnight) gave a younger age (19,760±200 BP), though no weak vacuum was used. No other enamel samples with collagen reference ages from the Paleolithic have been published so far.
Table 4 Results obtained by P Ditchfield on enamel from the woolly rhino specimen in 2009/2010. All subsamples were treated with 1.4M NaOCl, followed by the standard ORAU shell carbonate protocol (Brock et al. Reference Brock, Higham, Ditchfield and Bronk Ramsey2010).

If these interpretations are correct, the removal of the smaller, less crystaline crystallites reduces exogenous carbon concentration in the sample, but prolonged exposure to the acid solution could potentially lead to less good results. If further experiments with acetic acid reproduce our observations, then keeping reaction times short is key. Balter et al. (Reference Balter, Saliège, Bocherens and Person2002) suggest reaction times as short as 30 min to 1 hr for acetic acid under vacuum. As the only two specimens from the Paleolithic indicate improvements using HCl compared to acetic acid, HCl as a pretreatment should not yet be dismissed. However, it remains to be tested if that difference can also be observed when applying weak vacuum during acetic acid leaching. Furthermore, Zazzo (Reference Zazzo2014) also showed improved results when applying stronger acid treatments, as well as finer ground samples. Though both cause greater sample loss, it would be useful to investigate stronger HCl and acetic acid solutions and whether successive leaching can improve results.
While at this stage dating specimens from arid environments or younger time periods looks promising, Zazzo (Reference Zazzo2014) shows that enamel dates very often remain too young, even in 14C-depleted environments. As old carbon contamination is likely to have a negligible effect on 14C dating of enamel, more Paleolithic samples should be incorporated into future studies, since they are most sensitive to contamination with younger carbon.
CONCLUSION
Results indicate F14C values closer to the reference with stronger HCl solution. This may be due to preferred removal of smaller less crystalline crystallites containing higher inclusion of diagenetic carbon. The higher F14C value observed for UP-F5 (2M acetic acid) shows less effective contamination removal and requires further investigation.
This study is in line with published research suggesting that reliably dating dental enamel may be possible for younger periods. For Paleolithic samples, 14C dates remain substantially too young. Further investigation into pretreatment is necessary, especially on whether a stronger HCl solution leads to improved results and how HCl solutions compare to acetic acid leaching under weak vacuum. It is vital that more Paleolithic samples form part of this research, as they are most sensitive to younger carbon contamination and complement results obtained from less fossilized specimens.
ACKNOWLEDGMENTS
This research was conducted as part of a MSc in Archaeological Science at the University of Oxford in 2013. The author gratefully acknowledges support from the Oxford Radiocarbon Accelerator Unit, the Research Laboratory for Archaeology and the History of Art, and Wolfson College Oxford. Particular thanks to Richard Coppack from the Equine Veterinary Centre in Doncaster for sending the modern equine teeth, and to Peter Ditchfield for providing the woolly rhino data from 2009/2010.