INTRODUCTION
Research on the Upper Paleolithic in Eastern Europe reached a new stage at the turn of the 21st century. The main cultural complexes and their robust chronology are now established (Hoffecker Reference Hoffecker2002, Reference Hoffecker2017; Brantingham et al. Reference Brantingham, Kuhn and Kerry2004; Anikovich et al. Reference Anikovich, Sinitsyn, Hoffecker, Holliday, Popov, Lisitsyn, Forman, Levkovskaya, Pospelova, Kuz’mina, Burova, Goldberg, Macphail, Giaccio and Praslov2007; Vasil’ev et al. Reference Vasil’ev, Sinitsyn and Otte2017); the general patterns of the paleodiet have been determined (Richards et al. Reference Richards, Pettitt, Stiner and Trinkaus2001); and DNA was extracted and analyzed for several early modern humans (Seguin-Orlando et al. Reference Seguin-Orlando, Korneliussen, Sikora, Malaspinas, Manica, Moltke, Albrechtsen, Ko, Margaryan, Moiseyev, Goebel, Westaway, Lambert, Khartanovich, Wall, Nigst, Foley, Lahr, Nielsen, Orlando and Willerslev2014; Fu et al. Reference Fu, Posth, Hajdinjak, Petr, Mallick, Fernandes, Furtwangler, Haak, Meyer, Mittnik, Nickel, Peltzer, Rohland, Slon, Talamo, Lazaridis, Lipson, Mathieson, Schiffels, Skoglund, Derevianko, Drozdov, Slavinsky, Tsybankov, Cremonesi, Mallegni, Gely, Vacca, Gonzalez Morales, Straus, Neugebauer-Maresch, Teschler-Nicola, Constantin, Moldovan, Benazzi, Peresani, Coppola, Lari, Ricci, Ronchitelli, Valentin, Thevenet, Wehrberger, Grigorescu, Rougier, Crevecoeur, Flas, Semal, Mannino, Cupillard, Bocherens, Conard, Harvati, Moiseyev, Drucker, Svoboda, Richards, Caramelli, Pinhasi, Kelso, Patterson, Krause, Pääbo and Reich2016; Sikora et al. Reference Sikora, Seguin-Orlando, Sousa, Albrechtsen, Korneliussen, Ko, Rasmussen, Dupanloup, Nigst, Bosch, Renaud, Allentoft, Margaryan, Vasilyev, Veselovskaya, Borutskaya, Deviese, Comeskey, Higham, Manica, Foley, Meltzer, Nielsen, Excoffier, Lahr, Orlando and Willerslev2017). Among these sites, Sungir (a.k.a. Sunghir and Sungir’) is of primary interest and importance worldwide for Upper Paleolithic archaeology, physical anthropology and ecology, primarily because its burials are extremely rich in artifacts and adornments, good preservation of bones, and the possibility to reconstruct for the first time the shape and size of complex clothes (Bader Reference Bader, Bader and Lavrushin1998; Gilligan Reference Gilligan2019).
The Sungir site is located in the central part of the Russian Plain (56°10′30″N, 40°30′30″E), on the outskirts of the city of Vladimir, near the promontory created by the Klyazma River and the small Sungir Creek (Figure 1). It was discovered in 1955 and excavated under ON Bader’s leadership in 1957–1977. Three well-preserved human burials (one in Grave 1, and two in Grave 2) with a highly diverse assemblage of grave goods, undoubtedly the richest recorded one for the Paleolithic period worldwide (Bahn Reference Bahn2001: 428–429; Pettitt Reference Pettitt2011), were discovered in 1964 and 1969 (Bader Reference Bader1967, Reference Bader, Bader and Lavrushin1998; Trinkaus et al. Reference Trinkaus, Buzhilova, Mednikova and Dobrovolskaya2014). Studies on a smaller scale were conducted in 1987–1995 by NO Bader, and in 2000–2005 by NO Bader and AB Seleznev. The latest small-scale investigations were carried out in 2014–2015 by KN Gavrilov and SY Lev (Gavrilov et al. Reference Gavrilov, Voskresenskaya, Eskova, Lev, Mashchenko, Panin and Reynolds2021). So far, Sungir is unique for its large collection of artifacts and human fossils. The details of site’s archaeology, stratigraphy, and physical anthropology can be found in the Supplementary Online Material (Supplementary Figures S1–S4).

Figure 1 General topographic plan of the Sungir site and its environs; the site’s location is indicated by the ellipse. The inset shows the boundaries of the excavation pits dug in 1957–2015 (gray rectangles).
Studies of the chronology and diet for the people buried at Sungir were initially undertaken in the early 2000s (Pettitt and Bader Reference Pettitt and Bader2000; Kuzmin et al. Reference Kuzmin, Burr, Jull and Sulerzhitsky2004), and they continued in the 2010s (Dobrovolskaya et al. Reference Dobrovolskaya, Richards and Trinkaus2012; Marom et al. Reference Marom, McCullagh, Higham, Sinitsyn and Hedges2012; Kuzmin et al. Reference Kuzmin, van der Plicht and Sulerzhitsky2014; Nalawade-Chavan et al. Reference Nalawade-Chavan, McCullagh and Hedges2014). However, the previously obtained results of radiocarbon (14C) dating were not consistent. Attempts were made to extract the organic part of human bones free of possible contamination caused by the treatment with a butyral solution in alcohol and BF-2 glue (phenol polyvinyl acetate), as it is well-documented (Bader Reference Bader, Bader and Lavrushin1998). Hydroxyproline (HYP) radiocarbon dating is suggested as the most reliable compound when bones are treated with consolidants (Marom et al. Reference Marom, McCullagh, Higham, Sinitsyn and Hedges2012; Nalawade-Chavan et al. Reference Nalawade-Chavan, McCullagh and Hedges2014). Comparison with material treated with XAD resin indicates that HYP 14C ages tend to be slightly older (Devièse et al. Reference Devièse, Stafford, Waters, Wathen, Comeskey, Becerra-Valdivia and Higham2018). This could be due to either incomplete removal of contaminants using XAD resin or column bleed of the high performance liquid chromatography (HPLC).
Before the present study, the direct 14C ages were generated for the Sungir humans on bulk and ultrafiltered collagen, and HYP extracted from the collagen, and they varied from ca. 19,160 BP ca. 30,000 BP, respectively (Table 1). These dates are distributed in a kind of helter-skelter pattern, without clear trend (Figure 2), although the HYP dates seem to consistently provide the oldest 14C ages. Unfortunately, the parameters for quality of collagen in several cases are either reported incompletely or not given at all (Kuzmin et al. Reference Kuzmin, van der Plicht and Sulerzhitsky2014; see Table 1).
Table 1 The 14C dates for human burials of the Sungir site obtained prior to current study.

a BC – bulk collagen (non-ultrafiltered); UF – ultrafiltered collagen; HYP – hydroxyproline; AA – amino acids.
b Calib Rev 8.1.0 software was used (Reimer et al. Reference Reimer, Austin, Bard, Bayliss, Blackwell, Bronk Ramsey, Butzin, Cheng, Edwards, Friedrich, Grootes, Guilderson, Hajdas, Heaton, Hogg, Hughen, Kromer, Manning, Muscheler, Palmer, Pearson, van der Plicht, Reimer, Richards, Scott, Southon, Turney, Wacker, Adolphi, Büntgen, Capano, Fahrni, Fogtmann-Schulz, Friedrich, Köhler, Kudsk, Miyake, Olsen, Reinig, Sakamoto, Sookdeo and Talamo2020). Calibrated ranges combined; values are rounded to the next 10 years.
c Non-specified bone.
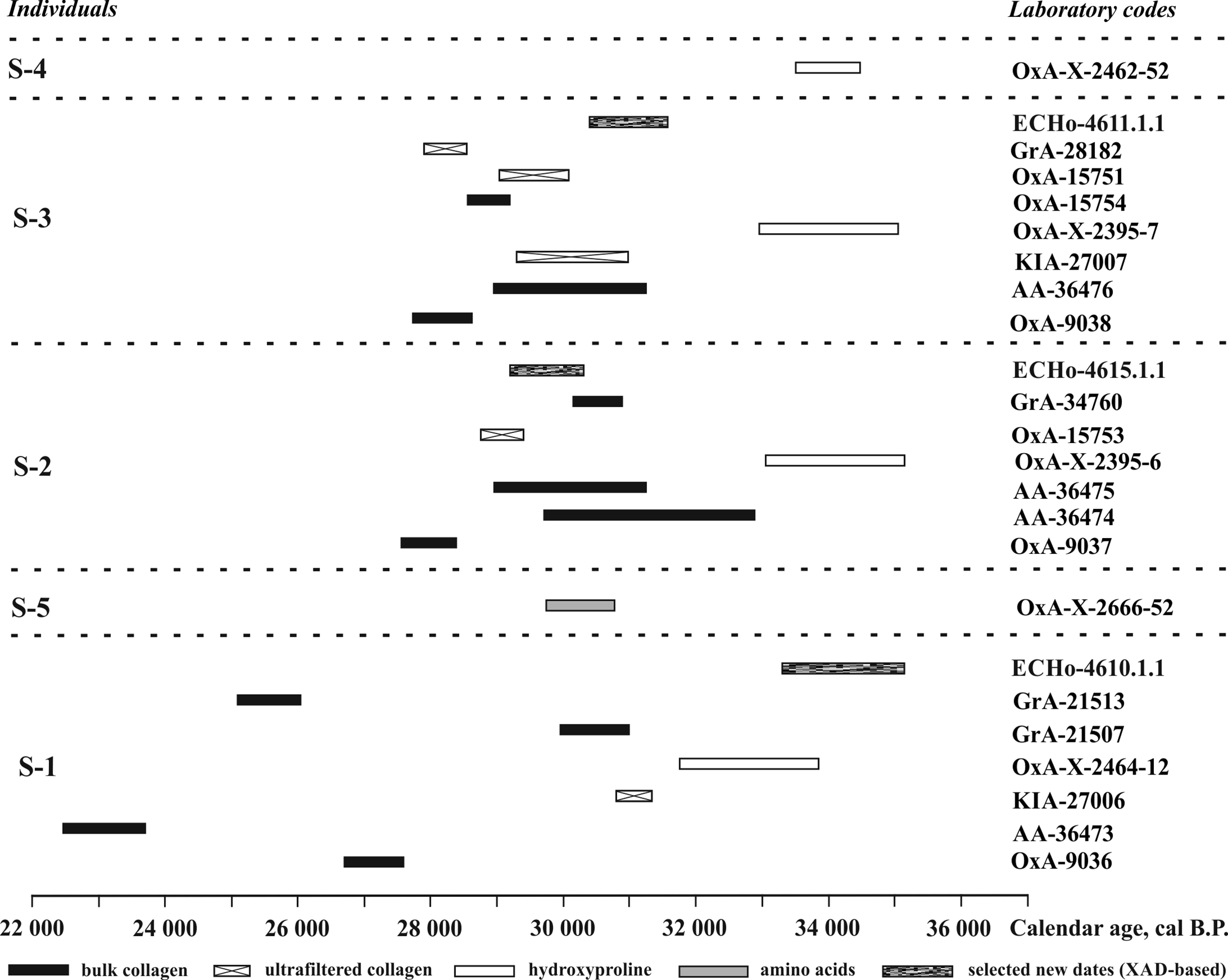
Figure 2 14C dates on Paleolithic humans from the Sungir site (see Table 1).
Re-evaluation of the site’s stratigraphy and spatial structure (including burials and habitation place), and correlation of 14C dates run on animal bones and 14C values produced for the human burials, were also timely tasks. We report here a new series of 14C dates obtained for the human bones in 2020–2022, with an analytical control of contamination by infrared spectroscopy. We also establish a reliable stratigraphy of the entire complex of Sungir, including the burials and occupation site; and the dietary patterns of the Sungir humans based on the carbon and nitrogen stable isotope values of collagen.
MATERIALS AND METHODS
Burials and Their Stratigraphy
The human burials (also called graves) at Sungir are situated in the southwestern part of the site, about 3 m apart from each other. Grave 1 contained the skeleton of an adult male (S-1). In the upper part of the grave, on a patch of ocher, a human cranium (S-5) lay on a large stone tablet. Grave 2 contained a double burial of sub-adult males (S-2 and S-3). The same grave contained a partial human femur labeled as S-4. In addition, bones of a postcranial skeleton were found in the upper part of Grave 2.
The data on ancient DNA (Sikora et al. Reference Sikora, Seguin-Orlando, Sousa, Albrechtsen, Korneliussen, Ko, Rasmussen, Dupanloup, Nigst, Bosch, Renaud, Allentoft, Margaryan, Vasilyev, Veselovskaya, Borutskaya, Deviese, Comeskey, Higham, Manica, Foley, Meltzer, Nielsen, Excoffier, Lahr, Orlando and Willerslev2017) allowed the identification of all major Sungir humans (S-1, S-2, S-3, and S-4) as males. Analysis of mtDNA genomes placed them in haplogroup U, common in the Paleolithic and Mesolithic of Western Eurasia and Siberia. Phylogenetic analysis of the Y chromosome sequence established that all these modern humans belong to the early divergent lineage of haplogroup C1a2, similar to the Kostenki 14 individual directly 14C-dated to ca. 37,990 cal BP (Marom et al. Reference Marom, McCullagh, Higham, Sinitsyn and Hedges2012; see also Kuzmin and Keates Reference Kuzmin and Keates2014). It was also found that S-1, S-2, and S-3 were not close relatives (third degree or nearer). Therefore, the double burial (S-2 and S-3) was not the joint grave for relatives (siblings), as it was previously suggested by some researchers.
We undertook a complete revision of data on the stratigraphy and distribution of artifacts and features (larger hearth pits and smaller hearths), disturbed areas during the extraction of clay for a brick factory in the 1950s, and the position of bone samples collected for 14C dating. In order to do so, all field documentation and archival records for 1958–2015, now stored at the Institute of Archaeology, Russian Academy of Sciences in Moscow, were digitized. Previous reconstructions of the stratigraphy and spatial position of artifacts at Sungir (Bader Reference Bader1978; Kaverzneva Reference Kaverzneva2004; Seleznev Reference Seleznev2004; Soldatova Reference Soldatova2019) were incomplete.
As a result of analysis, a two-dimensional map of the finds’ density (“heat-map”) was created. It allows us to see the concentrations of artifacts, bones, and other features, especially in excavation pits II and III. For Pit II with burials, we recorded in more detail the spatial distribution of different categories of finds, such as artifacts, concentrations of charcoal, red ocher, animal bones, and humic-enriched spots. Finally, we securely established the stratigraphic position of the level in the upper part of the cultural layer from which the burial pits were constructed.
Radiocarbon (14C) Dating
The 14C dates generated previously on the human and animal bones from Sungir are presented in Tables 1 and S1. The new 14C dating of the Sungir human bones (S-1, S-2, and S-3 individuals) was conducted at the Royal Institute for Cultural Heritage (abbreviation and laboratory code RICH; in Brussels, Belgium) in late 2020–early 2022; and at the National Museum of Natural History (laboratory code ECHo; in Paris, France) in early 2022 (Table 2). Materials were acquired upon agreement of scientific cooperation between the N. N. Miklouho-Maklay Institute of Ethnology and Anthropology (Moscow, Russia) and the Royal Institute for Cultural Heritage for projects in the field of Paleolithic chronology of Russia, signed on 1 October 2020.
Table 2 New 14C dates for human burials of the Sungir site obtained in 2020–2022.

a BC – bulk collagen (non-ultrafiltered); XAD – XAD-treated collagen.
b Calib Rev 8.1.0 software was used (Reimer et al. Reference Reimer, Austin, Bard, Bayliss, Blackwell, Bronk Ramsey, Butzin, Cheng, Edwards, Friedrich, Grootes, Guilderson, Hajdas, Heaton, Hogg, Hughen, Kromer, Manning, Muscheler, Palmer, Pearson, van der Plicht, Reimer, Richards, Scott, Southon, Turney, Wacker, Adolphi, Büntgen, Capano, Fahrni, Fogtmann-Schulz, Friedrich, Köhler, Kudsk, Miyake, Olsen, Reinig, Sakamoto, Sookdeo and Talamo2020). Calibrated ranges combined; values are rounded to the next 10 years.
c %C and %N are done on extracted collagen without XAD treatment.
d Not enough material left for stable isotope analysis.
At the RICH laboratory, for collagen extraction Longin’s (Reference Longin1971) method was used with additional steps. First, the samples were cleaned mechanically with a Dremel® rotary tool equipped with a diamond cut-off wheel. The porous parts of bone, which can be a source of contamination, were removed and the cortical part was selected. Between 0.5 and 1 g of a sample was placed into round-bottomed plastic tubes (16 × 100 mm) to be able to use Ezee™ syringe filters (polypropylene with a polyethylene filter and a 60–90 μm pore size) for the demineralization process. The samples were immersed in a 2.4 M HCl solution for 15 min, the HCl was removed using Ezee™ syringe filters and the samples rinsed thoroughly with Milli-Q™ water. This step also eliminates some organic contaminants (like fulvic acids), and breaks some collagen hydrogen bonds for the further solubilization in water (Longin Reference Longin1971). To remove any other contaminants such as humic acids (Arslanov and Svezhensev Reference Arslanov and Svezhentsev1993), the bone pieces were placed into a 0.25 M NaOH solution for 15 min, and rinsed with Milli-Q™ water and Ezee™ syringe filters. The pieces were again submerged in HCl at a lower concentration (0.3 M) for 5 min, in order to remove atmospheric CO2 which could have been absorbed during the previous step, and to neutralize the base if still present. After this procedure, the bones were rinsed again with Milli-Q™ water. The treated bone fragments were transferred into Duran® glass tubes, containing a pH3 HCl solution, and left at 90°C for 10 hr. Then, the solution was filtered with a Büchner funnel and a Millipore® glass fiber filter (7 μm pore size, i.e., about 525 kDa threshold), and the extracted materials were freeze-dried overnight. Prior to collagen extraction, the solvent procedure (see Wojcieszak et al. Reference Wojcieszak, den Brande, Ligovich and Boudin2020) was performed for RICH-27484.1.1 and RICH-27485.1.1. For RICH-30583.1.1, RICH-30584.1.1, RICH-30585.1.1 and RICH-30793.1.1, the samples were placed twice in toluene for 15 min in an ultrasound bath before the solvent procedure to eliminate the beeswax that was identified by FTIR on the surface of RICH-30584.1.1. The samples RICH-27985.1.1, RICH-27985.2.1, RICH-27986.2.1 and RICH-27986.1.1 were not suspected to contain contaminants and were not subjected to any solvent immersion. Sometimes, two collagen extractions were performed on different pieces of the same sample.
The collagen parameters (the yield; atomic C:N ratio; and the carbon and nitrogen contents) and the color and texture of the extracted collagen were checked to control the collagen quality (van Klinken Reference van Klinken1999). Well-preserved collagen is usually white and fluffy while degraded collagen appears brown and crystalline (Boudin et al. Reference Boudin, Bonafini, van den Brande and Berghe2017). For modern bones, the content of collagen is ca. 20% wt; after burial, the amount of collagen drops. The carbon and nitrogen contents should range between 15.3% to 47% wt, and 5.5% to 17.3% wt, respectively (Ambrose Reference Ambrose1990). The effectiveness of this collagen extraction method was proved by inter-laboratory dating of bone with a known upper age limit (Kuzmin et al. Reference Kuzmin, Fiedel, Street, Reimer, Boudin, van der Plicht, Panov and Hodgins2018). More information about the pre-treatment protocol is available in Wojcieszak et al. (Reference Wojcieszak, den Brande, Ligovich and Boudin2020).
The quality control of the pretreatment and the 14C dating was assessed using background/blank samples. The background sample for bones older than 5000 years is the last interglacial (MIS 5e) cervical vertebra of a Pleistocene bison (Bison priscus Boj.) from the Krasny Yar outcrop, Novosibirsk Province, Siberia, Russia. The fraction of the modern (percent of modern carbon, pMC) value for this bone is 0.38 ± 0.02 pMC.
All samples were transformed into graphite using the automatic graphitization system AGE (Ervynck et al. Reference Ervynck, Boudin and van Neer2018), and 14C concentrations were measured with accelerator mass spectrometry (AMS) at the Radiocarbon Laboratory of the Royal Institute for Cultural Heritage, using the 0.2 MV MICADAS AMS machine (Boudin et al. Reference Boudin, Van Strydonck, van den Brande, Synal and Wacker2015). The Calib Rev 8.1.0 software (available at http://calib.org/calib) and atmospheric 14C data from Reimer et al. (Reference Reimer, Austin, Bard, Bayliss, Blackwell, Bronk Ramsey, Butzin, Cheng, Edwards, Friedrich, Grootes, Guilderson, Hajdas, Heaton, Hogg, Hughen, Kromer, Manning, Muscheler, Palmer, Pearson, van der Plicht, Reimer, Richards, Scott, Southon, Turney, Wacker, Adolphi, Büntgen, Capano, Fahrni, Fogtmann-Schulz, Friedrich, Köhler, Kudsk, Miyake, Olsen, Reinig, Sakamoto, Sookdeo and Talamo2020) were used to transform 14C dates into calendar ages (Table 2).
At the National Museum of Natural History laboratory in Paris, samples were cleaned using XAD resin following Stafford et al. (Reference Stafford, Jull, Brendel, Duhamel and Donahue1987, Reference Stafford, Brendel and Duhamel1988, Reference Stafford, Hare, Currie, Jull and Donahue1991). Devièse et al. (Reference Devièse, Stafford, Waters, Wathen, Comeskey, Becerra-Valdivia and Higham2018) compared 14C dates prepared using both HPLC and XAD resin, and came to conclusion that these methods are currently the only ones that are able to remove environmental and museum-derived contaminants entirely.
Bone samples were demineralised in 0.2 M HCl, washed in 0.1 M NaOH for 20 min (if discoloration appeared, new NaOH was added for another 20 min), and washed again in 0.1 M HCl for 10 min. Samples were rinsed with Milli-Q water between each step. After that, bones were gelatinised in weak (pH 3) HCl acid at 90° C until dissolution, filtered using glass filter units (mesh size 10–20 μm), frozen using liquid nitrogen, and lyophillized in clean (baked out) vials. Lyophillized collagen was dissolved in 1 mL of pure (sub-boiling distilled) 6 M HCl, and hydrolyzed at 110°C for 24 hr. The hydrolysate was passed through pre-conditioned XAD columns, prepared with a filter frit at the bottom, filled with ± 100 μL (ca. 1 cm) of XAD 2 resin slurry, and covered with the top filter frit. The columns were washed with 20 mL of 1 M HCl and preconditioned with 10 mL of 6 M HCl. After the sample hydrolysate had passed through, the column was washed with one bed volume of 6 M HCl to collect any amino acids in the void space and added to the collected sample. The extracted material was dried and rinsed with Milli-Q water in small open beakers on the hotplate in the fumehood. Afterwards, collagen was transferred in 200 μL of Milli-Q water to combustion tubes using glass Pasteur pipettes, frozen in the refrigerator, and lyophillized.
For 14C dating, samples were connected to the CO2 extraction. After adding 900 mbar pure O2, collagen was combusted at 900°C for 10–20 min in the presence of a baked out silver strip (10 mg) to remove contaminants, and cleaned on the CO2 extraction line (water trap, NOx oven fitted with copper, and silver fibrewool). The CO2 gas was transferred to a semi-automated H2 reduction line using iron as a catalyst. Target samples were run alongside standards (bone blanks, oxalic acid, and phthalic acid). Graphite targets were pressed and analyzed on the same day with the ECHo-MICADAS at the Laboratoire des Sciences du Climat et de l’Environnement (LSCE) in Saclay, France. Data reduction was performed by BATS software (version 47) (Wacker et al. Reference Wacker, Christl and Synal2010). The first few scans were discarded to eliminate possible contamination of the target with ambient air between target pressing and AMS measurement. Radiocarbon ages were calculated from F14C (Reimer et al. Reference Reimer, Baillie, Bard, Bayliss, Beck, Bertrand, Blackwell, Buck, Burr, Cutler, Damon, Edwards, Fairbanks, Friedrich, Guilderson, Hogg, Hughen, Kromer, McCormac, Manning, Bronk Ramsey, Reimer, Remmele, Southon, Stuiver, Talamo, Taylor, van der Plicht and Weyhenmeyer2004), which is corrected for background and isotopic fractionation using 13C/12C. Measurement parameters such as 12C current and 13CH current were checked. Time and isobar corrections were made prior to validation. Normalisation, correction for fractionation, and background corrections were applied for each individual run by measuring the oxalic acid II NIST standard and the phthalic anhydride blanks. In order to take into account systematic errors, an error of 30% is imposed to the blank value.
Stable Isotope Analysis
Stable isotope analysis was performed at the RICH laboratory on a Thermo Flash EA/HT elemental analyzer, coupled to a Thermo DeltaV Advantage Isotope Ratio Mass Spectrometer via ConfloIV interface (ThermoFisher Scientific, Bremen, Germany) at the Department of Earth and Environmental Sciences, University of Leuven (Leuven, Belgium). Standards used were IAEA-N1, IAEA-C6, and internally calibrated acetanilide. Analytical precision was 0.25‰ for both δ13C and δ15N based on multiple measurements of the standard acetanilide. About 1 mg of collagen from each skeleton was used for this analysis.
At the National Museum of Natural History, bone collagen samples (320–380 μg each) (not treated with XAD resin) were weighed into tin capsules and analyzed with a Thermo Scientific EA Flash 2000, coupled to a Delta V Advantage isotopic mass spectrometer. Isotopic values of all samples were measured relative to the laboratory standard alanine, which has a reproducibility of 0.3% wt for N, and 0.6% wt for C. δ 13C and δ 15N values are reported relative to the VPDB and AIR, respectively. Analytical precision is ± 0.2‰ (2σ) for both δ 13C and δ 15N values.
Carbon and nitrogen stable isotope compositions were measured as the ratios of the heavy isotope to the light one (i.e., 13C/12C or 15N/14N), and are reported in delta (δ) notation as parts per thousand (per mill, ‰):
δ 13C (or δ 15N) = ([Rsample/Rstandard] − 1) × 1000
where R is 13C/12C or 15N/14N, relative to internationally defined standards for carbon (Vienna Pee Dee Belemnite, VPDB) and nitrogen (ambient inhalable reservoir, AIR).
Carbon and nitrogen concentrations in bone gelatin in relation to the bulk weight were also determined for all samples included in this study; these are referred to as the weight percentage of carbon and nitrogen (% C and % N). These quality indicators provide information on protein degradation. The atomic C:N ratio (C:Natom) of the bone collagen samples was used to classify the samples as uncontaminated or contaminated (DeNiro Reference DeNiro1985; Ambrose Reference Ambrose1990). Samples with values outside of the 2.9–3.6 range were regarded as unreliable.
For comparison of new stable isotope values for Sungir and other Early Upper Paleolithic sites in Central and Eastern Europe, all the available data are presented in Supplementary Online Materials, Table S2 (including previous δ 13C and δ 15N data for Sungir) and Figures S5–S6.
Fourier Transform Infrared (FTIR) Spectroscopy Analysis
FTIR spectra were acquired with a Bruker Vertex 70 in transmission mode with KBr pellets available at the Royal Institute for Cultural Heritage. A few milligrams of the samples were ground with KBr powder to form the pellets. A Deuterated Triglycine Sulphate (DTGS) detector was used to obtain a spectral range from 4000 to 370 cm–1 (Figures S7–S16). The spectra were recorded with 64 scans and a 4 cm–1 resolution employing the OPUS software. It was also used to apply an atmospheric compensation to remove the signal from atmospheric CO2 and H2O. Eight samples (four S-1 fragments, three vertebrae and a rib; two S-2 fragments of a rib and a vertebra; and two S-3 fragments of a rib and a vertebra) were analyzed before and after the collagen extraction, except for the S-1 fragment of a vertebra from which no collagen was left over after the 14C measurement. For the bones before collagen extraction, the surface was scraped with a scalpel for sampling. The attributions of the vibrational bands were deducted by comparing them to publications on the subject (Sato and McMillan Reference Sato and McMillan1987; Jackson et al. Reference Jackson, Watson, Halliday and Mantsch1995; Morris and Finney Reference Morris and Finney2004; Muyonga et al. Reference Muyonga, Cole and Duodu2004; Olsen et al. Reference Olsen, Heinemeier, Bennike, Krause, Honstrup and Thraned2008; Lebon et al. Reference Lebon, Zazzo and Reiche2014; Chen et al. Reference Chen, Zou, Mastalerz, Hu, Gasaway and Tao2015; Kontopoulos et al. Reference Kontopoulos, Penkman, Liritzis and Collins2019). The FTIR attribution of polyvinylacetate is described in Wei et al. (Reference Wei, Pintus and Schreiner2012).
RESULTS AND DISCUSSION
New 14C Dates and Their Evaluation
New 14C values for the two main Sungir graves with S-1, S-2, and S-3 individuals (Table 2) were run on presumably non-contaminated collagen; however, the real picture turned out to be more complicate. The quality of the collagen was controlled by FTIR spectroscopy, and collagen yield and C:Natom ratio (van Klinken Reference van Klinken1999; Brock et al. Reference Brock, Wood, Higham, Ditchfield, Bayliss and Bronk Ramsey2012). No traceable amount of contamination was found in some 14C-dated collagen using FTIR analysis, such as RICH-27985.1.1. However, the threshold for detection of consolidant using the FTIR method does not guarantee the complete removal of contamination, and this should be kept in mind. The RICH-produced dates are clearly younger compared to those previously produced on HYP (Tables 1–2).
According to our experience with the Sungir samples, a C:Natom ratio of more than 3.3 can testify the contamination by a consolidant. However, several samples with C:Natom of 3.2–3.3 returned 14C dates which were found to be unreliable and were rejected (see Table 2).
Before collagen extraction (see representative spectra in Figure S7), the FTIR spectra are characteristic of bone with the vibrational bands of the mineral and organic fractions (hydroxyapatite and collagen). Some additional bands showing the presence of calcite, quartz, and other silicates (such as clay) were also noticed, depending on the sample (on Figure S7, not all data are shown, only representative spectra). No organic consolidants were detected on the surface of the major part of bones, but it was found in the extracted collagen of most of the samples (Figures S9–10 and S13–16). For the S-2 vertebra, beeswax was identified on the surface of the bone; it was present in large quantity (Figure S8) but was not detected in the extracted collagen of RICH-30584 (Figure S9).
For the collagen analyses, the spectra of the S-1 (RICH-27985.1.1), S-2 (RICH-27484.1.1), and S-3 (RICH-27485.1.1) individuals did not show the presence of a contaminant (Figures S10–S12). S-2 and S-3 have a collagen content comprised between ca. 6–15%, demonstrating a good preservation (Table 2). For the S-1 samples (rib – RICH-27985.2.1, Figure S10; and vertebra – RICH-27986.1.1 and RICH-27986.2.1, Figure S13), contaminants were detected. The additional bands seen in the spectra compared to pure collagen spectra are highlighted in green in the tables of Figures S10 and S13. The samples belong to S-1, S-2, and S-3—RICH-30583.1.1, RICH-30793.1.1, RICH-30584.1.1, and RICH-30585.1.1—show clearly the presence of polyvinyl acetate (PVAc)-like substance in collagen (Figures S9 and S14–S16).
The wavenumbers detected can correspond to a modified PVAc with some phosphates. The pre-treatment performed with toluene and other solvents allowed to eliminate beeswax which was not detected by FTIR in the extracted collagen of RICH-30584.1.1 (Figure S9), only the PVAc-like compound with phosphates was detected in the collagen. Samples showing clear signs of contamination all have a younger age than the non-contaminated samples (Table 2). The more intense the vibrational bands of the contaminant are compared to the collagen signal, the younger the 14C dates are (Figures S10–S11). It is therefore plausible to assume that the contaminant is biobased or partially biobased because the 14C dates on contaminated collagen are younger than the 14C values of non-contaminated samples. This is possible because PVA glue is made of acetic acid (which can be biobased) and ethylene.
The FTIR spectra presented by Nalawade-Chavan et al. (Reference Nalawade-Chavan, McCullagh and Hedges2014) are similar to the ones in Figure S7, with a higher amount of calcite for their spectra of S-2 and S-3. Marom et al. (Reference Marom, McCullagh, Higham, Sinitsyn and Hedges2012) and Nalawade-Chavan et al. (Reference Nalawade-Chavan, McCullagh and Hedges2014) stated that their analyses allowed them to suspect the presence of a conservation material consisting of a polymer made of tree sap (termed kanefol) with polyvinybututyral, phenol/formaldehyde and ethanol; however, their spectra do not show the presence of these compounds. Bader (Reference Bader, Bader and Lavrushin1998) stated that the human bones were treated with a solution in ethanol of BF-2 glue (which is phenol-formaldehyde resin and polyvinyl acetate or polyvinyl butyral, dissolved in ethyl alcohol, acetone or chloroform), and butyral solution in ethanol.
The 14C dating of the S-1 individual was particularly challenging (Tables 1–2). In previous attempts, the collagen yields (when values were available) were low, from less than 1% to 0.6%, and, as a result, these 14C values, as well as the others, cannot be accepted as reliable (Table 1). In the first round of the current 14C dating campaign, a piece of the S-1 vertebra (sample ID B–2020) yielded a very small amount of collagen (0.5%) and was clearly contaminated (C:Natom = 3.6); the 14C date is 15,660 ± 52 BP (RICH-27486.1.1) (Table 2), and this is too young and therefore unreliable. Four other pieces (vertebrae and rib) were also selected. One of them (vertebra, sample ID C–2020) was found to be contaminated (Figure S13), with 14C dates of 19,751 ± 107 BP (RICH-27986.1.1) and 25,500 ± 189 BP (RICH-27986.2.1).
Out of two collagen extractions from the rib (sample ID A–2020), one was found to be contaminated (Figure S10), with a 14C date of 24,640 ± 171 BP (RICH-27985.2.1). The second extraction of collagen from this rib gave non-contaminated collagen as detected by FTIR (Figure S10), 14C-dated to ca. 26,100 ± 203 BP (RICH-27985.1.1). On the one hand, we cannot exclude the possibility of contamination by consolidant in low amount, and to be on the safe side we rejected this value as well (Table 2). On the other hand, this 14C date is very similar to an age for the S-5 cranium found on top of the S-1 burial pit—ca. 26,040 BP (Table 1). The S-5 cranium was 14C-dated using amino acids but not HYP.
The 14C date produced on XAD-treated collagen of S-1 vertebra is ca. 29,780 ± 420 BP (Table 2). This is statistically the same (χ2 (0.05) = 3.84, T’ = 2.19) as the HYP 14C age obtained for this individual (Nalawade-Chavan et al. Reference Nalawade-Chavan, McCullagh and Hedges2014), providing support that these are reliable age estimates for the S-1 individual.
The results of 14C dating for S-2 and S-3 skeletons were also not straightforward (Table 2). For S-2, three 14C values are from ca. 21,790 BP to ca. 25,910 BP (XAD). For S-3, three 14C dates are from ca. 24,930 BP to ca. 26,930 BP (XAD). Even though collagen preservation is good, these 14C ages are still considerably younger than the HYP dates performed on the same material, suggesting that contamination remnants were still present in these samples.
Correspondence to Site’s Stratigraphy and Animal 14C Dates
As for the previous reconstruction of the stratigraphic position for graves 1–2 (Bader Reference Bader1978, Reference Bader, Bader and Lavrushin1998), it was the result of an excavation method practiced by ON Bader. He was able to record the large areas enriched with humic matter and ocher (up to a size of 2 m across), according to arbitrary excavation levels, but it was more difficult to incorporate small pieces and spots of charcoal within the boundaries of burial pits into the site’s stratigraphy. Ocher, humic matter, and charcoal were concentrated in accordance with the orientation of the burial pits, starting from Horizon 2 (Figure S18). This marks Horizon 2 as the top of the burial pits. We can now establish that the graves were dug from the arbitrary level 2 or the boundary between arbitrary levels 2 and 3, that is, in the upper part of the paleosol containing a cultural layer (Figure 3, B, horizontal thick long-dashed line). Thus, the graves are relatively late objects in the stratigraphic structure of Sungir, and they were dug from the level between horizons 2 and 3 (Figure S18, see also Figure 3, B).
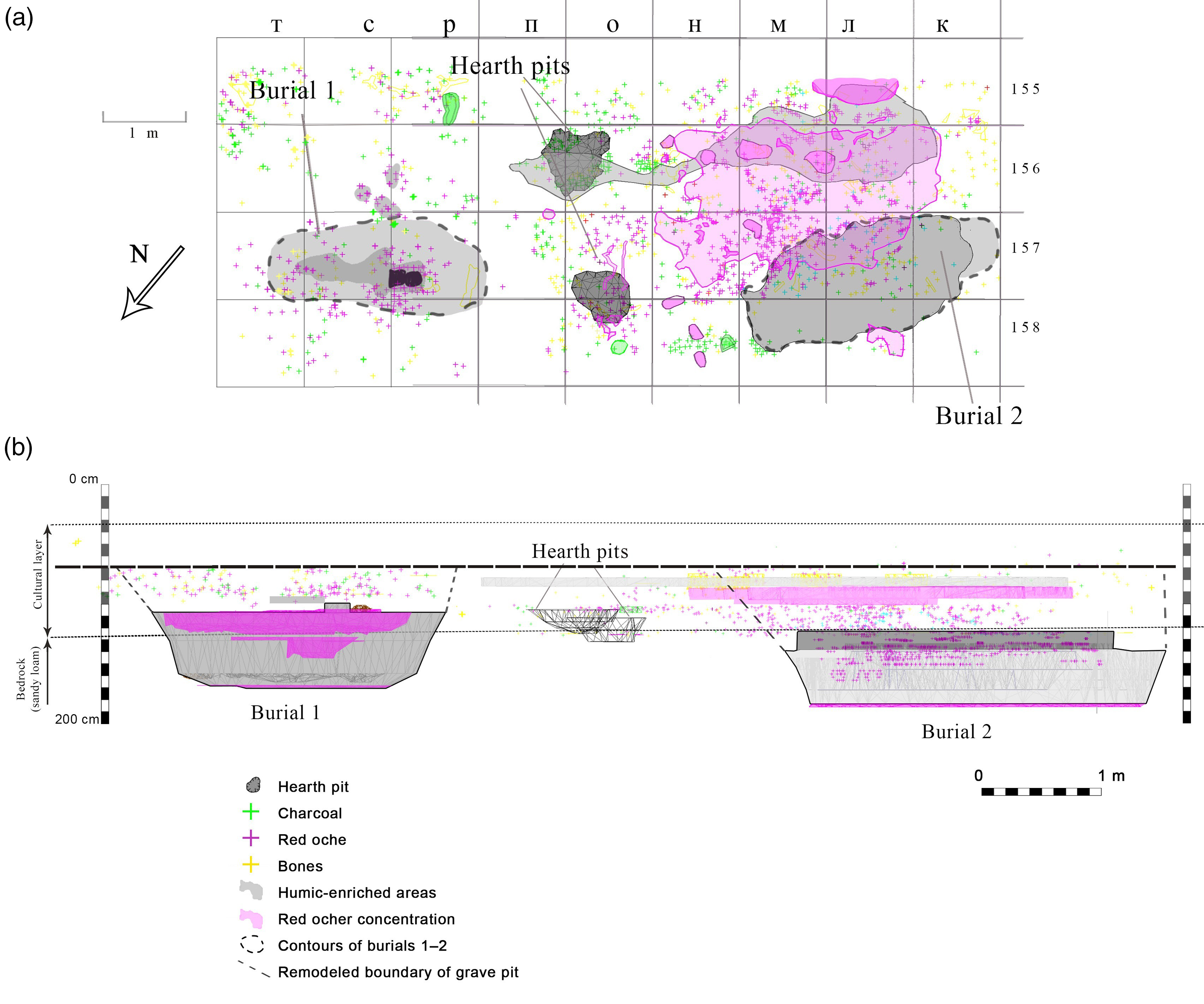
Figure 3 Spatial distribution of finds around burials (a) and their stratigraphy (b) at Sungir.
A large concentration of ocher above Burial 2 (Figure 3, A) is displaced ca. 1 m to the southeast in relation to the contour of the bottom of the burial (Figure 3, B). This shift can be explained in two ways. Firstly, it can mark the platform next to the grave pit. Secondly, such shift may be the result of the displacement of cultural remains caused by post-depositional processes (like solifluction) that occurred after the burial of the cultural layer. Deformations of this kind were recorded throughout the Sungir site (Bader and Lavrushin Reference Bader and Lavrushin1998). It is important to emphasize that these disturbances did not affect the strata underlying the paleosol (Figure 3, B, below thin dotted line). For this reason, the bottoms of the grave pits, as well as the human bones, turned out to be generally undisturbed.
In the absence of independent chronological markers—like the volcanic ash with a known age at the Kostenki 1 and 14 sites (e.g., Kuzmin Reference Kuzmin2019)—it is only possible to compare 14C dates of burials with 14C values on animal bones from the occupation layer of Sungir which can serve with some reservations as the terminus post quem. This required a complete revision of the site’s stratigraphy and spatial position of artifacts (Figures S17–S18). Based on the analysis of the stratigraphy and distribution of artifacts and faunal remains for an area near the burials (Figure S17), only some of the 14C dates run on animal bones can be correlated with the stratum related to the graves (Table S1). Based on original field documentation and reconstruction of the spatial structure of excavation pits I–III (Figures S17–S18), we checked the correspondence of information about the position of bones selected for 14C dating (Table S1). In some cases, there are clear mistakes with grid numbers and year of excavation, and we detected this for the first time.
After evaluation of the stratigraphic position of all 14C-dated animal bone samples, we found that among the early set of animal 14C values (Table S1) the specimen from Pit II, located most closely to the graves and originating from the layer below them, produced the 14C age of ca. 28,800 BP (GIN-9028) (Table S1; Figure S17). Several other samples of animal bones from excavation pits I and III, collected in the 1950s–1980s (Table S1) and presumably associated with a layer stratigraphically below the graves, are 14C-dated to ca. 26,300–27,600 BP. Unfortunately, it is not possible to establish precisely the stratigraphic relationship between all 14C-dated animal samples and graves due to incomplete recording during the excavations, and these dates can be considered only as tentatively corresponding to strata below the burials (Table S1).
The latest excavation campaign at the Sungir in 2014–2015 (Gavrilov et al. Reference Gavrilov, Voskresenskaya, Eskova, Lev, Mashchenko, Panin and Reynolds2021) gave a chance to recover animal bones associated with level below the graves. This was achieved by meticulous control of site’s stratigraphy, after the revision of Bader’s (Reference Bader1978) division of strata (see Stulova Reference Stulova2021). The new 14C dates on samples of reindeer and unidentified bones are within the interval of ca. 28,900–30,140 BP (Table S1). These particular 14C values can now serve (at least temporarily) as terminus post quem for the human burials, and are the earliest for the habitation (i.e., cultural) layer of the Sungir site.
The Different Radiocarbon Ages from the Sungir Burials
The wide ranging 14C ages obtained from the Sungir burials seem to indicate that various quantities of museum-derived consolidants were still present in some samples. Formaldehyde, which was used in the consolidation process of these samples, is known to induce cross-linking (Schellmann Reference Schellmann2007). It seems therefore most likely that some of the contamination from the consolidants had cross-linked to the collagen molecules, and it was not fully removed neither with classical ABA treatment nor with solvent washes. Only 14C dating of HYP fraction and collagen after extraction with XAD resin are able to remove cross-linked contamination, and it seems that even the XAD not able to remove all contamination in S-2 and S-3, as the HYP were still older, and more XAD resin should have been used.
We began our 14C dating campaign of Sungir humans in 2020 with expectation that vertebrae and ribs, as least informative bones for physical anthropological purposes (see Alekseeva and Bader Reference Alekseeva and Bader2000), were not treated by consolidants when discovered in the 1960s. This, however, turned out to be not true, as it is now confirmed by FTIR analysis of collagen extracted for dating. In some cases, the degree of contamination could be relatively small, and it is impossible to quantify using FTIR. In such a situation, the continuation of dating the unique human bones of Sungir—which after numerous samplings look like a “pocked lunar surface” (Pettitt Reference Pettitt2019: 1076)—does not look productive, unless the clear protocol for its analytical investigation prior to 14C dating will be established and executed. Nevertheless, there is a dearth of techniques that can measure the presence of consolidants as PVA glue with a high degree of sensitivity. The XAD treatment can be helpful in the removal of contamination from both the burial environment, such as humic acids, as well as museum-derived conservation products.
We have to keep in mind that the archaeological objects of the Sungir site were recorded at different levels. Post-depositional disturbances cannot be considered as the main reason for this. The experience from excavations (Bader Reference Bader1978; Seleznev Reference Seleznev2004; Gavrilov et al. Reference Gavrilov, Voskresenskaya, Eskova, Lev, Mashchenko, Panin and Reynolds2021) showed that the scale of post-depositional disturbances varied depending on the location. Both vertical and horizontal displacements did not lead to the destruction of artifact accumulations or shallow artificial depression-like pits in cultural layer. This conclusion is confirmed by ON Bader’s field drawings. Thus, we have reason to think that Sungir has a complex archaeological stratigraphy. Graves, charcoal, and accumulations of faunal bones are not necessarily simultaneous.
The four animal bones found below the burial layer (Table S1) date to ca. 28,800–30,140 BP, while the HYP 14C ages on the burials date to ca. 28,650–30,100 BP for S-1 and S-4 (Marom et al. Reference Marom, McCullagh, Higham, Sinitsyn and Hedges2012; Nalawade-Chavan et al. Reference Nalawade-Chavan, McCullagh and Hedges2014), The XAD 14C age of S-1 also falls within this age range. The 14C dates on the animal bones were done on bulk collagen, and it is unknown if they contain any cross-linked humic acids, which could make them slightly too young. Nevertheless, these 14C ages seem to concur that both the cultural layer and human burials date to roughly 28,800–30,100 BP. Narrowing down these ages further is difficult considering the larger errors on the 14C dates. The S-5 skull dated to ca. 26,040 BP was found above the S-1 burial and its younger age could be correct, keeping in mind the low collagen yield and high C:N ratio.
Diet of the Sungir Humans
A previous study of the human diet at Sungir, based on ratios of carbon and nitrogen stable isotopes in bone collagen, allowed researchers to establish that these people consumed mainly protein from terrestrial mammals (Richards Reference Richards, Hublin and Richards2009; Richards et al. Reference Richards, Pettitt, Stiner and Trinkaus2001; Trinkaus et al. Reference Trinkaus, Buzhilova, Mednikova and Dobrovolskaya2014). The average values for S-1–S-3 in this study (δ13C = –19.7 ± 0.2‰; δ15N = +11.8 ± 0.2‰) confirmed this conclusion (Table S2). They are similar to the stable isotopic composition for gray wolf (δ13C = –19.8‰; δ15N = +9.8‰). The average δ 15N value for reindeer (+5.7 ± 0.1‰) (Trinkaus et al. Reference Trinkaus, Buzhilova, Mednikova and Dobrovolskaya2014) (Figure S5) is within one trophic level below humans (enrichment on each level is ca. 3–5‰) who consumed reindeer protein (Drucker and Bocherens Reference Drucker and Bocherens2004; Hedges and Reynard Reference Hedges and Reynard2007). The results of stable isotope analysis for Pleistocene mammals in Eastern Europe, although not numerous, support this reconstruction (Drucker et al. Reference Drucker, Naito, Péan, Prat, Crépin, Chikaraishi, Ohkouchi, Puaud, Lázničková-Galetová, Patou-Mathis, Yanevich and Bocherens2017, Reference Drucker, Naito, Coromina, Rufi, Soler and Soler2021). Other terrestrial animals, like horse, saiga, and bison, were probably also consumed. The fish as possible source of protein should be also considered.
Zooarchaeological data are in accord with this conclusion; most of the animal bones from Sungir belong to reindeer, Arctic fox and horse; woolly mammoth and gray wolf are also frequently present (Alekseeva Reference Alekseeva, Bader and Lavrushin1998). Bones of other carnivores and ungulates—brown bear, wolverine, cave lion, saiga, and bison—are rare. Reindeer bones, often representing young animals, are heavily fragmented. Taking into account the location of the site near a large river (Figure 1), we can assume that people practiced seasonal (summer–fall) hunting of reindeer at the ford, and possibly fishing. This is similar to some Early and Mid-Upper Paleolithic sites in Central and Eastern Europe (Richards and Trinkaus Reference Richards and Trinkaus2009; Trinkaus et al. Reference Trinkaus, Soficaru, Doboş, Constantin, Zilhão and Richards2009) (Figure S6; Table S2). The human bones from these sites are 14C-dated to pre-Last Glacial Maximum (Table S2).
Issues Related to Archaeology and Subsistence of the Sungir
New data allow us to correlate the Sungir burials (Trinkaus et al. Reference Trinkaus, Buzhilova, Mednikova and Dobrovolskaya2015) with paleoclimatic data from the Greenland ice core records (Rasmussen et al. Reference Rasmussen, Bigler, Blockley, Blunier, Buchardt, Clausen, Cvijanovic, Dahl-Jensen, Hohnsen, Fischer, Gkinis, Guillevic, Hoek, Lowe, Pedro, Popp, Seierstad, Steffensen, Svennson, Vallelonga, Vinther, Walker, Wheatley and Winstrup2014). The burials can be associated with the GI-5.2 interstadial centered around ca. 32,300 cal BP, although they could be related to cold stadials GS-5.2 and GS-6. This was a later part of the MIS 3 period, with a warmer climate compared to the Last Glacial Maximum but still colder than today (Chapman et al. Reference Chapman, Shackleton and Duplessy2000; Van Meerbeeck et al. Reference Van Meerbeeck, Renssen and Roche2009), and people needed good fur clothes to protect themselves from the elements. The unique evidence of tailored garments at Sungir (see Figure S2) makes it a strong case of a developed stage in clothes manufacture, reconstructed based on two layers of beads on the lower and upper garments, which could effectively protect people from the cold environment around this period.
The new 14C dates obtained for the Sungir burials are in agreement with the reconstructed stratigraphic position of the graves. By taking into account all archaeological data, we can now say that Sungir is a complex settlement that was formed in several stages. Thus, the chronology of Sungir allows us to incorporate it into discussions of the “long” and “short” chronologies for the Upper Paleolithic in the central Russian Plain (Zaretskaya et al. Reference Zaretskaya, Gavrilov, Panin and Nechushkin2018). Scholars who are in favor of the “long” chronology suggest that a wide range of 14C dates reflects multiple occupations or rather prolonged settling (a few decades) of the sites. Those in favor of a “short” chronology select the 14C values that fit their opinion about the age of a particular cultural complex. According to them, it is hard to imagine that sites could have existed for millennia without changes of cultural traditions. Based on critical analysis of available data, we are inclined in favor of “long” chronology (see Gavrilov Reference Gavrilov, Vasil’ev, Sinitsyn and Otte2017; Zaretskaya et al. Reference Zaretskaya, Gavrilov, Panin and Nechushkin2018).
CONCLUSIONS
This study functions as a cautionary tale for researchers attempting to radiocarbon date archaeological material that was excavated many years ago. Assessing the presence of potential contamination through, for example, FTIR is essential before subjecting samples to destructive 14C dating.
The results reported in this study, together with previous data suggest that both the cultural layer with animal 14C dates and the human burials date to ca. 31,000–34,200 cal BP. The archaeological objects of the Sungir site were recorded at different levels, suggesting that Sungir has a complex archaeological stratigraphy and that graves, charcoal, and faunal accumulations are not necessarily simultaneous. The stratigraphic context of the graves allows us to conclude that they belong to the upper levels of the paleosol and cultural layer, respectively. Shallow pits that had appeared before the graves were found on the same spots as graves. In the future, it will be necessary to conduct dating of these features in parallel, using both HYP, bulk collagen, XAD-cleaned collagen, and other compounds. Quality control of the dated fractions should be performed on all of these features to ensure no contamination from either the burial environment or consolidants is present. The correspondence of the dates and the archaeological context of the samples should be critically important when assessing the reliability of the results obtained.
ACKNOWLEDGMENTS
We are grateful to M.M. Gerasimova for assistance with selecting samples for dating, and to colleagues and staff of the Royal Institute for Cultural Heritage, Institute of Archaeology, and N.N. Miklouho-Maklay Institute of Ethnology and Anthropology for laboratory assistance. The leadership of the N.N. Miklouho-Maklay lnstitute of Ethnology and Anthropology kindly agreed to participate in this study by providing samples for 14C dating. We acknowledge the support of the State Assignment of the Sobolev Institute of Geology and Mineralogy, Siberian Branch of the Russian Academy of Sciences, with funding provided by the Ministry of Science and Higher Education of the Russian Federation; and of the grant 075-15-2020-910 funded by the Ministry of Science and Higher Education of the Russian Federation. We thank F. Thil & N. Tisnérat (LSCE) and D Fiorillo (Service de Spectrométrie de Masse Isotopique du Muséum) for help with 14C dating and isotopic analysis. We are grateful to SG Keates for polishing the English.
Supplementary material
To view supplementary material for this article, please visit https://doi.org/10.1017/RDC.2022.61