INTRODUCTION
Worldwide, the number of scrapped tires that are produced is estimated to be 1 billion per year, and around 250 million car and truck tires are scrapped each year in Europe, which corresponds to 3 million tonnes by weight (Kyari et al. Reference Kyari, Cunliffe and Williams2005). Any kind of recycling may be extensively limited because of the irreversible chemical structure of the elastomer. Rubber is the main chemically cross-linking polymer of tires, which is insoluble and non-fusible, and therefore, cannot be remolded into other shapes without its severe degradation. Therefore, these scrap tires represent an important environmental problem that needs to be solved (Kaminsky and Mennerich Reference Kaminsky and Mennerich2001; Olazar et al. Reference Olazar, Lopez, Arabiourrutia, Elordi, Aguado and Bilbao2008).
Two European Commission directives were attempts by the European Union to control the management of tires. The first one is the European Commission’s End-of-Life Vehicles Directives 2000 (Directive 2000), which specified the separate collection of tires from vehicle dismantlers and boosts the recycling of tires. The second one is Waste Landfill Directives 1999 (Directive 1999) that banned land filling with tires. Consequently, these two Directives had an impact on waste tires management throughout the European Union (Kyari et al. Reference Kyari, Cunliffe and Williams2005). Directive (EU) 2018/850 which amends Directive 1999/31/EC (Directive 2018) introduced limits on landfilling from 2030 for all wastes that is suitable for recycling. In the European Union, the disposal rate of tires is close to 100% directed towards overcoming landfill problems (Sebola et al. Reference Sebola, Mativenga and Pretorius2018), approximately 58% of the tires are recycled and around 36% are used as energy recovery (ETRma 2021).
There are many ways to effectively deal with waste tires, which are based on two approaches. The first one involves the energy production by combustion or incineration of the tire; the other one is recovery of their components either by hydrogenation, liquefaction, or by the pyrolysis (Olazar et al. Reference Olazar, Lopez, Arabiourrutia, Elordi, Aguado and Bilbao2008).
Tires are a complex mixture of different materials not only rubber (ca. 40%) but also carbon black and silica (ca. 25%), metal (steel cord ca. 13% in passenger car tires and ca. 21% in truck tires) and, moreover, small organic and inorganic components such as oils and resins (6%), chemicals for vulcanization (6%), and anti-ageing agents. The composition of the tire depends on its type and, to a lesser extent, the producer (since each producer uses its own proportions). The main source of biogenic carbon in tires is the natural rubber and, to a much smaller degree, there are oils, stearic acid, rayon, natural fibers, and cotton in old tires. The ratio of natural to synthetic rubber is different in passenger car tires compared to truck tires. (Pehlken and Essadiqi Reference Pehlken and Essadiqi2005) reported that for passenger car tires it is 14% to 27%, and, for trucks, it is 27% to 14%. The U.S. Tire Manufacturers Association reported 19% to 24% for passenger car tires and, 34% to 11% for truck tires (APSnet. 2021). It is noteworthy that tires have a layered structure, different layers may contain various types of rubber. Starting from the tire part that is closest to the road surface and moving towards the center of the tire, the following elements can be distinguished (Continental 2022): (1) tread—natural and synthetic rubber, (2) jointless cap plies—nylon, embedded in rubber, (3) steel cord for belt plies—high-strength steel cords, (4) textile cord ply—rubberized artificial silk or polyester, (5) inner liner—butyl rubber, (6) sidewall—natural rubber, (7) bead reinforcement—nylon or aramid, (8) bead apex—synthetic rubber, and (9) bead core—steel wire embedded in rubber.
For the recycling of tires, there are many alternatives, like retreading, reclaiming, incineration grinding, etc., that have been used but all of them have many drawbacks. Pyrolysis is, currently, gaining new attention for tire recycling purposes; it can be considered as an unconventional method but it seems to be the most appropriate for complex materials, such as tires that cannot be remolded (De Marco Rodriguez et al. Reference De Marco Rodriguez, Laresgoiti, Cabrero, Torres, Chomon and Caballero2001). Tires are used as fuels and their effective application decreases the consumption of fossil fuels and energy costs. In this way the emission of further fossil-based carbon dioxide is also reduced, since the tires contain carbon from fossils themselves. The composition and combustion properties of tires are almost equivalent or even much improved compared to coal because of the presence of about 60–70% carbon, 6–7% hydrogen, and high calorific values (Rodríguez et al. Reference Rodríguez, Bermejo Muñoz, Zambon and Faure2017). As a result, the combustion of tires supplies heat and, in addition, this help to reduce the additional release of CO2 and SOx into the atmosphere (Krajcar Bronić et al. Reference Krajcar Bronić, Barešić and Horvatinčić2015; Rodríguez et al. Reference Rodríguez, Bermejo Muñoz, Zambon and Faure2017).
The use of biogenic fuels can prevent additional CO2 emission into the atmosphere. Until 2010, the required biogenic content in fuel was 5.75% and, according to EU directives 2009/28/EC, it was increased to 10% in 2020. Therefore, it is important to determine the biogenic content in wasted tires in order to check whether the materials and their recycling are eco-friendly. For this purpose, several methods have been used, but the radiocarbon method is well-known to provide a perfect means for distinguishing between carbonaceous material of biological and fossil origin (Krajcar Bronić et al. Reference Krajcar Bronić, Barešić, Horvatinčić and Sironić2017; Oinonen et al. Reference Oinonen, Hakanpää-Laitinen, Hämäläinen, Kaskela and Jungner2010). The dispersal of 14C on Earth from the atmosphere is governed by the carbon cycle. The radiocarbon method has a wide range of applications, due to this large scale labelling (Hajdas et al. Reference Hajdas, Ascough, Garnett, Fallon, Pearson, Quarta, Spalding, Yamaguchi and Yoneda2021). The half-life of the 14C isotope is 5730 years. Therefore, after about 10 half-lives, there is almost no 14C isotope in the sample. By detecting the ratio of 14C/12C isotopes in a sample, the radiocarbon analysis can distinguish between the bio and fossil-based carbon (Haverly et al. Reference Haverly, Fenwick, Patterson and Slade2019). Fossil carbon does not contain 14C anymore, while biomass material contains a certain amount (depending on the 14CO2 value in the atmosphere at the time of uptake). In this paper, we investigate the concentration of 14C isotope in waste tire pyrolysis products (such as rubber, oil, and recovered carbon black) to determine the bio-based carbon content. This investigation is conducted using liquid scintillation counting (LSC) and accelerator mass spectrometry (AMS) methodologies at Gliwice Radiocarbon and Mass Spectrometry Laboratory and National Laboratory for Age Determination, Trondheim, Norway. The purpose of these studies was not only a quantification of the biocarbon content in the tires, but also the testing of different measurement techniques. The research with tires from Poland was inspired by the tests performed on end-of-life tires (ELT) in France and Spain. The reported radiocarbon concentration in a passenger car, truck and, agro tires was 27–29 pMC, 50–51 pMC and, ca. 30 pMC, respectively (Rodríguez et al. Reference Rodríguez, Bermejo Muñoz, Zambon and Faure2017).
There are numerous standards that concern bio-based carbon content, which are founded on radiocarbon analysis that are applicable to different kinds of samples. Table 1 presents only those standards that could be applied to the bio-based carbon content found in the investigated samples.
Table 1 Characteristics of the standards for determining the content of biocarbon in brief.

SAMPLES
The tire rubber and the pyrolysis products, i.e., the recovered carbon black and pyrolysis oil, analyzed in this project are obtained from Contec Inc. in Warsaw, who deal with tire pyrolysis. The subsequent stages of the technological process applied by Contec Inc. can be described as follows:
-
In its first stage, the cord and steel wire are separated from the tires;
-
In the mechanical grinding process, different sizes of rubber particles are obtained: rubber dust, finely ground rubber, granules, or grits;
-
The raw material prepared using this method is s placed into the reactor via a feeder. In order to remove air from the feeder, the load is purged with nitrogen at the end of the feeder;
-
In the reactor, with the shape of a horizontal and stationary cylinder, the material is heated by a heating medium (a mixture of molten salts) with an auger, an anaerobic, and at low pressure. As a result, low-temperature hydrocarbon decomposition of the organic compounds occurs and pyrolysis gas and rCB (recovered carbon black) is produced. The temperature of the reactor is kept at 500°C;
-
Approximately 62% (by weight) of the feedstock is decomposed and received as pyrolysis gas, which is then cooled and liquefied, resulting in an oil fraction and non-condensable pyrolysis gas. The remaining mass leaves the reactor in solid form as rCB. A special system collects the recovered carbon black (rCB) from the reactor outlet. The temperature of the rCB is lowered using a conveyor cooling jacket;
-
At the recovered carbon black processing station, the material is subjected to magnetic separation with a yield of steel by the amount of 4% of the total weight of the wire (96% is recovered in the first stage); and
-
Pyrolytic gas that is collected from the reactor is directed to the process gas separation node. The fuel gas produced from the non-condensing part of the pyrolysis gas is then burned and the heat is used in the process.
Samples were delivered by Contec Inc. in three cycles with different proportions of truck and passenger car tires. Samples coming from the production cycle are numbered respectively 1, 2, and 3. The detailed samples categories are described in Table 2.
Table 2 Detailed composition of the samples. Rubber samples were taken immediately after the mechanical grinding process, rCB samples were collected after their exit from the reactor at a dedicated sampling point, oil samples were obtained from the oil tanks after passing through a filtration and sedimentation separation system.
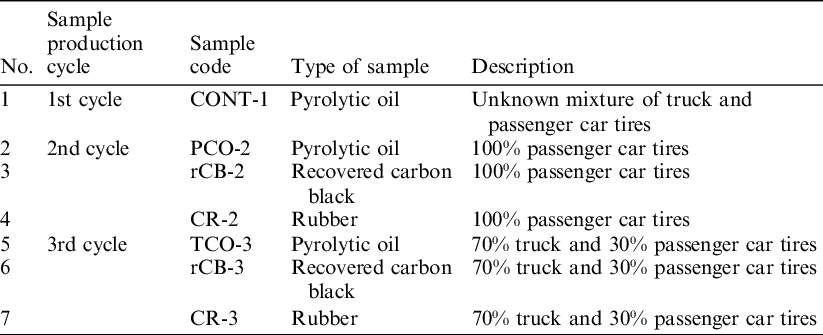
METHODS
The radiocarbon method was used because of its reliability when determining biogenic carbon fraction in materials. Benzene-LSC (GdS=GADAM Scintillation), EA-graphite-AMS (GdA=GADAM AMS), and quratz tubes CuO-graphite-AMS (TRa= Trondheim Radiocarbon AMS), are used to measure the 14C concentrations in LSC Gliwice Radiocarbon Laboratory, AMS Gliwice Radiocarbon Laboratory, and National Laboratory for Age Determination, Trondheim, Norway, respectively. Total carbon content in samples was analyzed using VarioMicroCube Elemental Analyzer in Gliwice Radiocarbon Laboratory with different masses in various experiments, mean of these values together with the uncertainties are mentioned in the Table 4. Different pretreatment methods are used for three different laboratories. The type of method and the mass of samples are mentioned in Table 3.
Table 3 Details of the pretreatment samples.

Table 4 Results on the concentration of 14C for each sample. Also reported
${\chi ^2}$
values for the samples of pyrolytic oil and recovered carbon black. The critical
${\chi ^2}$
values for four measurements = 11.34, and for three measurements = 9.21 (with confidence level,
$\alpha = 0.01$
). Biocarbon content was calculated according to the standard (EN16640 2017) for three different REF values.

On the basis of these results the variability of the 14C concentration within the different batches of tires was analyzed. The differences in the content of the 14C isotope in the passenger and truck tires were also examined.
Benzene Preparation for LSC
For the LSC measurements in the Gliwice Radiocarbon Laboratory, the samples were converted into benzene (Pazdur et al. Reference Pazdur, Fogtman, Michczyński and Pawlyta2003). Benzene was prepared by use of carbide synthesis, hydrolysis, and trimerization of acetylene for each sample. Metal reactors, in which the carbide synthesis was carried out, were mechanically cleaned before their use, rinsed with deionized water, and heated in a muffle furnace at a temperature of 700°C. Removable glass elements from the benzene production line were carefully washed with deionized water and dried at 80°C. All the chemicals were analytically pure, and the samples from Contec Inc. were not subjected to chemical pretreatment. Only the rubber samples were rinsed with boiling water.
Recovered carbon black was directly reacted with lithium to obtain Li2C2. Rubber, as well as the pyrolytic oil samples in the first step were placed into the internal reactor and then evaporated in hot lithium positioned at the bottom of the outer reactor. In the second step, the remainder of each sample from the internal reactor was transferred to the external reactor, then the reaction of synthesizing lithium carbide with a new batch of lithium was repeated. For all the samples, a reaction temperature of 700°C was used. The next steps involve hydrolysis (in which the product is acetylene C2H2) and trimerization (the product is benzene C6H6). The whole scheme for the preparation of benzene is presented in Figure 1. Benzene was dried by removal of water molecules with sodium metal and atmospheric radon was removed by keeping the benzene sample in a refrigerator for a month, since the half-life of radon is 3.8 days. For the removal of radon, we also followed a re-sublimation process for the two samples (CONT-1 and PCO-2), (Tudyka et al. Reference Tudyka, Pawełczyk and Michczyński2021) and involves a mixture of dry ice with ethanol (–78°C) or ice with salt (–21°C).
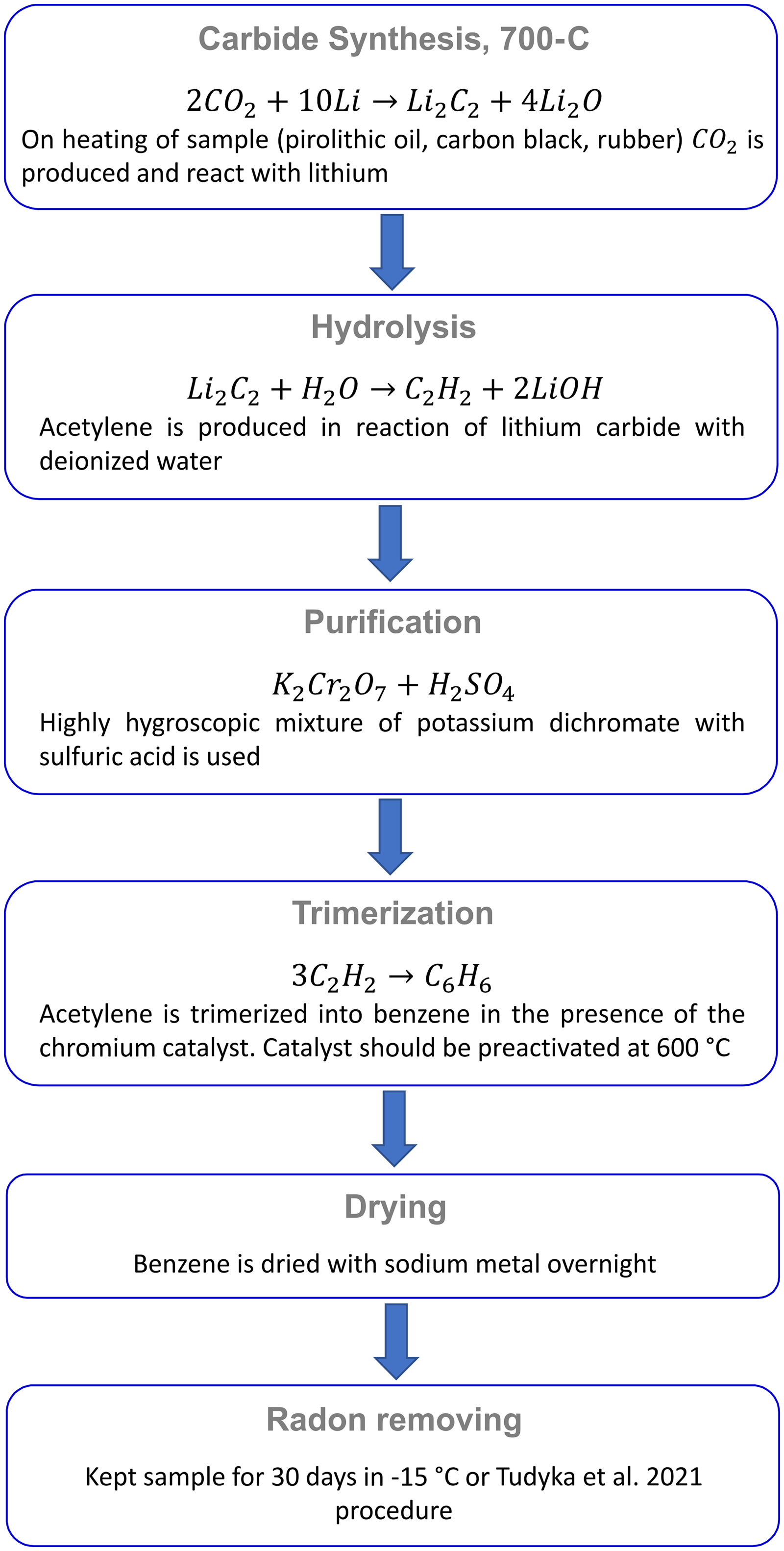
Figure 1 Benzene preparation methodology for LSC measurements in Gliwice.
The isotope ratio mass spectrometry (IRMS) is applied when calculating the standardized isotope fractionation correction and it was used for the measurement of the relative ratio of the stable carbon isotopes (13C/12C) in the samples. The catalytic combustion of the benzene samples was performed to produce CO2 with CuO. ANU-sucrose, NBS-22(oil), and IAEA-C3 standards were used for the determination of δ13C of the samples. LSC measurements were done by a Quantulus 1220TM spectrometer, butyl-PBD was used as scintillation agent with benzene (Pawlyta et al. Reference Pawlyta, Pazdur, Rakowski, Miller and Harkness1997).
Graphite Preparation for AMS
AMS measurements were done in two laboratories. All the samples were combusted, graphitized and measured at the National Laboratory for Age Determination, Trondheim, Norway. In addition, the pyrolytic oil and recovered carbon black samples were graphitized in the Gliwice Radiocarbon Laboratory and the 14C isotope concentration measurements were made at Poznan Radiocarbon Laboratory.
Graphite Preparation at Gliwice Radiocarbon Laboratory
For the AMS measurements, the graphite was prepared from samples by combustion in an Elemental Analyzer VarioMicroCube (Elementar) coupled to an AGE-3 graphitization system by IonPlus AG (Wacker et al. Reference Wacker, Němec and Bourquin2010). The radiocarbon backgrounds, and the graphite preparation method are described in (Piotrowska Reference Piotrowska2013). The method is used for the preparation of graphite targets from common samples (plant fossils, charcoal, peat, shells, and bones) and we used the same method for the oil and recovered carbon black. Tin boats were used for the solid samples, and tin capsules for the liquid samples were sealed by a special sealing press. The graphite was analyzed for its 14C concentration by Poznan Radiocarbon Laboratory (Goslar et al. Reference Goslar, Czernik and Goslar2004).
Graphite Preparation at the National Laboratory for Age Determination, Trondheim, Norway
Prior to graphitization of the CO2, the CO2 was obtained by certain combustion methods (which are different for the solids compared to the liquids). Solid samples of rubber and recovered carbon black were combusted and reduced in an Elemental Analyzer. The oil samples were frozen and then sealed with CuO in quartz tubes. After combustion in a muffle oven, the CO2 was reduced in the Fe-Zn manual reduction system. Graphite preparation methods, with backgrounds, are clearly explained in (Seiler et al. Reference Seiler, Grootes, Haarsaker, Lélu, Rzadeczka-Juga, Stene, Svarva, Thun, Værnes and Nadeau2019).
The determination of the bio-carbon content was based on the standard (EN16640 2017) Bio-based products–Bio-based carbon content–Determination of the bio-based carbon content using the radiocarbon method (Polish standard PN-EN 16640).
RESULTS
The results of the 14C isotope concentration in the pyrolysis products of the tires and their obtained by LSC and AMS measurements from both laboratories, alongside the bio-based carbon content according to (EN16640 2017), are listed in Table 4. The 14C concentration in samples is reported as the percent modern carbon (pMC), according to (Stuiver and Polach Reference Stuiver and Polach1977). Biocarbon content calculations require the adoption of the reference (REF) value, which amounts to an estimation of the age of the biochar in the tires that are subjected to pyrolysis. It should be noted, however, that this required an assumption on the age of the recycled tires. The REF value of 100% bio-based carbon in the determined year was used and expressed in pMC,based on the data reported by University of Groningen (Palstra and Meijer Reference Palstra and Meijer2018; CIO 2022). The recommended service life of tires is around 5 years, but tires are usually 10–15 years old on disposal. Account for the natural rubber used in the production of tires should also be considered, which originates from the sap of trees that are 5–25 years old. Therefore, the biocarbon content calculations were performed using REF values for 2006 = 105.4, 2011 = 103.3 (CIO 2022), and 1995 = 112 (Hua et al. Reference Hua, Turnbull, Santos, Rakowski, Ancapichún, De Pol-Holz, Hammer, Lehman, Levin and Miller2021)
DISCUSSION
Figure 2 presents comparisons of the results. The measurement results for the pyrolytic oil and recovered carbon black, using two techniques and in three different laboratories, display show good agreement (see the
${\chi ^2}$
values in Table 4). These are homogenized by the pyrolysis process and should therefore provide reproducible results. We calculated the bio-based content using different REF values and receive some differences. For each sample, the differences in the biobased carbon content values calculated for different REF values are proportional to the measured 14C isotope.

Figure 2 Comparison of the 14C isotope measurements. Measurement uncertainties are smaller than the size of the symbols used.
Pyrolytic oil from trucks, passenger cars and an unknown mixture of tires give 14C concentrations of 50.19 ± 0.22 pMC, 43.01 ± 0.58 pMC and 42.72 ± 0.31 pMC, respectively. Recovered carbon black (rCB) from car tires give 14C concentrations of 5.13 ± 0.03 pMC, while from truck tires give 14C concentrations of 6.70 ± 0.21 pMC, it is, thus, clear that some of the modern carbon from natural rubber converts to recovered carbon black in the pyrolysis process. Obviously, most of the organics that results from the decomposition of these rubbers is found in the pyrolysis oil and its non-condensing fraction, i.e., pyro gas. Nevertheless, some of the carbon molecules remain in the rCB structure as shown by the BET results (not presented in this work).
The radiocarbon concentration of the pyrolytic oil and rCB for truck tire samples are higher than those for passenger car tire since the natural rubber composition is higher in truck tires (APSnet. 2021). Pyrolytic oil samples have greater 14C concentrations when compared to rubber samples. In the oil, only the hydrocarbon parts (or such an essence) are present.
The measurement results for the rubber samples are extensively scattered and require a special discussion. For passenger car tires the 14C concentrations were found in the range 15.54–34.71 pMC, and for truck tires in the range 12.31–41.86 pMC. Most of these results are lower than the values obtained in the studies of (Rodríguez et al. Reference Rodríguez, Bermejo Muñoz, Zambon and Faure2017): 27–29 pMC for passenger car tires and 50–51 pMC for truck tires. The authors also examined different pieces of rubber from the same sample, by AMS measurements at the National Laboratory for Age Determination, Trondheim, Norway, and obtained variations in the results of the 14C concentrations ranging from 22–34 pMC in passenger car tires and 14–42 pMC in truck tires.
Small cut pieces of the rubber samples were provided for this study, as a result of a mechanical grinding process and mixing. LSC results are the average of the multiple pieces, while AMS results should be considered as point values. We expected that LSC results would converge, while the AMS results could be scattered. However, a significant spread occurs for both measurement techniques. This created suspicion that the obtained samples were not representative samples. Indeed, the co-authors from Contec Inc. explained that the rubber samples that were sent for testing mainly originated from the tread area, which explains the much lower concentration of 14C in the rubber compared to the pyrolysis oil. To obtain a representative sample, it would be necessary, for example, to sieve a finer fraction from a large batch of material. Since this action is not necessary from the point-of-view of the company dealing with tire pyrolysis, any tests can be performed for the selected type of tire by collecting sub-samples by yourself.
CONCLUSION
Three different sample pretreatment methods and two different 14C measurement methods were applied in the study. All the methods seem to be suitable and reliable for the investigation of the pyrolysis products from tires, as the measurements were performed in various laboratories and using different techniques that showed a compliance of the results. Only in the case of the measurements for the shredded tire rubber was it found that the provided samples were not representative of the entire batch of tires, which were subjected to the pyrolysis. In subsequent tests, it is planned to perform the measurements for rubber from different parts of a given type of tire. Further tests are required to determine the optimal REF value for calculating the biocarbon fraction in the type of samples that were tested.
ACKNOWLEDGMENTS
The research was supported by the SUT subsidy for the maintenance and development of research potential (14/020/BK_21/0007) and by Ministry of Science and Education through a special grant for the 14C and Mass Spectrometry Laboratory (209848/E-367/SPUB/2018/1). Rules for the allocation of funds at the Silesian University of Technology for research tasks were performed by young scientists (14/020/BKM21/0012) and PROM- International Scholarship Exchange of Doctoral students and Academic Staff (project No. POWR.03.03.00-IP.08-00-P13/18).