INTRODUCTION
Radiocarbon (14C) dating hydroxyapatite (or bio-apatite) from archaeological samples of cremated bone (CB) has become a standard procedure since Lanting et al. (Reference Lanting, Aerts-Bijma and van der Plicht2001). Results are frequently published in high-ranking journals, yet there are still unknowns in the carbon pathways during cremation and burial (Van Strydonck et al. Reference Van Strydonck, Boudin, Hoefkens and De Mulder2005, Reference Van Strydonck, Boudin and Mulder2010; Zazzo et al. Reference Zazzo, Saliège, Person and Boucher2009, Reference Zazzo, Saliège, Lebon, Lepetz and Moreau2012; Hüls et al. Reference Hüls, Erlenkeuser, Nadeau, Grootes and Andersen2010; Snoeck et al. Reference Snoeck, Brock and Schulting2014, Reference Snoeck, Schulting, Lee-Thorp, Lebon and Zazzo2016). The re-crystallization of CB makes it less susceptible to contamination from the burial environment, but perception of what sources of contaminants might still be present, influence the choice of pretreatment protocol (Van Strydonck et al. Reference Van Strydonck, Boudin, Hoefkens and De Mulder2005, Reference Van Strydonck, Boudin and De Mulder2009; Olsen et al. Reference Olsen, Heinemeier, Bennike, Krause, Margrethe Hornstrup and Thrane2008). Indeed, pretreatment protocols vary significantly between the three 14C laboratories that contributed to this study, which all have a long history of dating CB. They were among the six laboratories which participated in a cremated bone dating intercomparison (Naysmith et al. Reference Naysmith, Scott, Cook, Heinemeier, Van der Plicht, Van Strydonck, Bronk Ramsey, Grootes and Freeman2007), in which the analyzed material came from sites in Holland and Belgium, an area of mainly sandy soil with low carbonate levels (Scheele Reference Scheele2016). In that study, which produced uniform results within measurement errors, one laboratory (Kiel) pretreated the material using either an acetic acid treatment (Lanting et al. Reference Lanting, Aerts-Bijma and van der Plicht2001; Olsen et al. Reference Olsen, Heinemeier, Bennike, Krause, Margrethe Hornstrup and Thrane2008) or a leaching treatment (De Mulder et al. Reference De Mulder, Van Strydonck, Boudin, Leclercq, Paridaens and Warmenbol2007), but the other laboratories used only variations on the first treatment.
We compare methods of pretreatment, conversion to CO2 and AMS measurement of CB, employed at the Laboratory for Radiocarbon Dating (RICH) in Brussels (Belgium), the Leibniz Laboratory (KIA) in Kiel (Germany), and the Center for Isotope Research (CIO) in Groningen (The Netherlands). The aim is to test whether any differences in methods have a measurable influence on the obtained 14C results.
Different types of replicate measurements allow comparison of different steps of the laboratory protocols. Given the inter-laboratory differences in pretreatment protocols, we considered both type 1 replicates (independent dating by two laboratories of the same CB fragment (“true replicates”) or of different CB fragments from the same burial context, whose 14C ages are expected to be congruent), and type 2 replicates (conversion and measurement at two laboratories of material pretreated by one of them). Measurements of independent type 1 replicates provide an estimate of the interlab reproducibility, whereas replicating type 2 measurements of pretreated material at more laboratories provide insight into specific steps of the conversion and measuring process. A single grave was measured in duplicate within the same laboratory (KIA), and even though the results were congruent, we do not attempt to assess intra-laboratory reproducibility based on such limited data.
MATERIAL
White and fully calcined bone material was selected by visual inspection, following standards described in Olsen et al. (Reference Olsen, Heinemeier, Bennike, Krause, Margrethe Hornstrup and Thrane2008). The material came from two archaeological sites ca. 40 km apart in Southern Jutland, Denmark, both on sandy soil with low carbonate levels (Aarre ca. 8°dH, Aarupgaard ca. 10°dH). Both sites are urnfield cemeteries, spanning several phases of the Pre-Roman Iron Age, ca. 500–150 BC. There are only a few absolute dates available from this period and archaeologists instead rely on typo-chronologies (Jensen Reference Jensen2005). The cremated human remains were interred in ceramic urns and covered by small earthen mounds within a circular ditch. A minority of the graves contained metal artifacts, which can be approximately dated by seriation of typological traits.
Aarre urnfield cemetery is a large and well-documented site, with originally up to 1000 burials. Only three graves are included in this study, as most of the site was excavated in the 1950s, before cremated human remains were routinely stored by archaeological institutions (Becker Reference Becker1961; Lorange Reference Lorange, Foss and Møller2015).
Aarupgaard urnfield cemetery is an even larger site, with originally up to 1500 burials. Although there is no direct stratigraphic relationship between any of the burials, typo-chronology shows that the cemetery extended southwards over time. The site was totally excavated in the early 1970s and the cremated remains are today archived at the Laboratory of Forensic Anthropology at University of Copenhagen (Jørgensen Reference Jørgensen1975; Terkildsen Reference Terkildsen, Foss and Møller2015). Seventeen graves are included in this study, spanning the entire typo-chronological sequence (Jensen Reference Jensen2005). Both sites were sampled by a physical anthropologist, ensuring there were not multiple individuals in the selected cremations urns.
METHODS
To confirm that all bone samples were fully calcined, aliquots of powdered untreated CB samples were analyzed by Fourier-transform infrared spectroscopy (FTIR). The crystallinity index (CI) is a measurement of the re-crystallization in a sample, where high CI values indicate high burning temperatures and thus a higher degree of re-crystallization. CI was estimated as the splitting factor between the two absorption band at ca. 603 and ca. 565 cm-1 (CI = (A603 + A565)/Avalley) (Olsen et al. Reference Olsen, Heinemeier, Bennike, Krause, Margrethe Hornstrup and Thrane2008; Person et al. Reference Person, Bocherens, Saliège, Paris, Zeitoun and Gérard1995).
Overall, 58 graves were dated as part of the first author’s doctoral project, of which replicates from 16 graves are included in this study. Three rounds of selected samples from 20 graves were sent to the three laboratories involved in this study (Brussels [RICH], Kiel [KIA], and Groningen [CIO]), with a few samples replicated between laboratories. CIO uses the traditional acetic acid treatment (Lanting et al. Reference Lanting, Aerts-Bijma and van der Plicht2001), whereas RICH and KIA use variations of an acid-leaching treatment (Figure 1; De Mulder et al. Reference De Mulder, Van Strydonck, Boudin, Leclercq, Paridaens and Warmenbol2007).

Figure 1 Pretreatment protocols for cremated bone. Laboratory for Radiocarbon Dating (RICH) in Brussels (Belgium), the Leibniz Laboratory (KIA) in Kiel (Germany), and the Center for Isotope Research (CIO) in Groningen (The Netherlands).
RICH Protocol for Cremated Bone
Initially, ca. 30% by weight of each solid CB sample was leached in 1% hydrochloric acid. The bone was then ground to powder and ca. 1g was treated with 1% acetic acid (24 hr) to remove calcite. CO2 was extracted from the sample with 85% phosphoric acid (90°C). To remove any sulfur compounds, the CO2 was heated together with Ag for 30 min at 1000°C. The purified CO2 was reduced using H2 and Fe as catalyst and then pressed into targets for AMS measurements (De Mulder et al. Reference De Mulder, Van Strydonck, Boudin, Leclercq, Paridaens and Warmenbol2007; Van Strydonck et al. Reference Van Strydonck, Boudin and De Mulder2009).
AMS 14C dating was conducted using a Micadas (195.5 kV) AMS system (Boudin et al. Reference Boudin, Van Strydonck, van den Brande, Synal and Wacker2015). The resulting 14C-content was corrected for fractionation using the simultaneously AMS-measured 14C/12C and 13C/12C isotope ratios (Stuiver and Polach Reference Stuiver and Polach1977).
KIA Protocol for Cremated Bone
A 1.5g piece of CB was first crushed and washed 5 × 30 min in 0.6% (0.1M) acetic acid at room temperature to remove any calcite. After repeated rinsing in demineralized water, ca. 50% of the solid sample was leached in hydrochloric acid (10 mL 1% HCl 1h + 1.6mL HCl conc. until pH <1). After washing, drying and weighing, the sample was reacted with 60% phosphoric acid (85°C) to produce CO2. To remove any sulfur compounds, the CO2 was sealed in a quartz tube with CuO and silver wool and heated for 4 hr at 900°C. The purified CO2 was reduced at 600°C using H2 and iron powder as catalyst and then pressed into targets for AMS measurements (Hüls et al. Reference Hüls, Erlenkeuser, Nadeau, Grootes and Andersen2010).
AMS 14C dating was conducted using a HVE 3MV Tandetron 4130 AMS system (Nadeau et al. Reference Nadeau, Grootes, Schleicher, Hasselberg, Rieck and Bitterling1997). The resulting 14C-content was corrected for fractionation using the simultaneously AMS-measured 14C/12C and 13C/12C isotope ratios (Stuiver and Polach Reference Stuiver and Polach1977).
Some samples were pretreated in Groningen following the CIO protocol, but were subsequently converted to CO2 and dated in Kiel. They followed the KIA protocol from the phosphoric acid step onwards.
CIO Protocol for Cremated Bone
1.5% sodium hypochlorite is used to remove organic material from the entire CB sample (48 hr, 20°C), which was then rinsed with decarbonized water. 6% (1M) acetic acid was added to remove readily soluble calcite, absorbed carbonates and the less crystalline fractions of apatite (24 hr, 20°C) (Lanting et al. Reference Lanting, Aerts-Bijma and van der Plicht2001). The apatite was rinsed with decarbonized water to neutral pH, dried and crushed (~ 2 × 2 mm). CO2 was extracted from ca. 1800 mg apatite with 102% (1.89 kg/m3) phosphoric acid (24 hr, 25°C). To remove any sulfur compounds, the CO2 was heated with “Sulfix” particles (containing Ag2O and Co3O4; WAKO, mesh 8~20; min. 12 hr, 200°C). The purified CO2 was graphitized with iron as a catalyst and pressed into targets for AMS measurement.
AMS 14C dating was conducted using the previous HVEE 4130 2.5 MV Tandetron AMS system (Wijma et al. Reference Wijma, Aerts, van der Plicht and Zondervan1996) and the present Micadas (180 kV) AMS system, which is in operation since 2017 (Aerts-Bijma et al., in prep.). The resulting 14C-content was corrected for fractionation using the simultaneously AMS-measured 14C/12C and 13C/12C isotope ratios (Stuiver and Polach Reference Stuiver and Polach1977).
RESULTS
Initially, CIO results were often significantly younger than results from RICH and KIA, and inconsistent with the expected chronology of the Aarupgaard cemetery. Results from RICH and KIA were in good agreement with each other, and with the expected chronology, thus an anomaly was suspected in the CIO dating process, leading to offsets of 100–300 years. The anomaly was apparently unrelated to AMS measurement, as it occurred in measurements performed on both the previous HVEE AMS and the present Micadas system. Through laboratory testing at CIO and KIA, the source of the anomaly was identified as a contaminated batch of “Sulfix” in the period April 2017–March 2018 (see supplemental Appendix 1). The CIO laboratory protocol was updated following the test results, and this paper only reports results obtained using the updated protocol.
A total of 43 14C results on 20 samples are reported in this paper. Summary results on type 1 replicates are given in Table 1 and replicate sets of 14C measurements are shown in Figure 2. Summary results on type 2 replicates are given in Table 2 and replicate pairs of 14C measurements are shown in Figure 3 (see supplemental Appendix 2 for full details).

Figure 2 14C results (±1σ) on type 1 replicate dates from Aarupgaard.

Figure 3 14C results (± 1σ) on type 2 replicate dates from Aarre and Aarupgaard.
Table 1 14C results, summary statistics and χ2 test results on type 1 replicates from Aarupgaard.

* “True replicates.”
1 The absolute difference (yr) divided by its uncertainty.
2 T’(5%) = 6.0, df = 2.
3 Date used as both type 1 and 2 replicate.
4 T’(5%) = 3.8, df = 1.
Table 2 14C results, summary statistics and χ2 test results on type 2 replicates.

1 The absolute difference (yr) divided by its uncertainty.
2 T’(5%) = 3.8, df = 1.
Replicate measurements have been tested for consistency and weighted means calculated as described by Ward and Wilson (Reference Ward and Wilson1978) using the R_Combine function in OxCal (Bronk Ramsey Reference Bronk Ramsey1995). The function can be used where dates arise from the same event (within the resolution of the calibration curve, <5 yr), rather than just from the same sample. This certainly applies to the type 2 replicate measurements on apatite pretreated by CIO and type 1 “true replicates,” and we argue that it also applies to the remaining type 1 replicate measurements, as we expect different CB fragments from the same burial context to have congruent 14C ages.
Four out of six pairs of results on type 1 replicates pass the Ward and Wilson (Reference Ward and Wilson1978) χ2 test, i.e. are statistically consistent at the 5% significance level. CIO results are on average slightly older than RICH and KIA results, as are RICH results compared to KIA results. Grave 1076 results narrowly fail the χ2 test (T = 4.0, T’(5%)=3.8, ν=1), but would be regarded as consistent based on the traditional formula whereby their difference is less than 2σ (twice the uncertainty in the difference). A slight discrepancy like this might possibly reflect an inhomogeneity of 14C ages in the two dated CB fragments, caused by an uneven influence of “old-wood” from the pyre-fuel (Olsen et al. Reference Olsen, Heinemeier, Hornstrup, Bennike and Thrane2013). Grave 3869 results narrowly fail the χ2 test (T = 6.9, T’(5%) = 6.0, ν = 2), whereas if the test is limited to results from either KIA and RICH (T = 1.8, T’(5%) = 3.8, ν = 1) or RICH and CIO (T = 1.8, T’(5%) = 3.8, ν = 1) it is acceptable.
Fifteen out of 16 pairs of results on type 2 replicates are also statistically consistent at the 5% significance level. One or both dates on grave 230 must however be an outlier (T = 34.1, T’(5%) = 3.8, ν = 1, difference = 5.9σ), which cannot be explained by differences in wood-age offset, as the sample was homogenized by crushing before being split between laboratories. The KIA date fits the expected age range, whereas the CIO date is well back in the Late Bronze Age and can thus be rejected, based on the archaeological information. We do however have no technical explanation for this difference, with δ13C and %C values from both laboratories within the normal ranges.
Stable isotope values on δ13C (measured by AMS) are plotted against 14C ages in Figure 4. CIO has a slight tendency towards lower δ13C values compared to KIA, which could indicate incomplete conversion, but here probably reflects use of different AMS systems and does not account for the pattern of slightly higher 14C ages at CIO. %C results are plotted against 14C ages in Figure 5. Overall CIO has lower %C values (mean = 0.09%) than KIA (mean = 0.23%) and RICH values fall in between (mean = 0.16%, n = 2). Differences in %C do however not appear to be correlated with 14C ages.
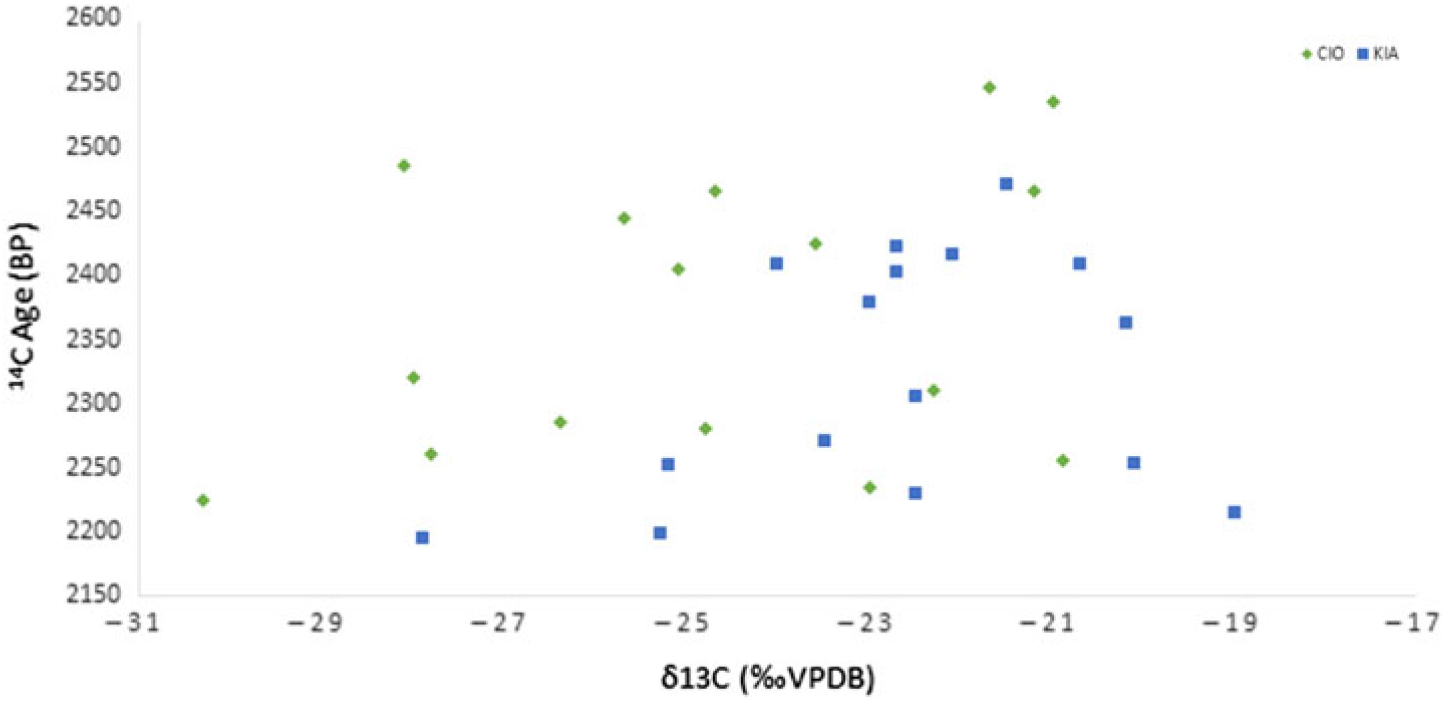
Figure 4 δ13C (AMS) values and 14C results on type 2 replicate dates from Aarre and Aarupgaard.

Figure 5 %C values and 14C results on type 2 replicate dates from Aarre and Aarupgaard.
DISCUSSION
The reported results from Aarupgaard, with the CIO date for grave 230 as an exception, are consistent with the typo-chronological phasing of the burials, based on an overall Bayesian model of the site chronology (Rose, dissertation in prep.). Results on the three graves from Aarre are consistent with the expected ages of the metal artifacts from these burials. Small wood-age offsets affecting the 14C ages of CB cannot be excluded based on typo-chronology alone.
The independent replicate pretreatment and measurement of the same CB fragment or of different CB fragments from the same burial context by two laboratories (type 1 replicates) should allow us to test whether differences in pretreatment have a measurable influence on the 14C results. Grave 3869 is the only one to be dated by all three laboratories, but while the three dates are not statistically consistent with a single 14C age, only the KIA and CIO measurements are significantly different to each other. The chi-test statistic is slightly too high for the three results to be considered accurate measurements of the same 14C age, but whether this means that the errors are slightly underestimated or whether there is a real difference between the 14C ages of the extracts (e.g. due to differences in pretreatment) is hard to answer. It is difficult to discern a clear pattern from a single case, but we find it interesting that CIO, which removed only 3% of the sample mass during pretreatment, produced the oldest date, RICH removed 36.5% and produced a younger date, whereas KIA, which removed 41.2%, produced the youngest date, which incidentally also fits the archaeological chronology best (Rose, dissertation in prep.). There is no clear linear relationship between % material removed and 14C ages and we do not know if even younger results would be obtained if even more material was removed. Applying the logic of mortar dating, the youngest (KIA) date would be the most reliable, but we do not know if this is the true date of the sample as we have too few measurements to observe the necessary plateau of 14C ages (Lindroos et al. Reference Lindroos, Heinemeier, Ringbom, Braskén and Sveinbjörnsdóttir2007). Results on the remaining five type 1 replicates are consistent (differences less than 2σ) and demonstrate 14C results on CB to be reproducible between the laboratories. Yet, we note that 14C ages appear to fit the same order of laboratories as in grave 3869, and we therefore cannot rule out that some post-depositional contamination was not completely removed under all laboratory protocols, but to investigate this further would necessitate a pretreatment protocol with stepwise etching (Van Strydonck et al. Reference Van Strydonck, Boudin and De Mulder2009).
CIO assumes apatite to be resistant to contamination and uses the least aggressive pretreatment method, removing calcite, absorbed carbonates and the less crystalline fractions of apatite (Van Strydonck et al. Reference Van Strydonck, Boudin, Hoefkens and De Mulder2005, Reference Van Strydonck, Boudin and De Mulder2009; Olsen et al. Reference Olsen, Heinemeier, Bennike, Krause, Margrethe Hornstrup and Thrane2008). KIA and RICH assume the apatite might also be diagenetically altered and consequently etch the outer 30–50% of the samples. The assumption is that any diagenetic carbon substitution must be greater near the surface of a bone. Our results cannot be used to test whether apatite can indeed be contaminated, but it is possible that any diagenesis was mainly restricted to the most soluble ≤5% of the sample, as this proportion of the samples mass was removed under all protocols. If diagenesis went beyond this ≤5%, we would expect the RICH and KIA dates to fit the archaeological chronology better than CIO dates, which is not the case (Rose, dissertation in prep.). The material comes from low carbonate burial environments, but we expect material from carbonate-rich environments might need different pretreatment (Van Strydonck et al. Reference Van Strydonck, Boudin and De Mulder2009).
All three laboratories use acetic acid to dissolve calcite, but add it at different points in the process and at different concentrations, temperatures and reaction times. After an initial sodium hypochlorite treatment, CIO treats the solid bone with acetic acid for 24 hr, thus targeting the surface of the sample along with the surface in the voids of the CB. KIA starts the pretreatment with acetic acid, but only washes the crushed bone for 5 × 30 min. RICH on the other hand uses acetic acid for 24 hr, but only after first leaching and grinding the sample. These differences in method result in varying weight losses: KIA removed less than 0.5% of the starting weights, CIO removed ≤5% and RICH removed ca. 15% (see supplemental Appendix 2 for full details). The comparatively high removal percentage by RICH, even though CIO uses a higher concentration of acetic acid, suggests that the increased surface area due to grinding is the decisive factor. These differences also suggest that much of the material dissolved during the 24 hr acetic acid treatments at CIO and RICH was apatite, not calcite.
The overall consistent results on type 1 replicate dates from the three laboratories indicate that CIO’s bleaching and acetic acid pretreatment was probably sufficient in most cases. The results do not show whether the weaker acetic acid wash in KIA would also be adequate in this case. Also, the opposite order of the acetic acid and the hydrochloric acid steps between KIA and RICH appears to have no influence on the results. Both outcomes would be expected, of course, if the samples were only contaminated by secondary calcite, without diagenetic alteration of apatite (or if diagenetically altered apatite was soluble in 6% acetic acid).
Type 2 replicate measurements were introduced to the study when anomalously younger results from Groningen were observed. Apatite pretreated by CIO was sent to KIA for CO2 extraction and AMS dating, allowing us to focus specifically on differences in the conversion and measuring processes between CIO and KIA. The results show that the differences in these processes between the Groningen and Kiel laboratories did not significantly affect the 14C results. CIO results are on average slightly older than KIA results, but differences in blank correction would account for most of this pattern; the KIA results are calculated using KIA’s blank correction, but as these samples were pretreated at CIO, the CIO blank correction is perhaps more appropriate.
There are no agreed indicators to assess sample quality when 14C dating CB, but quality nevertheless needs to be considered when judging if results are reliable. It is already common to test whether CB is fully calcined, but we suggest also reporting type of burial environment along with any other risk of contamination (ex. chalk manuring). Experimental studies have shown values of δ13C to be highly influenced, although to variable degrees, by fuel source, cremation temperature and duration (Van Strydonck et al. Reference Van Strydonck, Boudin, Hoefkens and De Mulder2005; Zazzo et al. Reference Zazzo, Saliège, Person and Boucher2009, Reference Zazzo, Saliège, Lebon, Lepetz and Moreau2012; Hüls et al. Reference Hüls, Erlenkeuser, Nadeau, Grootes and Andersen2010; Snoeck et al. Reference Snoeck, Schulting, Lee-Thorp, Lebon and Zazzo2016), but we expect δ13C values from a single site, or from sites that used similar cremation techniques, to cluster. A greater scatter may be expected for results measured by AMS rather than IRMS, but clear outliers will need further investigation. Comparing infrared spectroscopy (FTIR) and x-ray diffractometry (XRD) spectra at different stages in pretreatment, and giving more weight to results from samples whose spectra do not change much between pretreatment steps might here prove useful, but it needs to be further investigated. Inter-laboratory replication may be a useful approach to detecting contamination problems, particularly when there are significant differences in laboratory protocols; the consistency of results based on more or less aggressive pretreatments helps to validate the dates obtained.
CONCLUSION
We have replicated 14C dates of CB at three laboratories and shown differences in pretreatment, conversion to CO2 and AMS measurement to have no measurable influence on the majority of obtained results. The material comes from low carbonate burial environments and except for material from a single context any possible diagenesis was probably restricted to the more soluble ≤5% of the samples, as this proportion of the samples mass was removed under all laboratory protocols. The 14C results are reproducible and consistent with the expected archaeological chronology.
SUPPLEMENTARY MATERIAL
To view supplementary material for this article, please visit https://doi.org/10.1017/RDC.2019.70.
ACKNOWLEDGMENTS
Analyses were funded through the Center for Baltic and Scandinavian Archaeology (ZBSA)’s Man and Environment research theme and by the Deutsche Forschungsgemeinschaft (DFG, German Research Foundation - Projektnummer 2901391021 – SFB 1266) subproject G1: Timescales of Change - Chronology of cultural and environmental transformations of the Collaborative Research Center “Scales of Transformation: Human-environmental Interaction in Prehistoric and Archaic Societies.” The authors would like to thank Museum Sønderjylland and ARKVEST – Arkæologi Vestjylland for access to the material.