INTRODUCTION
Tollund Man—especially his head—is among the best-preserved human remains from prehistory. He belongs to the group of so-called “bog bodies,” which are naturally mummified bodies that have been found in acidic, anaerobic peat bogs throughout large parts of northwestern Europe, such as in Britain, Ireland, Denmark, northern Germany, and the Netherlands (e.g. van der Sanden Reference van der Sanden2013). Although bodies dating to almost all prehistoric and historic periods have been found in bogs, there is a clustering of interesting bog bodies dating to the European Late Iron Age and Roman Period (200 BC–AD 400) (van der Plicht et al. Reference van der Plicht, van der Sanden, Aerts and Streuerman2004) or in Denmark especially to the so-called Pre-Roman Iron Age (500–1 BC) and the Early Roman Iron Age (AD 1–200) (Mannering et al. Reference Mannering, Possnert, Heinemeier and Gleba2010). Tollund Man is one of these Iron Age finds.
From the time of his discovery, Tollund Man has attracted much scientific interest. Through the years he has therefore been subjected to a number of forensic and scientific investigations seeking to unravel the secrets of his death (which presumably was part of a ritual sacrifice) and to uncover as much as possible about his life and health and thereby also the living conditions of Iron Age people in general (Helbæk Reference Helbæk1950; Fischer Reference Fischer2012; Nielsen Reference Nielsen2017, Reference Nielsen2018; Zanello et al. Reference Zanello, Pallud, Jacqueline, Augias, Varlet, Devaux, Nielsen, Abi Haidar and Charlier2017). This article presents the results of the newest investigations regarding his diet and date.
Discovery and Preservation of Tollund Man
Tollund Man was found in 1950 in a raised bog in the valley of Bjældskovdal, located about 10 km west of Silkeborg, Central Jutland, Denmark (approx. 56°09’53.3”N, 9°23’34.7”E) (Figure 1a) (see Thorvildsen Reference Thorvildsen1951; Glob Reference Glob1969; Fischer Reference Fischer1980, Reference Fischer2012 for information on the discovery and subsequent investigations of Tollund Man). Today Bjældskovdal leads directly into the recreated, 360 ha Lake Bølling, but during the Iron Age the lake was much smaller and the majority of the area was covered by bog (Aaby Reference Aaby2006). The distance from Bjældskovdal to the small freshwater lake would at that time have been ca. 1 km.

Figure 1 (a) Maps showing the discovery location of Tollund Man in Bjældskovdal, a narrow valley leading into the basin of Lake Bølling (contains data from The Danish Agency for Data Supply and Efficiency). (b) Tollund Man after excavation at the Danish National Museum (photo by Lennart Larsen). (c) Close-up view of the well-preserved head of Tollund Man (photo by Arne Mikkelsen).
In 1927 and 1938, peat cutting in Bjældskovdal revealed two other bog bodies less than 100 m away from the discovery location of Tollund Man: Elling Woman and another body, which was never recovered due to a collapse of the trench cut into the peat. Like these bog bodies, Tollund Man was found by local peat cutters and, based on recommendations from Archaeology Professor P.V. Glob, Aarhus University, was transported in a block of peat to the Danish National Museum where he was excavated under more controlled conditions (Figure 1b). The local museum in Silkeborg was very keen on having the entire body preserved and put on display, but at the National Museum they deemed Tollund Man in his entirety to be too macabre a sight to be exhibited and, furthermore, they thought it would be too difficult to undertake a full-body preservation. It was therefore decided to focus primarily on conserving his well-preserved head (Figure 1c). Although today the conservation of a bog body would be approached completely differently, we are now in the fortunate situation that large parts of Tollund Man have not been treated by any chemicals but only dried. These parts—such as his bones, which despite the acidic bog environment are surprisingly well-preserved—are therefore very suitable for scientific investigations. The different parts of Tollund Man are all stored at Museum Silkeborg, where also the head is displayed together with a reconstruction of the body.
Diet of Tollund Man
How prehistoric people lived and what foods they ate represent fundamental aspects of culture that must be known if one wants to understand prehistoric societies. Analyzing the physical remains of prehistoric people is a way of obtaining this type of information. Based on analyses of the stomach contents of Tollund Man, we know that his last meal consisted of a kind of gruel primarily made up of hulled barley (Hordeum vulgare var. vulgare), flax (Linum usitatissimum), and seeds from Camelina and pale persicaria/curlytop knotweed (Polygonum lapathifolium/Persicaria lapathifolia), but which also contained the remains of a number of other plant species (Helbæk Reference Helbæk1950). However, until now, no attempts have been made to obtain information on his long-term diet. Stable isotope analyses of carbon and nitrogen in human bone collagen are standard practice in the study of past diet. The isotopic composition (δ13C and δ15N) provides information about long-term average diet and of certain dietary patterns. Unless the diet is extremely protein-deficient, bone collagen is largely produced from ingested protein. Collagen δ13C values predominantly reflect the dietary protein. δ15N values reflect only the dietary protein, as there is no nitrogen in the other nutrients (fats and carbohydrates). Thus, the combined measurement of carbon and nitrogen isotope ratios in bone collagen is very suitable for identifying dietary resources in human diets (e.g. Ambrose Reference Ambrose1993; Bonsall et al. Reference Bonsall, Cook, Hedges, Higham, Pickard and Radovanovic2004; Eriksson Reference Eriksson2004; Fischer et al. Reference Fischer, Olsen, Richards, Heinemeier, Sveinbjörnsdóttir and Bennike2007; Kanstrup Reference Kanstrup2008; Philippsen Reference Philippsen2013). Information on any possible marine or freshwater influence in the diet is, apart from contributing to the understanding of the lifestyle of ancient people, also vital for obtaining correct 14C dates as such influences require a reservoir correction of the radiocarbon (14C) results (e.g. Olsen and Heinemeier Reference Olsen and Heinemeier2009; Fernandes et al. Reference Fernandes, Grootes, Nadeau and Nehlich2015).
Since agriculture generally formed the basis of Iron Age societies, we would expect Tollund Man to show evidence of a terrestrial-based diet. However, as he was found in the lake district of Denmark it is possible that a certain amount of freshwater fish was included in the diet. Furthermore, preliminary results of a strontium isotope analysis of his femur indicate that within the last ten years prior to his death, Tollund Man, who died approximately at age 40, had been living at least 40 km away (Frei Reference Frei2017; Nielsen Reference Nielsen2018). Based on this analysis, it cannot be ruled out that Tollund Man had spent some time at the coast, which—even though a strong marine signal would never be expected— makes it even more relevant to measure the δ13C and δ15N on Tollund Man. It was therefore decided to undertake stable isotope analyses on samples from a rib and femur. The collagen in the femur reflects the average diet during adolescence and adulthood (Hedges et al. Reference Hedges, Clement, Thomas and O’Connell2007). We expect the rib to have a shorter turnover time than the femur, as it is less compact. Therefore, the isotope values of the rib collagen should be closer to those of the diet during adulthood and the last years of Tollund Man’s life.
Date of Tollund Man
One of the most pressing questions regarding Tollund Man is the date of his death. P.V. Glob assumed that Tollund Man died around the birth of Christ (Glob Reference Glob1969), but scientific dating was not conducted until 1977, when conventional 14C analyses of two samples of muscle tissue produced four dates ranging from 2260±75 BP to 2110±75 BP (K-2814A and B, Tauber Reference Tauber1980). In 1997 and 2000, new AMS dates were obtained on a rib (2345±40 BP, AAR-3328) and a piece of skin (2290±30 BP, GrA-14179) by Aarhus and Groningen, respectively (van der Plicht et al. Reference van der Plicht, van der Sanden, Aerts and Streuerman2004). Based on all the dates of Tollund Man (summarized in van der Plicht et al. Reference van der Plicht, van der Sanden, Aerts and Streuerman2004), the former director of Museum Silkeborg wrote in 2012 about Tollund Man that “we are now confident that his time of death lies between 375 and 210 BC, but further minor changes must be expected” (Fischer Reference Fischer2012:76). Although some uncertainty in 14C age determinations must sometimes be accepted when studying prehistoric objects, a desire to obtain a more precise date resulted in the decision to also 14C date the bones that were already planned to be analyzed for stable isotopes. Furthermore, the previous 14C analysis was conducted before the widespread introduction of ultrafiltration as the standard pretreatment protocol in many radiocarbon laboratories (e.g. Bronk Ramsey et al. Reference Bronk Ramsey, Higman, Bowles and Hedges2004). Ultrafiltration removes the smaller molecular weight proteins and has been shown to improve the accuracy of bone 14C age determinations (e.g. Bronk Ramsey et al. Reference Bronk Ramsey, Higman, Bowles and Hedges2004; Brock et al. Reference Brock, Bronk Ramsey and Higham2007). By analyzing different skeletal elements (i.e. rib and femur), we wish to address some considerations of the bioarcheology and remodeling of the different bones in order to see whether this can better resolve the timing of Tollund Man’s death.
METHODS
Samples were obtained from a rib and a femur, respectively, which are among the best preserved bones of Tollund Man. We followed a modified Longin procedure with ultrafiltration (Longin Reference Longin1971; Brown et al. Reference Brown, Nelson, Vogel and Southon1988; Brock et al. Reference Brock, Geoghegan, Thomas, Jurkschat and Higham2013). The surface of the bone pieces was cleaned with a scalpel, and bone minerals were dissolved in hydrochloric acid at 4°C for several days. The acid was renewed regularly until the mineral fraction was removed completely. Humic substances were removed using 0.2M NaOH at 4°C, with frequent changes of the NaOH, each step lasting several hours, until the solution stayed clear. The samples were subsequently rinsed with 0.01M HCl and gelatinized in 0.01M HCl at 58°C overnight, with an additional 3-day gelatinization afterwards. The extracted “collagen” was ultrafiltered in pre-cleaned Amicon Ultra Centrifugal Filters Ultracel − 30K, made of regenerated cellulose (REF UFC803096, LOT R4CA36137). The <30kDa fraction is usually discarded, but was dated here in addition to the >30kDa fraction (fresh type I collagen has molecular masses between 95 and 102 kDa). Both collagen fractions were weighed after ultrafiltration and freeze-drying.
The collagen was converted to CO2 by combustion in sealed evacuated quartz tubes with 200 mg CuO. The CO2 was reduced to graphite by the H2 reduction method using an iron catalyst and MgClO4 to remove the water (Vogel et al. Reference Vogel, Southon, Nelson and Brown1984; Santos et al. Reference Santos, Southon, Griffin, Beaupre and Druffel2007). The samples were 14C dated using the HVE 1MV tandetron accelerator AMS system at the Aarhus AMS Centre (Olsen et al. Reference Olsen, Tikhomirov, Grosen, Heinemeier and Klein2016). 14C dates are reported as uncalibrated 14C ages BP normalized to −25‰ according to international convention using online 13C/12C ratios (Stuiver and Polach Reference Stuiver and Polach1977). Dates in the article have been calibrated with OxCal v.4.3 (Bronk Ramsey Reference Bronk Ramsey2009) using the calibration curve IntCal13 (Reimer et al. Reference Reimer, Bard, Bayliss, Beck, Blackwell, Bronk Ramsey, Buck, Cheng, Edwards, Friedrich, Grootes, Guilderson, Haflidason, Hajdas, Hatté, Heaton, Hoffmann, Hogg, Hughen, Kaiser, Kromer, Manning, Niu, Reimer, Richards, Scott, Southon, Staff, Turney and van der Plicht2013) and are reported as calibrated ages BC.
Stable isotopes δ13C and δ15N were measured using a continuous-flow IsoPrime IRMS coupled to an Elementar PyroCube elemental analyser at the Aarhus AMS Centre (AARAMS), Aarhus University, Denmark. An in-house standard Gel-A was used as primary standard yielding ±0.2‰ and ±0.3‰ for carbon and nitrogen analysis respectively. Further, secondary in-house and international standard were used to check the normalization to the VPDB and AIR scale.
FTIR spectra were obtained using an Agilent Technologies Cary 630 ATR-FTIR instrument. Scans were performed in the range from 4000–650 cm−1 with a resolution of 2 cm−1. Each spectrum is an average of 64 scans. The spectra are baseline corrected and C/P and N (Amide I)/P ratios are calculated using the peak heights of 1630 cm−1 (“N”, Amide I), 1450 cm−1 (“C”, CO3 2−) and 1030 cm−1 (“P”, PO4 3−).
RESULTS AND DISCUSSION
The results obtained on Tollund Man’s bones are listed in Table 1. Here, we will start by discussing the quality criteria for bone collagen and the effects of ultrafiltration. This is followed by a discussion of the results of the stable isotope analysis and the 14C analysis.
Table 1 Results of the measurements on previous and this study’s samples of Tollund Man. The extracted bone collagen of the new samples (AAR-26386 and AAR-26387) was ultrafiltered. Both the >30kDa and the <30kDa fractions were analyzed. The >30kDa fraction of AAR-26386 was dated twice (1a and 1b), and the weighted mean value is presented under AAR-26386.1. For combined results a reduced χ2 test (95.4% confidence level) is given as X≤Y. The χ2 is passed if and only if X≤Y (Bevington and Robinson 2003).

* Dual inlet analysis, uncertainty ±0.05‰. n/a: information not available.
Pretreatment
Ultrafiltration separates the gelatinized collagen into two fractions determined by the cut-off rigidity (here 30 kDa) of the applied ultrafilter and was introduced by Brown et al. (Reference Brown, Nelson, Vogel and Southon1988). The <30 kDa fraction is normally disregarded as it is more likely to contain possible contaminants such as short-chain proteins from degraded collagen, salt products, fulvic acids and plant- or soil-derived amino acids (Brock et al. Reference Brock, Bronk Ramsey and Higham2007). Contaminating substances such as humic or fulvic acids can attach to the ends of the collagen fibers and larger proportions of contamination are therefore expected for the smaller molecules (cf. van der Plicht et al. Reference van der Plicht, van der Sanden, Aerts and Streuerman2004). The higher molecular weight protein is on the other hand hoped to only represent collagen originating from the bone sample itself (Brown et al. Reference Brown, Nelson, Vogel and Southon1988; Bronk Ramsey et al. Reference Bronk Ramsey, Higman, Bowles and Hedges2004). Thus, in most cases only the high molecular weight fraction (>30kDa) is used for 14C analysis, whereas the low molecular weight fraction is discarded.
The >30kDa collagen yield of Tollund Man’s bones (Table 1) is very similar to that of fresh bone at 22 wt % collagen (van Klinken Reference van Klinken1999). This is much higher compared with bones from different Danish Roman Iron Age Burial sites where yields in the range 0.4–13% have been reported (Figure 2; Jørkov Reference Jørkov2007). We suspect that part of the minerogenic fraction of the bones was dissolved in the acidic bog environment, thereby inflating the apparent collagen yield. The carbon (42.7%, >30 kDa) and nitrogen (13.5%, >30 kDa) weight percent fall within the expected range for good quality collagen (van Klinken Reference van Klinken1999). The carbon weight percentage is fairly close the expected collagen carbon content of fresh bones at 47%, whereas the nitrogen weight percentage is much lower than would be expected from fresh bones with a collagen weight percentage of 17% (Ambrose and Krigbaum Reference Ambrose and Krigbaum2003). The average C/N ratio of the >30 kDa fractions is 3.7, falling just outside the range between 2.8 and 3.6, which is considered to reflect well-preserved collagen (DeNiro Reference DeNiro1985). The higher C/N ratios can be explained as being caused by either additional carbon in the samples (i.e. contamination) or a preferential removal of nitrogen. We speculate that microorganisms in the nutrient-poor bog fed on the nitrogen from the bones. This could increase the δ15N values slightly, as microbes preferentially break the weaker bounds containing the lighter isotopes leaving behind an enriched 15N residual, i.e. bone collagen (Talbot Reference Talbot2001).
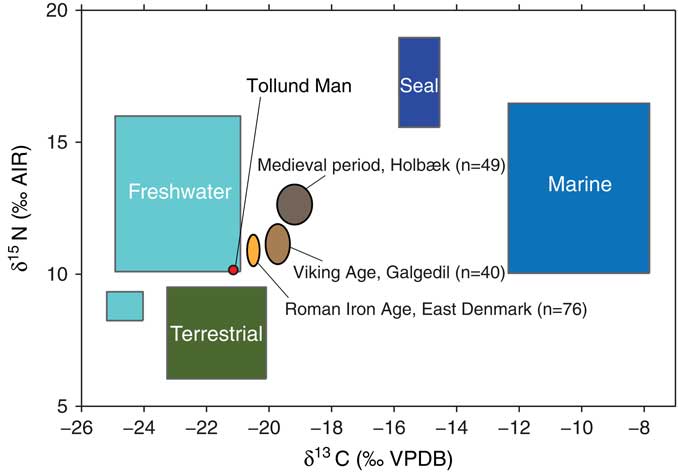
Figure 2 The Tollund Man isotope data compared with other published Danish isotope studies. The food item boxes are from different Mesolithic and Neolithic sites (Fischer et al. Reference Fischer, Olsen, Richards, Heinemeier, Sveinbjörnsdóttir and Bennike2007). Isotopic values on human bones from the Galgedil site on Funen (Kanstrup Reference Kanstrup2008), east Danish Roman Iron Age sites (Jørkov Reference Jørkov2007) and from a medieval cemetery in Holbæk on Zealand (Jørkov Reference Jørkov2007) are also plotted for reference. The ellipse axis denotes ±1σ and the center value is average of all humans for each site respectively.
FTIR spectra suggest that both fractions contain collagen (Figure 3b). However, the carbon and nitrogen weight percent values of the <30kDa fractions are much smaller than those of the >30kDa fractions, 31.0% and 10.3% for carbon and nitrogen respectively. These weight percentage values indicate poorly preserved collagen (van Klinken Reference van Klinken1999). Furthermore, the yield for the <30 kDa fraction is 12.1%. The <30 kDa fraction C/N ratios of 3.6 are compatible with a protein origin and the lower pretreatment yield indicates the gelatinized collagen contains only a minor fraction of low molecular weight proteins. The FTIR inferred average C/P and N/P ratios for the >30 kDa fraction are 2.83±0.02 and 6.04±0.04 respectively, whereas the low molecular weight fraction yields an average C/P ratio of 2.72±0.03 and an average N/P ratio of 5.4±0.4 (Table 1). Even though these differences in ratios are small it appears that the high molecular weight fraction contains less phosphate and/or higher carbonate and nitrogen (Amide I). Phosphate is dominantly derived from the bio-apatite fraction of the bone and hence a low phosphate content is preferred in the extracted collagen fraction as this would indicate better removal of the inorganic bone fraction. The lower carbon and nitrogen weight percentages together with the C/P and N/P ratios, however, suggest that the low molecular weight fraction is constitutionally different from the collagen fraction contained in the >30 kDa fraction (Table 1). As the <30k Da fraction usually is discarded, it is difficult to find references for comparison.

Figure 3 (a) 14C ages of the femur and rib samples together with previously published skin and bone AMS 14C ages. The weighted average 14C ages for the >30kDa fractions (2327±18 BP) and <30kDa fractions (2384±20 BP), respectively, are also shown together with the weighted average of the skin 14C age (GrA-14179) and the >30kDa 14C ages (AAR-26386.1 and AAR-26387.1) yielding 2318±15 BP. (b) FTIR absorbance spectra for the >30kDa and <30kDa fractions. For reference the >30 kDa laboratory background whale bone FTIR spectrum is also shown (BGD bone).
Interestingly, the isotopic signature of the low molecular weight fraction (<30 kDa) appears to be similar to bone collagen (>30 kDa) isotopic values (Table 1). If anything, the δ13C values of the <30 kDa fraction are slightly higher by ca. 0.9‰ than the values of the >30 kDa fraction, while the δ15N values of the <30 kDa fraction are lower by c. 0.3‰ than those of the >30 kDa fractions. Given the analytical precision of ±0.2‰ the δ13C difference on 0.9‰ is probably significant and suggest the low molecular weight carbon to be slightly enriched in 13C. As humic and fulvic acids have an isotopic δ13C signature closely resembling the plants from which they are derived, it would be expected that terrestrially derived humic and fulvic acids have δ13C values lower than −25‰. Thus, it is unlikely that the low molecular weight fraction is contaminated by humic or fulvic acids. Furthermore, humic and fulvic acids would probably have increased the carbon weight percentage if they were present in significant amounts. Likewise, a large amount of terrestrially derived plant amino acids in the low molecular weight fraction would probably have lowered the δ15N values contrary to what is observed. Aquatic plants, on the other hand, may assimilate bicarbonate, which would be 13C enriched and therefore amino acids derived from bicarbonate assimilating plants would be a probable source to the low molecular weight fraction. δ15N values in aquatic plants are very variable and are not unlikely to have values around 10‰ (Talbot Reference Talbot2001). Of course, it is also possible the low molecular weight protein is dominantly derived from the bone collagen and that the breaking to smaller molecules is caused by microbial activity. The subtle difference in the δ13C values would then have been caused by microbial metabolic fractionation, though it appears peculiar if microbial activity would leave the 15N values unchanged. However, the data presented here are too few to make any definitive conclusion on the origin.
The 14C ages of the <30 kDa fraction are older than the >30 kDa fractions (Table 1). The weighted average of the femur and rib <30 kDa fraction is 2384±20 BP and for the >30 kDa fraction the weighted average is 2327±18 BP. Thus, the 14C age difference between the two fractions can be calculated to 57 ± 27 14C years (Figure 3a). This corresponds to 2.1 standard deviations away from a 0 year difference, in other words, there is less than 5% chance that the two fractions are equal. Even though this difference is small, it suggests that the low molecular weight fraction contain foreign protein of a slightly different age than the bone collagen contained in the >30 kDa fraction.
The isotope data demonstrate the possibility of contamination of the <30kDa fraction and the 14C ages suggests that this might result in older dates. Thus the low molecular weight fraction (<30 kDa) appears different from the collagen fraction (>30 kDa) we will refrain from using the <30 kDa fraction in any further analysis. Furthermore, this may require caution when using previously published 14C age where the pretreatment methods may have included the low molecular weight fraction. However, due to the small 14C age difference between the two fractions this effect will be difficult to detect statistically.
Stable Isotope Analysis
The δ13C values of the bone collagen (>30 kDa) from the femur and the rib are −21.5 ± 0.2‰ and −21.0±0.2‰, respectively (see Table 1). The δ15N values are 10.3 ± 0.2 ‰ for the femur and 10.0±0.2 ‰ for the rib. Because the δ13C and δ15N values agree within uncertainties we cannot determine a significant shift in average diet during adolescence and adulthood of Tollund Man. The δ15N value of the femur is only 0.25‰ higher than that of the rib. Generally, a higher δ15N value would indicate a higher trophic level, e.g. increased meat or aquatic diets. However, the difference in δ15N values in the rib and femur is considered too small to indicate significant changes in the diet; usually, the shift is about 3–5‰ from one trophic level to the next (cf. Schoeninger and DeNiro Reference Schoeninger and DeNiro1984; Ambrose Reference Ambrose2000). We can therefore conclude that the average diet reflected in the femur is the same as that reflected in the rib.
The δ13C values of ca. −21‰ in Tollund Man indicate a purely terrestrial diet. Thus, nothing suggests that Tollund Man exploited marine resources during the last years of his life, in contrast to the Viking Age and Medieval individuals that are included as references in this study (Figure 2). From the Viking Age and Medieval periods, fish consumption is attested from various sources, and this fish intake results in a greater variation in δ13C values from individuals of this time (Jørkov Reference Jørkov2007; Price et al. Reference Price, Prangsgaard, Kanstrup, Bennike and Frei2014). The δ15N values of ca. 10‰ in Tollund Man are higher than in Neolithic individuals (ca. 9.6‰, Fischer et al. Reference Fischer, Olsen, Richards, Heinemeier, Sveinbjörnsdóttir and Bennike2007) who relied on agricultural products, but lower than in inland Maglemosian (Mesolithic) individuals (ca. 10.7‰, Fischer et al. Reference Fischer, Olsen, Richards, Heinemeier, Sveinbjörnsdóttir and Bennike2007) who utilized freshwater fish. Although the diet of Tollund Man may have included some freshwater fish, the slightly enhanced δ15N values compared to Neolithic individuals are probably best explained as being caused by the consumption of crops grown on manured fields (cf. Bogaard et al. Reference Bogaard, Fraser, Heaton, Wallace, Vaiglova, Charles, Jones, Evershed, Styring, Andersen, Arbogast, Bartosiewicz, Gardeisen, Kanstrup, Maier, Marinova, Ninov, Schäefer and Stephan2013). This interpretation would be in accordance with the evidence of manuring that we find in the field systems and in isotope values of carbonized grain from this period (Kanstrup et al. Reference Kanstrup, Holst, Jensen, Thomsen and Christensen2014; Nielsen and Dalsgaard Reference Nielsen and Dalsgaard2017). The fact that the δ15N values of Tollund Man are lower than those in the samples from later periods is probably partly because of even more intensive manuring practices in these periods as indicated by higher phosphate levels in the arable soils (e.g. Christensen Reference Christensen1997; Dalsgaard et al. Reference Dalsgaard, Karlsen and Larsen2000).
14C Dating
In an attempt to narrow down the date of the death of Tollund Man, we decided to evaluate whether some of the previous 14C dates could be combined with the new ones. Since conventional 14C dates are less precise than AMS dates, and since the sample treatment and possible contaminations are not known in detail, the conventional 14C dates obtained in 1977 were excluded (K-2812A and K-2814B, Figure 4). When taking a closer look at the four AMS dates (GrA-14179, AAR-3328, AAR-26386.1, and AAR-26387.1 in Table 1 and Figure 4), it becomes evident that the old date on the rib sample (AAR-3328), obtained without ultrafiltration, is about one standard deviation older than the newer AMS dates. This could possibly indicate contamination with older substances, as the indicated by the <30kDa 14C ages presented here. Therefore, we also excluded AAR-3328 from further analysis. The 14C age of the skin sample should be more indicative of time of death due to the lower turnover rate (GrA-14179). This date appears reliable and was therefore included in the final calculation of the 14C age of Tollund Man.

Figure 4 Calibrated probability distributions for selected previous published 14C ages together with femur and rib (>30kDa) samples from this study. The calibrated probability distribution of the weighted average is also shown as this is considered to be the best age estimate for Tollund Man. The modeled own age calibrated probability distribution is corrected for the bones’ inherent age. We assume that the carbon in the ribs is on average 5±3 calendar years old and in the femur 12±5 calendar years (statistics are taken from the OxCal combine function output). See text for details. ABA denotes acid-base-acid pretreatment.
The three AMS dates (GrA-14179, AAR-26386.1, and AAR-26387.1) agree well, and the weighted mean is therefore calculated to 2318±15 BP (Table 1, Figure 4). This narrowed the date of Tollund Man’s death to the period 405–380 cal BC at the 95.4% confidence interval (reduced χ2 test: 0.58≤3.00, Table 1). Such a precise date, covering an interval of only 25 years, is extremely rare when studying prehistoric human remains. It should be noted that including AAR-3328 in the weighted average yields a 14C age of 2320±15 BP (reduced χ2 test: 0.53≤2.60) corresponding to a negligible change of the result.
Because the carbon turnover rate in bones is much lower than skin, we tested the effect of the bones having an age offset compared to the skin. As an example, we assume that the carbon in the femur is on average 12±5 calendar years old and the carbon in the ribs 5±3 calendar years based on e.g. the work by Hedges et al. (Reference Hedges, Clement, Thomas and O’Connell2007). This was implemented using OxCal’s offset function, e.g.:
R_Date(“AAR-26386_.1 femur”, 2330, 23)
{
Offset(12,5);
};
As a result, the average shifted towards a slightly younger age (Figure 4). However, the shift is only very small. Including the uncertainties associated with collagen-turnover-corrections of the dates, we consider the 405–380 cal BC date to be the most reliable date of Tollund Man’s death. Thus, instead of applying an uncertain, speculative correction that almost does not change the date, we choose to report the date without the correction for collagen turnover.
Future Perspectives
The new date of Tollund Man makes one wonder whether new 14C analyses of Elling Woman, found in the same bog as Tollund Man, could also refine the date of this bog body. Elling Woman is not as well-preserved as Tollund Man and so far AMS 14C dates have only been undertaken on her fur cape. The cape has been dated to 2195±40 BP (AAR-3415) and 2210±30 BP (GrA14315), i.e. 362–201 cal BC (95.4% confidence interval) when using a weighted mean of 2205 ± 24 BP (χ2 test: 0.09≤3.84). Thus, Elling Woman seems to have been placed in the bog slightly later than Tollund Man. However, a date of 2350± 50 BP (GrA-15637), calibrated to 746–232 cal BC (95.4% confidence interval), has also been obtained (van der Plicht et al. Reference van der Plicht, van der Sanden, Aerts and Streuerman2004). It would therefore be interesting to redate Elling Woman in order to find out whether she was contemporary with Tollund Man, whether the memory of the sacrifice of Tollund Man still existed when Elling Woman was executed, or whether the two sacrifices were performed several generations apart. Ideally, new dates would be conducted on her hair or other body parts that have not received conservation treatment (with among other things organic oils) as the cape has. If Elling Woman is in fact younger than Tollund Man, however, we may not be able to narrow the date of her death any further due to the shape of the calibration curve.
CONCLUSION
The new 14C dates of Tollund Man have greatly increased the precision of the calibrated age. This illustrates that it is worthwhile to 14C date old finds again, especially if laboratory processes have been improved. In this case, ultrafiltration is a useful step in the pretreatment sequence as it appears to remove 14C-depleted contamination. The precise calibrated age of 405–380 cal BC (95.4% confidence interval), an interval of only 25 calendar years, was possible thanks to a combination of laboratory pretreatment, machine precision, averaging three 14C samples, and encountering a favorable interval on the calibration curve.
ACKNOWLEDGMENTS
Conservator Lars Vig Jensen is thanked for extracting the bone samples from the femur and rib of Tollund Man. We would also like to thank Ken Ritchie for improving the English of an earlier version of this article and the anonymous reviewers and the editors for their valuable comments. Bente Philippsen was supported by AU STAR – Science and Technology in Archaeological Research and by the Danish National Research Foundation under the grant DNRF119 – Centre of Excellence for Urban Network Evolutions (UrbNet).