INTRODUCTION
Archaeological sites in Arctic North America and Greenland are rich in sea mammal remains. Sites in the region encompassing Foxe Basin, northern Hudson Bay, and Hudson Strait are particularly rich in walrus (Odobenus rosmarus) remains, and many of them contain little or no terrestrial mammal remains. Despite excellent preservation, many sites go undated because of a pervasive distrust of dates on marine materials among archaeologists. The distrust arises from inadequate documentation of the size and regional variation of the marine reservoir effect on radiocarbon (14C) dates across the Arctic. This effect, on the order of centuries, is large with respect to site ages (less than 5000 years). For example, using the data presented by McNeely et al. (Reference McNeely, Dyke and Southon2006), Coulthard et al. (Reference Coulthard, Furze, Pieńkowski, Chantel Nixon and England2010) calculated average ΔR values (14C yr BP) with some minor modifications to the original geographic boundaries as presented in the McNeely et al. dataset: 65±60 yr (Hudson Strait), 110±65 yr (Hudson Bay), 145±95 yr (Ungava Bay), 150±60 yr (SE Baffin Island), 220±20 yr (NE Baffin Island), 310±90 yr (Foxe Basin), 335±85 yr (NW Canadian Arctic Archipelago), and 365±115 yr (James Bay). Following an early period of indiscriminate dating of various materials and inconsistent normalization of dates for isotopic fractionation among laboratories, McGhee and Tuck (Reference McGhee and Tuck1976) exposed inconsistencies in archaeological site age assessments that resulted when dates on terrestrial materials and uncorrected dates on marine materials were considered to be equally valid. The subsequent decision to avoid the dating of marine materials by most archaeologists, while perhaps rendering site ages less problematic, has hampered the ready dating of many sites, especially those not extensively excavated, resulting in poorly controlled chronologies in many instances.
A marine reservoir age is essentially the residence time of CO2, and the bicarbonate formed from it, in the ocean. It is the length of time spent in transit in the ocean between the time of absorption of the gas from the atmosphere and its ventilation back to the atmosphere at sites of ocean upwelling. During this transit time, the radioactive isotope of carbon (14C) decays, imparting an “apparent age” to the carbon in the water. The global average surface ocean (0–75 m depth, which mixes by wave action) reservoir age was about 400 years in AD 1830, the value R in 14C calibration programs for marine samples (Stuiver and Braziunas Reference Stuiver and Braziunas1993a), but it varied through time because of variable 14C production rates. Therefore, a marine reservoir age refers to the difference in the 14C content of a particular area of the surface ocean and the atmosphere at a specific point in time. Temporal variations in 14C content, including that caused by nuclear testing, are much larger in the atmosphere than they are in the ocean (Stuiver and Braziunas Reference Stuiver and Braziunas1993b) because new 14C is produced only in the troposphere and stratosphere. As a result, a simple subtraction of a single value for marine samples of any type will not accurately reflect the age of the samples. The time variance of R in the ocean is calculated in the Stuiver and Braziunas (Reference Stuiver and Braziunas1993a) carbon cycle model based on the tree-ring derived variance of 14C levels in the atmosphere. R also varies regionally due to oceanographic processes, including downwelling, upwelling and the seasonal duration of sea ice cover (Southon et al. Reference Southon, Kashgarian, Fontugne, Metivier and W-S Yim2002; Ascough et al. Reference Ascough, Cook and Dugmore2005). The regional departure from R is termed ΔR. Prior to 2006 there were only a limited number of measurements of reservoir ages available from the Canadian sectors of the Northwest Atlantic and Arctic Oceans (Mangerud and Gulliksen Reference Mangerud and Gulliksen1975). Then McNeely et al. (Reference McNeely, Dyke and Southon2006) used a large sample of marine mollusks to define the reservoir age and ΔR in Canadian waters. They established mean values for 12 major oceanographic regions based on coastal current configurations. These results showed a decreasing cascade of ages from the Pacific coast, through the Arctic Archipelago, to the Atlantic Coast. The rounded value of ΔR for the Foxe Basin region (including northern Hudson Bay), where surface water is derived mainly from the Arctic Ocean, is 295±130 yr based on 34 samples (see below).
Marine mollusk shells are ostensibly the most straightforward materials to use in assessing reservoir ages, because bivalve mollusks are non-migratory and the carbonate in their shells is deposited in isotopic equilibrium with the bicarbonate in the surrounding water. The shells therefore capture the local reservoir age. Measuring reservoir ages should be a simple task of collecting shells and 14C dating them to determine “apparent ages.” Unfortunately, nuclear bomb testing has spiked the atmosphere and the oceans with large amounts of artificial 14C, beginning around AD 1955 in the Northern Hemisphere, with the effect becoming strong after AD 1958 (Hua and Barbetti Reference Hua and Barbetti2004; Hua et al. Reference Hua, Barbetti and Rakowski2013). Hence, today local reservoir ages can be determined only on materials collected live prior to significant bomb testing. Therefore, only material in museum collections can be used to resolve this problem.
A by-product of the work by McNeely et al. was the finding that deposit-feeding mollusks have larger apparent ages on calcareous substrates (not on acidic substrates) than do suspension-feeding mollusks. The ΔR values derived by McNeely et al. (Reference McNeely, Dyke and Southon2006), therefore, excluded dates on deposit feeders. England et al. (Reference England, Dyke, Coulthard, McNeely and Aitken2013) examined this question further by comparing coupled dates on deposit and suspension feeders, and concluded that ages on deposit feeders can be as much as 2000 years too old. They speculated that the enhanced effect in deposit feeders was due to assimilation of bicarbonate from pore water derived in part from the carbonate sediment, which is 14C dead, rather than entirely from freely circulating ocean water. Foxe Basin and Hudson Strait are floored by Paleozoic carbonate rocks. These issues aside, mollusks have never been used in the Arctic to directly date archaeological sites, because they are not known to be present as food refuse.
Establishing reservoir corrections for marine mammals is potentially more problematic. Firstly, mammals may be migratory (e.g., Sergeant Reference Sergeant1965; Richard et al. Reference Richard, Heide-Jørgensen, Orr, Dietz and Smith2001; Heide-Jørgensen et al. Reference Heide-Jørgensen, Dietz, Laidre, Richard, Orr and Schmidt2003), hence averaging spatial reservoir effects. Secondly, they may dive to feed at depths greater than the 75 m surface water layer (e.g., Boeuf et al. Reference Boeuf, Costa, Huntley and Feldkamp1988; Laidre et al. Reference Laidre, Heide-Jørgensen, Dietz, Hobbs and Jørgensen2003), although walrus usually forage at depths of less than 80 m (Wiig et al. Reference Wiig, Gjertz, Griffiths and Lydersen1993; Born and Knutsen Reference Born and Knutsen1997; Jay et al. Reference Jay, Farley and Garner2001). Thirdly, different species have different diets (primarily shrimp, clams, and gastropods for bearded seals; primarily fish for ringed seals; primarily benthic invertebrates for walrus; see Naughton [Reference Naughton2012] for details) and tissues are assimilated from food, with potentially different carbon fractionation effects. Walrus may also indiscriminately include deposit-feeding mollusks in their food. Hence, we need to determine if reservoir ages established for mollusks can be applied to mammals to apply these techniques to improve archaeological chronologies. This paper is the first focused attempt to establish reservoir corrections for a specific marine mammal taxon from the North American Arctic based on live-collected, pre-bomb specimens. Most attempts to quantify local marine reservoir effects in the Arctic have utilized mollusks of known age, but several have attempted to utilize marine mammals. Olsson (Reference Olsson1980) estimated the marine reservoir effect for Sweden using a number of different pinniped and cetacean taxa with known dates of death. Dumond and Griffin (Reference Dumond and Griffin2002) used a paired-dating approach in which they examined marine mammal bone collagen and charcoal from contemporaneous archaeological contexts to derive local ΔR values for the eastern Bering Sea region. Furze et al. (Reference Furze, Pieńkowski and Coulthard2014) estimated ΔR for beluga on the basis of nine 14C dates (originally presented by Stewart et al. [Reference Stewart, Campana, Jones and Stewart2006]) from individuals distributed across the central and eastern Canadian Arctic. We present 28 new dates on walrus from the eastern Canadian Arctic and compare them to the previous dates on mollusks. We also present 35 coupled dates on caribou (Rangifer tarandus) and walrus from three archaeological site complexes in the region to see if the two methods produce compatible results.
MATERIALS AND METHODS
We obtained a list of live-harvested walrus samples in the collections of the Canadian Museum of Nature and subsequently permission to examine them. Of the samples available to us, three were from Prince Charles Island in central Foxe Basin, 21 were from islands in northern Hudson Bay and western Hudson Strait, within or directly adjacent the Foxe Basin region of McNeely et al. (Reference McNeely, Dyke and Southon2006), and three were from farther east in central Hudson Strait (Figure 1). The three walrus from Prince Charles Island correspond with the modern distribution of the Foxe Basin stock or population (Born et al. Reference Born, Gjertz and Reeves1995; COSEWIC 2006; Stewart Reference Stewart2008). These walruses are widely distributed throughout this area with their movements occurring on a seasonal basis due to changing ice conditions; the Foxe Basin stock appears to be isolated (Born et al. Reference Born, Gjertz and Reeves1995). On the basis of lead isotopic measurements consistent with divergent long-term residency patterns, there may be some internal stock distinctions within Foxe Basin (Outridge and Stewart Reference Outridge and Stewart1999), but genetic studies indicate a single interbreeding population (de March et al. Reference de March, Maiers and Stewart2002). The majority of the walrus samples were collected from individuals from areas associated with the presently recognized Northern Hudson Bay-Davis Strait (NHB-DS) stock (Born et al. Reference Born, Gjertz and Reeves1995; COSEWIC 2006; Stewart Reference Stewart2008). Walruses occur throughout the year in Northern Hudson Bay and western Hudson Strait, but recent genetic and satellite tracking demonstrate that some of these animals move to west Greenland (Andersen et al. Reference Andersen, Born, Doidge, Gjertz, Wiig and Waples2009; Dietz et al. Reference Dietz, Born, Stewart, Heide-Jørgensen, Stern, Rigét, Toudal, Lanthier, Jensen and Teilmann2014). As with the Foxe Basin stock, there may be some internal divisions in the NHB-DS stock (Outridge et al. Reference Outridge, Davis, Stewart and Born2003), but the internal population dynamics remain too poorly studied to formally define sub-groups (Stewart Reference Stewart2008).
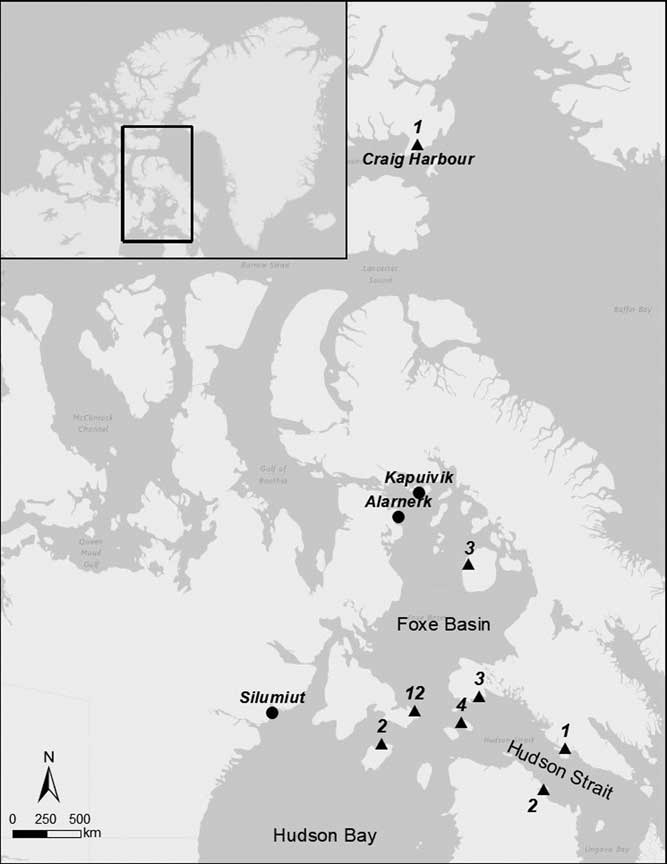
Figure 1 Map of the study area. Triangles denote areas where museum walrus specimens were collected; numbers adjacent to the triangles indicate the number of samples from each location. Archaeological sites from which paired caribou-walrus samples were obtained are indicated as circles.
We extracted subsamples of about 1 g of bone or ivory from 28 specimens, which are all available specimens collected at known localities and years before AD 1955. We also recorded information on sex, age, and size from archival notes and measured selected bone elements.
All samples had been stored in plastic wraps in climate-controlled rooms and were in an excellent state of preservation. Collagen was extracted from bones (museum and archaeological) at either the Archaeology Isotope Laboratory at The University of British Columbia (UBC) or at the University of California Irvine (UCI) following Beaumont et al. (Reference Beaumont, Beverly, Southon and Taylor2010), ultrafiltered to obtain the >30 kDa fraction, converted to CO2 and graphitized for AMS dating at The University of California at Irvine. Collagen quality was assessed from collagen yields (wt % of total bone mass) and by measuring C:N ratios (see Appendix in Supplementary Material). Carbon and nitrogen isotopic and elemental compositions were determined by CF-EA/IRMS using an IsoPrime continuous flow isotope-ratio mass spectrometer coupled to a Vario Micro elemental analyzer (Elementar, Hanau, Germany) (UBC) or a Finnigan Delta Plus isotope ratio mass spectrometer/Fisons NA1500NC elemental analyzer (UCI). Carbon and nitrogen isotopic compositions were calibrated relative to VPDB and AIR, respectively, using multiple well-characterized standard references materials. Measurement precision based on long-term reproducibility of standards was <0.1‰ for δ13C and <0.2‰ for δ15N at UCI. Standard uncertainty at UBC was determined to be ±0.20‰ for δ13C and ±0.17 for δ15N based on repeated analysis of internal and international standards, and sample replicates (Szpak et al. Reference Szpak, Metcalfe and Macdonald2017). Elemental compositions were calibrated using glutamic acid (USGS40) and internal methionine standards at UBC and known USGS24/IAEA N1 mixtures plus internal yeast and lysine standards at UCI. All 14C dates were conventionally normalized to δ13C=−25‰VPDB.
During the pre-bomb collection period (AD 1885–1955), 14C ages were affected by changing levels of 14C in the atmosphere as a result of (1) short-term natural variations that were characteristic of the Holocene (cosmic ray flux modulated by varying earth and sun magnetic fields), and (2) since about AD 1900, the burning of fossil fuels, the industrial effect, which reached 100 years in 1955 (Mangerud et al. Reference Mangerud, Bondevik, Gulliksen, Karin Hufthammer and Høisæter2006). These effects are combined in the Stuiver and Braziunas (Reference Stuiver and Braziunas1993a) model to produce “model ages” representing the apparent 14C age of marine biomass for a given collection year with 1σ errors (their Fig. 14; see also Stuiver et al. [Reference Stuiver, Pearson and Braziunas1986:982]). The regional offset ΔR is then the 14C age of a walrus minus the “model age” (McNeely et al. Reference McNeely, Dyke and Southon2006). Here we use model ages from IntCal13 (Reimer et al. Reference Reimer, Bard, Bayliss, Beck, Blackwell, Ramsey, Buck, Cheng, Edwards, Friedrich, Grootes, Guilderson, Haflidason, Hajdas, Hatté, Heaton, Hoffmann, Hogg, Hughen, Kaiser, Kromer, Manning, Niu, Reimer, Richards, Scott, Southon, Staff, Turney and van der Plicht2013), downloaded from the radiocarbon.org website. The error on ΔR is the square root of the sum of squares of the individual 1σ errors.
Another approach in estimating the reservoir effect is the comparison of dates on terrestrial and marine materials from contexts, either geological (e.g., Vickers et al. Reference Vickers, Ward, Utting and Telka2010; Ross et al. Reference Ross, Utting, Lajeunesse and Kosar2012) or archaeological (e.g., Southon et al. Reference Southon, Nelson and Vogel1990; Southon et al. Reference Southon, Oakland Rodman and True1995; Yoneda et al. Reference Yoneda, Hirota, Uchida, Uzawa, Tanaka, Shibata and Morita2001; Dumond and Griffin Reference Dumond and Griffin2002), wherein the materials are assumed to be contemporaneous. Following Stuiver and Braziunas (Reference Stuiver and Braziunas1993a), the terrestrial 14C ages are converted to equivalent surface water marine model ages (their Fig. 15 [updated in IntCal13]). The difference between the 14C age of the marine sample and the “marine model age” of the calibrated terrestrial sample is the estimated local ΔR. Savelle and Dyke (Reference Savelle and Dyke2014) presented caribou (Rangifer tarandus) dates from Alarnerk, a major archaeological site complex in northwestern Foxe Basin; the site was further excavated by Howse in 2015. Here we present walrus dates from 12 Dorset features and 2 Thule Inuit features from the site complex and compare them to the caribou dates (18 pairs). We also present 12 pairs of dates on caribou and walrus from Dorset winter houses and associated middens at the Kapuivik site on Jens Munk Island. The Jens Munk sites were surveyed by Dyke in 2002 and Savelle and Dyke in 2003 (Savelle et al. Reference Savelle, Dyke and Poupart2009; Savelle and Dyke Reference Savelle and Dyke2014). The new dates are from excavations by Desrosier and Kotar conducted in 2016. Finally we present five pairs of dates from the Thule Inuit Silumiut (KkJg-1) site in northwestern Hudson Bay (McCartney Reference McCartney1977). The caribou dates were calibrated using the CalPal online calculator and the error of a calibrated date is 68% of its range. The ΔR values were calculated using Calib ΔR application (http://calib.org/deltar) (Reimer and Reimer Reference Reimer and Reimer2017). Where average ΔR values are reported, these represent error-weighted means (Bevington Reference Bevington1969).
RESULTS
Museum Walrus Specimens
The 14C ages and the calculated ΔR values for the live-collected walrus specimens are presented in Table 1, with co-ordinates for sample locations provided in the Appendix (see Supplementary Material). Two other samples, not used to calculate ΔR, are statistical outliers. One is from Craig Harbour on SE Ellesmere Island at the head of Baffin Bay, far distant from the other samples considered here. The other is from western Hudson Strait. Its large apparent age and marginal C:N ratio led us to date a second collagen extract from bone chunks that were sonicated in acetone and methanol prior to decalcification, leading to a result accordant with the others. We suspect that a conserving agent was inadvertently included in the first collagen extract but was successfully removed by the solvent treatments applied to the second.
Table 1 14C ages of live-collected walrus from Foxe Basin and vicinity. Marine model ages from IntCal13.

Stable isotope ratios, carbon and nitrogen contents and C:N ratios were tightly clustered, in expected ranges for well preserved collagen (DeNiro Reference DeNiro1985; Ambrose Reference Ambrose1990; van Klinken Reference van Klinken1999) and for walrus in the Canadian Arctic (Coltrain et al. Reference Coltrain, Hayes and O’Rourke2004; Jaouen et al. Reference Jaouen, Szpak and Richards2016). There were no indications of sample (collagen) quality issues other than the one noted above.
The ΔR results (Table 1) suggest that all samples can be treated as a single population. The small subset of samples (3) from Central Hudson Strait averages 190±20 yr, and the small subset (3) from central Foxe Basin averages 175±45 yr. The large subset (21) from western Hudson Strait and northern Hudson Bay averages 155±50 yr, necessarily close to the overall average of 160±50 yr. The spread of only 35 years in subset means and standard deviation of 50 years indicates that all results overlap at two standard deviations and differences are not significant (One-way ANOVA, F [2,24]=0.80, p=0.46). Therefore 160±50 yr is our best estimate of ΔR for walrus from this region.
The ΔR results (Table 2) for suspension feeding mollusks from the Foxe Basin region yield a rounded error-weighted average of 295±130 yr (n=32). This distribution is significantly different from that for walrus (Mann-Whitney U test, U=212, p=0.001).
Table 2 14C ages of live-collected mollusks from Foxe Basin region (McNeely et al. Reference McNeely, Dyke and Southon2006). See original for species. Results differ slightly from original because marine model ages are from IntCal13 and errors are not rounded.

* Error weighted mean of two replicate samples.
Comparative Dating
Of the 12 Dorset Paleoeskimo features pair-dated at Alarnerk (total 16 pairs), 11 are dug-in, shallow winter house depressions or their associated middens, and the other (08DCA78F1) is Meldgaard’s ocher grave (Lynnerup et al. Reference Lynnerup, Meldgaard, Jakobsen, Appelt, Koch and Frøhlich2003). The five pair-dated features (12 pairs) at Jens Munk Island are three dug-in Dorset winter houses and associated middens and a nearby diffuse discard area (03DCA205). All features appear to represent single cultural layers overlying raised beach gravel. The two Thule Inuit features at Alarnerk are semisubterranean sod dwellings. Those at Silumiut are similar Thule dwellings and associated middens. Such dwellings, and perhaps also the Dorset winter dwellings, may represent multigenerational occupations (see below).
In all cases, as expected, walrus 14C ages exceed caribou ages (Table 3). The ΔR estimates range from 82±34 to 438±23 yr with two additional outliers at 540±34 and 698±27 yr. Excluding the outliers, the rounded mean and standard deviation are 270±90 yr (n=33).
Table 3 Comparative dating of caribou and walrus remains from Alarnerk (08DCA) and Jens Munk (03DCA) archaeological sites, northwestern Foxe Basin and from Silumiut site (KkJg-1) in northwestern Hudson Bay. Marine model ages from IntCal13. An asterisk indicates dates on tooth/tusk.

* Note that this value was calculated using the method presented by Southon et al. (Reference Southon, Oakland Rodman and True1995) as the Reimer and Reimer (Reference Reimer and Reimer2017) application cannot calculate ΔR values when the 14C age is <199 yr.
DISCUSSION
The significant difference between the ΔR results for museum specimens of walrus and mollusks indicates that results from one cannot be applied to the other. It is possible that the difference will disappear with much larger sample sizes, but these will be difficult to attain given the limited availability of museum walrus collections. Because walrus feed almost exclusively on mollusks, predominantly Mya spp. in Foxe Basin (Fisher and Stewart Reference Fisher and Stewart1997), and yet have a smaller ΔR, the difference between the distributions is problematic. We suggest several possible interpretations. First, the molluscan taxa represented in Table 2, although excluding obligate deposit feeders (Portlandia and Macoma), differ from those consumed by walrus and the difference in estimated ΔR (walrus vs. mollusk) may reflect taxonomic differences among the mollusks in the relative proportions of carbon in their shells that are derived from dissolved organic and dissolved inorganic sources, which is known to occur (Lorrain et al. Reference Lorrain, Paulet, Chauvaud, Dunbar, Mucciarone and Fontugne2004; Gillikin et al. Reference Gillikin, Lorrain, Bouillon, Willenz and Dehairs2006).
The four largest molluscan ΔR values in Table 2 are for Astarte spp. and an additional five Astarte values exceed the molluscan mean. Thus, Astarte, an infaunal suspension feeder (McNeely et al. Reference McNeely, Dyke and Southon2006), may also derive some of its shell carbonate from pore water. The single Mya measurement in Table 2 has a ΔR of only 110±40 yr, which overlaps with the lower end of the walrus values. Secondly, the mollusk meat consumed by walrus may differ in 14C content from that of the mollusk shells. Walrus ingest primarily meat, because they use their tongues to suction the meat and discard shells (Kastelein et al. Reference Kastelein, Muller and Terlouw1994) and the carbon that would be eventually incorporated into the collagen in the walrus bones or teeth would have been derived from dietary protein (Jim et al. Reference Jim, Ambrose and Evershed2004). Table 4 presents δ 13C values for both shells and body tissue of several mollusk species collected live from Resolute Bay in the Canadian Arctic Archipelago (R. McNeely unpublished data). In all species, the tissue discriminates against the heavier isotope(s). That should, therefore, increase the walrus ΔR with respect to that of the mollusk shell. However, these fractionation effects are corrected in conventional 14C age calculations. Thirdly, the walrus may have fed in areas with large terrestrial carbon input. However, the walrus collagen δ13C values are not consistent with this suggestion and there are no large estuarine areas or drainage basins near the sample sites, many of which are on islands. Fourthly, there should be differences in the 14C content of mollusk shells and soft tissue because the shell is inert once precipitated, while the soft tissue continuously turns over. Studies quantifying the rate of soft tissue turnover in marine invertebrates are generally lacking, but the organic carbon in the soft tissue of blue mussel was found to have a half-life of 8.9 days (Dubois et al. Reference Dubois, Jean-Louis, Bertrand and Lefebvre2007).
Table 4 Comparison of stable isotope values in shells and tissues of marine mollusks from Resolute Bay.

Some mollusks can live for well over one hundred years (Weidman et al. Reference Weidman, Jones and Lohmann1994; Sejr et al. Reference Sejr, Sand, Jensen, Petersen, Christensen and Rysgaard2002). Consequently, depending on the position along the shell’s growth axis from which the sample was taken, there may be a significant difference in the period of time reflected in the 14C content of the shell and the soft tissue that would have been consumed by the walrus. Finally, the sampled walrus may have fed over a region large enough to significantly sample areas with lower reservoir ages, in this case margins of the Labrador Sea. However, Foxe Basin walrus are thought to reside year-round in the region, retreating to tidally induced polynyas (open water) in the winter (Born et al. Reference Born, Gjertz and Reeves1995). The first interpretation, therefore, seems the most reasonable.
The variance of the mollusk ΔR values is much greater than that of the walrus values despite similar sample sizes; standard deviations of 130 and 50, respectively. The smaller variance for walrus may reflect their choice of a small number of mollusk taxa as food, primarily Mya sp. (see above). The greater variance for mollusks may reflect poorly documented species dependencies of ΔR alluded to above. However, it may be due in part to the greater standard errors (up to 80 years vs. 15 years for walrus) in these somewhat earlier 14C analyses. McNeely et al. (Reference McNeely, Dyke and Southon2006) excluded several dates from their data set based on non-reproducible paired datings of individual specimens by different laboratories.
The ΔR for the Craig Harbour walrus (300±15 yr) is much larger than any of the ΔR values from the Foxe Basin-Hudson Strait region. Craig Harbour is in the Canadian Arctic Archipelago region of McNeely et al. (Reference McNeely, Dyke and Southon2006), which has a larger ΔR for mollusks than does the Foxe Basin Region. The larger ΔR for Craig Harbour is thus in the right direction and it is indistinguishable from that for suspension feeding mollusks (300±95 yr) in its region.
The results from comparative dating of caribou and walrus are consistent in the sense that the caribou dates are all younger but the inferred ΔR values are discordant with the results from the museum specimens of walrus (Figure 2). Ignoring 2 outliers, the mean ΔR value of 270±90 yr is significantly larger than the value for museum walrus (160±50 yr) (Mann-Whitney U test; U=119, p<0.001) but statistically indistinguishable from the mollusk value of 295±130 yr (U=408, p=0.12). The outlier samples cannot represent time variance in ΔR because other samples of similar ages are not outliers. We suggest instead that the outliers represent the clearest cases where the walrus remains truly are much older than the caribou. Note that the differences cannot be due to differential temporal spans of carbon integration by walrus and caribou. The average walrus lifespan is 20–30 yr (Fay Reference Fay1985), while caribou average 7–12 yr, with antlers being shed annually and therefore only representing a single year of growth (Naughton Reference Naughton2012:570).

Figure 2 Histogram of ΔR values measured from museum specimens of walrus and estimated from paired datings of walrus and caribou from archaeological features.
Aside from the outliers, we suggest that the discordance between the archaeological and museum ΔR values is best interpreted in light of the museum results. In short, the assumption that the archaeological walrus and caribou from each feature are strictly contemporaneous is not supported in at least half the cases (Group 2 of Table 3). The assumption is based on the fact that all of the archaeological samples came from what appear to be single cultural layers and on the notion that close proximity of bones indicates closeness in age. Nevertheless, it is possible that these layers, be they house floors or midden layers, accumulated over considerable intervals, the well-known palimpsest effect in archaeology (the studies of palimpsest formation among the Nunamiut of Alaska by Binford (Reference Binford1982, Reference Binford1983) are especially relevant in this context). This would be especially true of semisubterranean dwellings, from which all three sites samples were collected. Because these dwellings represent considerable costs in time and energy, they would invariably be reused on a seasonal basis, often for several generations, as were similar dwellings in Alaska (Rainey Reference Rainey1947; Spencer Reference Spencer1959; see also Savelle Reference Savelle1987). Within such dwellings, individual occupation layers, especially in the case of shallow Paleoeskimo dwellings, are rarely identifiable, with material from multiple occupations often simply swept aside into common refuse areas (see e.g., Habu and Savelle Reference Habu and Savelle1994; Savelle and Habu Reference Savelle and Habu2004). Poor soil development further exacerbates the palimpsest problem, especially at Paleoekimo sites in Foxe Basin, where very thin horizontal sheet middens predominate, in contrast to the more substantial mound middens in, for example, southern Hudson Bay (e.g., Taylor Reference Taylor1968) and Labrador (e.g., Jordan Reference Jordan1980). In the eight features where two or more caribou bones were dated, only one returned dates within error. The others indicate minimum occupation intervals of 48–329 yr, based on the mean calibrated ages.
Considering the miniscule percentage of available bones that have been dated, it is improbable that the oldest and youngest were included. Nevertheless, even that duration can account for the discrepancy between the two sets of results. Furthermore, some of the walrus remains may have been scavenged from older sites. In that regard, we noted that walrus skulls, mandibles and rarely tusk fragments are conspicuous on the surface of Dorset middens, which are extensive but typically are only a decimetre or so thick. However, most of the maxillae and mandibles lack teeth, suggesting that well preserved bone, especially ivory, may have been a preferred raw material for later occupants. A tooth (tusk) can be removed far more easily from a walrus skull that is decades or more old than from the skull of a freshly killed animal, where thick maxillary bone has to be removed (see e.g., LeMoine and Darwent [Reference LeMoine and Darwent1998] for the various steps in the removal of tusks from fresh skulls by Paleoeskimos). Our paired datings included five on walrus teeth/tusks (Table 3). All of these yielded ΔR estimates above the mean of Group 1 and three of them are above the overall mean. Nevertheless, the high ΔR estimates derived from other walrus bone elements indicates that they too have long residency at sites in comparison to caribou. The middens and floor refuse may have accumulated in highly spotty patterns, leading to age differences between nearby specimens. Specifically, therefore, we propose that only samples with apparent ΔRs of <260 years (Figure 2; 18 out of 35 samples; Group 1, Table 3) represent approximately contemporaneous pairs. The mean ΔR of that group is 209±46 yr, which is still significantly older than the museum results (Mann-Whitney U test; U=119, p=0.004).
CONCLUSION
14C dates on museum specimens of walrus from the Foxe Basin-Western Hudson Strait region of the eastern Canadian Arctic indicate that a ΔR value of 160±50 yr be used in calibrating dates on walrus from this region. The significant difference between these dates and a similar set of dates on museum specimens of mollusks argues against applying mollusk based corrections to sea mammals at this time. The results of comparative dating of caribou and walrus from archaeological features provide maximum estimates of reservoir ages that are more varied than the directly measured ages. Although about half of inferred ΔR values overlap the museum specimen results, the others indicate that the assumption of contemporaneity does not hold true.
ACKNOWLEDGMENTS
We are grateful to Dr. Kamal Khidas, Curator of Vertebrate Collections for permitting access to and sampling of the walrus specimens at the Canadian Museum of Nature in Gatineau, Quebec and to Dr. Roger McNeely for providing the data on Resolute Bay mollusks, and to Samantha Walker for producing Figure 1. Funding for Arctic field work was provided by a Social Sciences and Humanities Research Council of Canada (SSHRC) Insight Grant (JMS, ASD) and aircraft and other logistical support was provided by Polar Continental Shelf Project, Natural Resources Canada.
SUPPLEMENTARY MATERIAL
To view supplementary material for this article, please visit https://doi.org/10.1017/RDC.2018.50