INTRODUCTION
Neptunium is the first transuranic element discovered and it belongs to the actinide series. Neptunium metal is silvery and tarnishes when exposed to air. The element normally exhibits five oxidation states, ranging from +3 to +7. Np is present in the environment as a result of atmospheric nuclear weapons testing in the 1950s and early 1960s, from releases from nuclear fuel reprocessing facilities, and nuclear accidents such as Chernobyl. There are 22 isotopes of neptunium which are known today, the most long-lived isotope being 237Np, with a half-life of 2.14×106 yr. 237Np is a byproduct of nuclear reactor operation and plutonium production. It is mainly produced by the following three processes: the successive thermal neutron capture on 235U, followed by a beta decay, i.e, 235U(n,γ)236U((n,γ)237U(β-)237Np; high-energy neutron-induced 238U(n,2n)237U reaction, followed by a beta decay; directly by alpha decay of 241Am (T 1/2 = 432.7 yr). It has been estimated that during the period between 1945 and 1980, about 40 TBq of 237Np were released into the environment (Beasley et al. Reference Beasley, Kelley, Maiti and Bond1998). The activity inventory of 237Np is orders of magnitude lower in the environment compared to 239+240Pu or 241Am. Determination of 237Np is rather complicated, because of its very low specific activity and lack of suitable yield tracer such as high purity 236Np. Due to the low 237Np concentration present in the environment, previous studies concerned samples contaminated by other sources. Here we focused on the region only affected by the global fallout, which is located in Guangxi, south of China.
Methods previously employed to determine 237Np include alpha spectroscopy, neutron activation analysis, inductively coupled plasma mass spectrometry, thermal ionization mass spectrometry, accelerator mass spectrometry, and other mass spectrometry. Alpha spectroscopy is capable of producing good results with lower limits of detection but requires tedious chemical separations and long counting times. The greatest advantage of alpha spectroscopy is the relatively low cost of equipment. On the other hand, for the detection of 237Np, alpha counting is unable to identify the alpha particles emitted by 234U and 230Th, due to the small energy differences. In recent years, ICP-MS has become one of the most powerful methods for the determination of ultra-trace levels of 237Np in many kinds of samples such as environmental, biological samples, and uranium fuels. However, abundance sensitivity is limited by molecular interferences, such as 235UH2-. A detection limit of 0.02 pg/mL with a conventional quadrupole ICP-MS (Muramatsu et al. Reference Muramatsu, Yoshida, Tagami, Uchida and Ruhm2001) and as low as fg/mL or fg/g with SF-ICP-MS could be achieved for actinides (Becker Reference Becker2003; Varga et al. Reference Varga, Suranyi, Vajda and Stefanka2007).
Because of the lack of molecular isobars, AMS is the most sensitive detection technique for 237Np (down to 105 atom/sample, ca. 0.001 μBq) and has the highest abundance sensitivity. However, very few 237Np measurements have been reported by AMS. Environmental samples such as mud, water and sediment were analyzed by AMS at the Australian National University (Fifield et al. Reference Fifield, Clacher, Morris, King, Cresswell, Day and Livens1997; Keith-Roach et al. Reference Keith-Roach, Day, Fifield and Livens2001). It was estimated that sensitivity by AMS is ca. 105 atoms (4 × 10–17 g) of 237Np. A method for AMS measurement of 237Np was set up at the China Institute of Atomic Energy, and a sensitivity less than 10–11 has been achieved for the isotopic ratio 237Np/238U (Wang et al. Reference Wang, Jiang, He, Dong, Chen, He, Wang, Wu and Hu2013). More details about analytical techniques and sample preparation for determination of 237Np are reviewed in Thakur and Mulholland (Reference Thakur and Mulholland2012). The aim of this work is to determine activity concentration of 237Np in surface soil samples collected from Guangxi, south of China.
EXPERIMENTAL
Sample Preparation
Surface soil and sediment samples were collected at 4 locations in 2012–2016 (Table 1). At each location, approximately 500 g (dry weight) of soil was collected at 0–10 cm depth. These locations have been used for monitoring various radionuclides (e.g., 137Cs) in the environment. Preliminary gamma spectrometry results for 137Cs are listed in Table 1. In total, 17 samples were selected, of which 15 were analyzed for 237Np. Twelve samples were collected from 3 different monitoring locations, the other 5 samples were collected from the region near the newly built nuclear power plant (Figure 1).
Table 1 137Cs and 237Np radioactivity in collected sample from Guangxi, China.
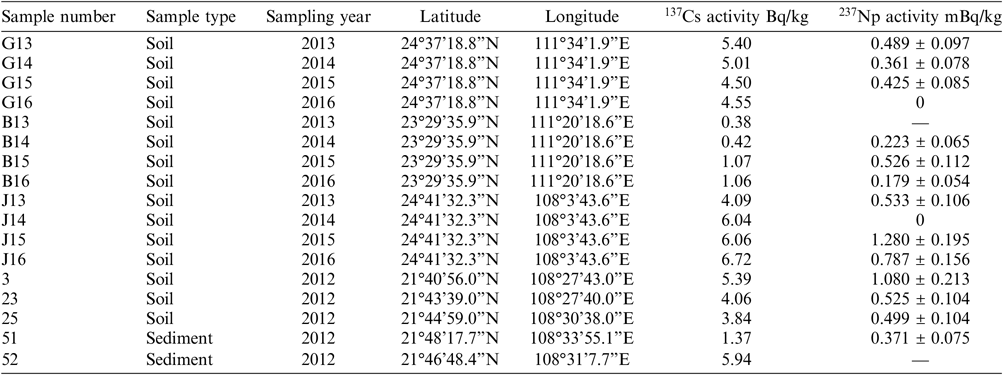
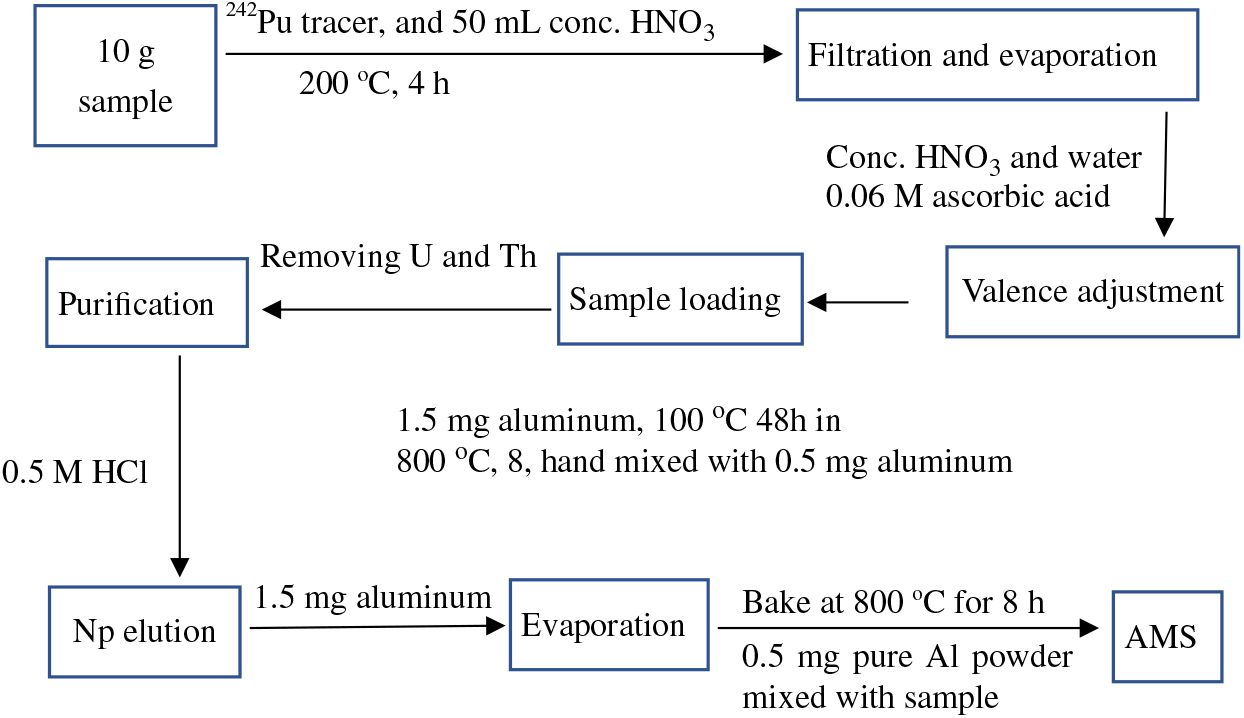
Figure 1 Flow chart of the analytical procedure for the determination of 237Np in environmental samples.
The radiochemical procedure used in this study for the preparation of 237Np use a modification of the method for ICP-MS (Kim et al. Reference Kim, Kim and Lee2004). The flow chart of the analytical procedure for the determination of 237Np in environmental samples is shown in Figure 1. After baking at 500ºC for 2 hr, about 10 g of sample was weighed and placed into a Teflon beaker. Consequently, 3 pg of 242Pu (i.e. 1010 atoms) as tracer was added and mixed with the sample for radiochemical separation.
Samples were dissolved using 50 mL concentrated HNO3 on a hot plate at 200ºC for 4 hr to mix plutonium into solution, filtered and then vaporized to approximately 1 mL. The resulting solution was dissolved by adding concentrated HNO3, then adjusted to acidity of 5 M HNO3 by adding de-ionized water and then filtered. Ascorbic acid was added to the solution and allowed to stand for 1 hr to carry out the redox reaction. Separation and purification of 237Np was achieved with anion-exchange chromatography using TEVA resins. In the first purification stage, the prepared sample solution was loaded into a preconditioned 2-mL TEVA column with 0.5 M HCl. Then, the column was washed with 13 mL 5 M HNO3 followed by 7 mL 9 M HCl and 10 mL 1 M HNO3 to remove uranium and thorium. After that, neptunium and plutonium were eluted with 0.5 M HCl. The eluant was transferred to a 10-mL centrifuge tube, and then 0.5 mL Al(NO3)3 solution equivalent to 1.5 mg aluminum was added. The centrifuge tube was heated at 100ºC for 48 hr. What remained was weighted and then transferred to a quartz crucible followed by 8 hr of baking at 800ºC. The resulting sample was crushed in an agate mortar and mixed with 0.5 mg aluminum powder. The mixture was pressed into an aluminum sample holder for AMS measurement. Serial standard samples quantified using the isotopic dilution technique with 242Pu and 237Np as tracers, and chemical blank samples were prepared. The standard samples were prepared by dilution of the standard solutions of 237Np (NIST 4341a standard reference material, 152.3 ± 1.4 Bq/g) and 242Pu (NIST 4334I standard reference material, 26.77 ± 0.18 Bq/g). Three dilution series samples including single 237Np, single 242Pu and mixture of 237Np and 242Pu were made following the preparation procedure described above. To test the reliability and repeatability of the treatment procedure, several batches of standard samples were prepared during different time periods. Two uranium samples were used for tuning the AMS system, and two chemical procedural blanks were prepared to check the detection limit.
The AMS Technique
The 237Np measurements were performed at the AMS facility at the Center for Isotopic Research on Cultural and Environmental Heritage (CIRCE) in Caserta, Italy. Detailed information of the main elements of CIRCE AMS has been provided previously (Terrasi et al. Reference Terrasi, Rogalla, De Cesare, D’Onofrio, Lubritto, Marzaioli, Passariello, Rubino, Sabbarese and Casa2007; De Cesare et al. Reference De Cesare, Guan, Quinto, Sabbarese, De Cesare, D’Onofrio, Gialanella, Petraglia, Roca and Terrasi2010; Guan et al. Reference Guan, Wang, De Cesare and Terrasi2017). The process of 237Np AMS measurement is similar to that of 236U, plutonium and other actinide measurements. An initial pilot beam of 238U16O- at a terminal voltage of 2.680 MV was used for tuning purposes and transmission measurement because the intensity of 237Np is not large enough to obtain measurable current, neither in standard samples nor environmental samples. Scaling the optimizing parameters for 238U, the operating parameters for 237Np and 242Pu are calculated: all magnetic fields are kept constant and electric and magnetic rigidities of the various beams are matched using the bouncer voltage applied to the injection magnet vacuum chamber, the terminal voltage and electrostatic analyzer voltage. Table 2 summarizes the operating parameters for the various actinides measured with CIRCE AMS facility. Negative NpO- and PuO- ions are extracted by sputtering the sample with a Cs+ beam in a commercial ion source (40 samples, MC-SNICS, NEC). These ions are pre-accelerated to 50 kV and passed through a high-resolution injection system including an electrostatic analyzer and an injector magnet. Cycling between 242Pu and 237Np was done by fast changing the bouncer voltage on the injection magnet, terminal voltage and the voltage of high energy electrostatic analyzer. Sequential injection into the accelerator was set 100 s for 242Pu16O– and 237Np16O–. The durations of injection of 237Np and 242Pu is 20 s. Samples are typically counted for 100 s at both mass 242 (242Pu) and mass 237 (237Np) for 3 times, always ending with a 242Pu measurement. Standards of known amounts of 237Np and 242Pu in aluminum oxide were run periodically to give the relative counting efficiencies for Np and Pu, allowing quantitative measurement of the amount of Np in each sample.
Table 2 The AMS operating parameters for transmission of 242Pu and 237NP.

237Np Measurement Results
The reliability of the 237Np results was verified by the linearity and reproducibility of the results. Four batches, with 3 standard samples for each batch with the concentration of 237Np from 0.3 to 30 pg were measured (Figure 2). The counts in Figure 2 are the average value of three cycles, and the values on a 95% confidence level (2σ). In our experiment, the 237Np counts in 4 batches within 100 s for 30 pg samples varied from 250 to 650. The uncertainty of 237Np are less than 10%, 15%, and 25% for 30 pg, 3 pg, and 0.3 pg 237Np, respectively. A good linearity is observed for each batch for 237Np counts recorded by the final gas ionization chamber versus the 237Np concentration. The count rates of samples taken in different batches fluctuated greatly, indicating a variability of the sample preparation process. Sometimes, the chemical yield is not strictly constant and even varied by three times, as it has been reported in the earlier 237Np preparations (La Rosa et al. Reference La Rosa, Gastaud, Lagan, Lee, Levy-Palomo, Povinec and Wyse2005). The specific research on this fluctuation reason, such as chemical yield and/or experimental operation skills will be undertaken in the near future. In fact, the chemical yield can be confirmed by alpha spectroscopy or HPGe detector, but this needs a large amount of 237Np and 242Pu standard material. We did not carry out this step. ICP-MS is an ideal choice to determine the chemical yields, we will also investigate this method in the future. Figure 3 shows the gated 242Pu counts measured by the ionization chamber vs sputter time for seven environmental samples. For the first half hour of the experiments, counts of 242Pu in each sample are relatively stable. In order to estimate the maximum sputter time, all the cathodes were weighted before and after experiment: the results indicated that only a part (about 30–50%) material in the cathode was consumed.
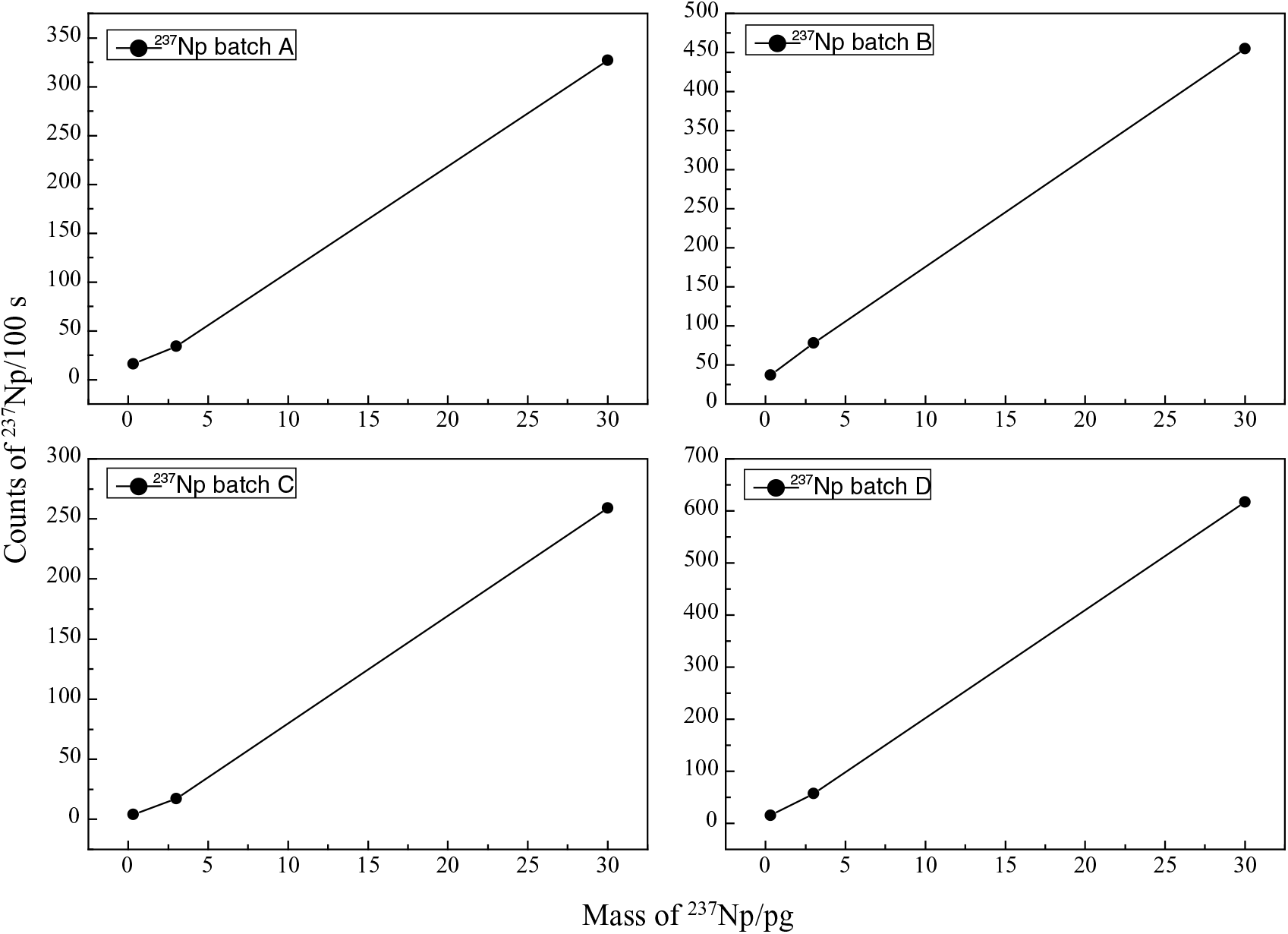
Figure 2 Linearity of the AMS measurements for 237Np with 4 batches of standard samples.
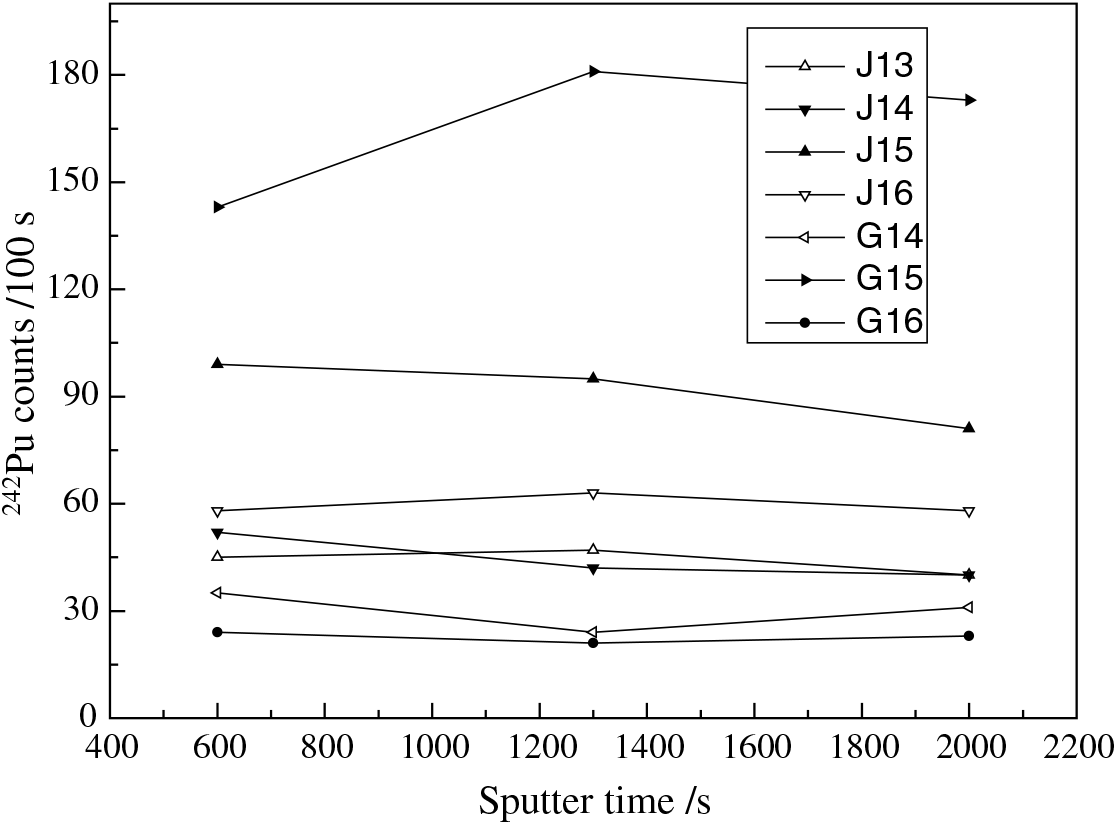
Figure 3 242Pu gated counts in each 100 s measurement time as a function of sputter time. Each sample was measured for 3 cycles.
A batch of standard samples containing known amounts of 242Pu and 237Np dispersed in aluminum oxide was prepared for determining relative negative ion formation probabilities of PuO– and NpO– by AMS. Ratios of abundance of the isotope of interest (e.g. 237Np and 242Pu) to aluminum were approximately 10–8. The standards were run periodically to give the relative negative ion formation probabilities for Np and Pu, allowing quantitative measurement of the amount of 237Np and 242Pu. In our results, count rates of 0.170 ± 0.018 cps/pg and 0.061 ± 0.006 cps/pg were obtained for 237Np and 242Pu, respectively, from several samples. If we assume that 237Np and 242Pu have the same chemical yield, then we can get a relative negative ion formation probability of Np/Pu of 0.353 ± 0.004.
Determination of 237Np in environmental samples was then carried out: the concentration of 237Np was normalized to the 242Pu according to the relative negative ion formation probabilities of Pu and Np. The results are listed in Table 1. The 237Np concentrations in both samples G16 and J14 are lower than the detection limit, and two samples B13 and 52 were contaminated during sample preparation. In addition to these samples, the activity concentrations of 237Np in environmental samples collected from Guangxi, which are only affected by the global fallout, varied between 0.179 ± 0.054 and 1.280 ± 0.195 mBq/kg. In all the chemical procedural blank samples and two uranium tuning samples, we could not get any counts of neither 237Np nor 242Pu within 500 s. 237Np activity concentrations in forest litter samples from Poland with pure global fallout were 0.099 mBq/kg and 2.21 mBq/kg (La Rosa et al. Reference La Rosa, Mietelski and Wyse2010). A preliminary comparison between our experiment and Poland, shows that the 237Np concentrations are of the same order of magnitude.
CONCLUSIONS
The reliability of the chemical analytical procedure for the determination of 237Np using 242Pu as a tracer for 237Np AMS measurement was demonstrated. Standard samples and environmental samples were prepared and measured, and the linearity and reproducibility of 237Np was verified (Figure 2). The counts of 242Pu in each environmental sample are relatively stable in the first half hour (Figure 3). If we assume that 237Np and 242Pu have the same chemical recoveries, the relative negative ion formation probability of Np/Pu was found to be 0.353 ± 0.004 in this study, and there is a significant difference between this value and the earlier study (Fifield et al. Reference Fifield, Clacher, Morris, King, Cresswell, Day and Livens1997). According to our preliminary analysis, the difference is more likely caused by binder effects. Different binder materials, such as Nb, Ag, Fe, and Al, will be used to further investigate this issue. Since the sample containing 2 mg of aluminum will run for at least 4000 s, the ultimate sensitivity for 237Np is estimated be 1.5 × 10–15 g. Due to an insufficient amount of standard material, we did not measure the chemical yield, so we cannot obtain the accuracy result of negative ion formation probability in this study. The average value of 237Np concentration in the region of interest is about 20 pg/kg (i.e. 0.53 mBq/kg). We observed a very good consistency of 237Np concentration with pure global fallout from different locations between our experiments and the early report (La Rosa et al. Reference La Rosa, Mietelski and Wyse2010). Improvements in chemical recoveries and choice of the ideal binder material will lead to greater reliability and sensitivity.
ACKNOWLEDGMENTS
This work was supported by the National Natural Science Foundation of China (Grant Nos. 41166002, 11665006, and 41773004), Guangxi Natural Science Foundation (Grant Nos. 2016GXNSFAA380142, 2016GXNSFFA380006, and 2017GXNSFAA198251), Innovation Team and Outstanding Scholar Program in Guangxi Colleges, and the One-Hundred-Talents Program of Guangxi colleges. Two anonymous reviewers are thanked for their useful comments and suggestions.