INTRODUCTION
Paleoenvironmental and paleo-geographic reconstruction are usually carried out through the analysis of facies on cores, high resolution seismic surveys, geomorphological surveys and related morphostratigraphy and archaeological evidence correlation and interpretation. Radiocarbon (14C) measurements play a key role since peat levels or fossil shells sampled in cores allows to properly define evolutionary timescales. The reconstruction of the paleogeographic evolutionary model of the Mar Piccolo semi-enclosed sea basin in Taranto (Southern Italy), has been possible thanks to the availability of these data and in particular to the detailed facies analysis carried out on two cores. All together, they allowed to reconstruct the local Holocene sea level curve (Valenzano et al. Reference Valenzano, Scardino, Cipriano, Fago, Capolongo, De Giosa, Lisco, Mele, Moretti and Mastronuzzi2018a). In this area, sandwiched volcanic ash and peat levels represent chronological constraints providing the timing of the different evolution stages. This is in particular possible thanks to the identification of the “Pomici di Mercato” a Vesuvius eruption (dated to ca. 8900 BP) on the base of the geochemical features of the associated tephra layer and to 14C (AMS) analyses carried out on samples indicative of past sea-level found before and after the tephra sedimentation. In the studied cores, peats are always preceded and overlain, in stratigraphic continuity, by muddy-sands levels characterized by the abundant presence of coupled valves in living position of the bivalve Cerastoderma glaucum (Poiret, 1789) and Corbula gibba (Olivi, 1792).C. glaucum is a benthic filter feeder lamellibranchia that lives in waters at various depths tolerating widely fluctuating salinities (Carboni et al. Reference Carboni, Bergamin, Di Bella, Esu, Pisegna Cerone, Antonioli and Verrubbi2010). In low salinity waters, for example, it is found at high depth (Boyden Reference Boyden1971, Reference Boyden1972; Krzyminska Reference Krzyminska1993; Kandeel et al. Reference Kandeel, Saad, Afaf and Marwa2017).
In particular, in the Mediterranean basin, C. glaucum, is associated with lagoons/inner basins environments. It is considered a good sea level marker with maximum 2 m of approximation (i.e., Gravina et al. Reference Gravina, Ardizzone, Scaletta and Chimenz1989; Scarponi and Kowalewski Reference Scarponi and Kowalewski2004; Ferranti et al. Reference Ferranti, Antonioli, Mauz, Amorosi, Dai Pra, Mastronuzzi, Monaco, Orrù, Pappalardo, Radtke, Renda, Romano, Sansò and Verrubbi2006; Antonioli et al. Reference Antonioli, Ferranti, Fontana, Amorosi, Bondesan, Braitenberg, Dutton, Fontolan, Furlani, Lambeck, Mastronuzzi, Monaco, Spada and Stocchi2009; Negri Reference Negri2009; Pavlopoulos et al. Reference Pavlopoulos, Triantaphyllou, Karkanas, Kouli, Syrides, Vouvalidis, Palyvos and Tsourou2009; Di Rita et al. Reference Di Rita, Simone, Caldara, Gehreles and Magri2011; Primavera et al. Reference Primavera, Simone, Fiorentino and Caldara2011; Carboni et al. Reference Carboni, Bergamin, Di Bella, Esu, Pisegna Cerone, Antonioli and Verrubbi2010; Taviani et al. Reference Taviani, Angeletti, Çağatay, Gasperini, Polonia and Wesselingh2014; Lambeck et al. Reference Lambeck, Anzidei, Antonioli, Benini and Verrubbi2018). Indeed, in Mar Piccolo some samples can live up to 5 m depth (as shown by modern surveys) meaning that only a detailed facies analysis of sediment cores allows to safely use them as good sea level markers. Due to the abundance of well preserved and complete specimens of C. glaucum, some samples extracted from the Mar Piccolo cores were submitted to 14C age determinations in order to validate peat and tephra indications. Because of the evident inconsistency between peat/tephra ages and C. glaucum ones, an extensive accelerator mass spectrometry (AMS) 14C dating campaign was then planned and carried out on this taxa. Samples from sediment cores, living specimens from Mar Piccolo and from a bay along the SW side of the Ugljan Island (Croatia), as well as specimens collected in the Mar Piccolo in the 1960s obtained from the collection of the Museum of the Department of Biology of the University of Bari were analyzed.
Indeed, though AMS 14C dating on C. glaucum are widely used in paleo-environmental reconstructions, large age offsets and age incongruity have been reported in different coastal areas of the Mediterranean sea (e.g., Sabatier et al. Reference Sabatier, Dezileau, Blanchemanche, Siani, Condomines, Bentaleb and Piquès2010; Orrù et al. Reference Orrù, Mastronuzzi, Deiana, Pignatelli, Piscitelli, Solinas, Spanu and Zucca2014). These offsets can be explained as due to the combination of marine reservoir effect (MRE) and hardwater effect (HWE). The origin and mechanisms originating these effects are quite different. Marine effects are associated with the delayed dissolution and mixing of atmospheric carbon dioxide into the oceans and to the interplay of water circulation phenomena such as the upwelling of 14C-depleted deep waters. These phenomena result in apparently older ages of marine organisms when compared with coeval organism fixing (radio)carbon from the atmosphere. The difference between the 14C conventional ages of a marine and a coeval “terrestrial” organism is referred as reservoir age, R(t), which is typically time and location dependent. For the calibration of 14C ages obtained on marine samples, a marine calibration curve is derived from the atmospheric curve by applying a simple box model and by correcting for local effects by using a ΔR term defined as the difference between the local reservoir offset and the global value (Stuiver et al. Reference Stuiver, Reimer and Braziunas1998; Reimer et al. Reference Reimer, Bard, Bayliss, Beck, Blackwell, Bronk Ramsey, Buck, Cheng, Edwards, Friedrich, Grootes, Guilderson, Haflidason, Hajdas, Hatté, Heaton, Hoffmann, Hogg, Hughen, Kaiser, Kromer, Manning, Niu, Reimer, Richards, Scott, Southon, Staff, Turney and van der Plicht2013). As reference, an average modern pre-industrial reservoir age of 400 yr is assumed while an average ΔR = 58 ± 15 yr was measured for the Mediterranean basin (Siani et al. Reference Siani, Paterne, Arnold, Bard, Mativier, Tisnerat and Bassinot2000; Reimer and McCormac Reference Reimer and McCormac2002). The origin of the hardwater effect (HWE) is more variable and different phenomena have been identified as responsible for it. Among them, one can consider the dissolution of carbonates of geological origin, the input from sediment rich of old organic matter and the melting of glacier ice (Fernandes et al. Reference Fernandes, Bergemann, Hartz, Grootes, Nadeau, Melzner, Rakowski and Hüls2012). In particular, hardwater offset ages as large as 1200 yr have been reported in lacustrine environments (Zoppi et al. Reference Zoppi, Albani, Ammerman, Hua, Lawson and Serandrei Barbero2001). Indeed, similar phenomena are expected for the studied area, at present corresponding to a semienclosed sheltered sea-basin that is supplied by freshwater flows from karst acquifer hosted in Mesozoic units.
The aim of this study is to estimate the possible presence of 14C age offset on C. glaucum in the Mar Piccolo basin in Taranto, by analyzing modern and living samples. Throughout the paper, we define age offset the difference between the 14C age measured on a C. Glaucum specimen sampled in the Mar Piccolo in Taranto and the age measured on a coeval marine organism taken in the open sea from the same geographical area. The effect of the measured age offset on the interpretation of sedimentary core sequences obtained from the same area is also highlighted and discussed.
Geological and Morphological Settings of Mar Piccolo Area
The Mar Piccolo basin (Taranto, Southern Italy) is located on the northern Ionian coast (Figure 1). It is a semi-enclosed sea basin today connected with the Mar Grande and then to Ionian Sea by two channels: the northwestern one, called Canale di Porta Napoli, a natural channel today partially filled by human activity, and the eastern one, called Canale Navigabile, artificially excavated in the late 19th century (Mastronuzzi et al. Reference Mastronuzzi, Boccardi, Candela, Colella, Curci, Giletti, Milella, Pignatelli, Piscitelli, Ricci and Sansò2013).
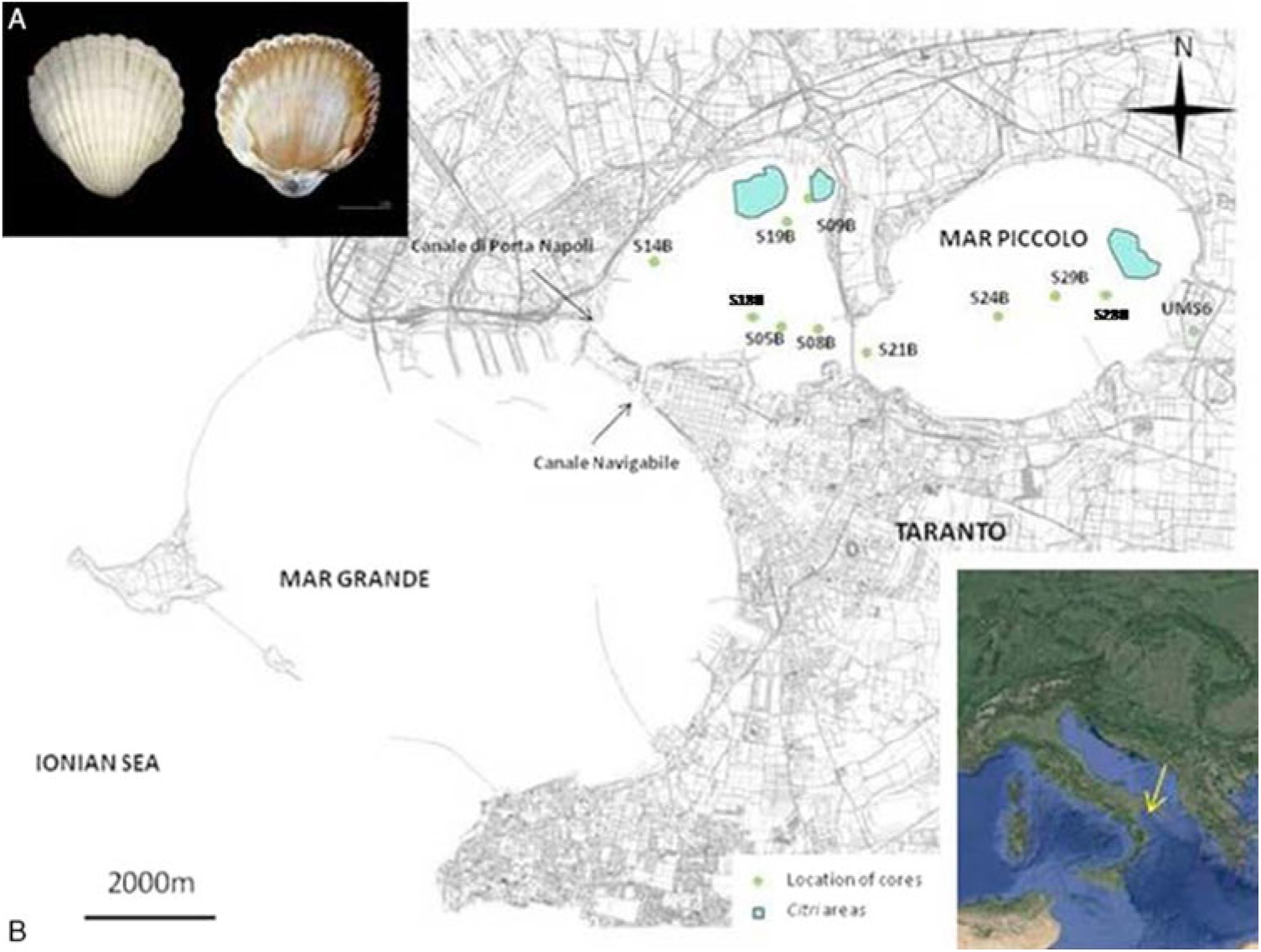
Figure 1 (A) A sample of C. glaucum analyzed. (B) The Mar Piccolo basin in Taranto along the Ionian coast of Apulia (Southern Italy) and the location of cores samples (Basemap: Apulian Technical Regional Map).
The stratigraphic succession of the Mar Piccolo basin is represented, from the bottom, by Mesozoic limestone (Calcare di Altamura Fm.) covered in transgression by Upper Pliocene–Lower Pleistocene calcarenite (Calcarenite di Gravina Fm.). The latter passes upwards and laterally, to the interfingered argille subappennine informal unit (Lisco et al. Reference Lisco, Corselli, De Giosa, Mastronuzzi, Moretti, Siniscalchi, Marchese, Bracchi, Tessarolo and Tursi2016; Valenzano et al. Reference Valenzano, D’Onghia, De Giosa and Demonte2018b) (Figure 2).These units are covered by marine terraced deposits. The lowermost overlays the argille subappennine unit and is ascribed to the Last Interglacial Time, corresponding to the Marine Isotope Substage 5.5 (= MIS 5.5; Shackleton Reference Shackleton2000) (e.g., Amorosi et al. Reference Amorosi, Antonioli, Bertini, Marabini, Mastronuzzi, Montagna, Negri, Rossi, Scarponi, Taviani, Angeletti, Piva and Vai2014; Negri et al. Reference Negri, Amorosi, Antonioli, Bertini, Florindo, Lurcock, Marabini, Mastronuzzi, Regattieri, Rossi, Scarponi, Taviani, Zanchetta and Vai2015). During the following sea level low stand associated with the global glacial growth, the area today occupied by the Mar Piccolo and the Mar Grande, was incised by a river network that reached, during the Last Glacial Maximum (MIS 2), the past sea level at about 120/140 m below the present one (e.g., Mastronuzzi and Sansò Reference Mastronuzzi and Sansò1998, Reference Mastronuzzi and Sansò2003; Mastronuzzi et al. Reference Mastronuzzi, Boccardi, Candela, Colella, Curci, Giletti, Milella, Pignatelli, Piscitelli, Ricci and Sansò2013). The postglacial sea level rise induced the flooding of the river valley that assumed the features of an elongated rias, partially filled by sediments. Indeed, the Mar Piccolo basin was fed mainly by seawater incoming by the present Canale di Porta Napoli. In a secondary way, a river catchment shaped on carbonate Mesozoic and Plio-Pleistocenic units, and subaerial and submarine karst springs, nourished by the deep karst water table hosted in the Mesozoic units, supplied the rias of a blend of salt and fresh waters. The process was characterized by two phases. During the first one, from about 15 to 7 ka BP, the fast sea level rise submerged the valley with the formation of beaches and peatlands in the most protected areas; in this phase there was an important contribution of fresh water from springs and tributaries. In the second, with the reduction of the sea level rise at about 7ka BP, and with the stabilization of a warmer, and drier climate, many of the springs were submerged and the waves widened the valley shaping it to the present morphology of an inlets whose flanks are continuously reshaped by wave erosion (Valenzano et al. Reference Valenzano, Scardino, Cipriano, Fago, Capolongo, De Giosa, Lisco, Mele, Moretti and Mastronuzzi2018a).

Figure 2 Geological map of the Mar Piccolo basin modified by Valenzano et al. (Reference Valenzano, D’Onghia, De Giosa and Demonte2018b) after Lisco et al. (Reference Lisco, Corselli, De Giosa, Mastronuzzi, Moretti, Siniscalchi, Marchese, Bracchi, Tessarolo and Tursi2016). (A) and (B) location of the studied area and (C) its geological map:1. Calcare di Altamura Fm (Cretaceous); 2. Calcarenite di Gravina Fm (Upper Pliocene-Lower Pleistocene); 3. Argille subappennine informal unit (Pleistocene); 4. Marine terrace deposits (MIS 5); 5. Alluvial deposits; 6. Holocene marine sediments; 7. Submarine springs; 8. Ephemeral drainage networks; 9. Buried faults; 10. Bathymetric contour, every 5 m; 11. Topographic contour, every 10 m. (D) Stratigraphic sketch of the sedimentary units.
The reconstruction of Holocene geomorphological evolution has been possible thanks to the availability of 29 different sediment cores drilled in the Mar Piccolo seafloor up to about –50 m from the present mean sea level and by the detailed analysis of two of them (Figure 3). Sandwiched levels of tephra and peat allow to obtain age constraints by mean of 14C age determinations and geochemical analysis. Moreover, peat levels indicate the past positions of sea level before and after the sedimentation of the tephra layers ascribed to the Pomici di Mercato volcanic event, which occurred about 8900 yr BP (Valenzano et al. Reference Valenzano, D’Onghia, De Giosa and Demonte2018b). Just above and below the peat levels, massive muddy-sands without any evidence of lamination or reworking are characterized by the abundant presence of C. glaucum and C. gibba. These dominant taxa are always accompanied by fragments of freshwater lacustrine or continental species, such as Planorbis, Helix, Pomatia and Rumina spp. while brackish/marine species, such as Bittium reticulatum (da Costa, 1778) and Cerithium vulgatum (Bruguière, 1792) have been found in very low percentages. Anyway they are generally common in eurythermal and euryhaline biocoenoses on hard and/or algal substrata of lagoonal or marine sheltered environments. Taken together, this evidence indicate that the presence of C. glaucum in the cores above the peat levels, is connected to sea-level transgression over the peatlands; whereas its presence below the peat levels, is connected to a transgressive muddy-sands above the ravinement surface. Only after a detailed facies analysis, due to its presence in a transgressive system, C. glaucum can be used as a marker of sea level. Its presence corresponds to the development of a lagoonal/marine environments characterized by sheltered waters with very low energy.
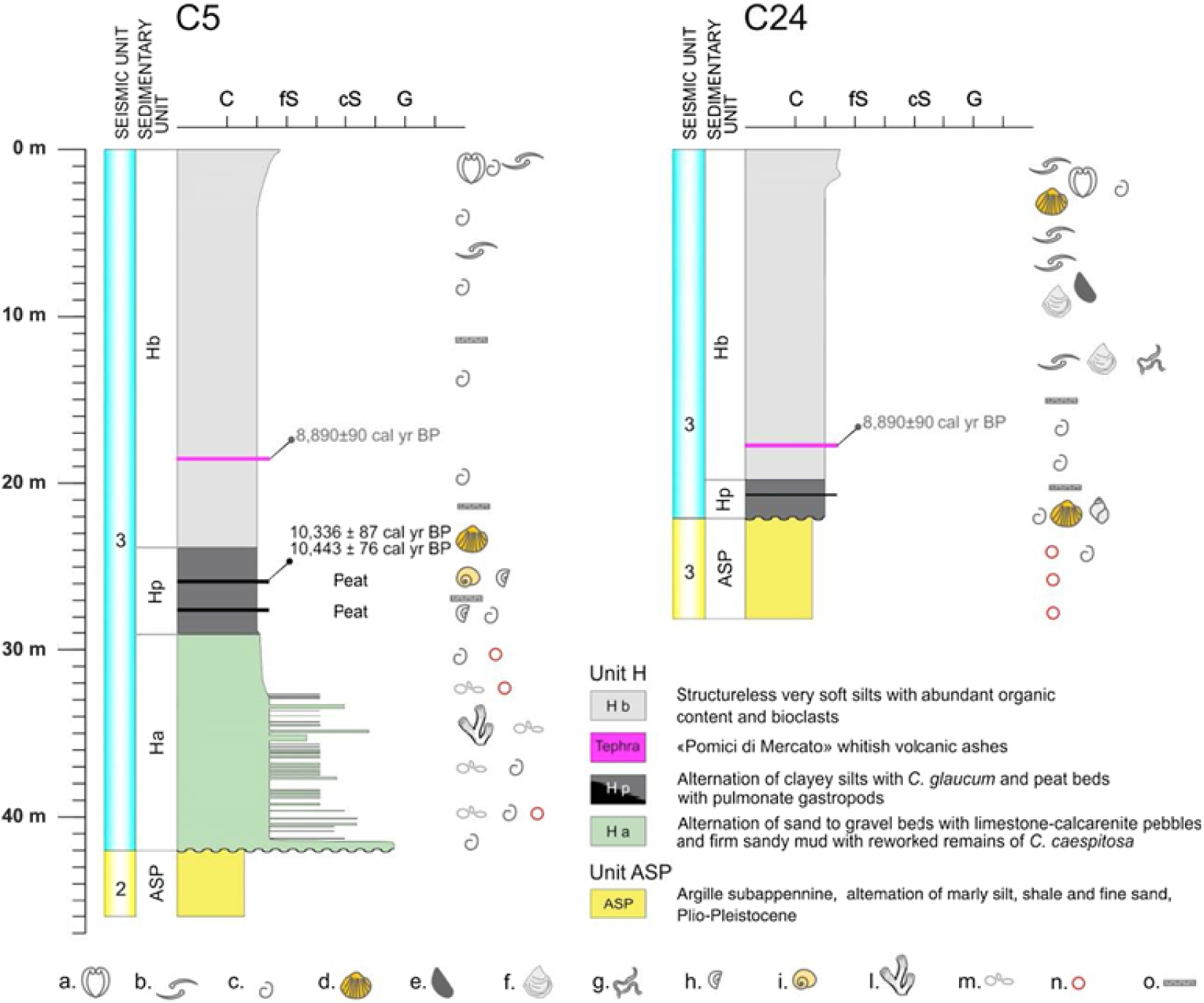
Figure 3 Sedimentary units of cores analyzed by Valenzano et al. (Reference Valenzano, Scardino, Cipriano, Fago, Capolongo, De Giosa, Lisco, Mele, Moretti and Mastronuzzi2018a). Key: a. articulated bivalves; b. disarticulated valves; c. unspecified marine mollusc fragments; d. Cerastoderma glaucum; e. Mytilus spp.; f. ostreidae.; g. Polychaeta spp.; h. pulmonate gastropod fragments; i. pulmonate gastropods; l. Cladocora caespitosa fragments; m. carbonatic nodules; n. iron oxides/hydroxides; o. organic matter. Note: The bold black lines indicate peat levels and the bold magenta lines indicate tephra levels. (From Valenzano et al. Reference Valenzano, Scardino, Cipriano, Fago, Capolongo, De Giosa, Lisco, Mele, Moretti and Mastronuzzi2018a.)
These paleogeographic features correspond to the present dynamic of the Mar Piccolo which is characterized by a low hydrodynamic and by very slow water circulation, where the exchange velocity with the connected Mar Grande is estimated to be about maximum of 45 days for the eastern part of the basin (De Pascalis et al. Reference De Pascalis, Petrizzo, Ghezzo, Lorenzetti, Manfè, Alabiso and Zaggia2016).
Today, detailed hydrogeological studies show that the Mar Piccolo basin can be considered a drainage basin for the ground water springs located both along the coast and underwater (locally known as citri) (i.e.: Zuffianò et al. Reference Zuffianò, Basso, Casarano, Dragone, Limoni, Romanazzi, Santaloia and Polemio2015; Valenzano et al. Reference Valenzano, D’Onghia, De Giosa and Demonte2018b and references therein), influencing the basin salinity whose value is normally around 37 psu (lower than the value of about 38 psu of the close open sea; De Pascalis et al. Reference De Pascalis, Petrizzo, Ghezzo, Lorenzetti, Manfè, Alabiso and Zaggia2016). In particular, a mean groundwater outflow into the basin was estimated to be as large as 75.2 × 106 m3 per year (Zuffianò et al. Reference Zuffianò, Basso, Casarano, Dragone, Limoni, Romanazzi, Santaloia and Polemio2015). Chemical analyses also show that groundwater is typically undersaturated with calcite and dolomite produced by Mesozoic and Plio-Pleistocene local geological basement dissolution.
14C measurements also shown a large depletion in the 14C concentration, which was estimated for one of the spring to be 40.3 ± 0.2 pMC (Zuffianò et al. Reference Zuffianò, Basso, Casarano, Dragone, Limoni, Romanazzi, Santaloia and Polemio2015).
MATERIALS AND METHODS
In this study, 19 AMS 14C dating analyses were carried out on C. glaucum sampled from different sediment cores up to a maximum of 30 m from the seafloor (Figure 1 and Table 1). Only samples in physiological position with coupled valves were selected and analyzed. The presence of C. glaucum in physiological position, can be considered typical of eurythermal and euryhaline biocoenoses on hard and/or algal substrata of lagoonal or marine brackish or shallow water sheltered environments (0–2 m), with low hydrodynamism and absence of redeposition, characterized by different types of soft bottoms. When possible, two or three samples were selected from the same position along each core in order to assess the repeatability of the analyses.
Table 1 14C age obtained on fossil Mar Piccolo’s samples. Results are calibrated with Calib 7.0.4 software (dataset: Marine13; ΔR:58 ± 15; Siani et al. Reference Siani, Paterne, Arnold, Bard, Mativier, Tisnerat and Bassinot2000; Reimer and McCormac Reference Reimer and McCormac2002).
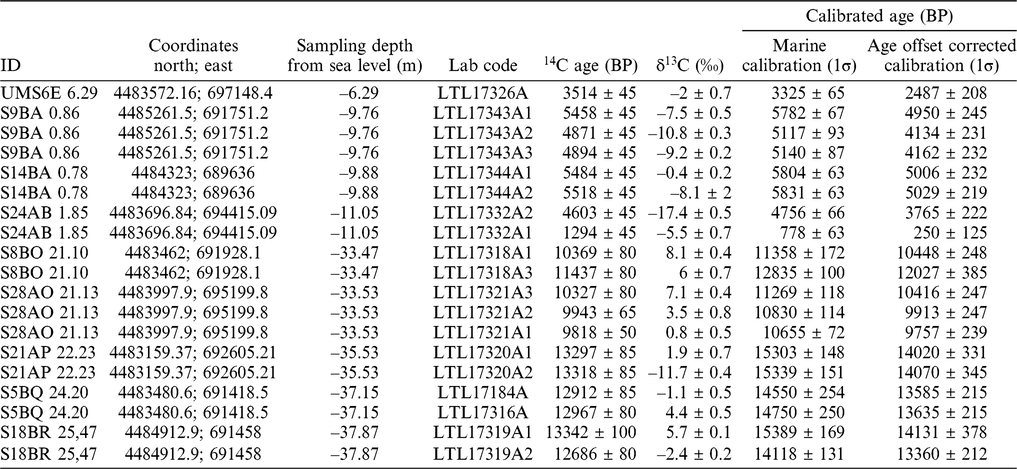
Moreover, three samples collected live in 2017 in the same basin and two specimens sampled in the 1960s from the collection of the Museum of the Department of Biology (University of Bari) were used for the estimation of the local age offset in 2017 and 1968–1969. For the 1968–1969 samples, the museum report clearly indicated that the samples were sampled live. Three more samples collected alive in a bay along the SW side of the Ugljan Island (Croatia) (Figure 4) were submitted to the same analysis (Table 2). Samples derive from the innermost part of an open bay protected by strong winds, but characterized by a continuous change of water with the near deep Adriatic sea. Here C. glaucum live up to a depth of about 1 m in a muddy-sandy bottom in association with algae and gastropods like C.vulgatum and B. reticulatum.
Table 2 Results obtained on museum and 2017 samples.


Figure 4 (A1 and A2) Location of Croatian samples (Basemap: Google Satellite) and (B) the bay of sampling. (Photo by Paul Leber from Panoramio—Google Earth).
Samples were processed at the chemical laboratories of CEDAD (Centre for Applied Physics, Dating and Diagnostics), Department of Mathematics and Physics “Ennio de Giorgi” University of Salento in Lecce (Italy). After a preliminary observation at the optical microscope, they were treated with H2O2 to remove the uppermost layer and then converted to CO2 by acid hydrolysis with H3PO4. Extracted carbon dioxide was then reduced to graphite at 600°C with high purity hydrogen on Fe powder (Calcagnile et al. Reference Calcagnile, Quarta, D’Elia, Rizzo, Gottdang, Klein and Mous2004, Reference Calcagnile, Quarta and D’Elia2005).
AMS measurements were carried out at CEDAD using the 14C beamline installed on the 3 MV Tandetron accelerator (Mod. 4130 HC by High Voltage Engineering Europa BV). IAEA (International Atomic Energy Agency) C6 (sucrose) samples were used for normalizing the measured 14C/12C isotopic ratios. The measured isotopic ratios were corrected for isotopic mass fractionation by using the ∂13C term measured on line with the accelerator and for machine and sample processing background estimated by measuring IAEA C1 samples (Carrara Marble), completely depleted in 14C, using the same processing used for the samples. 14C ages (in yr BP: before present, 1950 AD) and 14C content (in pMC = percent modern carbon) were then calculated according to Stuiver and Polach (Reference Stuiver and Polach1977).
RESULTS AND DISCUSSION
The data obtained for the samples collected in 1968–1969 and collected live in Taranto and Croatia are shown in Figure 5 and summarized in Table 2. In the same Figure, the 2017 atmospheric concentration estimated from the Hammer and Levin (Reference Hammer and Levin2017) data is also shown as a range which includes the seasonal variability. It can be observed that the samples collected in Taranto (both the 1968–1969 and 2017 ones) have 14C concentrations below 100 pMC. In order to correctly interpret these data, the current available literature data on 14C distribution across the Mediterranean basin were considered.

Figure 5 14C concentrations measured for the modern samples. The atmospheric 14C concentration as derived from Hammer and Levin (Reference Hammer and Levin2017) is given for comparison. The range takes into account the seasonal variability.
For the Western Mediterranean basin, in the Ligurian subbasin, a significant uptake of bomb 14C was measured in the period between 1953 and 1963 with Δ14C value above zero after 1961–1962. In particular in the period between 1963 and 2000, Δ14C values ranging between 50 and 90‰ were measured with the maximum value of 90 ‰ reached in 1972 on the coral Cladocora caespitosa (Linnaeus 1767; Tisnérat-Laborde et al. Reference Tisnérat-Laborde, Montagna, McCulloch, Siani, Silenzi and Frank2013). Indeed, a significant variability has to be expected between different locations across the Mediterranean which has been recently well simulated by high resolution regional models which predicts for the Ionian Sea in the Taranto region Δ14C values higher than for the Ligurian Basin (Ayache et al. Reference Ayache, Dutay, Mouchet, Tisnérat-Laborde, Montagna, Tanhua, Siani and Jean-Baptiste2017). According to the output of these models, calculated in Ayache et al. Reference Ayache, Dutay, Mouchet, Tisnérat-Laborde, Montagna, Tanhua, Siani and Jean-Baptiste2017 for the year 1977, we assumed a Δ14C = 90 ± 10‰ for Taranto in 1968–1969.
For the estimate of the present-day expected 14C concentration in the Taranto surface water we used recent data obtained for the Mediterranean surface water obtained in 2011 during the R/V Meteor MT84_3 Mediterranean Sea Cruise (Tanhua et al. Reference Tanhua, Alvarez, Mintrop, McNichol and Key2012, Reference Tanhua, Hainbucher, Cardin, Alvarez, Civitarese, McNichol and Key2013). The closest data point available from these data set is Δ14C≈44 ‰for the open Ionian Sea. This value is consistent with the output of the high resolution regional model by Ayache et al. (Reference Ayache, Dutay, Mouchet, Tisnérat-Laborde, Montagna, Tanhua, Siani and Jean-Baptiste2017) and predicting for the Taranto area a slightly higher Δ14C of about 65 ± 5‰ (Ayache et al. Reference Ayache, Dutay, Mouchet, Tisnérat-Laborde, Montagna, Tanhua, Siani and Jean-Baptiste2017). Considering a decreasing rate of the 14C concentration of ≈2‰ per year this would correspond to an expected value for Taranto in 2017 of 53 ± 5‰. The results obtained for both the 1968–1969 and the 2017 samples clearly indicate a local depletion of the 14C concentration since the measured values are significantly lower than the expected one.
In order to analyze the results obtained for the Croatian samples, the data published by Macchia et al. (Reference Macchia, D’Elia, Quarta, Gaballo, Braione, Maruccio, Calcagnile, Ciceri, Martinotti and Wacker2013) were considered. In this case the 14C in DIC (Dissolved Inorganic Carbon) extracted from sea water in 2010 obtained from Northern Adriatic surface water correspond to a Δ14C = 31.8 ± 2.7‰. In this case, the 2017 value of 17.8‰ was derived from the 2011 data by considering a decline rate of 2‰ per year.
In particular, the two samples from Mar Piccolo gave statistically consistent results with an average Δ14C = –17.5 ± 0.8‰ which is much lower than the expected value of 90‰ estimated above.
In addition, the 2017 samples from Mar Piccolo show a significant depletion in the 14C content with all the measured values below 0‰. For these samples, an average 14C content corresponding to Δ14C = –37.5 ± 11.5‰ can be estimated, where the uncertainty was estimated as scattering of the three data points.
The average 14C concentration for the Croatian samples corresponds to 12.5 ± 8.2‰ which overlaps within one standard deviation with the value of 17.8‰ expected for 2017.
We also observe here that the average 14C concentration measured for the Croatian samples corresponds to 102.1 ± 0.8 which overlaps within one standard deviation with the 2017 atmospheric concentration ~101.5 estimated on the base of the data by Hammer and Levin (Hammer and Levin Reference Hammer and Levin2017). The results obtained on these samples can be easily explained by the particular local geomorphological and hydrological features. The bay is shaped in a Mesozoic rocky body but: (1) no river supply is present, (2) no important underwater springs are present, and (3) it is characterized by a continuous exchange of deep water. Our data then indicate a significant offset in the 14C ages for the C. glaucum specimens sampled in 2017 and 1968–1969 in Taranto which is not detected (at least within the level of uncertainty achievable in this study) for the Croatia samples. This seems to point towards a site-dependent aging effect more than to a phenomenon dependent on the species (for instance related to the feedings habits).
The magnitude of the age offset has been estimated according to Coularis et al. (Reference Coularis, Tisnérat-Laborde, Pastor, Siclet and Fontugne2016) and Keaveney and Reimer (Reference Keaveney and Reimer2012) as:

Where F14CTaranto,year corresponds to the 14C concentration measured for the Taranto samples either in 2017 or 1968–1969 and F14Cref,year is the corresponding reference value for the same year estimated as described above. In this way, the local age offset effect was estimated to be 642 ± 100 yr for 2017 and 820 ± 100 yr for 1968–1969. These values overlap between each other and indicate and average offset age of 730 ± 125 yr.
The origin of this age offset, which has to be considered additional to the marine reservoir effect, is probably found in the presence of hardwater flows of karst origin.
The results obtained for the sediment core samples are given in Table 1. The obtained conventional 14C ages have been then calibrated to calendar ages by using the Marine13 curve and a ΔR value of 58 ± 15 yr (Siani et al. Reference Siani, Paterne, Arnold, Bard, Mativier, Tisnerat and Bassinot2000; Reimer and McCormac Reference Reimer and McCormac2002). In the same table, we also list the calibrated ages obtained after correcting for the age offset estimated for the modern samples. In Figure 6, the data obtained, corrected for both the marine and the hardwater effects by using the value estimated for the modern samples, are plotted as a function of their depth.
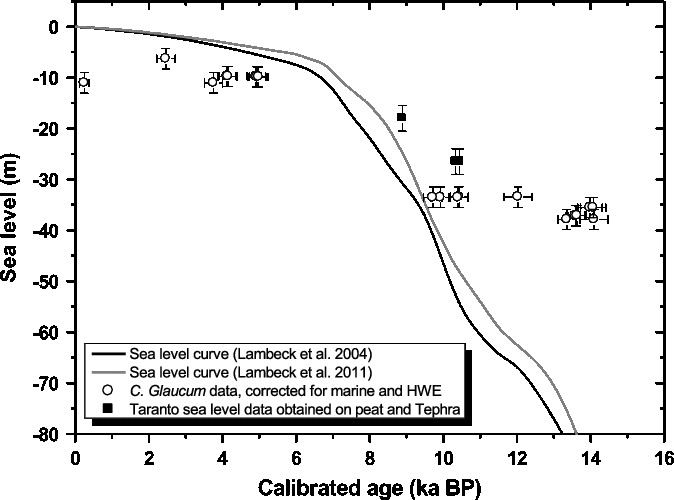
Figure 6 Results obtained on fossil samples from the mar Piccolo cores in comparison with sea level model curves elaborated by Lambeck et al. (Reference Lambeck, Antonioli, Purcell and Silenzi2004, Reference Lambeck, Antonioli, Anzidei, Ferranti, Leoni, Scicchitano and Silenzi2011).
In Valenzano et al. (Reference Valenzano, Scardino, Cipriano, Fago, Capolongo, De Giosa, Lisco, Mele, Moretti and Mastronuzzi2018a), in order to reconstruct the Taranto area paleo-environmental evolution, two time-constraints were identified in the same cores containing C. glaucum analyzed in this study. They were a peat level, immediately preceded and overlayed by levels containing C. Glaucum (Figure 3), (which can be considered a good sea level marker) at –26.5 ± 2.5 m and a tephra level at –18.5 m attributed to the “Pomici di Mercato”. For the first one, two consistent 14C dates, obtained on atmospheric samples, are available to 9188 ± 75 (LTL17186A and LTL17186B) and 9255 ± 75 BP which can be calibrated to 10,336 ± 87 and 10407 ± 110 cal BP. Tephra level is dated to 8890 ± 90 cal BP (Figure 3). It was found at a mean depth of –32.4 m msl (19 m bsb), suggesting that it deposited in a very shallow basin (at a depth of about 6–7 m); its use in the present study can be considered only for chronological purposes. When these data (black squares in Figure 6) are compared with the available sea level rise curves (Lambeck et al. Reference Lambeck, Antonioli, Purcell and Silenzi2004, Reference Lambeck, Antonioli, Anzidei, Ferranti, Leoni, Scicchitano and Silenzi2011; gray and black curves in Figure 6) different considerations can be made.
Data derived from the use of the peat levels indicate a very slow uplift that is in agreement to that already known for the area of Taranto and corresponding to about 0.128 mm/yr (Mastronuzzi et al. Reference Mastronuzzi, Antonioli, Anzidei, Auriemma, Alfonso and Scarano2017, Reference Mastronuzzi, Antonioli, Anzidei, Bruckner, De Martini, Ferranti, Monaco, Moretti, Pagliarulo, Scicchitano, Smedile, De Giosa, Fago, Lisco, Lo Presti, Pignatelli, Milella, Piscitelli, Scardino, Spampinato and Valenzano2018). On the other hand, for this part of the Mediterranean basin no other deterministic sea level curves are available except for Sibari (Ferranti et al. Reference Ferranti, Pagliarulo, Antonioli and Randisi2011; Cafaro et al. Reference Cafaro, Cotecchia, Lenti, Pagliarulo, Bilotta, Flora, Lirer and Viggiani2013). Unfortunately, the plain of Sibari corresponds to a semigraben in the Appenine chain (Ferranti et al. Reference Ferranti, Pagliarulo, Antonioli and Randisi2011; Cafaro et al. Reference Cafaro, Cotecchia, Lenti, Pagliarulo, Bilotta, Flora, Lirer and Viggiani2013), which means that a direct correlation between the curve extrapolated for the Appenine Chain (Sibari) and another for the stable foreland (Taranto) is not possible.
When comparing the data obtained for the C. glaucum samples (after correcting them for both the marine and hardwater effects) with the available sea level rise curves, two different time ranges have to be considered. The most recent samples, dated between ~10 ka BP to the present, shows results which are in acceptable agreement with the Lambeck curve. On the other hand, the samples extracted from the lower levels give inconsistent data: their age is essentially not related to the sampling position. In particular, they gave 14C ages significantly older than expected.
The observed differences between the upper and lower level can be explained when considering the evolution of the Mar Piccolo Basin. According to Valenzano et al. (Reference Valenzano, Scardino, Cipriano, Fago, Capolongo, De Giosa, Lisco, Mele, Moretti and Mastronuzzi2018a), the Mar Piccolo evolution can be interpreted as result of erosional and depositional processes in transitional low-energy settings of an incised-valley system during last sea-level cycle, due to the interplay of fluvial incisions and reduced magnitude wave-tide action.
This means that when the oldest specimens lived, the Mar Piccolo had the characteristics of a rias, fed mainly by fresh water rich of fossil calcium carbonate dissolved in the groundwater deriving from the dissolution of Murge limestones, coming from karst springs and from streams of Murge, giving rise to a significantly larger hardwater effect.
CONCLUSIONS
Samples of C. glaucum from the Mar Piccolo basin, Ionian Sea, Southern Italy, were submitted to AMS 14C dating analyses. Both fossil samples, selected from sediment cores and modern, post bomb samples were dated. The modern samples were both collected live in 2017 and obtained from museum collections. The obtained 14C results show a significant offset towards older 14C ages. The aging effect, additional to the marine one, is estimated to be 730 ± 125 yr. The comparison of the data obtained on the Taranto samples with those obtained on C. glaucum samples collected live in the NorthernAdriatic sea suggests that the measured effect is related to the site and not to the specie. The most probable explanation of the effect is related to the presence in the studied basin of hardwater flows, which are depleted in 14C as the results of the dissolution of carbonate rocks of geological age.
The results obtained on known-age samples of C. glaucum allowed to better explain, the data obtained from samples extracted from sediment core. Indeed the comparison of the data obtained on C. glaucum with reference sea-level rise curves point towards a time dependency of the measured offset effect.
The results of the analysis performed on these mollusca coming from this area indicate that C. glaucum is not always a suitable 14C samples for sea-level rise studies. To use it as good indicator of sea level marker, it is necessary that (1) its meaning as sea level markers should be assessed through a preliminary facies analysis and (2) any possible age offset on 14C determination can be excluded.
This study confirms the importance of a detailed knowledge of the sources of carbon for the organisms which are submitted to 14C dating analyses as already proved previously for other mollusca as for example the continental gastropod Helix sp. (Quarta et al. Reference Quarta, Romaniello, D’Elia, Mastronuzzi and Calcagnile2007; Mastronuzzi and Romaniello Reference Mastronuzzi and Romaniello2008; Romaniello et al. Reference Romaniello, Quarta, Mastronuzzi, D’Elia and Calcagnile2008).
ACKNOWLEDGMENTS
This study was conducted within the framework of the Collaboration Agreement (ex article 15 of law 241/90) “Activities of common interest preparatories for the remediation, environmentalization and redevelopment of the Mar Piccolo of Taranto” between the Government Commissioner for the Remediation of the Taranto area (Dr. V. Corbelli) and University of Bari and the National Research Council (responsible for the geological survey TEOT Phases D and G: G. Mastronuzzi, University of Bari). The authors gratefully acknowledge the Italian Ministry of Education, University and Research (MIUR Funds - PhD in Geosciences, Bari University) and CoNISMa (Project CIG57930987B2 Funds—Responsible M. Moretti). We wish to thank all the staff at the Polo Scientifico e Tecnologico Magna Grecia (Taranto) for the analyses carried out on the cores.