INTRODUCTION
The northeastern Brazilian coast comprises many active and stabilized eolian features formed under the influence of northeast (NE) trade winds blowing across a wave-dominated coastal system. In this area, the Lençóis Maranhenses dune field stands out as one of the major active coastal dune fields of South America, with an area of approximately 1000 km2. So far, the Lençóis Maranhenses dune field and the surrounding stabilized dunes remain poorly studied in relation to their sedimentology, stratigraphy, and evolution through time (Hesp et al., Reference Hesp, Maia and Claudino-Sales2009), especially with regard to the influence of relative sea level (RSL) and climate changes on dune field growth and stabilization. The usefulness of dune sediments for inferring climate changes has been emphasized for desert continental areas (Lancaster et al., Reference Lancaster, Kocurek, Singhvi, Pandey, Deynoux, Ghienne and Lô2002; Munyikwa, Reference Munyikwa2005; Singhvi and Porat, Reference Singhvi and Porat2008; Chase, Reference Chase2009; Fujioka et al., Reference Fujioka, Chappell, Fifield and Rhodes2009; Mason et al., Reference Mason, Lu, Zhou, Miao, Swinehart, Liu, Goble and Yi2009; Xu et al., Reference Xu, Lu, Yi, Vandenberghe, Mason, Zhou and Wang2015), focusing on variations in precipitation and their influence on the presence or absence of vegetation for dune stabilization. The sediment supply supports coastal dune field growth in a complex way because it is influenced by RSL changes combined with other climate sensitive factors such as alongshore drift, onshore winds, and inland precipitation. Also, the formation and preservation of eolian deposits depend on a combination of factors. Sediment availability, wind action, and topography control the development of the sand sea, and factors such as the depth of the water table and vegetation growth control the accumulation and the preservation of the eolian sand deposit (Kocurek, Reference Kocurek1999; Kocurek and Lancaster, Reference Kocurek and Lancaster1999). Some studies propose that the evolution of Quaternary coastal dune fields, at least on a time scale of tens to hundreds of thousands of years, is more related to RSL changes than to precipitation variations, with dune field development being frequently linked to high RSL during interglacial periods (Lees, Reference Lees2006; Giannini et al., Reference Giannini, Sawakuchi, Martinho and Tatumi2007; Bateman et al., Reference Bateman, Carr, Dunajko, Holmes, Roberts, McLaren, Bryant, Marker and Murray-Wallace2010). Also, according to Tsoar (Reference Tsoar.2005) and Yizhaq et al. (Reference Yizhaq, Ashkenazy and Tsoar2009), wind speed is the dominant factor controlling dune mobility in areas with rainfall greater than 800 mm per year, in which the influence of precipitation on vegetation is enhanced. This is the case for coastal dune fields in wet tropical areas, such as in the Lençóis Maranhenses region. Inland of the Lençóis Maranhenses active dune field, the coexistence of stabilized dunes (Figs. 1 and 2) under similar climatic conditions is a result of hysteretic behavior of dune mobility (Yizhaq et al., Reference Yizhaq, Ashkenazy and Tsoar2009). This dune bistability and the question of the transition between the two states (active or stabilized by vegetation) increase the complexity of interpreting the response of coastal eolian systems to climate changes and of using eolian dune sediments as a paleoclimatic proxy. As argued by Chase (Reference Chase2009), using the example of continental southern Africa, dune ages as paleoclimatic proxy do not show a clear relationship with paleoaridity, and the interpretation of the ages has to take into account other factors such as the interaction between vegetation, sediments, and wind strength dynamics.

Figure 1 (A) Map of northeastern Brazil, with indication of the Lençóis Maranhenses eolian system (red rectangle) and the sediment cores (GeoB 3910-2: Jaeschke et al., Reference Jaeschke, Ruhlemann, Arz, Heil and Lohmann2007; GeoB 3129-1: Weldeab, et al., Reference Weldeab, Schneider and Kolling2006; PL07-39PC: Lea et al., Reference Lea, Pak, Peterson and Hughen2003) used for paleoclimate comparison. (B) Enlarged map of the area indicated by the red rectangle in panel A, showing the location of active dune fields and geologic units of the studied area (based on Vasconcelos et al. [Reference Vasconcelos, Veiga, Colares, Ribeiro, Gomes, Medeiros and Forgiarini2004] and this study). (For interpretation of the references to color in this figure legend, the reader is referred to the web version of this article.)

Figure 2 (color online) DEM (Digital elevation model) (A) and Landsat images (year 2000) of the Lençóis Maranhenses region. (A) Elevation model based on SRTM (Shuttle Radar Topography Mission) data and position of the geologic profile (P–P’). (B) Stabilized barchanoids, parabolics, and depositional lobes blocking the drainage and forming the Caçó Lake. (C) Stabilized parabolic dunes and active depositional lobe with barchanoid chains. Note the predominance of parabolic dunes (B and C) on the stabilized features and barchanoid chains on the active dunes (C).
In this article, we used geomorphological surveys and optically stimulated luminescence (OSL) dating to characterize the spatial distribution and stabilization time of the vegetated eolian deposits located inland of the Lençóis Maranhenses active dune field. Grain-size and heavy mineral analyses were used to determine sediment source areas for the paleodune system. We investigate whether coastal dune fields from the tropical Brazilian coast could respond to Late Pleistocene climate and RSL changes. Periods of dune field stabilization were evaluated under precipitation changes (Cruz et al., Reference Cruz, Vuille, Burns, Wang, Cheng, Werner, Edwards, Karmann, Auler and Nguyen2009; Zhang et al., Reference Zhang, Chiesse, Mulitza, Zabel, Trindade, Hollanda, Dantas, Govin, Tiedemann and Wefer2015) and sea level changes (Corrêa, Reference Corrêa1996; Rabineau et al., Reference Rabineau, Berná, Olivet, Aslanian, Guillocheau and Joseph2006) recorded for the northeastern Brazilian coast and inland areas during the Late Pleistocene.
STUDY AREA
The Lençóis Maranhenses dune field is situated in a coastal tropical area with a mean temperature of 26°C (Nimer, Reference Nimer1989) and a tidal range from 2 to 4 m (Fig. 1). The annual rainfall and winds are seasonally influenced by latitudinal shifts of the Intertropical Convergence Zone (ITCZ). The precipitation is concentrated in the period from January to June (1480 mm) under the ITCZ influence and prevalence of weak winds (Tsoar et al., Reference Tsoar, Levin, Porat, Maia, Herrmann, Tatumi and Claudino-Sales2009). Conditions favorable for sand eolian transport occur from July to December, when the NE trade winds are prevailing on the coast and the rainfall is strongly reduced (143 mm). The trade winds are also responsible for waves reaching the coast from the north and NE, with the predominance of most energetic NE waves during the first semester (Pianca et al., Reference Pianca, Mazzini and Siegle2010). Consequently, the wave system generates a main littoral drift system to the west (Bittencourt et al., Reference Bittencourt, Dominguez, Martin and Silva2005). As a result, high eolian transport is associated with high sand input to the shoreface and foreshore because of the intensification of the westward littoral drift.
The continental shelf adjacent to the study area has a mean declivity of 0.06° from the shoreline to −70 m water depth (Fig. 1) with predominance of quartz sands at the surface (Kowsmann and Costa, Reference Kowsmann and Costa1979). The mean declivity increases to 3° at the continental slope from −70 m to −200 m water depth.
The onshore area has Quaternary sedimentary deposits overlaying the Cretaceous Itapecuru Formation (Grajaú Basin) to the south and the Neogene Barreiras Formation (Vasconcelos et al., Reference Vasconcelos, Veiga, Colares, Ribeiro, Gomes, Medeiros and Forgiarini2004; Fig. 1) to the east. Sandy deposits covering the Barreiras Formation have been characterized, in part, as eolian deposits (Rossetti, Reference Rossetti2004; Almeida-Filho et al., Reference Almeida-Filho, Rossetti, Miranda, Ferreira, Silva and Beisl2009) as well, consisting probably of older Quaternary eolian generations (Fig. 1). The Caçó Lake is about 5 km long, 0.5 km wide, and with maximum depth of 12 m (Sifeddine et al., Reference Sifeddine, Alburquerque, Ledru, Turcq, Knoppers, Martin and Mello2003). It is situated on top of older Quaternary eolian deposits, dammed by stabilized dune field features (Fig. 2), and is an important paleoclimate record from the last 21 ka (Ledru et al., Reference Ledru, Mourguiart, Ceccantini, Turcq and Sifeddine2002; Sifeddine et al., Reference Sifeddine, Alburquerque, Ledru, Turcq, Knoppers, Martin and Mello2003; Jacob et al., Reference Jacob, Disnar, Boussafir, Sifeddine, Turcq and Alburquerque2004). The Parnaíba River, which is the eastern boundary of the study area, has a mean discharge of 763 m3/s (available at http://mapas-hidro.ana.gov.br/Usuario/DadoPesquisar.aspx [accessed June 2, 2017]) and is the principal sediment source to the coast.
MATERIALS AND METHODS
Geomorphology and sediment sampling
Eolian dunes stabilized by vegetation were mapped using Landsat satellite images from the years 1990 and 2000 (available at https://zulu.ssc.nasa.gov [accessed August 30, 2017]). A total of 11 sand samples were collected for OSL dating in pits and outcrops (Fig. 3). Sampling in pits was performed at 1 to 2 m depth to avoid bioturbation in areas previously mapped as stabilized eolian dunes in Figure 1. Migrating dunes, such as barchans and parabolics, are characterized by sediment recycling and self-cannibalization resulting in a constant resetting of the quartz OSL clock (Chase, Reference Chase2009). We therefore interpret the dating results of stabilized eolian features as the ages of last dune activity, immediately antecedent to the stabilization by vegetation, considering that the OSL dating samples were collected at the top of stabilized dunes. Another five samples were collected in outcrops of the older eolian deposits and at the base of the stabilized dunes unit with preserved surface morphology. Those five ages are interpreted as the record of the accumulation phases of the eolian system. A total of 51 sediment samples, corresponding to 36 from stabilized eolian features, 10 from the older eolian deposits, and 5 from the Barreiras Formation, were collected for grain-size and heavy mineral analyses. Code, location, and other additional information about the studied samples can be found in Supplementary Table 1.

Figure 3 (A and B) Stabilized eolian dunes covered by forest vegetation as seen in the field. (C and D) Outcrops of paleodunes covering the alluvial Barreiras Formation. Red dots indicate Optically stimulated luminescence dating samples. Ages in ka. (For interpretation of the references to color in this figure legend, the reader is referred to the web version of this article.)
OSL dating methods
OSL dating was carried out on coarse-grain quartz aliquots. The preparation of quartz aliquots was performed under red/orange light and consisted of separation of 120–150 µm sand grains through wet sieving, followed by treatment with hydrogen peroxide (29% H2O2) and hydrochloric acid (3.75% HCl) in order to remove organic matter and carbonate minerals. Afterward, etching with hydrofluoric acid (HF 48–51%) for 40 minutes was performed to remove the outer rind of quartz grains damaged by alpha particles and to reduce the amount of feldspar grains. Density separation with lithium polytungstate solution at densities of 2.75 g/cm3 and 2.62 g/cm3 was executed to isolate quartz grains from heavy minerals and remnant feldspar grains, respectively. The OSL measurements were carried out with an automated Risø DA-15 TL/OSL system in the Radiation Dosimetry Laboratory at Oklahoma State University (Stillwater, OK, USA). The built-in 90Sr/90Y beta source gives a dose rate of 95.9±4.1 mGy/s. Optical stimulation was carried out with blue LEDs (470 nm), delivering 31 mW/cm2 to the sample. A Hoya U-340 filter was used for light detection in the ultraviolet band. The heating rate used in thermal treatments was 5°C/s. The equivalent doses were determined using the single aliquot regenerative-dose protocol (Murray and Wintle, Reference Murray and Wintle2000; Wintle and Murray, Reference Wintle and Murray2006), which is summarized in Table 1. The preheat temperature of 200°C was determined with a plateau test and dose-recovery test carried out using 20 aliquots from four samples (LM-1c-1, LM-19B, LM-24EB, and LM-40) representing the spatial variability and age range. Doses for the dose-recovery test were chosen to be close to the expected natural dose and were 18 Gy, 5 Gy, 130 Gy, and 8 Gy, respectively, applied to aliquots from samples LM-1c-1, LM-19B, LM-24EB, and LM-40. The aliquots were bleached under sunlight for 4 hours before luminescence measurements. Mean dose recovery has a 1.02 calculated-to-given dose ratio, with three aliquots higher than the 1.10 limit, two from LM-24EB and one from LM-19B. More information about plateau and dose-recovery tests can be found in the Supplementary Materials.
Table 1 Single aliquot regenerative-dose protocol used in this study. D0=0 Gy for measurement of the natural signal. Regeneration doses D1 through D4 ranged from 1 to 300 Gy, with values varying between samples. D5 known dose for dose-recovery test, D6=D1 for recycling ratio, D7=0 for recuperation, and D8=D4 for feldspar test after infrared stimulation. The test dose was 20–25% of the expected natural dose. OSL, optically stimulated luminescence.

The concentrations of 40K, 238U, and 232Th used for dose-rate estimation were determined by gamma ray spectrometry with a high-resolution HPGe detector encased in a 15-cm-thick lead shield. Samples were dried before packing in sealed plastic containers and stored for at least 28 days for radon equilibration before measurement. Radionuclide concentrations were obtained by comparison with gamma ray spectra from standards for geologic materials. Beta and gamma dose rates were calculated using the conversion factors given by Adamiec and Aitken (Reference Adamiec and Aitken1998). The contribution of cosmic radiation to the dose rate was calculated as described by Prescott and Hutton (Reference Prescott and Hutton1994).
Grain-size and heavy mineral analyses
Sediment samples were submitted to grain-size analysis by laser diffraction using Malvern Mastersizer 2000 equipment. Grain-size distributions were represented by 0.125 phi intervals. The calculation of mean diameter, standard deviation, and skewness was made according to the Pearson moments analytic method. Folk and Ward’s (Reference Folk and Ward1957) criteria were used for the nominal classification of the grain-size results. Heavy mineral analysis was performed on the very fine sand fraction (62–125 µm). Heavy mineral grains were separated by settling in bromoform (CHBr3, density=2.83 g/cm3). Magnetic heavy minerals were extracted using a hand magnet. The nonmagnetic heavy mineral fraction was analyzed under a polarized light microscope, and at least 200 transparent and nonmicaceous grains were identified and quantified using the ribbon counting method (Galehouse, Reference Galehouse1971).
RESULTS
Geomorphology
Stabilized eolian dunes are observed in an area of approximately 16,000 km2, which is 16 times larger than the Lençóis Maranhenses active dune field and extends to distances of up to 135 km from the present shoreline or 160 km along the main wind direction. The eolian sediments overlie the Miocene Barreiras Formation, which consists of sand, silt, and orthoconglomerate layers with ferricrete at the top of the formation (Fig. 3) and the Cretaceous Itapecuru Formation in the southwestern portion of the studied area (Vasconcelos et al., Reference Vasconcelos, Veiga, Colares, Ribeiro, Gomes, Medeiros and Forgiarini2004). In the southeast, the occurrence of paleodunes is discontinuous over older eolian deposits (Fig. 1). Stabilized parabolic eolian dunes (up to 20 m high) and deflation flats are predominant on the central part of the studied area (Fig. 4), which points to an eolian system under deflation processes and relatively low sand supply compared with the active Lençóis Maranhenses dune field. In contrast, eolian features linked to relatively high sand supply, such as barchanoid chains and precipitation ridges (up to 30 m high), occur bordering the southernmost limits of the paleodune system. The eolian deposits are 1 to 2 m thick in the low-sand-supply western areas, increasing in thickness to the east, where eolian sand deposits up to 15 m thick are observed in outcrops.
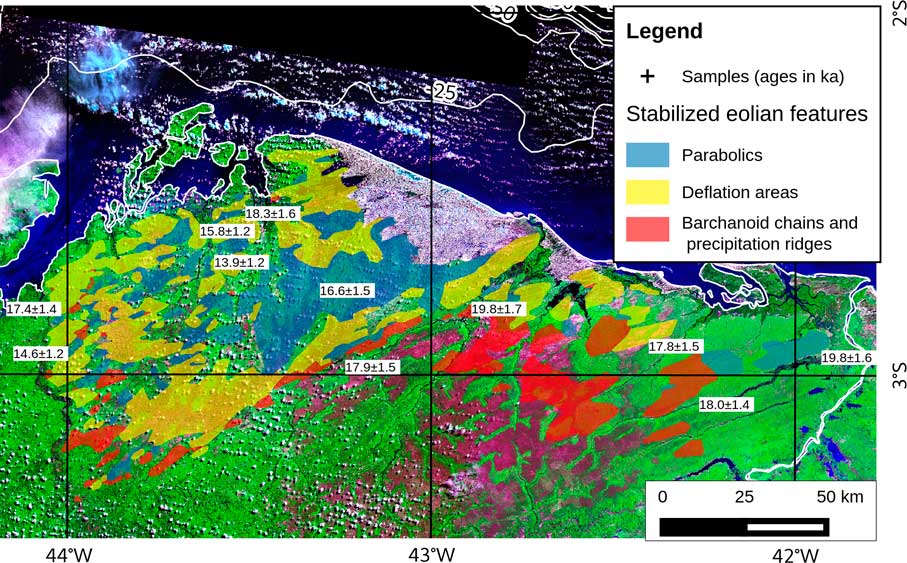
Figure 4 (color online) Optically stimulated luminescence (OSL) ages (in ka) and dominant types of stabilized eolian features. OSL ages were obtained from samples collected at the top of stabilized dunes. Landsat images correspond to the year 2000.
OSL ages
The studied sediment samples contain quartz grains with high luminescence sensitivity, low recuperation, and recycling ratios close to 1.0 for most of the aliquots. These OSL characteristics indicate quartz grains suitable for equivalent dose estimation. The only exceptions were samples LM-1a-3 and LM-24-EB, which showed some quartz aliquots with poor recycling ratios, requiring a higher number of measured aliquots for equivalent dose estimation. The burial environment is characterized by low dose rates, ranging from 0.29±0.02 to 1.08±0.08 Gy/ka, as a result of low potassium, uranium, and thorium contents. Thus, the cosmic dose rate has a relatively high contribution to the total dose rate. Significant variations of the cosmic dose rate over time are not expected for samples collected at the top of stabilized dunes with still preserved morphology because of high rates of sediment deposition followed by surface stabilization. No significant changes in burial depth occur under this condition. On the other hand, older eolian sediments buried by younger eolian sediments can show significant changes in burial depth through time and high age uncertainty. The burial ages obtained for the studied eolian deposits range from 13.9±1.2 to 240±20 ka and represent several phases of eolian sediments accumulation and preservation (Table 2). The last phase of stabilization of the paleodune system, derived from the ages of the stabilized eolian features, occurred in a relatively narrow time span from 19 to 14 ka ago. OSL ages from outcrops represent previous sediment accumulation and preservation phases around 27.0±2.0, 119±10, 145±13, and 240±20 ka. Ages from surface stabilized eolian features show a spatial pattern characterized by older ages near the coastline and younger ages inland (Fig. 4). Considering that luminescence ages for the top of surface paleodune features represent the last deposition of eolian sands prior to dune stabilization by vegetation (Chase, Reference Chase2009), the OSL ages indicate that the stabilization of the paleodune system started 19 ka ago near the coastline (older ages) and advancing until 14 ka ago inland (younger ages).
Table 2 Optically stimulated luminescence age results.

Grain size and heavy minerals
The studied paleodune deposits consist of well- to moderately sorted fine to medium sand. Grain-size distributions are mainly positively skewed, and rare granules occur in few samples. No differences between older eolian and stabilized eolian deposits were observed despite the presence of silt and clay fractions in the older eolian deposits (27.2–240 ka), probably resulting from postdepositional processes. The stabilized dune field deposits are slightly finer and better-sorted westward. The underlying Barreiras Formation sediments are classified as poorly sorted and strongly positively skewed fine sand.
Mean heavy mineral concentration in the very fine sand fraction is 2.1% (weight percent). The heavy minerals (average percent of grains in all samples) are mainly represented by zircon (58%), rutile (24%), kyanite (10%), sillimanite (3%), tourmaline (2%), and andalusite (1%). Trace minerals (less than 1%) include apatite, anatase, titanite, hornblende, and staurolite.
DISCUSSION
Paleogeography and sediment sources
The OSL age results are indicative of dune field activity during the different paleoclimate periods, with multiple ancient phases of eolian accumulation at least since the Marine Oxygen Isotope Stage (MIS) 7 to accumulation through stabilization by vegetation at MIS 2. The last Lençóis Maranhenses paleodune system activity and stabilization can be related to the last glacial period, synchronous with dune field development in continental areas of the Southern Hemisphere (Munyikwa, Reference Munyikwa2005; Singhvi and Porat, Reference Singhvi and Porat2008; Tripaldi and Zárate, Reference Tripaldi and Zárate2016). However, this is an expressive record of a coastal dune field development during low RSL in a tropical area, whereas initiation and growing of coastal dune fields is commonly associated with rising/high RSL (Lees, Reference Lees2006; Giannini et al., Reference Giannini, Sawakuchi, Martinho and Tatumi2007; Bateman et al., Reference Bateman, Carr, Dunajko, Holmes, Roberts, McLaren, Bryant, Marker and Murray-Wallace2010). This association has a preservation bias because low-stand RSL dune fields tend to be eroded or drowned during the following transgression. Thus, when preserved, low-stand sea dune fields are less accessible to studies than high-stand sea dune fields. In the study area, the preservation and outcropping of low-stand dune fields were probably possible as a result of their huge dimensions and their development over elevated areas (10 to 90 m above present sea level). With a continental margin break at −70 m, the paleoshoreline remained at approximately 65 km from the present shoreline during almost the whole last low-stand RSL period. Therefore, considering the dimensions and ages of the studied paleo–dune field, it is valid to assume the continental margin adjacent to the Lençóis Maranhenses region as having been a contiguous paleodune area. The large predominance of terrigenous sands as seabed sediments in the continental shelf offshore the studied paleodune deposits (Kowsmann and Costa, Reference Kowsmann and Costa1979) suggests the presence of drowned eolian deposits.
Paleodunes with stabilization ages of 19.8±1.6 ka (LM-30) and 18.0±1.4 ka (LM-39) are eastward limited by the Parnaíba River (Fig. 4). Considering that the main direction of dune migration is from NE to southwest, the Parnaíba River was a potential source of sand for the paleodune system. Deposits of the accumulation (older eolian deposits) and stabilization (stabilized dunes) phases have a very similar heavy mineral assemblage, which differs from the heavy mineral assemblage of the Parnaíba River sands (Hilbert et al., Reference Hilbert, Guedes and Giannini2016). The distinction between heavy minerals of paleodunes and river sands is evidenced by the content of metamorphic minerals such as kyanite, sillimanite, andalusite, and staurolite. These metamorphic minerals represent about 15% of the heavy mineral suite in stabilized dune field sands and 3% in the Parnaíba River sands (Hilbert et al., Reference Hilbert, Guedes and Giannini2016). Also, the stabilized dune field deposits have a heavy mineral assemblage very similar to that observed in sands of the active dune field. Metamorphic minerals represent 16% of the heavy mineral assemblage in the active dune field sands (Hilbert et al., Reference Hilbert, Guedes and Giannini2016), with the exception of hornblende, which is almost absent in paleodunes because of postdepositional dissolution but shows significant concentration (7%) in active dune field sands. The Barreiras Formation has a heavy mineral assemblage very similar to the studied eolian deposits but with the presence of authigenic anatase and leucoxene.
The similarity between stabilized and active dune field heavy mineral assemblages (Hilbert et al., Reference Hilbert, Guedes and Giannini2016) suggests that eolian sediments are supplied not only by the Parnaíba River but also by other sediment sources eastward of the Parnaíba River mouth, which feed the coastal eolian system through westward alongshore drift (Fig. 5). Thereby, the formation of dunes nearby the Parnaíba River channel indicates a lower water discharge before around 19 ka. This condition (Fig. 6) would be unable to stop dune migration and the littoral drift, as observed in the modern eolian system with the internal drainages of the Lençóis Maranhenses active dune field (Hilbert et al., Reference Hilbert, Guedes and Giannini2016).

Figure 5 (color online) Map of major river systems, rock types outcropping in northeastern Brazil, and heavy mineral assemblages found in fluvial, coastal (Hilbert el al., 2016), and eolian sediments. Pie graphs represent the area distribution of the types of rocks cut by Piauí (Parnaíba River basin) and Ceará drainage networks. Arrows are the main sedimentary transport paths. Geologic map based on Schobbenhaus and Bellizia (Reference Schobbenhaus and Bellizia2001). And, andalusite; Ky, kyanite; Sill, sillimanite; St, staurolite.

Figure 6 (color online) Paleogeography of the studied sector of the northeastern coast of Brazil at 18 ka ago. Dune fields would have covered an estimated area of at least 16,000 km2 or possibly even 26,000 km2 if a paleoshoreline at the continental margin break were to be considered. This would be one of the largest Quaternary dune field systems in South America.
Development of coastal dune fields in northeastern Brazil and changes in the ITCZ
The precipitation and wind strength in northern South America are strongly dependent on the dynamics of the ITCZ. Seasonal changes in the ITCZ are correlated with variations in the intensity of trade winds in northeastern Brazil (Tsoar et al., Reference Tsoar, Levin, Porat, Maia, Herrmann, Tatumi and Claudino-Sales2009). Studies performed in the last decades that improved the understanding of paleoclimate in tropical South America (e.g., Seltzer et al., Reference Seltzer, Rodbell and Burns2000; Cruz et al., Reference Cruz, Vuille, Burns, Wang, Cheng, Werner, Edwards, Karmann, Auler and Nguyen2009; Kanner et al., Reference Kanner, Burns, Cheng and Edwards2012; Zhang et al., Reference Zhang, Chiesse, Mulitza, Zabel, Trindade, Hollanda, Dantas, Govin, Tiedemann and Wefer2015) are focused on temperature and precipitation changes. The reconstruction of wind changes for South America is restricted to high-latitude arid zones, where the transport and accumulation of eolian dust occur in marine depositional settings (Gaiero, Reference Gaiero2007). Also, the record of Late Pleistocene climate changes in northeastern Brazil is based on marine sediment cores from the continental margin offshore the Parnaíba River mouth (Arz et al., Reference Arz, Patzold and Wefer1999; Behling et al., Reference Behling, Arz, Pätzold and Wefer2000; Zhang et al., Reference Zhang, Chiesse, Mulitza, Zabel, Trindade, Hollanda, Dantas, Govin, Tiedemann and Wefer2015), on speleothems (Cruz et al., Reference Cruz, Vuille, Burns, Wang, Cheng, Werner, Edwards, Karmann, Auler and Nguyen2009), and on geochemical and palynological data from the Caçó Lake (Ledru et al., Reference Ledru, Mourguiart, Ceccantini, Turcq and Sifeddine2002; Sifeddine et al., Reference Sifeddine, Alburquerque, Ledru, Turcq, Knoppers, Martin and Mello2003; Jacob et al., Reference Jacob, Disnar, Boussafir, Sifeddine, Turcq and Alburquerque2004), which is located in the central portion of our study area. These climate records are for inland areas and are based mainly on changes in precipitation and/or vegetation cover. There is little information about the climate changes in coastal areas, and very few studies deal with changes in wind systems (Tsoar et al., Reference Tsoar, Levin, Porat, Maia, Herrmann, Tatumi and Claudino-Sales2009) because of the lack of paleoclimate proxies sensitive to changes in wind strength in tropical areas.
Changes in precipitation and trade wind strength attributable to shifts of the ITCZ in the study area are the strongest forces acting on the building and stabilization of coastal eolian dune fields. NE trade winds are responsible for the wave systems that control the westward alongshore sediment transport as well as the onshore eolian sediment transport that supports dune field construction (Tsoar et al., Reference Tsoar, Levin, Porat, Maia, Herrmann, Tatumi and Claudino-Sales2009). Thus, NE trade winds control the sand supply to the beach system and onshore eolian transport capacity of sand. Hence, coastal dune field construction in the Lençóis Maranhenses area would record intensification of the action of NE trade winds on the northern Brazilian coast. Inversely, the dune field stabilization would record weakening of these trade winds and an increase of humidity. In the northeastern Brazilian coastal zone, the modern scenario represented by the coexistence of active and stabilized dune fields characterizes a bistability regime (sensu Yizhaq et al., Reference Yizhaq, Ashkenazy and Tsoar2009). With high annual precipitation rates, a decrease of wind strength would lead the eolian system to full stabilization by vegetation. Otherwise, an increase of wind strength would drive the system to activation if climatic conditions were not favorable to maintain the vegetation and the stabilized sediments became available to the eolian transport. However, conditions leading to a major reactivation of the stabilized dune field probably have not occurred since around 13.9±1.2 ka (sample LM-24), as also already observed by Tsoar et al. (Reference Tsoar, Levin, Porat, Maia, Herrmann, Tatumi and Claudino-Sales2009) in other regions along the northeastern Brazilian coast. The modern scenario, with high RSL and water table, widespread vegetation cover, and moderate winds, reflects a state with low sediment availability but with relatively high transport capacity and huge sediment supply from the beach system. With no sediment available to wind transport within the paleo–dune field, a new phase of dune field construction is underway at the active Lençóis Maranhenses dune field. Climate changes able to decrease wind strength and favor vegetation growth leading to the accumulation of the sand strata should be recorded by OSL ages of dune stabilization over the whole dune field. At Lençóis Maranhenses paleodune system, this is indicated by burial ages of sediment samples retrieved from the top of stabilized dunes with preserved depositional morphology (Fig. 3D). The time period required for dune field construction and stabilization is dependent on the size of the eolian system. Larger dune fields take more time to develop than smaller dune fields. As a result, the resolution of climatic variations that can be recorded by ages of eolian system stabilization depends on dune field size. Thus, the stabilization of larger dune fields will depend on the occurrence of extreme and/or long duration climate changes.
Response of the Lençóis Maranhenses paleodune system to deglacial abrupt climate events and sea level rise
The comparison of the Lençóis Maranhenses eolian record with Caçó Lake and northeastern Brazil marine sediment records (Fig. 7) allows us to understand the response of coastal eolian dunes and their vegetation cover to climate changes and RSL changes as boundary conditions. This is especially important for constraining the response of coastal areas to climate changes and for improving the spatial reconstruction of Late Pleistocene paleoclimate. The paleoclimate data set provided by sediment cores from the Caçó Lake (Ledru et al., Reference Ledru, Mourguiart, Ceccantini, Turcq and Sifeddine2002; Sifeddine et al., Reference Sifeddine, Alburquerque, Ledru, Turcq, Knoppers, Martin and Mello2003; Jacob et al., Reference Jacob, Disnar, Boussafir, Sifeddine, Turcq and Alburquerque2004) and offshore northeastern Brazil (Behling et al., Reference Behling, Arz, Pätzold and Wefer2000; Zhang et al., Reference Zhang, Chiesse, Mulitza, Zabel, Trindade, Hollanda, Dantas, Govin, Tiedemann and Wefer2015) comprises records from the same period of activity and subsequent stabilization as the Lençóis Maranhenses paleodune system. The entire period of activity of the Lençóis Maranhenses paleodune system cannot be determined precisely in this study, but the luminescence age of 27.2±2.2 ka (LM-27-T sample) represents a sediment accumulation phase that indicates eolian deposits building and activity of the eolian system during the last glacial period. Additionally, assuming that the Caçó Lake was formed by the damming of a drainage by these paleodunes and that its older sediments were deposited around 21 cal ka BP (Sifeddine et al., Reference Sifeddine, Alburquerque, Ledru, Turcq, Knoppers, Martin and Mello2003), dune field construction occurred during the last glacial maximum (23–21 ka). This agrees with high and variable influx of quartz to Caçó Lake between 21 and 17.4 cal ka related to nearby active dunes (Sifeddine et al., Reference Sifeddine, Alburquerque, Ledru, Turcq, Knoppers, Martin and Mello2003) and representing sediment available for wind transport. This period of dune field activity in the Caçó Lake area ends approximately at the onset of the dune field stabilization period from 19 to 14 ka ago given by our OSL ages. Moreover, our results suggest that dune stabilization started close to the shoreline and progressed downwind, with dunes still active at the Caçó Lake region between 17.9±1.5 ka (LM-51 sample) and 16.6±1.5 ka (LM-19B sample). Stabilization starting close to the shoreline suggests a significant decrease in sediment supply from the beach system accompanied by a decline in transport capacity of the wind and/or less sediment supply from littoral drift. Widespread occurrence of eolian features typical of relatively low sand supply, such as parabolic dunes and deflation areas, is the result of low sediment availability attributable to a higher water table and vegetation growth. Sedimentation rates in the continental margin offshore the Parnaíba River were very low at least from 30 ka until the beginning of the Heinrich stadial 1 (HS-1) event at 18 ka (Zhang et al., Reference Zhang, Chiesse, Mulitza, Zabel, Trindade, Hollanda, Dantas, Govin, Tiedemann and Wefer2015), which can be attributed to low precipitation rates in the Parnaíba River catchment until 18 ka. A wetter period, with high water table, is compatible with lake level rise in Caçó Lake between 17.4 and 12.9 ka, inferred by Sifeddine et al. (Reference Sifeddine, Alburquerque, Ledru, Turcq, Knoppers, Martin and Mello2003) based on the increased goethite and siderite formation in the lake during this time interval. Meanwhile, Jacob et al. (Reference Jacob, Disnar, Boussafir, Sifeddine, Turcq and Alburquerque2004) argue that the same humid period starts at 19.2 cal ka as demonstrated by a major presence of silt and kaolinite pointing to denser vegetation and soil development in the Caçó Lake area. This wetter period coincides with dune stabilization in the Lençóis Maranhenses eolian system from 19 to 14 ka, and high sedimentation rates in the continental margin resulted from precipitation increase in the Parnaíba River catchment (Zhang et al., Reference Zhang, Chiesse, Mulitza, Zabel, Trindade, Hollanda, Dantas, Govin, Tiedemann and Wefer2015) during the HS-1. High water table, denser vegetation, soil development, and increase of precipitation limited sediment availability and transport capacity and thus led the eolian system to stabilize and accumulate (Fig. 7).

Figure 7 Comparison of sediment state (sensu Kocurek, Reference Kocurek1999) of the Lençóis Maranhenses area from the last 30 ka with relative sea level (RSL) changes (Corrêa, Reference Corrêa1996; Rabineau et al., Reference Rabineau, Berná, Olivet, Aslanian, Guillocheau and Joseph2006), Caçó Lake (Ledru et al., Reference Ledru, Mourguiart, Ceccantini, Turcq and Sifeddine2002; Sifeddine et al., Reference Sifeddine, Alburquerque, Ledru, Turcq, Knoppers, Martin and Mello2003; Jacob et al., Reference Jacob, Disnar, Boussafir, Sifeddine, Turcq and Alburquerque2004), and marine sedimentation rates in the continental margin near the Parnaíba River mouth (Zhang et al., Reference Zhang, Chiesse, Mulitza, Zabel, Trindade, Hollanda, Dantas, Govin, Tiedemann and Wefer2015). BA, Bølling-Allerød interstade; Clal, contemporaneous influx (availability limited); CLltl, contemporaneous and lagged influx (transport limited); HS-1, Heinrich stadial 1; HS-2, Heinrich stadial 2; Sal, stored sediment (availability limited); Stal, stored sediment (availability and transport limited); YD, Younger Dryas.
The HS-1 event has been associated with weakening of the Atlantic meridional overturning circulation (AMOC) and a consequent southward shift of the ITCZ in northern South America (Weldeab et al., Reference Weldeab, Schneider and Kolling2006; Baker et al., Reference Baker, Diz, Vautravers, Pike, Knorr, Hall and Broecker2009; Mulitza et al., Reference Mulitza, Chiessi, Schefuß, Lippold, Wichmann, Antz and Mackensen2017) and Africa (Stager et al., Reference Stager, Ryves, Chase and Pausata2011). This southward shift of the ITCZ is suggested by the increase of sea surface temperatures (SSTs) at the equatorial Atlantic around the northeastern Brazilian coast (Weldeab et al., Reference Weldeab, Schneider and Kolling2006; Jaeschke et al., Reference Jaeschke, Ruhlemann, Arz, Heil and Lohmann2007) and the relatively low SST in the Cariaco basin (Lea et al., Reference Lea, Pak, Peterson and Hughen2003; Fig. 8). The drier climate through most of the Late Pleistocene suggests a higher eolian drift potential at the northeastern Brazilian coast under trade winds and weak ITCZ influence. Also, the shoreline (foreshore), as sediment source to the eolian system, was relatively stable in the continental margin break during most of the last glacial period, which must have favored the development of larger coastal dune fields. Therefore, with the ITCZ placed northward, the boundary conditions of the eolian system are favorable to dune field construction, with high sediment supply, high sediment availability and high transport capacity, and contemporaneous and lagged influx (transport-limited) sediment state (sensu Kocurek, Reference Kocurek1999; Fig. 7). These favorable conditions for the development of dune fields shifted to conditions suitable for dune stabilization at the onset to the HS-1. The stabilization of the Lençóis Maranhenses paleodune field, indicated by our OSL ages, suggests decreased trade wind strength over the Lençóis Maranhenses eolian system during HS-1. This condition resulted in low eolian drift potential and reduced coastal sediment input by alongshore wave transport. Also, high water table and vegetation growth decrease sediment availability resulting in a stored sediment (available and transport-limited) state (Fig. 7; Kocurek, Reference Kocurek1999). This coastal paleoclimate scenario is consistent with a southward shift of the ITCZ during the HS-1 (Denton et al., Reference Denton, Anderson, Toggweiler, Edwards, Schaefer and Putnam2010) induced by the weakening of the AMOC (Mulitza et al., Reference Mulitza, Chiessi, Schefuß, Lippold, Wichmann, Antz and Mackensen2017).

Figure 8 Comparison of optically stimulated luminescence (OSL) ages of dune field stabilization in the Lencóis Maranhenses area, relative sea level (RSL) changes (Corrêa, Reference Corrêa1996; Rabineau et al., Reference Rabineau, Berná, Olivet, Aslanian, Guillocheau and Joseph2006), sea surface temperature (SST) near the northeastern Brazil coast (GeoB 3910-2: Jaeschke et al., Reference Jaeschke, Ruhlemann, Arz, Heil and Lohmann2007; GeoB 3129-1: Weldeab, et al., Reference Weldeab, Schneider and Kolling2006), SST on Cariaco basin (PL07-39PC: Lea et al., Reference Lea, Pak, Peterson and Hughen2003), and δ18O of North Greenland Ice Core (North Greenland Ice Core Project members, 2004). OSL ages of dune field stabilization are coincident with the increase of SST near the northeastern Brazilian coast and relatively low SST on Cariaco basin, suggesting the weakening of trade winds during the Heinrich stadial 1 (HS-1) event. Light-gray area indicates period favorable to stabilization of eolian systems (BA, Bølling-Allerød interstade); dark gray areas indicate periods favorable to eolian system development (HS-1; YD, Younger Dryas) NGRIP, North Greenland Ice Core Project.
The Younger Dryas (YD) stade around 12–13 ka is recorded in the Caçó Lake sediments as a very humid period (Ledru et al., Reference Ledru, Mourguiart, Ceccantini, Turcq and Sifeddine2002; Sifeddine et al., Reference Sifeddine, Alburquerque, Ledru, Turcq, Knoppers, Martin and Mello2003) and in the continental margin offshore the Parnaíba River mouth as a phase of high sedimentation rates (Zhang et al., Reference Zhang, Chiesse, Mulitza, Zabel, Trindade, Hollanda, Dantas, Govin, Tiedemann and Wefer2015). However, this wetter episode after the HS-1 is not seen in the Lençóis Maranhenses eolian record. The lack of OSL ages of stabilized dunes during the YD stade is suggestive of no eolian deposition, which can be explained by the short period of favorable conditions for dune field construction during the Bølling-Allerød (BA) interstade between the HS-1 and YD. The increase in trade wind strength during the BA interstade would be below the threshold to reactivate the stabilized dune field. Also, the RSL rising during the deglaciation maintained the water table high and kept the system with low sediment availability. Probably, in similarity to the modern scenario, the eolian dune field restarted a new phase of construction from the beach system. However, the dune field developed during the BA interstade would have had a smaller size and/or would have been located in a submerged area considering that the coastline was at the outer continental shelf in this epoch. Thus, eolian dunes that potentially developed during the BA interstade and stabilized through the YD stade were submerged or eroded during the early Holocene coastline transgression. Another Heinrich event, the Heinrich stadial 2 (HS-2) event, was recorded in sediment cores by Zhang et al. (Reference Zhang, Chiesse, Mulitza, Zabel, Trindade, Hollanda, Dantas, Govin, Tiedemann and Wefer2015) in the continental margin near the Parnaíba River mouth, but their sedimentation rates are considerably lower than during the HS-1 event. Even if HS-2 had triggered a stabilization event in the dune field, the period after HS-2 was more favorable to sedimentary reworking because of the low RSL (high availability), drier glacial climate with the ITCZ northward, and a longer period of time compared with the BA interstade. In order to investigate if the Lençóis Maranhenses has recorded other climate events, such as HS-2, it is necessary to study the preserved eolian deposits, at outcrops, with high-resolution sampling.
CONCLUSIONS
OSL ages allow us to determine the chronology of stabilization of one of the largest Quaternary dune field systems (at least 16,000 km2) recorded in South America. Dune stabilization by vegetation growth occurred during a 5 ka period (from 19 to 14 ka) and was triggered by a decrease in wind strength and an increase in precipitation during the HS-1 event. This climate shift combined with deglacial RSL rise drove conditions of reduced sediment availability and dune field stabilization. The 5 ka time period for dune field stabilization shows the spatially irregular and resilient response of the eolian system to millennial abrupt climate changes. The diachronous response time of the eolian system to climate variations affects its capacity to record successive climate events separated by time intervals smaller than the recurrence time of the dune field development or reactivation, as seems to be the case for Late Pleistocene climate events like the HS-1 event and the successive YD event. The eolian record provided by the Lençóis Maranhenses paleodune system indicates a weakening of trade winds during the HS-1 event and provides additional information for solving the northeastern Brazil deglacial paleoclimate puzzle.
ACKNOWLEDGMENTS
This work was supported by FAPESP (Fundação de Amparo à Pesquisa do Estado de São Paulo) grants 2009/54232-4 and 2009/10521-2 and by CNPq (Conselho Nacional de Desenvolvimento Científico e Tecnológico) grants 303530/2009-0 and 307055/2013-2. We would like to thank the editors Ashok Singhvi and Lewis Owen for handling the manuscript and the two anonymous reviewers who provided constructive critical remarks on the manuscript.
SUPPLEMENTARY MATERIAL
For supplementary material/s referred to in this article, please visit https://doi.org/10.1017/qua.2017.79