INTRODUCTION
Shandong Province in eastern China is one of the major areas of loess accumulation outside the Chinese Loess Plateau (CLP) (Fig. 1a and b) (Liu, Reference Liu1985). Stratigraphic and sedimentological research on the Shandong loess has continued since the 1890s (Skertchly, Reference Skertchly1895; Zdansky, Reference Zdansky1925; Pearson, Reference Pearson1928; Yang, Reference Yang1936; Liu, Reference Liu1965), and chronological and paleoclimatic studies, as well as provenance investigations, have become a research focus since the 1980s (Li and Zhao, Reference Li and Zhao1981; Cao et al., Reference Cao, Li and Shi1987; Xu et al., Reference Xu, Ding, Yu and Ni2015, Reference Xu, Kong, Jia, Miao and Ding2018; Tian et al., Reference Tian, Sun, Lü, Cao, Zhang and Lü2019). However, the provenance of the Shandong loess is debated. Early research, based on modern dust storm observations, suggested that the fine particles in the coastal loess of Shandong Province were transported by the East Asian winter monsoon from the arid region in northwestern China, while the coarse particles were derived from the adjacent continental shelf exposed during glacial periods, transported by local northerly winds (Liu, Reference Liu1985). Subsequently, the presence of marine microfossils (e.g., foraminifera and radiolarians) in the coastal loess suggested that the exposed continental shelf was an important source (Cao et al., Reference Cao, Li and Shi1987, Reference Cao, Liu, Shi, Chang, Song, Yuan and Guo1993; Cheng et al., Reference Cheng, Li, Lv and Ju1995, Reference Cheng, Fu and Ju1996; Zhao, Reference Zhao1996). For the loess deposits in the piedmont of the Central Shandong Mountains (PCSM) in Shandong Province, several researchers have proposed that they are a mixture of local dust from the adjacent floodplains or the continental shelf of Bohai Gulf and long-distance-transported dust from the arid region in northwestern China, on the basis of the characteristics of grain size, mineral and elemental composition, and the absence of marine microfossils (Zhang et al., Reference Zhang, Xin and Nie2004, Reference Zhang, Xin, Jiang, Jiang and Huang2005; Xin, Reference Xin2005; Wang et al., Reference Wang, Peng, Feng, Zhang and Ding2014; Ding et al., Reference Ding, Cao, Xu and Ni2015; Jia et al., Reference Jia, Xu, Kong and Ding2016; Xu et al., Reference Xu, Ni and Ding2016). However, other studies, using elemental analyses, have concluded that fluvial sediments in the North China Plain are the main source (Peng et al., Reference Peng, Hao, Wang, Ding, Zhang, Wang and Guo2016). Therefore, the provenance of the Shandong loess needs to be further investigated.
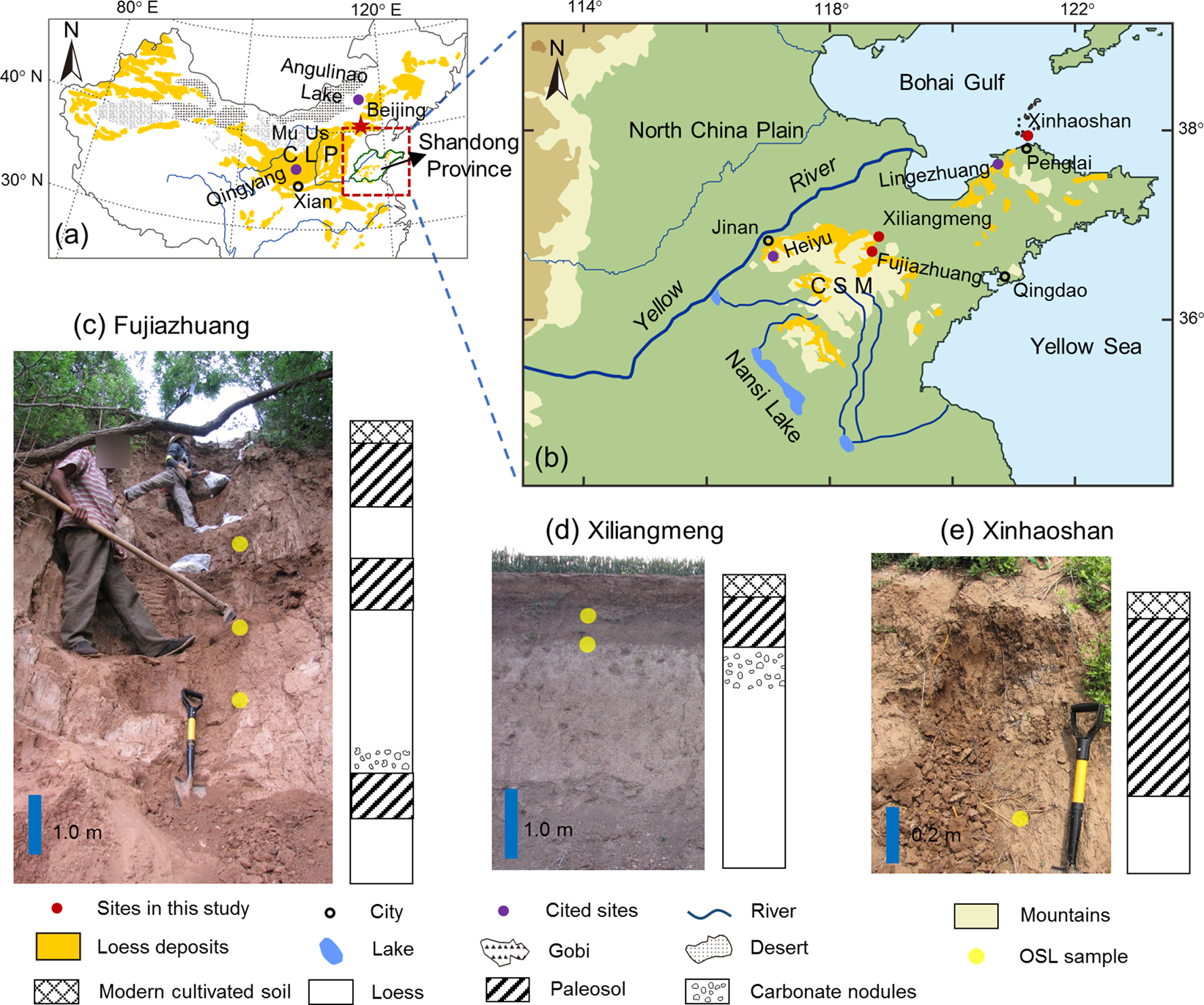
Figure 1. (color online) Distribution of loess deposits in China (a) (modified from Liu, Reference Liu1985; Zhang et al., Reference Zhang, Xin and Nie2004); location of study sites (b) and photographs and stratigraphic columns of loess sections at Fujiazhuang (c), Xiliangmeng (d), and Xinhaoshan (e). CLP, Chinese Loess Plateau; CSM, Central Shandong Mountains. The locations of Lake Angulinao (Lu et al., Reference Lu, Fang, Friedrich and Song2018) and the loess sections at Heiyu (Kong, Reference Kong2018), Lingezhuang (Tian et al., Reference Tian, Sun, Lü, Cao, Zhang and Lü2019), and Qingyang (Yang and Ding, Reference Yang and Ding2008) are also shown.
The loess deposits of the CLP are transported by the East Asian winter monsoon from the vast arid region in northwestern China (e.g., Liu, Reference Liu1985; Sun, Reference Sun2002; Chen et al., Reference Chen, Li, Yang, Rao, Lu, Balsam and Ji2007; Sun et al., Reference Sun, Tada, Chen, Liu, Toyoda, Tani, Ji and Isozaki2008; Yan et al., Reference Yan, Sun, Chen and Ma2014). Previous studies have demonstrated that the grain size of loess deposits in the CLP is controlled primarily by the source-to-sink distance: the greater the dust transport distance, the finer the grain size of the dust deposits (Yang and Ding, Reference Yang and Ding2004, Reference Yang and Ding2008, Reference Yang and Ding2017; Ding et al., Reference Ding, Derbyshire, Yang, Sun and Liu2005). Thus, the grain size of the loess deposits in the CLP shows a southward-fining pattern, from which a model based on dust transport distance and grain-size parameters was developed (Yang and Ding, Reference Yang and Ding2004, Reference Yang and Ding2017). This approach can be used to explore the provenance of the Shandong loess.
In the CLP, the coarse grain size and low magnetic susceptibility (MS) values of the loess units indicate a cold and dry glacial climate, while the fine grain size and high MS of the paleosol units indicate a warm and humid interglacial climate (Heller and Liu, Reference Heller and Liu1984; An et al., Reference An, Kukla, Porter and Xiao1991; Ding et al., Reference Ding, Yu, Rutter and Liu1994, Reference Ding, Derbyshire, Yang, Yu, Xiong and Liu2002; Yang and Ding, Reference Yang and Ding2004, Reference Yang and Ding2008, Reference Yang and Ding2010). Therefore, grain size and MS have become two of the most widely used proxies for characterizing loess-paleosol stratigraphy. However, it remains unclear whether these two proxies are effective for characterizing loess-paleosol alternations (i.e., glacial–interglacial cycles) in loess deposits outside the CLP region. Here we present grain size and MS records of three loess-paleosol sequences in Shandong Province. We then compare them with the results from previously studied loess sections in Shandong Province and the CLP, with the aim of addressing the provenance and paleoclimate significance of the Shandong loess.
GEOLOGICAL SETTING
The area of loess accumulation in Shandong Province lies within the warm temperate zone with a subhumid monsoon climate. The modern mean annual temperature and precipitation in the studied region are 11–14°C and 550–950 mm, respectively, with over 60% of the precipitation concentrated in the summer season. The landforms comprise mountains in the central part, plains in the west and north, and hills in the east (Bureau of Geology and Mineral Resources of Shandong Province, 1991). The loess deposits are distributed in the PCSM and the coastal area (including the offshore islands) of Bohai Gulf (Fig. 1b; Liu, Reference Liu1985; Zhang et al., Reference Zhang, Xin and Nie2004). Typically they have thicknesses of <10 m (Zhang et al., Reference Zhang, Xin and Nie2004), and very thick (>30 m) loess sequences are preserved only in the PCSM (Zheng et al., Reference Zheng, Zhu, Huang and Lu1994; Peng et al., Reference Peng, Zhu, Xiao, Qiao, Gao and Chen2011).
MATERIALS AND METHODS
Three loess sections—at Fujiazhuang (36°39.6′N, 118°26.8′E), Xiliangmeng (36°52.2′N, 118°28.5′E), and Xinhaoshan (37°54.8′N, 120°43.2′E) (Fig. 1b)—were logged and sampled. The Fujiazhuang and Xiliangmeng sections are situated in the PCSM and have elevations of ~163 m and ~23 m above sea level (asl), respectively. The Xinhaoshan section (~20 m asl) is situated in South Changshan Island near Penglai City (Fig. 1b).
Samples
In the Fujiazhuang section, the upper part (~7.45 m thick) was sampled. The section can be divided into seven stratigraphic units comprising a modern cultivated soil and three loess-paleosol couplets (Fig. 1c). The two paleosol layers (between the depths of 0.4 m and 3.05 m) in the upper part of the section are dark gray in color. Abundant carbonate nodules are present between the depths of 5.25 m and 5.65 m, immediately above the reddish paleosol unit in the lower part of the section. The Xiliangmeng section is 4 m thick and comprises three units from top to bottom: a modern cultivated soil, a darkish paleosol, and a yellowish loess unit (Fig. 1d). Below the paleosol unit (i.e., in the uppermost part of the loess unit), carbonate nodules are common between the depths of 1.05 m and 1.5 m. The Xinhaoshan section is 1.1 m thick, consisting of a modern cultivated soil, a brownish paleosol, and a yellowish loess, from top to bottom (Fig. 1e).
A total of 174 samples were taken at 5–10 cm intervals from the three sections for grain size and MS analyses. Six samples were also collected for optically stimulated luminescence (OSL) dating (Fig. 1c–e). In addition, a modern dust storm sample was collected in Penglai City (Fig. 1b), which is located ~13 km south of the Xinhaoshan section, to compare its grain-size characteristics with those of the samples from the loess sections.
Methods
Dating
Chinese loess is composed mainly of silt-sized particles (Liu, Reference Liu1985; Yang and Ding, Reference Yang and Ding2004, Reference Yang and Ding2014; Peng et al., Reference Peng, Hao, Wang, Ding, Zhang, Wang and Guo2016; Xu et al., Reference Xu, Kong, Jia, Miao and Ding2018) and thus is suitable for fine-grained quartz (4–11 μm) OSL dating (Aitken, Reference Aitken1985). This method has been widely used to date the loess deposits of the CLP (e.g., Lu et al., Reference Lu, Wang and Wintle2007; Kang et al., Reference Kang, Wang and Lu2013), as well as those in Shandong Province (e.g., Peng et al., Reference Peng, Zhu, Xiao, Qiao, Gao and Chen2011; Kong et al., Reference Kong, Xu and Jia2017; Li et al., Reference Li, Li, Wang, Ma, Wang and Liang2019). In this study, the fine-grained quartz fraction (4–11 μm diameter) was selected for OSL dating. All samples were pretreated with 30% H2O2 and 37% HCl to remove organic matter and carbonates, respectively. The 4–11 μm polymineralic fraction was separated according to Stokes′ law and then immersed in 30% H2SiF6 for three days to obtain the fine-grained quartz component (Lu et al., Reference Lu, Zhang and Xie1988). Quartz aliquots were deposited on 9.7-mm-diameter stainless steel discs using acetone for further experiments (Lu et al., Reference Lu, Wang and Wintle2007).
Optical luminescence measurements were made with an automated Daybreak 2200 OSL reader equipped with infrared (880 ± 60 nm) and blue (470 ± 5 nm) LED units and a 90Sr/90Y beta source (0.048 Gy/s) for irradiation (Lu et al., Reference Lu, Wang and Wintle2007). The stimulation conditions were 125°C for 50 s with a power of ~45 mW/cm2 (Wang et al., Reference Wang, Lu and Li2005; Lu et al., Reference Lu, Wang and Wintle2007). Equivalent doses (De) were determined using the sensitivity-corrected multiple aliquot regenerative-dose (SMAR) protocol (Wang et al., Reference Wang, Lu and Li2005; Lu et al., Reference Lu, Wang and Wintle2007). These measurements were performed in the State Key Laboratory of Loess and Quaternary Geology in Xian. For dose rate determination, the concentrations of U (ppm), Th (ppm), and K (%) were measured using plasma emission spectrometry (U, Th) and inductively coupled plasma mass spectrometry (K) in the China Institute of Atomic Energy. The ages were calculated using the DRc program (Tsakalos et al., Reference Tsakalos, Christodoulakis and Charalambous2016) based on De and the dose rate.
MS and grain-size analyses
MS and grain-size analyses were conducted on bulk samples in the Key Laboratory of Cenozoic Geology and Environment, Chinese Academy of Sciences. Air-dried samples were weighed and packed in nonmagnetic plastic boxes and measured using a Bartington Instruments MS3 magnetic susceptibility meter. For grain-size analysis, samples of ~0.1–0.2 g were treated with diluted H2O2 and HCl on a hot plate to remove organic matter and carbonate, respectively. Ultrasonic treatment with the addition of a 0.05 mol/L solution of (NaPO3)6 was then used to disperse the samples before grain-size measurements, which were made using a Mastersizer 3000 laser particle-size analyzer.
RESULTS
Chronology
The OSL signals of all aliquots of each sample decayed to the background level in less than 10 seconds (Fig. 2a and d). The dose–response curve shows a growth trend denoting unsaturated signals (Fig. 2b and e). For each sample, the De values of all aliquots are normally distributed, suggesting that the quartz grains were bleached before burial (Fig. 2c and f). The average De of all aliquots was then used to calculate the age of the samples (Table 1).

Figure 2. (color online) De determination for two representative samples from the Fujiazhuang (FJZ-110; a–c) and Xiliangmeng (XLM-18; d–f) sections using the sensitivity-corrected multiple aliquot regenerative-dose (SMAR) protocol. (a, d) Decay curves of optically stimulated luminescence (OSL) intensity. (b, e) Corrected OSL dose response curves and De determination. (c, f) De distribution curves and cumulative probability curves.
Table 1. OSL dating results for six samples from the Fujiazhuang (FJZ), Xiliangmeng (XLM) and Xinhaoshan (XHS) sections.

The loess-paleosol units in the three sections are labeled using the Li-Si system, which is applied to the loess deposits of the CLP (Liu, Reference Liu1985; Ding et al., Reference Ding, Rutter and Liu1993; Yang and Ding, Reference Yang and Ding2010). The studied part of the Fujiazhuang section contains three paleosol units. The OSL samples from around the two closely spaced paleosols (0.4–3.05 m depth) in the upper part produced reversed dates: 11.0 ± 0.8 ka for the depth of 2.15 m and 8.5 ± 0.7 ka for the depth of 3.20 m (Fig. 3). The latter is around the transition from the glacial loess to the interglacial paleosol unit, which was possibly disturbed by increased biological activity during interglacial soil development. This bioturbation can result in an OSL age that is younger than the sample's burial age, which has been previously observed in eolian deposits (Bateman et al., Reference Bateman, Boulter, Carr, Frederick, Peter and Wilder2007; Miao et al., Reference Miao, Hanson, Wang and Young2010; Hanson et al., Reference Hanson, Mason, Jacobs and Young2015). Despite the apparent age inconsistency, the results confirm that the two paleosols were developed during the Holocene; thus, they are designated S0-1 and S0-2. The loess unit (L1) below the Holocene soil unit (S0) accumulated during the last glacial period, as indicated by the OSL age of 25.4 ± 4.0 ka at the depth of 4.3 m. The paleosol unit (S1) in the lower part of the Fujiazhuang section formed during the last interglacial period, according to previous studies (Fig. 3; Liu et al., Reference Liu, Li and Wang2000; Peng et al., Reference Peng, Zhu, Xiao, Qiao, Gao and Chen2011). The studied part of the Fujiazhuang section is thus correlative with the loess-paleosol sequence of the last glacial–interglacial cycle in the CLP (Yang and Ding, Reference Yang and Ding2014).

Figure 3. Optically stimulated luminescence (OSL) ages, median grain size (Md), magnetic susceptibility (χ), and grain size (<20 μm% and >63 μm%) of the loess-paleosol sequences at Fujiazhuang (a–d), Xiliangmeng (e–h), and Xinhaoshan (i–l).
In the Xiliangmeng section, the paleosol unit underlying the modern cultivated soil is visually striking in the field because of its dark color. The OSL samples from the middle (0.65 m depth) and lower (0.9 m depth) parts of the paleosol have ages of 11.3 ± 1.0 ka and 18.5 ± 1.5 ka, respectively, indicating that this sequence was deposited mainly since the last glacial period (Fig. 3). Likewise, the Xinhaoshan loess deposits accumulated since the last glacial period, with a basal age of 12.0 ± 0.9 ka for the Holocene soil unit (S0) (Fig. 3).
Grain-size distributions
The grain-size range of the loess samples from the Fujiazhuang, Xiliangmeng, and Xinhaoshan sections is 0.4–200 μm, and the distributions are bimodal (Fig. 4). In the Fujiazhuang and Xiliangmeng sections, the principal mode is between 30 μm and 40 μm, and in the Xinhaoshan section it is between 30 μm and 70 μm. In addition, all three sections have a secondary mode at 0.7–1 μm (Fig. 4a–c). The grain-size distributions of the paleosol samples are bimodal (e.g., the samples from the depths of 1.05 m [S0-1] and 5.85 m [S1], Fig. 4a), or trimodal (e.g., the samples from the depths of 2.65 m [S0-2] and 5.95 m [S1], Fig. 4a). The bimodal distribution is characterized by a principal mode at 30 μm or 50 μm and a minor mode at <1 μm, similar to the grain-size characteristics of the loess samples; by contrast, the trimodal distribution has two principal modes, of around 40 μm and 4 μm in the Fujiazhuang and Xiliangmeng sections (Fig. 4a and b) and around 70 and 10 μm in the Xinhaoshan section (Fig. 4c). In addition, there is a minor mode at <1 μm in all three sections (Fig. 4a–c). The modern dust sample from Penglai City has the grain-size range of 0.2–200 μm; the distribution is bimodal with a principal mode at 60 μm and a secondary mode near 0.5 μm (Fig. 4d).

Figure 4. Grain-size distributions of representative samples from the Fujiazhuang (a), Xiliangmeng (b), and Xinhaoshan (c) sections. Also shown are the grain-size distributions of a modern dust storm sample from Penglai City, loess samples from other sites in Shandong Province (Lingezhuang [Tian et al., Reference Tian, Sun, Lü, Cao, Zhang and Lü2019] and Heiyu [Kong, Reference Kong2018]), the central Chinese Loess Plateau (Qingyang [Yang and Ding, Reference Yang and Ding2008]), and a shallow lacustrine sample from Lake Angulinao in northeastern China (Lu et al., Reference Lu, Fang, Friedrich and Song2018) (d).
In contrast to the pattern of coarse loess grain size and fine paleosol grain size observed in loess-paleosol sequences across the CLP, the median grain size in the Fujiazhuang section shows no systematic changes associated with the loess-paleosol alternations, except that several coarse grain-size spikes occur in both loess and paleosol units (Fig. 3a). Moreover, the S1 paleosol unit is even coarser grained than the loess units above and below (Fig. 3a). In the Xiliangmeng section, the median grain-size values are centered around 10 μm in S0 and within the range of 15–35 μm in L1 (Fig. 3e). In the Xinhaoshan section, S0 has median grain sizes of 15 to 35 μm with large variations (Fig. 3i). The sand content (>63 μm%) in the Fujiazhuang and Xiliangmeng sections is generally less than 10% but reaches 20% in a few samples from soil units S0 and S1, while the sand content is up to 30% in the Xinhaoshan section (Fig. 3). In all three sections, the sand content exhibits the same trend of variation as that of the median grain size, but the pattern is the opposite to that of the <20 μm particle content (Fig. 3). In general, the grain size is finer in the loess deposits of the PCSM area (Fujiazhuang and Xiliangmeng) than in those of the offshore island (Xinhaoshan).
Magnetic Susceptibility
In the three loess-paleosol sequences, both the loess and paleosol units are characterized by low MS values (Fig. 3). In the Fujiazhuang section, the highest MS values (~80 × 10–8 m3/kg) are observed in the modern cultivated soil and in the last interglacial paleosol unit (S1) (Fig. 3b), while the values for the Holocene soil (S0) and the last glacial loess unit (L1) fall within the range of 20–60 × 10–8 m3/kg (Fig. 3b). In the Xiliangmeng section, the MS values are as low as 8–20 × 10–8 m3/kg, except for relatively high values in the modern cultivated soil (Fig. 3f). Likewise, in the Xinhaoshan section, the modern cultivated soil has high MS values (>100 × 10–8 m3/kg), whereas the underlying S0–L1 couplet has low values (~60 × 10–8 m3/kg) (Fig. 3j).
DISCUSSION
Provenance of the Shandong loess
It is widely accepted that the arid region in northwestern China is the main source of the loess deposits of the CLP (e.g., Liu, Reference Liu1985; Sun, Reference Sun2002; Chen et al., Reference Chen, Li, Yang, Rao, Lu, Balsam and Ji2007; Sun et al., Reference Sun, Tada, Chen, Liu, Toyoda, Tani, Ji and Isozaki2008; Yan et al., Reference Yan, Sun, Chen and Ma2014). Previous studies have shown that the grain size of loess is controlled primarily by the distance between the source area and the site of deposition, while the effect of the transporting wind intensity is minor (Ding et al., Reference Ding, Sun and Liu1999, Reference Ding, Derbyshire, Yang, Sun and Liu2005; Yang and Ding, Reference Yang and Ding2004, Reference Yang and Ding2008, Reference Yang and Ding2017). On the basis of the grain-size results for 53 loess sections from across the CLP, Yang and Ding (Reference Yang and Ding2008) found that with increasing distance to the southern margin of the Mu Us Desert, the median grain size of the last glacial loess unit (L1) decreases rapidly from 50–60 μm in the north (within ~100 km of the desert margin) to 15–20 μm in the south (~360 km from the desert margin). For the last interglacial paleosol unit (S1), the median grain size gradually decreases southward, from 14–16 μm in the north to 8–9 μm in the south (Yang and Ding, Reference Yang and Ding2004, Reference Yang and Ding2008, Reference Yang and Ding2017). On the basis of the spatial pattern of loess grain-size changes in the CLP, a relationship between grain size and dust transport distance was established (Yang and Ding, Reference Yang and Ding2004, Reference Yang and Ding2017):

where X is the median grain size (μm) of loess deposits and Y (km) is the minimum source-to-sink distance.
The grain-size distributions of all of the loess samples and most of the paleosol samples in the Fujiazhuang, Xiliangmeng, and Xinhaoshan sections are bimodal and positively skewed (Fig. 4). These characteristics are consistent with those of the modern dust (Fig. 4d) and of loess deposits in the CLP (Yang and Ding, Reference Yang and Ding2004; Fig. 4d), indicating a typical aeolian origin of the Shandong loess. The last glacial loess unit L1 in the three sections has a median grain-size range of 12–35 μm (Fig. 3), which is coarser than that of the L1 loess unit in the southern part of the CLP (15–20 μm) but is similar to the contemporaneous loess unit in the central CLP (20–30 μm) (Yang and Ding, Reference Yang and Ding2004). For the interglacial paleosols S0 and S1, the median grain size has the range of 6–35 μm (Fig. 3), which is also coarser than the contemporaneous paleosol unit in the southern part of the CLP (8–9 μm) (Yang and Ding, Reference Yang and Ding2004). In addition, for the three loess sections in Shandong Province, there is no striking difference between the grain size of glacial loess and that of interglacial paleosol units, which contrasts with the pattern of “coarse-grained loess and fine-grained paleosol” commonly observed in the CLP (e.g., Yang and Ding, Reference Yang and Ding2004, Reference Yang and Ding2014).
Using the aforementioned “grain size–distance” model (Yang and Ding, Reference Yang and Ding2004, Reference Yang and Ding2017), we obtained a source-sink distance of ~200–300 km for the loess deposits in the PCSM and a distance of ~100–200 km for the loess deposits in the coastal area of Bohai Gulf. The estimated source-sink distances for the Shandong loess are much shorter than the distance to the arid region in northwestern China (>900 km) (Fig. 1); this indicates the importance of proximal, local dust sources for the Shandong loess, irrespective of glacial–interglacial cyclicity. Modern meteorological observations (Fu et al., Reference Fu, Xu, Cheng and Xu1994; Gao et al., Reference Gao, Wang, Zhang, Song, Gao and Zang2008) and historical documents (Cai, Reference Cai1989) have shown that dust activity in Shandong Province occurs mainly in spring when northeasterly winds prevail (Fig. 5), supporting a northeasterly provenance for the Shandong loess. Therefore, we conclude that the extensive fluvial deposits in the North China Plain and the continental shelf of Bohai Gulf, transported by the Yellow River and its tributaries (Wu et al., Reference Wu, Xu, Zhang and Ma1996, Reference Wu, Xu and Yang2000; Xu et al., Reference Xu, Wu, Zhu and Yang1996), are the major sources of the Shandong loess. Previous studies have shown that elemental ratios (TiO2/Al2O3, K2O/Al2O3, and Na/K) (Peng et al., Reference Peng, Hao, Wang, Ding, Zhang, Wang and Guo2016; Xu et al., Reference Xu, Ni and Ding2016, Reference Xu, Kong, Jia, Miao and Ding2018), Nd–Sr isotope composition, and uranium isotope ratios (234U/238U) of the Shandong loess are strikingly different from those of the CLP loess, but comparatively close to those of sediments in lower reaches of the Yellow River (Li et al., Reference Li, Li, Xu, Li and Chen2017; Zheng, Reference Zheng2018). This evidence strongly supports our finding.

Figure 5. (color online) Surface circulation over Shandong Province. February–March mean surface winds (a) and July–August mean surface winds (b) based on the NOAA-CIRES-DOE Twentieth Century Reanalysis V3 data for January 1836 to December 2015 (https://psl.noaa.gov/data/gridded/data.20thC_ReanV3.html).
Comparison of the sections presented here with those in previous studies shows that the median grain size of both the glacial loess and interglacial paleosol units in the coastal area is coarser than that of the corresponding units in the PCSM (Fig. 6). This indicates a substantial dust contribution from the adjacent continental shelf to the coastal loess, especially during the last glacial period when sea level fell substantially and desert steppe dominated the coastal areas (Fig. 6m–o; Liu and Xia, Reference Liu and Xia1992; Liu et al., Reference Liu, Zhang and Shen2004; Yi et al., Reference Yi, Yu, Ortiz, Xu, Qiang, Huang, Shi and Deng2012; Li et al., Reference Li, Li, Liu, Qiao, Ma, Xu and Yang2014; Li et al., Reference Li, Li, Yang, Chen and Chen2018), as supported by the presence of marine microfossils in the coastal loess (Cao et al., Reference Cao, Li and Shi1987, Reference Cao, Liu, Shi, Chang, Song, Yuan and Guo1993; Cheng et al., Reference Cheng, Li, Lv and Ju1995, Reference Cheng, Fu and Ju1996; Zhao, Reference Zhao1996). Furthermore, the prominent stratigraphic grain-size variability of the loess deposits of the PCSM indicates frequent changes in the location of dust sources due to frequent diversions of the Yellow River, which are documented in various historical records (e.g., Wu et al., Reference Wu, Xu, Zhang and Ma1996, Reference Wu, Xu and Yang2000; Xu et al., Reference Xu, Wu, Zhu and Yang1996; Wang, Reference Wang2005; Chen et al., Reference Chen, Syvitski, Gao, Overeem and Kettner2012).

Figure 6. Comparison of the stratigraphy, median grain size (Md), and magnetic susceptibility (χ) records of loess sections in the piedmont of the Central Shandong Mountains (Heiyu [a, b], Fujiazhuang [c, d], and Xiliangmeng [e, f] [Kong, Reference Kong2018]), the coastal area (Xinhaoshan [g, h] and Lingezhuang [i. j] [Tian et al., Reference Tian, Sun, Lü, Cao, Zhang and Lü2019]), and the central Chinese Loess Plateau (Qingyang [k, l] [Yang and Ding, Reference Yang and Ding2008]). Pollen record (herb percentage; Li et al., Reference Li, Li, Yang, Chen and Chen2018) and sea-level changes (Yi et al., Reference Yi, Yu, Ortiz, Xu, Qiang, Huang, Shi and Deng2012) in Bohai Gulf are also shown (m–o).
In addition, the trimodal grain-size distribution of a few paleosol samples from the Shandong loess (Fig. 4a–c) is similar to that of shallow lacustrine deposits in northeastern China (Lu et al., Reference Lu, Fang, Friedrich and Song2018; Fig. 4d), indicating the influence of enhanced hydrological conditions during interglacial periods. This is evidenced by the presence of gravels in a few loess sequences in Shandong Province (Zhang et al., Reference Zhang, Xin and Nie2004; Kong et al., Reference Kong, Xu and Jia2019). Pollen records from alternating marine and fluvial sediments of the Bohai Gulf indicate the presence of broad-leaved forest-steppe vegetation in the North China Plain during the last interglacial period and Holocene, which coincided with a high sea level in Bohai Gulf and paleosol development in the Shandong loess areas (Fig. 6m–o; Liu et al., Reference Liu, Zhang and Shen2004; Yi et al., Reference Yi, Yu, Ortiz, Xu, Qiang, Huang, Shi and Deng2012; Li et al., Reference Li, Li, Yang, Chen and Chen2018).
Paleoclimatic implications of the results
In the CLP, the grain size and MS of loess-paleosol sequences are reliable signals of the alternation of glacial loess and interglacial paleosol units (Heller and Liu, Reference Heller and Liu1984; An et al., Reference An, Kukla, Porter and Xiao1991; Ding et al., Reference Ding, Yu, Rutter and Liu1994, Reference Ding, Derbyshire, Yang, Yu, Xiong and Liu2002; Yang and Ding, Reference Yang and Ding2004, Reference Yang and Ding2008, Reference Yang and Ding2010); that is, the glacial loess units are characterized by a coarse grain size and low MS while the interglacial paleosol units are characterized by a fine grain size and high MS. However, this pattern is not consistently observed in the Shandong loess. In contrast to the CLP, several parts of the Holocene soil unit (S0) have almost the lowest MS values in both the Fujiazhuang and Xiliangmeng sections (Fig. 6). In addition, paleosol units S1 in the Fujiazhuang section and S0 in the Xinhaoshan section are coarser grained than the loess units above and below (Fig. 6). Therefore, the changes in grain size and MS in the Shandong loess do not effectively reflect loess-paleosol alternations.
The Shandong loess region lies to the east of the CLP and has a subhumid climate (Fig. 1b). Today, the climate is warmer and wetter in the Shandong loess region than in the CLP. The high organic matter content of the Holocene soil (S0) at Fujiazhuang and Xiliangmeng, as indicated by its dark color, reflects a large vegetation biomass under a wet climate (Fig. 1c and d). Therefore, a gleying process due to a reducing soil environment may be responsible for the low MS values observed in paleosol S0 unit. As mentioned in the preceding, the alternating loess and paleosol units during the last glacial–interglacial cycle in the Shandong loess region can be readily correlated with those in the CLP (Fig. 6; Yang and Ding, Reference Yang and Ding2008; Kong, Reference Kong2018; Tian et al., Reference Tian, Sun, Lü, Cao, Zhang and Lü2019). In this context, although the proxies for loess-paleosol stratigraphy developed in the CLP may not be applicable to the Shandong loess, the loess-paleosol alternations in both regions are a common product of global glacial–interglacial cycles.
CONCLUSIONS
OSL dating of the three loess sections in Shandong Province reveals that the Fujiazhuang loess-paleosol sequence accumulated mainly since the last interglacial period and that the loess-paleosol sequences at Xiliangmeng and Xinhaoshan were deposited since the last glacial period.
The grain size of the glacial loess and interglacial paleosol units in Shandong Province is much coarser than that of the contemporaneous units in the southern part of the CLP but similar to those in the central CLP, indicating a proximal source for the Shandong loess. Using the grain size–transport distance model established for the CLP, the estimated source-sink distance is ~200–300 km for the loess deposits in the PCSM area and ~100–200 km for those in the coastal area. This suggests that the fluvial deposits of the Yellow River system in the North China Plain, as well as sediments on the adjacent continental shelf, are the major provenance for the Shandong loess. In the three studied sections, the grain-size distributions of most of the samples are bimodal and positively skewed; however, a trimodal distribution occurs in a few paleosol samples, indicating the effects of reworking by intensified hydrological processes on the Shandong loess during interglacial periods.
In contrast to the pattern of “coarse grain size and low MS of loess units” versus “fine grain size and high MS of paleosols units” observed in the loess-paleosol sequences of the CLP, several paleosol units in the Shandong loess are very coarse grained and have low MS values, probably due to frequent changes in dust sources caused by diversions of the Yellow River and local hydroclimatic conditions. Although the proxies for loess-paleosol stratigraphy developed in the CLP may not be applicable to the Shandong loess, the loess-paleosol alternations in the Shandong loess, like those of the CLP, are still a product of global glacial–interglacial cycles.
ACKNOWLEDGMENTS
This study was supported by the National Natural Science Foundation of China (grant 41725010), the Strategic Priority Research Program of Chinese Academy of Sciences (grants XDB31000000 and XDB26000000), and the Key Research Program of the Institute of Geology & Geophysics, CAS (grant IGGCAS-201905). We thank Yangyang Li and Xiaoxiao Yang for assistance in the field and laboratory. We are grateful to Nathaniel Rutter, Huayu Lu, and Nicholas Lancaster for their helpful comments and suggestions and Guest Editor Slobodan Marković for handling the manuscript.