INTRODUCTION
Reconstruction of paleoenvironments at the Pleistocene-Holocene transition (PHT) in North America has been the focus of much research over the last 100 years, and the basin of pluvial Lake Bonneville has served as a critical laboratory in the Desert West (e.g., Gilbert, Reference Gilbert1890; Eardley, Reference Eardley1938; Currey, Reference Currey1990; Morrison, Reference Morrison and Morrison1991; Oviatt et al., Reference Oviatt, Sack, Currey, Gierlowski and Kelts1994, Reference Oviatt, Thompson, Kaufman, Bright and Forester1999, Reference Oviatt, Miller, McGeehin, Zachary and Mahan2005; Madsen et al., Reference Madsen, Oviatt, Young, Page, Madsen, Schmitt and Page2015a; Oviatt and Shroder, Reference Oviatt and Shroder2016). Changes in the terrestrial and stratigraphic records deposited during the late Pleistocene regressive phase of pluvial Lake Bonneville provide information on regional climatic drivers. Locally, these changes influenced the wetland habitats that were key to early occupation of the Great Basin by humans, ca. 13,000–9500 cal yr BP (Willig et al., Reference Willig, Aikens and Fagan1988; Beck and Jones, Reference Beck and Jones1997, Reference Beck, Jones, Madsen, Schmitt and Page2015; Graf and Schmitt, Reference Graf and Schmitt2007; Grayson, Reference Grayson and Grayson2011; Madsen et al., Reference Madsen, Schmitt and Page2015b; Smith et al., Reference Smith, Duke, Jenkins, Goebel, Davis, O'Grady, Stueber, Pratt and Smith2020).
Around the time of the Bølling-Allerød warming, ca. 15,000 cal yr BP, Lake Bonneville receded from its sill-controlled stillstand marked by the Provo shoreline, having stabilized for some time at that level following the catastrophic sill failure at Red Rock Pass. After 15,000 cal yr BP, evaporation outpaced input from runoff and precipitation and the closed-basin lake regressed rapidly. Lake Bonneville's levels dropped precipitously and reached low-stands similar to the average elevation of the modern Great Salt Lake by 13,000 cal yr BP (Godsey et al., Reference Godsey, Oviatt, Miller and Chan2011; Oviatt, Reference Oviatt2015). While the climate-driven pluvial lake retreated, however, an overflow river—the Old River Bed river—emanating from remaining surface connection to the Sevier drainage, flowed onto the basin floor as an inland delta (Oviatt et al., Reference Oviatt, Madsen and Schmitt2003; Madsen et al., Reference Madsen, Oviatt, Young, Page, Madsen, Schmitt and Page2015a; Bradbury et al., Reference Bradbury, Jewell, Fernandez, Lerback, DeGraffenried and Petersen2020) in what is now the southern extent of the Great Salt Lake Desert (GSLD) (Figure 1). This delta, and its associated water bodies and distributary network, is referred to herein as the Old River Bed delta (ORBD). As shown in Figure 1, many of its primary arterial channels have been mapped by aerial imagery and ground truthing during the course of archaeological research (Duke, Reference Duke2011; Madsen et al., Reference Madsen, Oviatt, Young, Page, Madsen, Schmitt and Page2015a), but there is evidence for a vast network of eroded channels covering ~2,000 km2 extending north of Knolls Dunes almost to Interstate 80 and into the western part of the basin toward Wendover, Utah (Madsen et al., Reference Madsen, Oviatt, Young, Page, Madsen, Schmitt and Page2015a; Clark et al., Reference Clark, Oviatt and Page2016, Reference Clark, Oviatt, Hardwick and Page2020). The characterization as an inland delta is best demonstrated for the early Holocene, to which most of the channels have been dated and have been the focus of archaeological work (Duke, Reference Duke2011; Madsen et al., Reference Madsen, Oviatt, Young, Page, Madsen, Schmitt and Page2015a), but, as described in this study, the ORBD may have emptied into a large water body in the southern GSLD during the terminal Pleistocene. The ORBD supported an extensive wetland complex and, for ca. 3500 years, sustained a primary wetland habitat and provided resources for human inhabitants (Arkush and Pitblado, Reference Arkush and Pitblado2000; Duke and Young, Reference Duke, Young, Graf and Schmitt2007; Schmitt et al., Reference Schmitt, Madsen, Oviatt, Quist, Graf and Schmitt2007; Duke, Reference Duke2011, Reference Duke2015; Madsen et al., Reference Madsen, Schmitt and Page2015b; Madsen, Reference Madsen, Oviatt and Shroder2016; Smith et al., Reference Smith, Duke, Jenkins, Goebel, Davis, O'Grady, Stueber, Pratt and Smith2020).
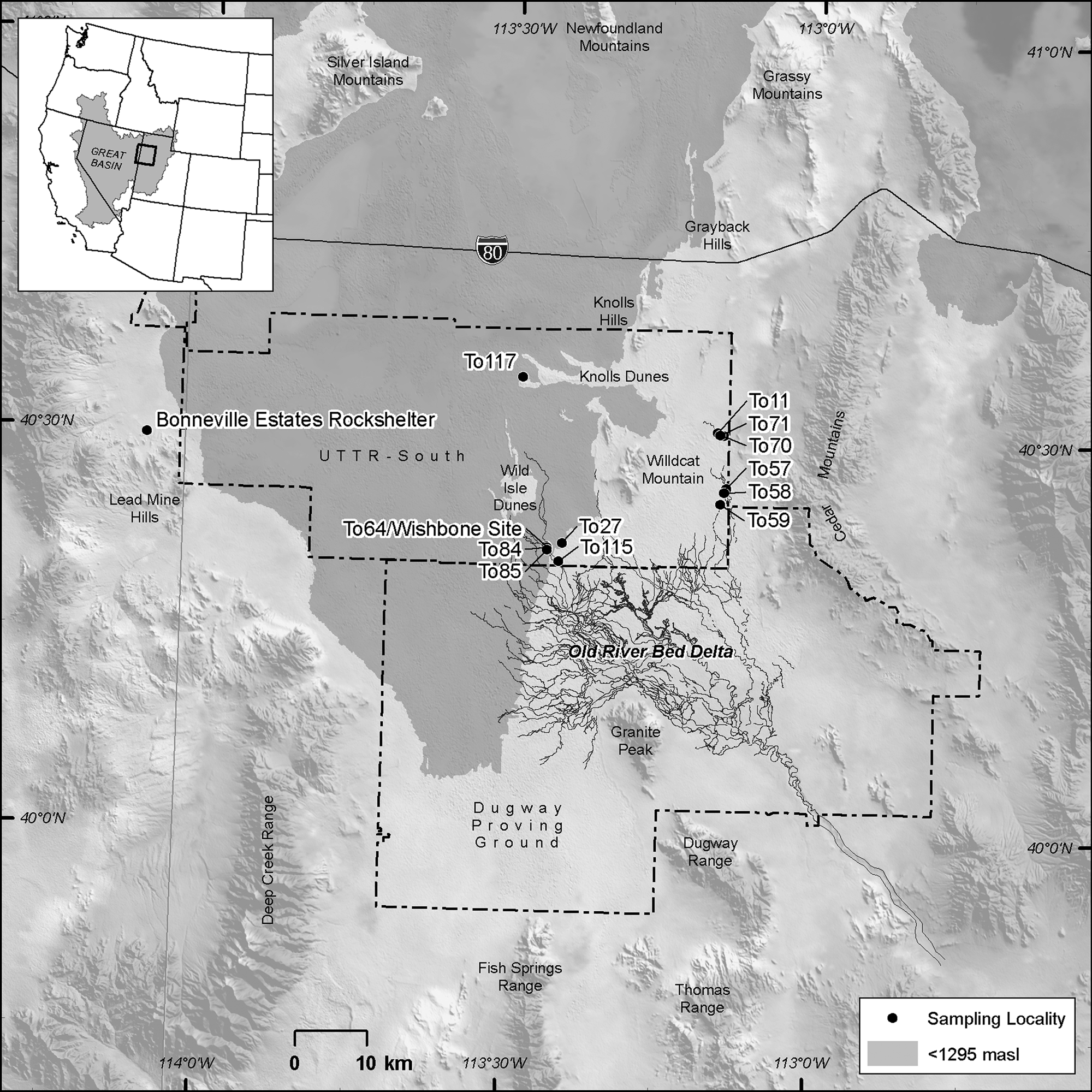
Figure 1. General location of the study area and sampling locations. Areas below the modern 1295-meter elevation contour within the Bonneville basin are shaded in gray. Black Channels of Madsen et al. (Reference Madsen, Oviatt, Young, Page, Madsen, Schmitt and Page2015a) are shown in the map.
Question and purpose
While there has been significant study of Pleistocene transgressive and regressive cycles of pluvial Lake Bonneville (Oviatt and Shroder, Reference Oviatt and Shroder2016), our understanding of the dynamic response of ORBD landforms—distributary avulsions, inter-channel ponding, floodplain and levee stability, and occasional transgressive inundation—is evolving. The overlapping and occasionally cross-cutting fluvial, paludal, and lacustrine facies present in the ORBD highlight a dynamic delta. What was the character of local environments preserved in the stratigraphic record of the ORBD, and how did they change through time? In this study, we focus on the paleoenvironmental record of mollusks, ostracodes, and the Sr-isotope signatures of groundwater from PHT sediments to clarify early post-Bonneville history in the northern part of the ORBD and relate it to human occupations that were among the earliest in the Great Basin.
ORBD non-marine mollusks and ostracodes are sensitive to environmental factors such as water temperature, salinity, and pH. In general, species and communities colonize, thrive, and retreat (or die) due to changes in water chemistry (Forester, Reference Forester1991). Additional environmental variables, such as currents (stream flow), turbidity, and bedload, influence population composition and maturity patterns (Rutherford, Reference Rutherford2000; Dillon and Stewart, Reference Dillon and Stewart2003). For example, pea clams (Pisidium) develop robust communities in well-oxygenated, lotic (flowing) waters, but do not colonize poorly oxygenated, lentic (slow-flowing or stagnant) waters where Physella flourishes. Similarly, terrestrial gastropods, such as Oxyloma, prefer riparian settings near water sources (Pilsbry, Reference Pilsbry1948). Based on our knowledge of modern communities, and inferences of past communities elsewhere, it is possible to infer past environmental conditions at localities where these organisms are found.
Here, we present results of collection and analyses of mollusks and ostracodes from 12 localities on the ORBD (Fig. 1). We open with a brief presentation of background on the regressive phase of pluvial Lake Bonneville, the formation of the ORBD, and human use. This is followed by the geography of the ORBD localities. Following a methods section, we provide detailed results on the biological composition of locality samples, supported by species identification and taphonomic patterns, and the 87Sr/86Sr ratio analysis of mollusk shells (Stagnicola caperata, Valvata humeralis, Helisoma (Planorbella) trivolvis, and Gyraulus parvus) at a few key localities. The results provide a generalized stratigraphy reflecting delta dynamics across the PHT. The stratigraphy includes a discussion of age control based on a suite of radiocarbon dates from natural organic-rich outcrops and excavated profiles. These serve as the basis for presentation and interpretation of six temporal biofacies evident in the stratigraphic and spatial record of the ORBD. We close with general discussion, conclusions, and recommendations for future study.
BACKGROUND
Gilbert (Reference Gilbert1890) was the first to recognize the ancient shorelines of what he would call Lake Bonneville and studied its dramatic transgressions and regressions based on field observations of its shorelines and sediments. Examination of early Holocene records in the Bonneville basin by Oviatt et al. (Reference Oviatt, Madsen and Schmitt2003, Reference Oviatt, Madsen, Miller, Thompson and McGeehin2015) identified evidence of freshwater on the basin floor at that time, most likely derived from groundwater discharge rather than from lake transgression. Oviatt (Reference Oviatt2014) described the Gilbert episode and reinterpreted the highest rise of Great Salt Lake (post-Lake Bonneville) at about the end of the Younger Dryas interval, ca. 11,500 cal yr BP.
Other efforts confirmed and refined these works (e.g., Currey Reference Currey1990; Oviatt Reference Oviatt, Hershler, Madsen and Currey2002, Reference Oviatt2015; Benson et al., Reference Benson, Lund, Smoot, Rhode, Spencer, Verosub, Louderback, Johnson, Rye and Negrini2011; Godsey et al., Reference Godsey, Oviatt, Miller and Chan2011). In the Burmester core, Oviatt et al. (Reference Oviatt, Thompson, Kaufman, Bright and Forester1999) found evidence of only four deep-lake cycles in Brunhes time (since 780 ka), the last of which is the Bonneville Lake cycle, which began ca. 30,000 cal yr BP. As Lake Bonneville retreated near the end of the Pleistocene, a lake in the main body (the Great Salt Lake basin) separated from the lake in the Sevier basin to the south (Oviatt et al., Reference Oviatt, Madsen and Schmitt2003). The Sevier basin lake is named Lake Gunnison (Oviatt, Reference Oviatt1988). Lake Gunnison remained at its threshold as Lake Bonneville regressed, producing the river that flowed along the Old River Bed that debouched ~50 km to the north onto the basin floor in what is now referred to as the Great Salt Lake Desert (Madsen et al., Reference Madsen, Oviatt, Young, Page, Madsen, Schmitt and Page2015a). Overflow ceased at ca. 11,500 cal yr BP (Oviatt et al., Reference Oviatt, Madsen and Schmitt2003; Madsen et al., Reference Madsen, Oviatt, Young, Page, Madsen, Schmitt and Page2015a), but groundwater from the Sevier basin sustained the ORBD until ca. 9500 cal yr BP. Discharge from the Sevier basin continues today, although at less volume and to a lower water table. Sr-isotope signatures obtained in this and previous studies are consistent with this interpretation.
Human use
The first people to leave clear physical evidence of their presence entered the eastern Great Basin at approximately the beginning of the Younger Dryas chronozone (YDC). This evidence comes in the form of Western Stemmed Tradition (Smith et al., Reference Smith, Duke, Jenkins, Goebel, Davis, O'Grady, Stueber, Pratt and Smith2020) projectile points, stone tools, and radiocarbon-dated remains, usually hearths, found in the dry caves and rockshelters overlooking the Bonneville basin floor. Bonneville Estates Rockshelter, located in the Lead Mine Hills near Blue Lake ~60 km northwest of the area described in this paper (Fig. 1), contains an extensive record of Western Stemmed points and a series of hearths demonstrating human occupation beginning at least by ca. 12,900–12,700 cal yr BP (Goebel, Reference Goebel, Graf and Schmitt2007; Graf, Reference Graf, Graf and Schmitt2007; Goebel and Keene, Reference Goebel, Keene, Parezo and Janetski2014; Goebel et al., Reference Goebel, Hockett, Rhode and Graf2021). Smith Creek Cave, located in the Snake Range overlooking the Snake Valley arm of the basin (~150 km southwest of the area described in this paper), contains similar evidence and timing (Bryan, Reference Bryan, Touhy and Randall1979; Goebel and Keene, Reference Goebel, Keene, Parezo and Janetski2014). At both sites, a large, lanceolate projectile point type (known as Haskett) represents the first diagnostic Western Stemmed evidence (Duke, Reference Duke2015). Goebel and Keene (Reference Goebel, Keene, Parezo and Janetski2014) associate a hearth dating to 10,540 ± 40 14C BP (ca. 12,660–12,490 cal yr BP) with Haskett at Bonneville Estates Rockshelter. At Smith Creek Cave, several stem fragments are consistent with Haskett technology (Bryan, Reference Bryan, Touhy and Randall1979) and are strongly associated with radiocarbon ages on four hearths and a piece of cordage considered by Goebel and Keene (Reference Goebel, Keene, Parezo and Janetski2014) to represent the strongest evidence for the site's early occupation, ca. 12,600–12,100 cal yr BP. The location of these sites suggests that the extensive wetland resource base of the Bonneville basin—inclusive of the ORBD, but also including those fed by Deep Creek, Fish Springs, and other minor discharge distributaries—served as a key draw for the earliest substantial occupations by people in the region.
Sites such as these are not often preserved on the basin floor, but the horizontally distributed record of stone tools associated with the shifting hydrology of the ORBD through time provides some measure of human occupation (Duke, Reference Duke2011; Madsen et al., Reference Madsen, Schmitt and Page2015b). Hundreds of sites throughout the ORBD and alongside paleochannel landforms dating between ca. 12,300–9500 cal yr BP represent this association (Madsen et al., Reference Madsen, Oviatt, Young, Page, Madsen, Schmitt and Page2015a). One known exception, the Wishbone site (42TO6384; herein referred as To64), provides the first buried and stratigraphically intact evidence of people on the ORBD at ca. 12,300 cal yr BP (Duke et al., Reference Duke, Rice, Young and Byerly2018a; Smith et al., Reference Smith, Duke, Jenkins, Goebel, Davis, O'Grady, Stueber, Pratt and Smith2020), as will be discussed in the current study. As in the early strata at Bonneville Estates Rockshelter and Smith Creek Cave, this site is directly associated with Haskett projectile points.
Projectile points are the most distinctive artifacts for tracking human use. They exhibit stylistically diagnostic modifications through time and are widespread at the ground surface of the ORBD (Duke, Reference Duke2011; Beck and Jones, Reference Beck, Jones, Madsen, Schmitt and Page2015). The points fall into two broad categories: the above-mentioned Western Stemmed Tradition and the split-/indented-base Pinto type. These occur in a relative early-to-late sequence, respectively, that has been observed elsewhere in the Great Basin, especially the Mojave Desert (see discussions by Haynes, Reference Haynes, Allen and Reed2004; Sutton et al., Reference Sutton, Basgall, Gardner, Allen, Jones and Klar2007). Together, they are associated with a cohesive lithic technological tradition strongly associated with regional wetland use (Duke, Reference Duke2011; Grayson, Reference Grayson and Grayson2011; Madsen et al., Reference Madsen, Schmitt and Page2015b; Smith et al., Reference Smith, Duke, Jenkins, Goebel, Davis, O'Grady, Stueber, Pratt and Smith2020). Western Stemmed points represent a group of related styles with variably shaped stems. While their specific chronology is unclear, they are broadly time sensitive on the ORBD, with those styles that are larger being older (e.g., Haskett, Cougar Mountain), and those that are smaller being younger (e.g., Silver Lake, Bonneville, Stubby) (Duke, Reference Duke2011; Rosencrance, Reference Rosencrance2019; Smith et al., Reference Smith, Duke, Jenkins, Goebel, Davis, O'Grady, Stueber, Pratt and Smith2020). The larger styles, Haskett at least, are also largely associated with late Pleistocene, and the YDC specifically. Pinto represents a significant stylistic departure in the early Holocene at ca. 10,000 cal yr BP (Haynes, Reference Haynes, Allen and Reed2004; Janetski et al., Reference Janetski, Bodily, Newbold and Yoder2012). This style exhibits an indented to split stem base, but no substantial change in point size from the smaller size that immediately precedes Western Stemmed styles. Of primary importance to the current study, the record of these point styles shows that people's intensity of use of the ORBD wetlands across the Pleistocene-Holocene transition increased as their long-range mobility decreased (Duke, Reference Duke2011), likely by some combination of changing climatic conditions (Madsen et al., Reference Madsen, Oviatt, Young, Page, Madsen, Schmitt and Page2015a), the extinction of certain megafauna (Duke, Reference Duke2015), and the disappearance of alternative basin wetland habitats to the ORBD (Duke, Reference Duke2011; Duke and King, Reference Duke and King2014).
Study area and sampling localities
The ORBD is in the southern GSLD in western Utah (Fig. 1). This study is an outgrowth of archaeological work conducted for the U.S. military, which emphasized an extensive record of early human presence in the desert west (Arkush and Pitblado, Reference Arkush and Pitblado2000; Duke and Young, Reference Duke, Young, Graf and Schmitt2007; Schmitt et al., Reference Schmitt, Madsen, Oviatt, Quist, Graf and Schmitt2007; Duke, Reference Duke2011, Reference Duke2015; Madsen et al., Reference Madsen, Schmitt and Page2015b). The portion of interest here lies within the southern part of the Air Force's Utah Test and Training Range, where our research stems from cultural resources compliance inventories and limited archaeological excavations (Byerly et al., Reference Byerly, Duke, Young and Rice2018; Duke et al., Reference Duke, Rice, Young and Byerly2018a, Reference Duke, Young and Rice2018b). The geographic boundaries of the basin include the Cedar Mountains to the east, the Deep Creek Range to the southwest, and Granite Peak to the south, but the ORBD extends north into the central basin near the isolated geologic features of Wildcat Mountain and the Knolls hills (Clark et al., Reference Clark, Oviatt, Hardwick and Page2020).
The distal reaches of the delta of the ORBD include inverted gravel channels (Black channels of Madsen et al., Reference Madsen, Oviatt, Young, Page, Madsen, Schmitt and Page2015a) and a subtle, low-relief topography of weakly inverted sand channels, distributary meanders, meander and oxbow ponds and embayments, deflated mud flats, and occasional capping dunes. Delta features extend as far north as Interstate 80, extending over 60 kilometers from proximal segments preserved along the foot of Granite Peak.
To investigate spatial and temporal variability across the delta (and subject to limitations of access restriction), we focused on four areas of the delta (Fig. 1). The medial area between the inverted gravel channels and the Wild Isle Dunes (Localities To27, To64, To84, To85) is where archaeological test excavations revealed a thin (<1.5 m), but informative, stratigraphic section of the ORBD. Dateable, organic-rich black mats provide temporal markers interspersed in the delta facies with microfauna assemblages. The east delta localities comprise two clusters in distal delta channels and flats below the Cedar Mountains (Localities To57, To58, To59 on the generally open playa; Localities To11, To70, and To71 in the sandsheets and dunes further north). At these localities, black mats are exposed at the modern surface (or within a centimeter or two of the surface) where thin channel sands and silty muds preserve the organic wetland remnants. A single locality (To117) along the windward margin of the Knolls hills highlights the rare remnants of distal delta channels near the northernmost reaches of the distributary system. These distal delta channels are shown in Clark et al.'s (Reference Clark, Oviatt and Page2016, Reference Clark, Oviatt, Hardwick and Page2020) DPG and BSF maps, respectively, and in Figure 1.
Materials and methods
The stratigraphy of the ORBD deposits is revealed where erosion from hydroaeolian planation (Currey, Reference Currey1990, p. 193–194) exposed delta landform remnants in low-relief adjacent to channel forms and deflation basins. We also exposed detailed stratigraphic profiles in conjunction with geoarchaeological studies; these included archaeological units, geomorphological shovel probes, limited backhoe trenching, radiocarbon dating, and a preliminary core extraction. Such investigations have proven useful for defining broad temporal associations with the shifting hydrology of the distributary network for the otherwise undatable archaeological sites on the ground surface (Duke, Reference Duke2011; Madsen et al., Reference Madsen, Schmitt and Page2015b).
The presence of organic-rich black mat sediments has been crucial to reconstructing ORBD distributary chronology. Black mats are sedimentary strata altered by the accretion, inclusion, or penetration of organic material that draw our attention to profile sections and, in present-day arid environments such as the ORBD, highlight the likelihood that a profile may preserve a trove of information about environmental conditions unmatched in the modern setting (Quade et al., Reference Quade, Forester, Pratt and Carter1998, p. 129; Harris-Parks, Reference Harris-Parks2016, p. 94). Although attempts have been made to characterize the black mats in time, space, and constituents (Harris-Parks, Reference Harris-Parks2016), the label of “black mat” is applied to various dark, organic-rich strata or soil horizons that punctuate otherwise light-colored profiles (Haynes, Reference Haynes2008, p. 6520). Pigati et al. (Reference Pigati, Springer and Honke2019, p. 52) refer to similar strata or horizons simply as “paleowetland deposits.” Black mats have been especially informative in arid and semi-arid settings of the American Great Basin and Southwest where they mark the reactivation or formation of mesic habitats within the generally drying landscape of the Pleistocene-Holocene Transition (Quade et al., Reference Quade, Forester, Pratt and Carter1998; Haynes, Reference Haynes2008; Pigati et al., Reference Pigati, Springer and Honke2019). In the ORBD, black mats tend to mark sedimentary strata—often in multiple intercalated beds—that contain organic residues of former wetland habitats (decayed and carbonized plant and faunal remains), algal accretion in shallow water, and/or concentration of micronized charcoal reduced from wildfire flotsam and windrows along former shore zones. Due to the organic enrichment of these strata, although organic contributions may come from a variety of sources, they provide locally unique opportunities to define the spatial and temporal positions of the environments where they once formed.
To obtain the samples used in this investigation, we focused on collecting materials from geoarchaeological localities across the distal region of the ORBD, practically defined as the portion north of the UTTR-Dugway Proving Ground (DPG) military boundary (also see Madsen et al., Reference Madsen, Oviatt, Young, Page, Madsen, Schmitt and Page2015a) (Fig. 1). Geoarchaeological locality numbers were assigned to our sampling locations to make this distinction. We collected sediment samples for microfossils with cleaned trowels from sediment within stratigraphic layers. Target sample volume was ~0.25–0.5 liters. At the laboratory, we analyzed the samples for mollusks and ostracodes. Mollusks were floated to separate the macro- and microfossils. Sample-size was ~200 g, except for samples RBM-11 and RBM-14 that were 500 g each. We processed ~100 g of sediments for the micropaleontological (ostracodes and micromollusks) analysis. The sediments were prepared using a set of three sieves (18 [1 mm], 140 [106 μm], and 230 [63 μm] US Standard) to retain the smallest valves and shells (Palacios-Fest, Reference Palacios-Fest2010). All samples were examined to identify fossil contents and biological assemblages. The biological composition included mollusks, ostracodes, and, rarely, gyrogonites (Supplemental Tables 1, 2). All species were identified using reference collections, as well as the relevant literature on taxonomy by group (Wood, Reference Wood1967; Delorme, Reference Delorme1970, Reference Delorme1971; Clarke, Reference Clarke1981; Dillon, Reference Dillon2000; Dillon and Stewart, Reference Dillon and Stewart2003).
Mollusk shells were analyzed with a magnifying lens and measured with a digital caliper to determine the height, width, and aperture when appropriate. The samples were prepared using routine procedures described elsewhere (Palacios-Fest, Reference Palacios-Fest2010). A low-magnification microscope was used to identify the biological composition, diversity, abundance, maturity, and taphonomic features for micromollusks and ostracodes.
When possible, specimens were identified to the species level. Diversity, total and relative abundance, population density, and maturity (a ratio of the adult/juvenile shells) were recorded for reconstructing the paleoenvironmental history of the site. Other taphonomic parameters, such as fragmentation, abrasion, and encrustation, indicate the living and burial conditions of the shells. For example, a high degree of fragmentation and/or abrasion is interpreted as an indicator of transport (Adams et al., Reference Adams, Smith and Palacios-Fest2002), whereas increasing mineral overgrowth on the shells indicates excess salt concentration in the soils favoring the adherence of “exotic” minerals to the shells after burial (Adams et al., Reference Adams, Smith and Palacios-Fest2002). The maturity ratio allows us to differentiate a biocenosis (life assemblage) from a thanatocoenosis (death assemblage). That is, faunal assemblages consisting of a suite of adults and juvenile shells more frequently represent a local, living population (Whatley, Reference Whatley1983). By contrast, if juveniles or adults dominate the assemblage, it is possible to infer a thanatocoenosis that, in turn, may indicate the population is not local but either transported (if naturally) or selectively gathered (if by human activity).
We used 18 shell samples of four gastropod species (Stagnicola caperata, Valvata humeralis, Helisoma (Planorbella) trivolvis, and Gyraulus parvus) for strontium isotope (87Sr/86Sr) analysis. Each individual Sr analysis consisted of multiple shells with a combined total weight, depending on available material, of ~10–100 mg. The exceptions were samples To64-1-7-750, To27-1-1-15, and To84-1-6, which consisted of shell material weighing <1 mg. Shells were crushed and sonicated for 30 minutes in ultra-pure Milli-Q water (Millipore, 18.2 MΩ cm) and rinsed with Milli-Q until the solution was clear. After drying, the non-shell organic material was removed by sonicating the samples in 10% H2O2 (Fisher Chemical catalog number: H325) for 30 minutes. After rinsing the samples multiple times with Milli-Q water, the shells were then leached for five minutes in 5% glacial acetic acid (Sigma Aldrich catalog number: 338826) to remove non-shell carbonates.
The resulting shell material was then dissolved in 8M HNO3 for separation of Sr. After dissolution, strontium was separated from samples using Sr resin (Sr-Spec Resin®, Eichrom, Lisle, Illinois, Part number SR-B100-A) and acid solutions made from twice-distilled acid. Separation procedures are modified from Horwitz et al. (Reference Horwitz, Chiarizia and Dietz1992). Strontium samples were loaded onto degassed Rhenium (Re) filaments using a TaF5 activator (Charlier et al., Reference Charlier, Ginibre, Morgan, Nowell, Pearson, Davidson and Ottley2006) to enhance ionization.
Strontium was analyzed using a VG Sector 54 multi-collector thermal ionization mass spectrometer in dynamic collection mode at the University of Arizona. The 87Sr/86Sr ratios were corrected for mass fractionation using 86Sr/88Sr = 0.1194. NIST SRM 987 standards analyzed with the samples yielded a value of 0.7102406 ± 0.00001 (n = 2), and sample ratios were normalized to 0.710245 (Faure and Mensing, Reference Faure and Mensing2005). Total process blanks were an insignificant proportion of the total strontium separated from each sample.
Radiocarbon dating by Accelerator Mass Spectrometry (AMS) of material from black mat sediments and archaeological hearth charcoal provides our sample chronology. Where possible, samples were obtained from plant parts, if visible in the field, or from manual flotation in the laboratory; otherwise, bulk sediment was used. Samples were submitted to Beta Analytic (Beta) for pretreatment and analysis. Black mat plant remains are carbonized and resemble charred material. They may indeed be the product of low-temperature combustion, albeit not burned in the common sense, and therefore remain susceptible to instability in the base treatment. Beta's acid/alkali/acid pretreatment, typical for charred material, yielded adequate samples in each case and results that were consistent with stratigraphic expectations and the many prior dates collected on the ORBD (Madsen et al., Reference Madsen, Oviatt, Young, Page, Madsen, Schmitt and Page2015a). Bulk sediment was subjected to acid washes. In most cases, the black mats represent relatively short periods, on the order of a few hundred years or less, of waterbody (usually channel) activity and are thus not subject to averaging of long periods of time. They also have low potential for humate contamination given the sparse to barren nature of Holocene vegetation in the GSLD. More work could be done to clarify precision of the ages derived from our samples (see Pigati et al., Reference Pigati, Springer and Honke2019), but the results are appropriate to the temporal scale of comparison in the current study. Calibrated ages were generated using the IntCal20 curve (Reimer et al., Reference Reimer, Austin, Bard, Bayliss, Blackwell, Bronk Ramsey and Butzin2020) calibration in the Calib 8.2 program (Stuiver et al., Reference Stuiver, Reimer and Reimer2021).
Most of our sampling localities coincide with archaeological sites, although the latter vary from being directly or approximately associated to completely unassociated with the radiocarbon dates (see Table 1). Only one site is directly represented by charcoal from a human-made hearth and another by a charcoal lens that appears to represent another hearth. The Wishbone site (42TO6384) is definitive, with a fully excavated central hearth containing willow wood charcoal and a surrounding assemblage of food refuse (mostly waterfowl) and stone tools (Duke et al., Reference Duke, Rice, Young and Byerly2018a). Site 42TO5136, the Hello site, is located ~200 m from Wishbone and is another Haskett-associated site found on the ground surface and eroding from a modern dissection in an ORBD landform (Duke et al., Reference Duke, Young and Rice2018b). Limited subsurface testing encountered a charcoal lens containing burned grass/sedge stems, willow wood, and waterfowl bone. The other sites have only indirect temporal associations (e.g., Pleistocene vs. Holocene age) with the radiocarbon or mollusk samples via proximity with dated ORBD landforms and strata. These estimations (Table 1) are based on assemblage information, especially time-diagnostic projectile points as discussed above, and horizontal spatial relationships with dated channel sets (Hirschi, Reference Hirschi2006; Duke, Reference Duke2011; Madsen et al., Reference Madsen, Oviatt, Young, Page, Madsen, Schmitt and Page2015a).
Table 1. Radiocarbon age estimates. *Calibrated ages rounded to decade. §Age Relationship represents an assemblage-based and distributary-associated age determination as Pleistocene (PL) or early Holocene (EH), followed by the site's direct or estimated relation to the calibrated median probability age of the dated sample (also see Materials and Methods).

RESULTS
Stratigraphic sequence, temporal controls, and lithofacies
We define six major stratigraphic units (Fig. 2A, B). The distal delta channel landforms evident in whole or part at the study localities are related in one way or another to each of these units. The presence or absence of some strata depends on location within the study area.
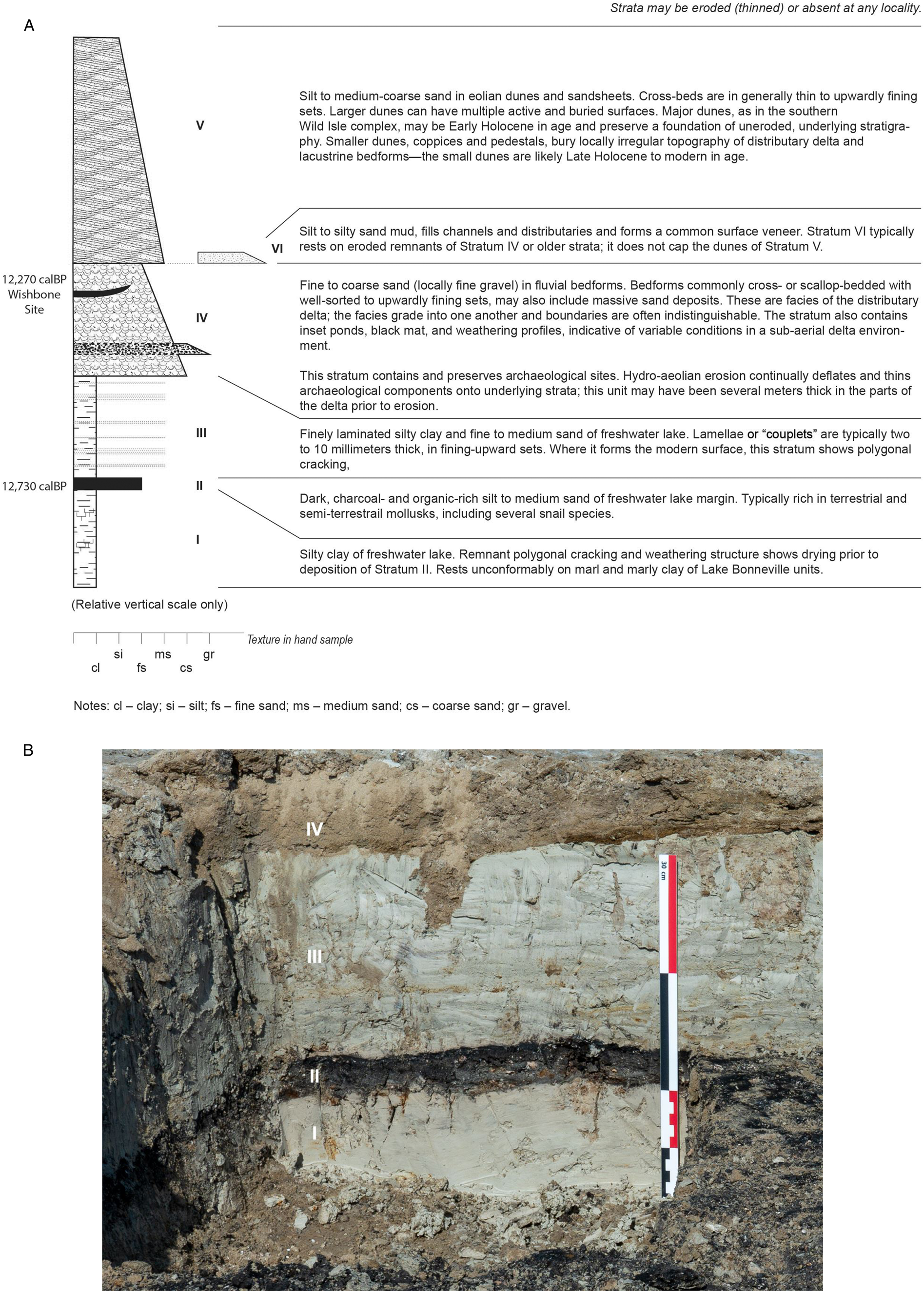
Figure 2. (A) Generalized stratigraphy; (B) Stratigraphic section at Locality To85-8, showing clear and abrupt contact between the sedimentary units. Stratum I is massive silty clay of freshwater lake; Stratum II is organic- and charcoal-rich silt to medium sand (black mat) of freshwater margin; Stratum III is finely laminated silty clay and fine to medium sand of freshwater lake; Stratum IV at modern surface is fine to coarse sand (locally fine gravel) of prograding delta; this deltaic stratum also contains inset ponds, black mat, and weathering profiles—facies common to the inland delta.
Stratum I, which consists of blocky to marl-like clay and clayey silt indicative of deep water, sits atop Lake Bonneville marl. We do not know the timing of the transition to the Stratum 1 clay, but we estimate the age of regression of Lake Bonneville to elevations similar to that of the Wishbone site by 13,000 cal yr B.P., or as much as several hundred years earlier; greater precision is not possible at this time (Godsey et al., Reference Godsey, Oviatt, Miller and Chan2011; Oviatt, Reference Oviatt2015). Polygonal cracking at the Stratum I/II interface suggests an undetermined period of desiccation within the study area prior to a resurgence of lacustrine conditions that are represented by Stratum II.
Stratum II is a distinct organic- and charcoal-rich black mat that contains abundant terrestrial and semi-terrestrial mollusks. This prominent stratum is well dated, with multiple assays of carbonized wood fragments and organic sediment, and is found across a large area of the study area. Seven samples of Stratum II sediment provide a median probability calibrated age of 12,730 cal yr BP and a two-sigma range of 12,760–12,680 cal yr BP. The composition of Stratum II is similar to the YDC black mat elsewhere in North America, such as at Murray Springs, Arizona (Haynes and Huckell, Reference Haynes and Huckell2007), Tule Springs (Haynes, Reference Haynes, Wormington and Ellis1967; Springer et al., Reference Springer, Manker and Pigati2015), and other sites around the world illustrated in Wolbach et al. (Reference Wolbach, Ballard, Mayewski, Adedeji, Bunch, Firestone and French2018). Understanding that carbonized wood is mostly material that decayed in situ, and that charcoal is the result of burning, the visual physical appearance suggests widespread sorting of microscopic charcoal in water. We have no data to suggest the source of wildfires, or whether they might be naturally or culturally generated (but see Cushing et al., Reference Cushing, Wenner, Noble and Daily1986; Rick et al., Reference Rick, Wah and Erlandson2012; Lightfoot et al., Reference Lightfoot, Cuthrell, Striplen and Hylkema2013). However, during microscope work and fossil picking, the senior author recognized certain cubic forms of very small charcoal-like fragments. By contrast, in his personal experience, carbonized wood has a sapropelic appearance (amorphous).
Laminated clays alternating with clay-sand couplets form Stratum III. The prominent lobate Black channels of the proximal delta, now topographically inverted due to Holocene erosion, probably formed in the debouchment of the Old River Bed river into the shallow lake where Stratum III was forming. Stratum III, as with Stratum II, is distinct to our central basin sampling locations.
Stratum IV consists of sandy mud that contains localized black mats, finely laminated sands, and massive sand deposits. The vast bulk of what is known and dated of the ORBD represents Stratum IV deposits in the early Holocene to ca. 9500 cal yr BP, with decline thereafter. Our sample localities throughout the study area represent evidence of the stratum's persistence.
Erosion during middle to late Holocene time has differentially scoured landforms of the remnant delta. Erosion processes include hydroaeolian planation (Currey, Reference Currey1990), which involves cyclic wetting and drying and deflation of fine-grained (silt- and clay-size) particles. Entrainment of fine-grained particles by raindrop impacts and sheetwash are likely to have been involved in the erosion. Coarser-grained particles (sand) are blown relatively short distances and end up in eolian dunes on the mudflat surfaces and at basin margins (Stratum IV). Strata V and VI represent dunes and the muddy veneer of the mudflats, respectively, and are the deposits of sediment that was eroded from the mudflat surface not far from the deposition sites. Strata V and VI unconformably overlie the eroded wetland strata in some places. Dunes of Stratum V often preserve the underlying geological and archaeological deposits, preserving pedestaled remnants of the delta (or other inverted landforms) that stand in low relief above the surrounding scoured landscape. In a few places, a relatively well-preserved archaeological record is locally evident at the stratigraphic boundary between Stratum III and Stratum IV.
Biological composition and biofacies
The ORBD samples contain a rich and diverse paleontological mollusk and ostracode assemblage (Table 2), which are sometimes associated with calcareous algae. Supplemental Tables 1 and 2 summarize the ecological requirements of the mollusks and ostracodes, respectively, identified in this study. Figures 3 and 4 show the population density and relative abundance. Species associations with PHT radiocarbon age estimates (Table 1) show change through time in the local environment. Seventeen species of extremely rare to very abundant (1–756) gastropods and pelecypods were identified; whose adulthood ratios indicate a predominantly adult population, although juveniles are common. Twenty-three very rare to extremely abundant (5–3448) ostracode species were recovered. In both cases, we used the following abundance (numbers of individuals) bins: extremely abundant (>1000), very abundant (501–1000), abundant (101–500), moderately abundant (51–100), common (21–50), rare (6–20), and extremely rare (≤5). Suites of adults and juveniles characterize this group's stratigraphic distribution, whereas calcareous algae, only present in seven sites, are extremely rare to rare.
Table 2. Paleontological composition and taphonomic parameters. *Statistical count up to 300 specimens per sample. + = Mollusk float sample. § = Small sample collected for ostracodes only.
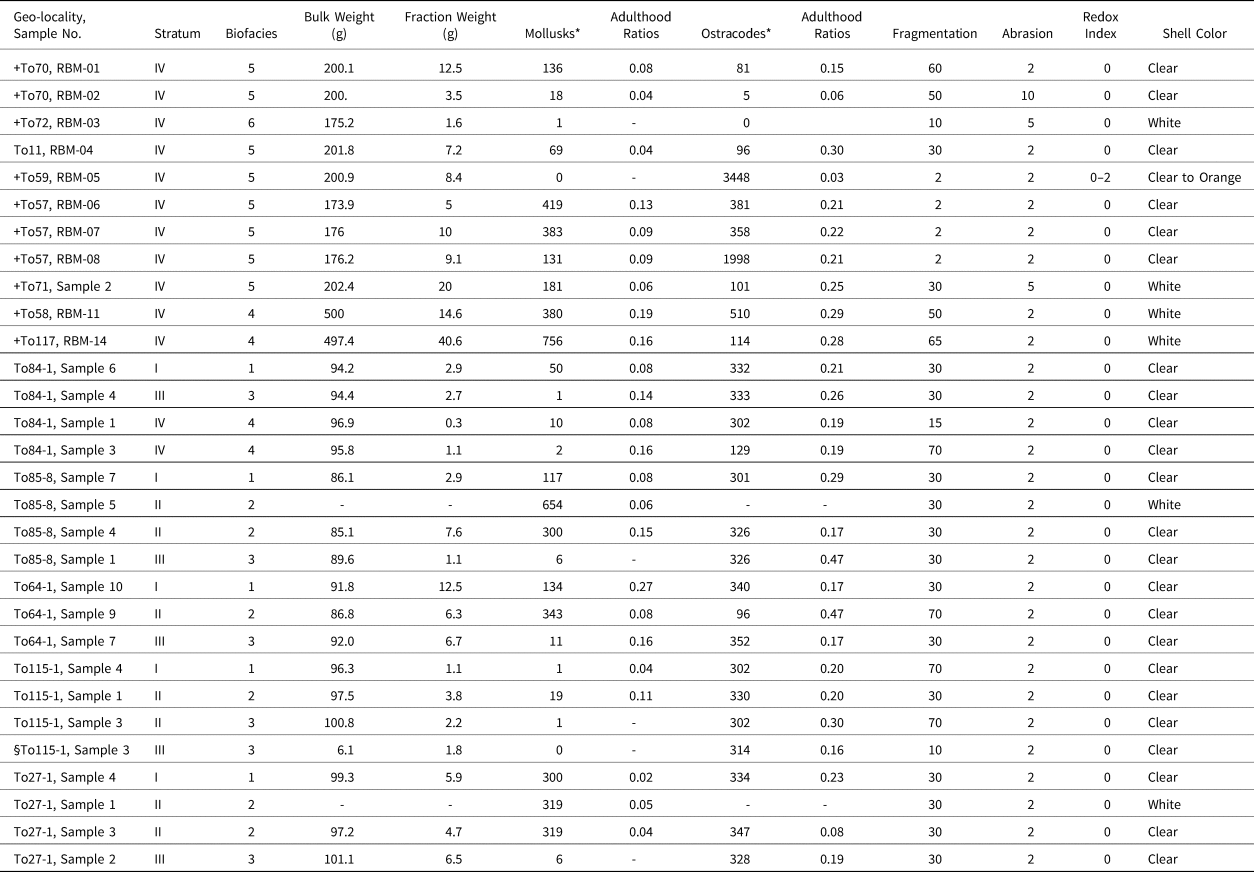

Figure 3. Mollusk biochronostratigraphy showing species relative abundance within the lithofacies and biofacies discussed in this investigation. Cold water indicators: Planorbula campestris and Valvata humeralis (see Supplemental Table 1).
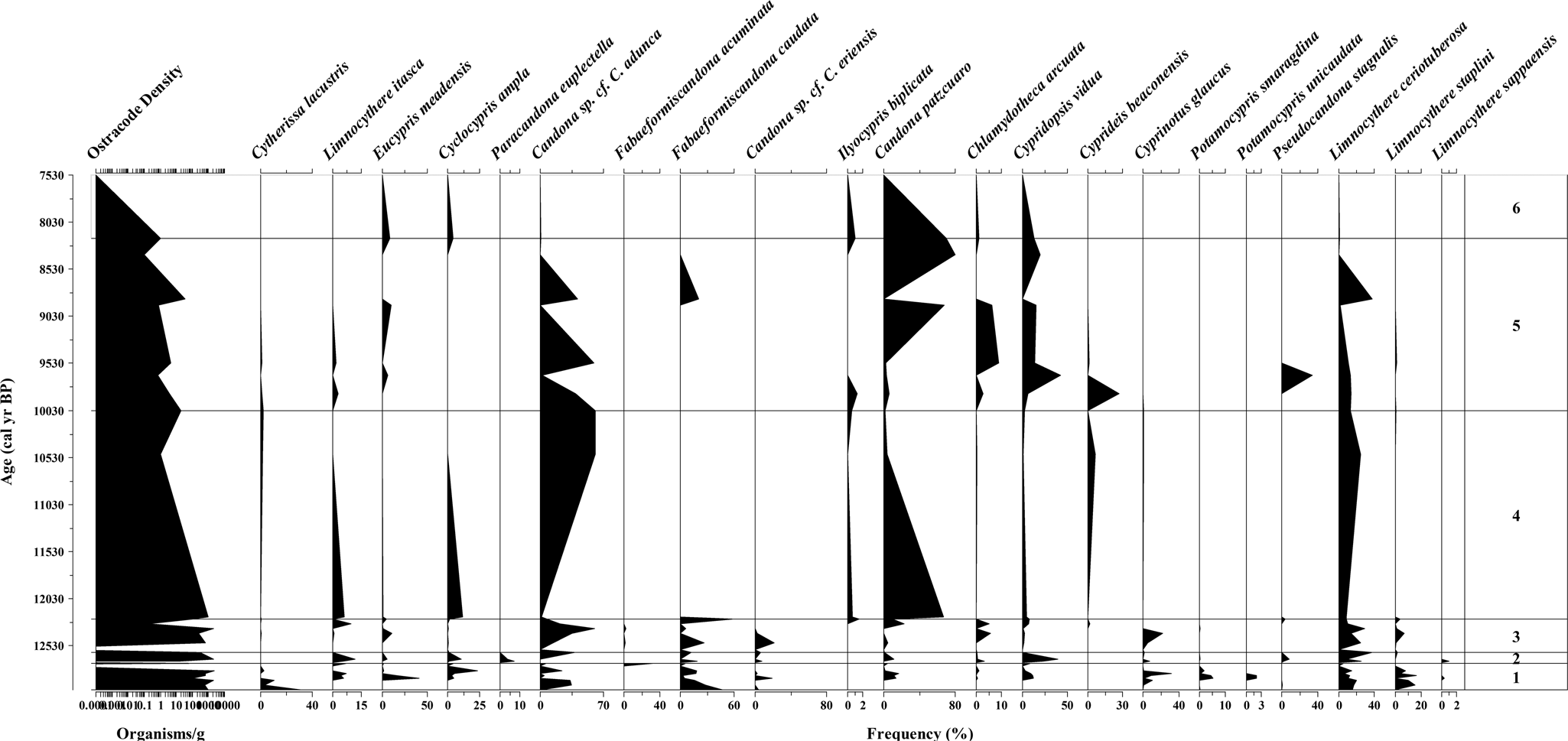
Figure 4. Ostracode biochronostratigraphy showing species relative abundance within the lithofacies and biofacies discussed in this investigation. Cold water indicators: Cyclocypris ampla, Eucypris meadensis, Candona sp. cf. C. eriensis, Cytherissa lacustris, Limnocythere itasca and Fabaeformiscandona caudata (see Supplemental Table 2).
The taphonomic features indicate a broad range of fragmentation (2–60%), but limited abrasion (2–5%), and the shells are rarely oxidized (redox index). These patterns indicate mixed evidence of reworking and/or post-burial diagenetic effects (Whatley, Reference Whatley1983; Adams et al., Reference Adams, Smith and Palacios-Fest2002). A dysoxic ecosystem would commonly generate a light- to dark-gray coating of shells. By contrast, an oxidizing milieu would produce light- to dark-orange stains of the shell. Shells were mostly clear or white, rarely orange, implying well-oxygenated waters with no subaerial exposure, with one exception (Table 2).
With a change in lithology, we also identified a change in the biological composition. We have identified six biofacies across the PHT and potentially into the middle Holocene (ca. 13,000–7500 cal yr BP; Figs. 3, 4). Biofacies 1–4 correspond with the generalized stratigraphic units (I–IV) identified for reaches of the ORBD in the central southern GSLD, and the Wishbone site vicinity, while the later Biofacies (5 and 6) correspond to well-dated, near-surface sedimentary deposits of the delta nearer the east margin of the basin. Biofacies 5 and 6 are likewise found in deposits of the ORBD (i.e., Stratum IV), although they rest on older Lake Bonneville stratigraphy. The time periods for each biofacies represent the direct or constrained 95th percentile confidence ranges of associated radiocarbon ages (see Table 1), rounded to the nearest 100 years.
Biofacies 1 (ca. >12,900 cal yr BP)
Biofacies 1 corresponds with the deposition of Stratum I (Table 1). Its timing likely predates the YDC onset based on our numerous dates from immediately after the onset from Stratum II. The presence of polygonal cracking at the Stratum I/II interface suggests that the lake lowered to below the study area prior to rising again to deposit Stratum II. We anticipate that deposition of Stratum I, and the Biofacies 1 environment in which it was deposited, represents a period of hundreds, not thousands, of years based on what we know of the regression of Lake Bonneville through the area prior to 12,900 cal yr BP (Godsey et al., Reference Godsey, Oviatt, Miller and Chan2011; Oviatt, Reference Oviatt2015)—effectively the onset of the YDC—but we do not have specific timing for our samples. Polygonal cracking at the top of Stratum I suggests a short dry period prior to the deposition of Stratum II.
Biologically, Biofacies 1 is dominated by the gastropods Armiger crista, Planorbula campestris, Gyraulus parvus, and Helisoma (Planorbella) trivolvis, and more rarely Valvata humeralis and Fossaria parva. The ostracode assemblage is composed of Limnocythere itasca, Eucypris meadensis, Cyclocypris ampla, Candona sp. cf. C. adunca, Fabaeformiscandona caudata, Candona sp. cf. C. eriensis, Candona patzcuaro, Cypridopsis vidua, Cyprinotus glaucus, Potamocypris smaragdina, Potamocypris unicaudata, Limnocythere ceriotuberosa, and intermittently Cytherissa lacustris and Limnocythere sappaensis. These species represent a generalized post Lake Bonneville subaquatic system that was still cold and possibly wet. Based on the co-occurrence of C. ampla and C. lacustris, it is presumed that water temperature was <14°C.
Biofacies 2 (12,800–12,500 cal yr BP)
Biofacies 2 corresponds with Stratum II and represents a shallow (<~2 m), lake-margin wetland. Its timing immediately post-dates the YDC onset based on a series of radiocarbon age estimates. The total 95th percentile confidence range for the eight age estimates we have for Stratum II covers 300 years, but scrutiny of the probability areas of these suggests that this stratum was deposited in perhaps 100 years or less, ca. 12,700 cal yr BP.
The biological composition of Biofacies 2 consists of the mollusks Oxyloma haydeni kanabensis, Lymnaea stagnalis jugularis, Lymnaea sp., V. humeralis, F. parva, G. parvus, and Planorbella sp., with decreasing occurrences of A. crista, P. campestris, and H. (P.) trivolvis. The ostracode assemblage is dominated by L. itasca, C. vidua, Pseudocandona stagnalis, and L. ceriotuberosa, with the brief appearance of Fabaeformiscandona acuminata, F. caudata, Candona sp. cf. C. eriensis, Chlamydotheca arcuata, Paracandona euplectella, and Cyprinotus glaucus. The occurrence of P. euplectella and P. stagnalis advocate for a lakeside marsh environment, whereas the presence of Ch. arcuata suggests seasonal warm water input. These species represent the above-mentioned generalized environment that was still cold (~14°C) with warming episodes compared to before and after this unit.
Biofacies 3 (ca. 12,600–12,400 cal yr BP)
Biofacies 3 corresponds with the deposition of Stratum III. Stratum III indicates a deepening of the Stratum II lake to levels no longer supporting emergent lakeside marshes (these were presumably pushed to the south with the lake margin). We have no direct radiocarbon dating on Stratum III, and the 95th percentile confidence ranges for the underlying Stratum II and overlying Stratum IV end and begin at ca. 12,500 cal yr BP, respectively, leaving no intervening time. Here, we provide a working range of 12,600–12,400 cal yr BP for Biofacies 3 based on the relative probabilities associated with radiocarbon ages from Stratum II and Stratum IV, but we suspect a longer period. Biofacies 2/Stratum II is discussed above. Biofacies 4 is based on the dates for human-made hearths found within Stratum IV, ~50 cm above the Strata III/IV interface. Stratum IV represents varied sediments of the ORBD after the Stratum III-lake had receded and could have happened quickly, but Stratum III is ~30 cm thick at these sites, suggesting a lacustrine stand of some duration.
Biofacies 3 is dominated by the gastropods A. crista and H. (P.) trivolvis, with intermittent or minor occurrence of P. campestris, V. humeralis, Stagnicola caperata, Physella virgata, and G. parvus. The ostracodes associated with this biofacies include Candona sp. cf. C. adunca, F. caudata, and L. ceriotuberosa, with the rare appearance of C. lacustris, E. meadensis, Candona sp. cf. C. eriensis, Ilyocypris biplicata, C. patzcuaro, Ch. arcuata, C. vidua, C. glaucus, P. stagnalis, and Limnocythere staplini. These species represent fresher-water conditions than the previous Biofacies 2, with the intermittent occurrence of seasonal species such as Ch. arcuata. Armiger crista, P. campestris, and V. humeralis, along with E. meadensis and Candona sp. cf. C. eriensis, support a cold-water environment, once again <14°C. It represents the ORBD Lake.
Biofacies 4 (ca. 12,500–12,000 cal yr BP)
Biofacies 4 represents the environment at about the time of the Wishbone site occupation. As with Biofacies 5 and 6, it is found within Stratum IV deposits of the ORBD, but during the Pleistocene. Inclusive of both the human-made hearth at the Wishbone site and the charcoal lens/possible hearth at the nearby Hello site (Table 1), which are dated to effectively the same time, we apply an approximate age range of 500 years. Biofacies 4 is based on two samples from the latter site collected from Stratum IV immediately above (To84-1, Sample 1) and below (To84-1, Sample 3) the charcoal lens. We thus assume that the age range is generally representative of Biofacies 4 and that Biofacies 4 is representative of the paleoenvironment at the times of the Wishbone and Hello site occupations.
The mollusk composition of Biofacies 4 contains H. (P.) trivolvis, G. parvus, P. virgata, and more rarely S. caperata, V. humeralis, Anodonta oregonensis, L. stagnalis jugularis, and O. haydeni kanabensis. Candona patzcuaro, C. ampla, L. itasca, and to a lesser concentration Cyprideis beaconensis, I. biplicata, C. vidua, and L. ceriotuberosa constitute the ostracode assemblage of Biofacies 4. Unexpectedly, Candona sp. cf. C. adunca is abundant throughout Biofacies 4. The unique presence of A. oregonensis indicates slow-flowing to stagnant wetland conditions, in sandy to silty substrates. These species represent a generalized environment with increasing temperature (>14°C, but ≤20°C; Supplemental Table 2). This is the ORB Delta cooler stage.
Biofacies 5 (10,200–8000 cal yr BP)
Biofacies 5 is again from the ORBD (Stratum IV), but represents a late early Holocene environment from ca. 10,200 cal yr BP until approximately the onset of the middle Holocene. This represents an 1,800-year gap from the end of the Biofacies 4 interval, but only because we did not sample the intervening time frame. We do not know at what time the transition between the two environments occurred, or if there might be other distinguishable biofacies.
Biofacies 5 is composed of the mollusks P. virgata and G. parvus, with a decreasing occurrence of P. campestris, V. humeralis, S. caperata, and A. crista. Lymnaea stagnalis jugularis, O. haydeni kanabensis and the pea clams Pisidium (Cyclocalyx) casertanum and P. (C.) compressum are restricted to the base of the record, whereas H. (P.) trivolvis is extremely rare throughout the biofacies. The ostracodes include Candona sp. cf. C. adunca, L. ceriotuberosa, C. patzcuaro, Ch. arcuata, C. vidua, and more rarely F. acuminata, C. beaconensis, I. biplicata, L. staplini, and P. stagnalis, which occur intermittently throughout. However, E. meadensis reappears towards the top of the record to prevail in Biofacies 6. The faunal assemblage advocates for warming conditions relative to Biofacies 4. Estimated water temperature would have ranged from 13–24°C or more as the area was desiccating.
Biofacies 6 (ca. 8600–7400 cal yr BP)
Biofacies 6 is within the middle Holocene and suggests persistent surface groundwater and limited remnant wetlands between ca. 8600–7400 cal yr BP based on the 95th percentile confidence range of a single date (Table 1). Uppermost Biofacies 6 only contains the mollusks H. (P.) trivolvis. No ostracodes were present in this interval. The sole occurrence of H. (P.) trivolvis indicates the ORBD was desiccating to a status similar to today where there is surface groundwater and wetland habitat in the GSLD.
The strontium isotope evidence
The measured 87Sr/86Sr ratios are presented in Supplemental Table 3 and Figure 5. Samples To84-1-Sample 6, To64-1-Sample 7, and To27-1-Sample 1-15 are presented in Supplemental Table 3, but their data are not included in the resulting regressions or Figure 5 because the small amount of material and/or the low concentration of these samples precluded precise measurement of the 87Sr/86Sr ratios and the resulting uncertainty is much larger than the normal and accepted precision levels.
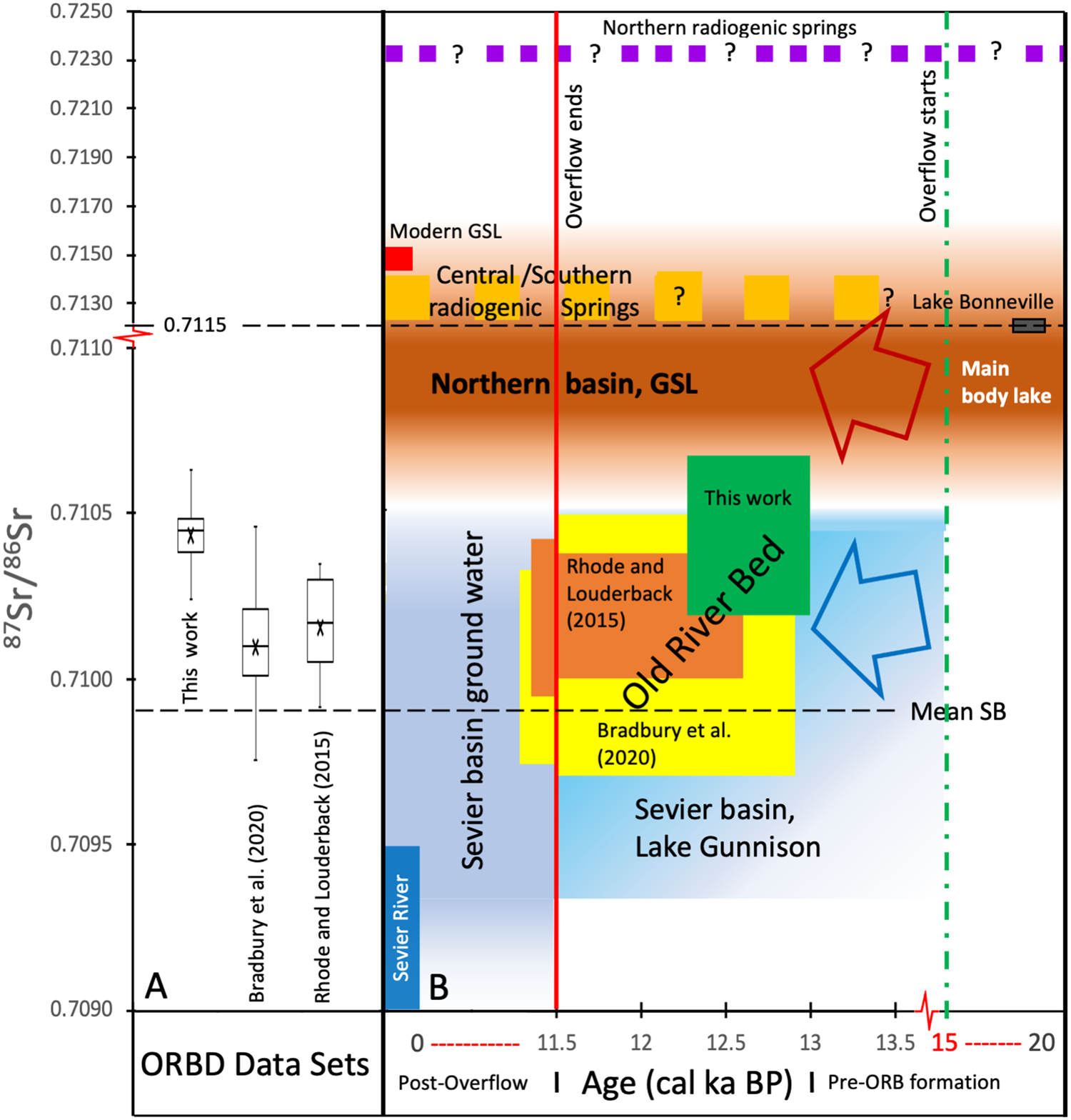
Figure 5. (A) Box plots of 87Sr/86Sr ratios of gastropods from Rhode and Louderback (Reference Rhode, Louderback, Madsen, Schmitt and Page2015), Bradbury et al. (Reference Bradbury, Jewell, Fernandez, Lerback, DeGraffenried and Petersen2020), and this work. The mean values are specified with an X. (B) Schematic diagram comparing the ranges of 87Sr/86Sr ratios of various water sources through time and of gastropods from the Old River Bed Inland Delta. Age is in cal yr BP and centered around ages of likely Gunnison overflow (note scale change at 11,500 cal yr BP and 14,000 cal yr BP). The vertical scale has a scale change at 0.7110 to accommodate the much more radiogenic values of northern water sources. The vertical dashed green line at 15,000 cal yr BP marks the age of likely onset of overflow from Lake Gunnison via the ORBID into the northern basin (Godsey et al. Reference Godsey, Oviatt, Miller and Chan2011). The red vertical line marks cessation of overflow at ~11,500 cal yr BP (Oviatt, Reference Oviatt1988). See text for discussion. (For interpretation of the references to color in this figure legend, the reader is referred to the web version of this article.)
The gastropods in this study have 87Sr/86Sr ratios between 0.71025–0.71063, with a mean value of 0.71044. Stratigraphic correlations and superposition result in calibrated ages for the samples of between 12,250 to >12,750 cal yr BP. The 87Sr/86Sr ratios and calibrated 14C ages of the two other published data sets of gastropods from the ORBD are also shown in Figure 5. The 87Sr/86Sr ratios of Rhode and Louderback (Reference Rhode, Louderback, Madsen, Schmitt and Page2015) range from 0.70991–0.71035 (mean of 0.71016), with ages between 9990–12,570 cal yr BP (rounded to nearest decade). The data of Bradbury et al. (Reference Bradbury, Jewell, Fernandez, Lerback, DeGraffenried and Petersen2020; Rust channel sample excluded because the authors concluded direct influence of extant stream water) has a larger overall range in 87Sr/86Sr ratios and ages, with values between 0.70975–0.71046 (mean of 0.71010) and 9300–12,900 cal yr BP, respectively. Despite the larger overall range of 87Sr/86Sr ratios, the Bradbury et al. (Reference Bradbury, Jewell, Fernandez, Lerback, DeGraffenried and Petersen2020) dataset has a very similar mean to the Rhode and Louderback (Reference Rhode, Louderback, Madsen, Schmitt and Page2015) dataset. In contrast, our dataset has an appreciably more radiogenic average 87Sr/86Sr ratio of 0.70144. The two published datasets also include samples from the period after Lake Bonneville had dropped below the ORBD threshold (~1395 m), although no significant difference in the range of 87Sr/86Sr values is observed in these younger 9300–11,500 cal yr BP samples. The Bradbury et al. (Reference Bradbury, Jewell, Fernandez, Lerback, DeGraffenried and Petersen2020) and Rhode and Louderback (Reference Rhode, Louderback, Madsen, Schmitt and Page2015) samples are from the proximal ORBD, 10–15 km to the southeast.
DISCUSSION
Lake Bonneville had regressed to below our study area sometime after 15,000 cal yr BP, and probably between 14,000–13,000 cal yr BP (Godsey et al., Reference Godsey, Oviatt, Miller and Chan2011; Oviatt, Reference Oviatt2015). Our geomorphological data demonstrate that the basin nevertheless remained wet, with a large waterbody, which we call the Old River Bed lake (ORBDL), at ~1295 m asl; the ORBDL occupied a large area in the GSLD (Fig. 1). This lake is represented by Strata I and III. The ORBD channel system originated in this context, extending northward from where the ORB river emptied onto the flat basin floor in the area now occupied by Dugway Proving Ground. The lake margins shifted and the lake reshaped itself through time causing the delta margins to shift—at times fluvial channels emptied into the lake, and at other times the delta formed a widespread inland distributary system that did not empty directly into the lake. By the early Holocene (Madsen et al., Reference Madsen, Oviatt, Young, Page, Madsen, Schmitt and Page2015a) the ORBD supported extensive wetlands. Our mollusk and ostracode data track well with this characterization, showing a shift from colder to warmer behind the changes that ultimately resulted in complete desiccation in the middle Holocene. These changes had implications for human populations, which were likely drawn to the rich and developing wetlands on the ORBD.
The biological composition of mollusks and ostracodes reached its highest diversity in samples from ORBDL Stratum I, reflecting cold conditions, lowered evaporation, and surface water input prior the onset of the YDC. Many species established a biocenosis. Figure 3 shows a diverse mollusk and abundant ostracode population. Ostracodes are also diverse and rich (Fig. 4). Locally, L. sappaensis, C. glaucus, C. lacustris, and Candona sp. cf. C. adunca are common. However, Candona sp. cf. C. adunca is not abundant in this interval. Cytherissa lacustris is erratic throughout the area, but most abundant in Locality To115. The occurrence of the Holarctic species P. campestris (snail) and the ostracodes C. ampla and C. lacustris indicates a shallow, cold-water environment with a salinity no higher than 300 mg/L total dissolved solids (TDS) (Curry et al., Reference Curry, Delorme, Smith, Palmer, Stiff, Horne, Holmes, Rodriguez-Lazaro and Viehberg2012). Based on the temperature tolerance listed in Supplemental Table 2 for ostracodes, we infer that water temperature during deposition of Biofacies 1 was <14°C (Bunbury and Galweski, Reference Bunbury and Gajewski2009). The co-occurrence of C. ampla and C. lacustris during this interval is consistent with boreal conditions (Curry and Baker, Reference Curry and Baker2000) and with higher dissolved oxygen concentrations (>3 mg/L) in an oligotrophic to mesotrophic setting (Belis et al., Reference Belis, Lami, Guilizzoni, Ariztegui and Geiger2008). The 87Sr/86Sr ratios of Stratum I show some of the highest values recorded in this study, ranging from 0.71033–0.71048 (Supplemental Table 3). These values suggest that, at this time, Lake Gunnison (0.70912–0.71049 87Sr/86Sr ratios) contributed freshwater to Stratum I.
There is currently no direct indication of people using the ORBD in Stratum I times, although we know they had begun occupying the region by at least 12,900 cal yr BP based on radiocarbon dates on hearth features at Bonneville Estates Rockshelter on the west margin of the basin (Graf, Reference Graf, Graf and Schmitt2007; Goebel and Keene, Reference Goebel, Keene, Parezo and Janetski2014; Smith et al., Reference Smith, Duke, Jenkins, Goebel, Davis, O'Grady, Stueber, Pratt and Smith2020; Goebel et al., Reference Goebel, Hockett, Rhode and Graf2021). We surmise that the developing wetland habitat of the ORBD to the south (i.e., up the gradient and above the level of the ORBDL) was a key draw to the area for these people (also see Madsen et al., Reference Madsen, Schmitt and Page2015b).
The dark, organic- and charcoal-rich sedimentary deposit of Stratum II hosts a diverse ostracode and mollusk assemblage consisting of a mixed population of adults and juveniles indicating an in situ assemblage (>1:5 adulthood ratios). The dominant ostracode species in Biofacies 2 (Stratum II) across the area are F. acuminata, C. vidua, F. caudata, L. ceriotuberosa, L. itasca, and Candona sp. cf. C. adunca, although their occurrence throughout the southern GSLD is uneven (Fig. 4). Gastropods V. humeralis (the most abundant across the area), F. parva, S. caperata, and G. parvus, A. crista, H. (P.) trivolvis, and Oxyloma haydeni kanabensis (juveniles) are present in this unit (Fig. 3).
The ostracodes from Stratum II include two species, L. sappaensis, and P. euplectella, not recorded elsewhere in the ORBD, although neither of them is abundant. In the case of L. sappaensis, the scarce shells might have been reworked. Paracandona euplectella, however, fits well with the hypothesis that the black mat was deposited in a lakeside wetland or extended shallow pond. To the best of our knowledge, this is the first report of P. euplectella in the southern GSLD. The assemblage, however, is consistent with a spring-fed system or perennial lentic stream discharge into a peat bog or a vast, shallow wetland. The disappearance of C. lacustris and occurrence of L. itasca and Cypridopsis vidua may be associated with freshwater conditions from groundwater influx and/or additional freshwater sources (Pfaff et al., Reference Pfaff, Schulmeister, Cronin, Smith and Aber2005) generating more mesotrophic conditions than during Biofacies 1. Based on the temperature range of the ostracode faunal assemblage, the site was still cold (<14°C), but the water was shallower and with greater groundwater influx (Supplemental Table 2). The 87Sr/86Sr ratios range between 0.71024–0.71063, still under the influence of Lake Gunnison.
As with Biofacies 1, there is no direct indication of human occupation of the ORBD at this time. Any location where Stratum II was identified in the current study would have been under water (Fig. 1). We do not know how far the waterbody in which Stratum II was deposited would have extended, but based on its location and elevation, the area would have been a shallow wetland with abundant emergent vegetation for 10 km or more in the northwest-southeast direction and unavailable to humans. Much as with Stratum I, people may have accessed peripheral areas in Stratum II times, but we do not have direct evidence for this.
The rich and diverse microinvertebrate fauna (ostracodes and mollusks) of Biofacies 3, representing the depositional environment of Stratum III, indicates that a shallow lake occupied the GSLD for, at least, 200 years and received alternating input of ground water and runoff during the time Biofacies 3 existed. The fossils in this unit show little abrasion, implying in situ deposition in a permanent water body. The slow flow of horizontal currents introduced the superposed layers of ostracode valves throughout the area. The abundance of Candona sp. cf. C. adunca and L. ceriotuberosa in the ostracode-only lens implies that the site became deeper, contrasting with the mollusk signature across the area. Shallow, weedy environments containing abundant root casts support this interpretation. The ostracode lens is consistent with a brief deepening episode during deposition of Stratum III in response to water-table fluctuations or wet-dry events that characterized a fluctuating climate during the YDC. These deposits' marly nature supports the hypothesis that this stratum is a facies deposited in the ORBDL.
The ostracode marl of Stratum IV (in which abundant carbonate grains were identified under the microscope) contains mollusk shells and ostracodes of Biofacies 4. Figures 3 and 4 show the transition from freshwater to saline water in an increasingly drier environment in the upper half of Stratum IV (Fig. 4). Increasingly drier conditions are inferred from the association of C. vidua, C. patzcuaro, and L. ceriotuberosa despite the dominance of F. caudata, which is consistent with Delorme's (Reference Delorme1969, p. 1473) interpretation of the Candona rawsoni group (C. patzcuaro belongs to this group). The four species tolerate a wider range of environmental conditions, although F. caudata's temperature range is more limited than that of the associated fauna. Temperature unlikely exceeded 20°C (Supplemental Table 2).
Humans visited the Biofacies 4 environment, as demonstrated at the Wishbone site (42TO6384; To64), which contains stone tools, waterfowl bones (food refuse), and two Haskett-style projectile points of the Western Stemmed technological tradition (Duke et al., Reference Duke, Rice, Young and Byerly2018a; Smith et al., Reference Smith, Duke, Jenkins, Goebel, Davis, O'Grady, Stueber, Pratt and Smith2020). The central hearth at the site yielded three statistically consistent willow-wood charcoal dates (10,370 ± 30, 10,370 ± 40, 10,430 ± 40 14C BP) with a combined radiocarbon age of 10,390 ± 20 14C BP (ca. 12,300 cal yr BP) (Table 1). The Wishbone site occupants took advantage of the newly formed wetland, occupying linear dry, streamside landforms that provided deep access to the ORBD marshes. The nearby Hello site was occupied at about the same time, based on the date from a charcoal lens that appears to be a hearth. The Biofacies 4 samples are from immediately above and below this lens, and thus generally represent the environment at the time of these occupations. The timing of Biofacies 4 is tied to the age of the site hearths, which could extend as late as ca. 12,000 cal yr BP, but continuous human occupation of the ORBD wetlands (and deposition of Stratum IV), as represented by Western Stemmed and Pinto points, is well established through the early Holocene (Duke, Reference Duke2011; Madsen et al., Reference Madsen, Schmitt and Page2015b).
A eurytopic faunal assemblage characterized Biofacies 5, beginning ca. 9500 cal yr BP. Ostracodes are abundant, but then decline abruptly after ca. 7900 cal yr BP. Five ostracode species dominate (F. acuminata, L. ceriotuberosa, C. patzcuaro, C. vidua, and Ch. arcuata), with rare appearances of L. itasca and C. lacustris. The latter is occasional but consistent throughout most of the unit. Mollusks are also the most diverse and abundant in this phase. The species associated with Biofacies 5 include G. parvus, V. humeralis, P. virgata, and P. campestris. Two species, L. stagnalis jugularis and O. haydeni kanabensis, occur only at the base of the record. At a time of gradually rising temperatures, it is inferred that Biofacies 5 indicates a period of dilute, cold-water input into the wetlands through groundwater discharge in the ORBD area that originated in the Sevier basin (Oviatt et al., Reference Oviatt, Madsen and Schmitt2003, Reference Oviatt, Madsen, Miller, Thompson and McGeehin2015; Madsen et al., Reference Madsen, Oviatt, Young, Page, Madsen, Schmitt and Page2015a; Rhode and Louderback, Reference Rhode, Louderback, Madsen, Schmitt and Page2015; Bradbury et al., Reference Bradbury, Jewell, Fernandez, Lerback, DeGraffenried and Petersen2020) (Figs. 3, 4). However, these biofacies likely reflect constant water temperature and water chemistry changes, as shown in Supplemental Table 2. The temperature range during Biofacies 5 was 13–24°C, the upper limit for C. beaconensis, and the lower limit for Ch. arcuata.
The temporal range for Biofacies 5 is significant in that it largely post-dates the bulk of early Holocene channels of the ORBD, which formed early in that time interval (ca. 11,700–10,200 cal yr BP), and represent its maximum active wetland size (Madsen et al., Reference Madsen, Oviatt, Young, Page, Madsen, Schmitt and Page2015a). This interval also represents the most intensive period of occupation by people (Duke, Reference Duke2011; Madsen et al., Reference Madsen, Schmitt and Page2015b). Madsen et al. (Reference Madsen, Schmitt and Page2015b, p. 239) reported 70 radiocarbon age estimates on black mat plant material or organic sediment from across the ORBD and found the majority to date to this period; only five, dating between ca. 9900–9100 cal yr BP, occur within the time of Biofacies 5. Thus, we have effectively bookended the period of intensive human use of the ORBD with the data herein and do not know when the transition from Biofacies 4 to 5 occurred. Madsen et al. (Reference Madsen, Schmitt and Page2015b, p. 49) described the period of our Biofacies 5 as marking “…the last pulses of wetland environments. As surface flow stopped reaching the Wild Isle and Wildcat reaches of the delta, wetland productivity would have become increasingly isolated, until replaced by a generally desiccated playa and dune landscape.”
Our paleoenvironmental data support this interpretation, and the archaeological data do as well. People appear to have substantially diminished or even discontinued their use of the ORBD between ca. 9800–9500 cal yr BP, with the emergence of ground stone use for intensive seed processing at Danger Cave at the basin margin near Wendover, Utah, at about the same time (Rhode et al., Reference Rhode, Madsen and Jones2006). Our Biofacies 5 samples come from the east distal delta, east of Wildcat Mountain, and are associated with late-dating ORBD channels that were not previously recognized (Fig. 1). Duke (Reference Duke2011) argued that archaeological patterning changes through time alongside shifting distributary output in a west-to-east manner on the distal portion of the ORBD between Wild Isle Dunes and Wildcat Mountain. This spatial pattern is shown in a temporal framework by a shift from large projectile points, such as the Haskett style from Wishbone to smaller Western Stemmed styles and Pinto; the east delta region sites are dominated by Pinto over Western Stemmed.
Biofacies 6 is marked by the sharp disappearance by the middle Holocene of the cold-water indicators typical of Biofacies 5. The sole occurrence of H. (P.) trivolvis indicates a semi-arid environment that periodically flooded, which permitted arrival of this pulmonate snail. Biofacies 6 may be interpreted as a desiccation episode as the area evolved into the semi-arid region of today (mean low of 5°C; mean high of 19°C). The poor record prevents us from inferring the temperature range at this interval, but we estimate temperatures >20°C.
Although surface water representing the last vestiges of the ORBD was present for Biofacies 6, it was not likely associated with the bulk of the archaeological record in the east delta. This interval is well understood to be too late for Western Stemmed projectile points, and Pinto points do not appear to have persisted to this time either. The best spatial association between sites and dated channels in the area suggests most human activity dates to the early part of the Biofacies 5 period (ca. 10,200–9500 cal yr BP). The minor wetlands of Biofacies 6 may represent spot occurrences of surface groundwater along relict, otherwise desiccated, ORBD channels during short cool/wet periods versus a declining persistence of the ORBD per se. The presence of a few large, corner-notched points not otherwise found on the ORBD, but consistent with those seen in nearby caves and rockshelters in times consistent with Biofacies 6 (Hoskins, Reference Hoskins2016; Hockett and Goebel, Reference Hockett and Goebel2019), may be the representative archaeological signature for these final wetlands.
Regression of Lake Bonneville as a closed-basin lake was probably caused by global or hemispheric dry climatic conditions coincident with the Bølling-Allerød Interstadial (14,700–13,300 cal yr BP) (Godsey et al., Reference Godsey, Oviatt, Miller and Chan2011). The Bølling-Allerød Interstadial was followed by the YDC (Rasmussen et al., Reference Rasmussen, Andersen, Svensson, Steffensen, Vinther, Calusen and Siggaard-Andersen2006; Steffensen et al., Reference Steffensen, Andersen, Bigler, Clausen, Dahl-Jenzen, Fischer and Goto-Azuma2008; Cheng et al., Reference Cheng, Zhang, Spötl, Baker, Sinha, Li and Bartolomé2020), which is of particular interest in the context of this paper. Cheng et al. (Reference Cheng, Zhang, Spötl, Baker, Sinha, Li and Bartolomé2020) gave the following bounding ages for the YDC: 12,870 ± 30 to 11,600–11,700. If these ages are rounded off to the nearest 100 yr, the duration of the YDC lasted from ca. 12,900–11,700 cal yr BP, or for 1200 yr.
Literature on the climate of the YDC in western North America is vast, but the following references are pertinent to interpretations of YDC paleoclimate in the Bonneville basin (e.g., Quade et al., Reference Quade, Forester, Pratt and Carter1998; Wigand and Rhode, Reference Wigand, Rhode, Herschler, Madsen and Currey2002; Oviatt et al., Reference Oviatt, Miller, McGeehin, Zachary and Mahan2005; Hall et al., Reference Hall, Penner, Palacios-Fest, Metcalf and Smith2012). It is possible that part of the apparent spatial variability in YDC climate that has been documented in western North America (Meltzer and Holliday, Reference Meltzer and Holliday2010) is a function of temporal variability in climate during the ca. 1200 years of the YDC (perhaps climate was cool and dry early in the YDC, then became cool and wet later in the YDC?). Different authors working in different places and with different proxies have concluded that YDC climates were wet and cool, dry and cool, warm and wet, or other possibilities (Meltzer and Holliday, Reference Meltzer and Holliday2010). Precision and accuracy of dating control, gradual accumulation of more and better evidence, and the evolution of thoughts and interpretations, may also be factors in the lack of agreement among studies. Although it is possible to legitimately arrive at different interpretations of YDC climate, it seems likely that in the Bonneville basin climate was relatively cool and dry compared to times immediately before and after the YDC (Oviatt, Reference Oviatt2014; Rhode, Reference Rhode, Oviatt and Shroder2016); in this paper we adopt this interpretation.
Two ostracode species are particularly relevant in this investigation because of their limited occurrence and apparent association with cold- and/or deep-water environments: Cytherissa lacustris and Candona sp. cf. C. adunca. Cytherissa lacustris, described initially from deep lakes (Delorme, Reference Delorme1970; Delorme and Zoltai, Reference Delorme and Zoltai1984), is currently known to live in shallow, spring-fed wetlands of the Arctic Circle (Bunbury and Gajewski, Reference Bunbury and Gajewski2009; Krzymińska and Namiotko, Reference Krzymińska and Namiotko2011), which resemble the ORBD environments. It is a geographically widespread species distributed from shallow to deep waters (as deep as 200 m in Lake Constance, Switzerland; Meisch, Reference Meisch2000), but common at 20–30 m depth (e.g., Mondsee, Austria; Danielopol, Reference Danielopol, Danielopol, Carbonel and Colin1990). A cold stenothermic form, C. lacustris thrives better in oligo-mesotrophic lakes, but it is also common in shallow systems. Anthropogenic eutrophication affects it severely (Wilkinson et al., Reference Wilkinson, Bubikyan and Gulakyan2005). Forester (Reference Forester, Ruddiman and Wright1987) reported C. lacustris from shallow, cold groundwater-fed wetlands in Lake Bonneville. Oviatt (Reference Oviatt2017) argued that C. lacustris, an important marker in Lake Bonneville marl, thrives in cold water with low total dissolved solids (TDS) (Delorme, Reference Delorme1969). Cytherissa lacustris is not common in this basin, but appears in response to pulses of cold, fresh groundwater into the lake. We cannot discard the possibility that the specimens of C. lacustris in our samples have been reworked because the carapace is robust and easily transported with no damage. However, the occurrence of adults and juveniles advocates for a local population.
Candona sp. cf. C. adunca, the endemic and extinct ostracode species of Lake Bonneville, was found by Lister (Reference Lister1975) in cores taken by A.J. Eardley from the southeast margin of Great Salt Lake (the Saltair and Section 28 cores), but he did not provide stratigraphic details. The species appears to be restricted to the late transgressive phase of Lake Bonneville and persisted into the early regressive phase when Lake Bonneville was freshest (Oviatt, Reference Oviatt2017). The specimens identified here as Candona sp. cf. C. adunca correspond well with Lister's (Reference Lister1975, p. 10) original description and illustrations of the species.
Our specimens are identical to Oviatt's (Reference Oviatt2017, fig. 4) illustration of the species. Lister (Reference Lister1975) highlighted the pronounced posteroventral projection of the outer margin. Like Lister's species, our specimens lack the pronounced posterodorsal hump characterizing Fabaeformiscandona caudata. In terms of size, Candona sp. cf. C. adunca in this study is larger than F. caudata and in the same size range of those of Lister (Reference Lister1975) and Oviatt (Reference Oviatt2017). The possibility exists that our specimens could be another form of Fabaeformiscandona (such as F. acuminata), but a higher posterodorsal hump distinguishes our specimens of F. acuminata. It is a puzzle to precisely interpret Candona sp. cf. C. adunca in the ORBD wetland deposits; we think it is likely that the specimens identified in this study as Candona sp. cf. C. adunca are indeed the species. A possibility that we think is less likely, but which we haven't been able to fully evaluate, is that Candona sp. cf. C. adunca, and perhaps other ostracodes, were reworked in low-energy conditions from older Lake Bonneville deposits.
As far as we are able to determine, Candona sp. cf. C. adunca lived in cold-water environments. What seems more relevant, though, is that it is associated with other cold-water indicators, such as the ostracode L. itasca and the gastropod P. campestris. Their co-occurrence with more fragile forms such as L. itasca suggests all these species co-existed. To the best of our knowledge, Candona sp. cf. C. adunca has not been found in non-Bonneville marl deposits in the southern GSLD. This is the first formal report of Candona sp. cf. C. adunca in the area that appears to be supported by the 87Sr/86Sr isotopes identified in deposits of the ORBD.
The 87Sr/86Sr ratios of Rhode and Louderback (Reference Rhode, Louderback, Madsen, Schmitt and Page2015) range from 0.70991–0.71035 (mean of 0.71016), with ages between 9,990–12,570 cal yr BP (rounded to nearest decade). Irrespective of the differences in the datasets, all samples except To84-1-6, To27-1-3-10, and To27-1-1-14 (all from Stratum II) are within the range of values of Lake Gunnison (0.70930–0.71049; mean of 0.70989; Hart et al., Reference Hart, Quade, Madsen, Kaufman and Oviatt2004). Rhode and Louderback (Reference Rhode, Louderback, Madsen, Schmitt and Page2015, p. 25, Table 2.1) used the fact that their 87Sr/86Sr values are within the range of Lake Gunnison values to conclude that there was “continued overflow from Lake Gunnison via the ORBD or leakage of groundwater from the Sevier basin through alluvium.” Bradbury et al. (Reference Bradbury, Jewell, Fernandez, Lerback, DeGraffenried and Petersen2020) cited the geomorphological studies of Oviatt et al. (Reference Oviatt, Madsen and Schmitt2003) that support high energy flow through the older channels of the ORBD to posit that their older samples had a Lake Gunnison water source via overflow as well. They also concluded that their samples younger than the likely age of the cessation of overflow at ca. 11,500 cal yr BP (Oviatt, Reference Oviatt1988) were sourced from groundwater from the Sevier basin, which is thought to likely have a comparable Sr isotopic range as the former Gunnison waters (Madsen et al., Reference Madsen, Oviatt, Young, Page, Madsen, Schmitt and Page2015a; Rhode and Louderback, Reference Rhode, Louderback, Madsen, Schmitt and Page2015).
Because their data are bound by the Gunnison values, Rhode and Louderback (Reference Rhode, Louderback, Madsen, Schmitt and Page2015) and Bradbury et al. (Reference Bradbury, Jewell, Fernandez, Lerback, DeGraffenried and Petersen2020) ruled out both Sr influx from more radiogenic northern water sources (Gilbert-episode lake; Oviatt, Reference Oviatt2014) and discharge from mountain and piedmont aquifers recharged during Lake Bonneville times (Oviatt et al., Reference Oviatt, Madsen, Miller, Thompson and McGeehin2015; Schmitt and Lupo, Reference Schmitt and Lupo2018), respectively. Their Sr data as well as ours, however, do not rule out either of these as contributors to the waters of the ORBD (as well as not ruling out radiogenic spring contributions; see Hart et al., Reference Hart, Quade, Madsen, Kaufman and Oviatt2004; Bradbury et al., Reference Bradbury, Jewell, Fernandez, Lerback, DeGraffenried and Petersen2020). For example, mixing between non-radiogenic Sr reservoirs, such as an earlier version of the Sevier River and one or multiple radiogenic reservoirs present at the time, could produce all of the ORBD 87Sr/86Sr values. Radiogenic spring water contribution to ORBD sediments is discussed in Bradbury et al. (Reference Bradbury, Jewell, Fernandez, Lerback, DeGraffenried and Petersen2020), but insufficient evidence exists of a direct radiogenic contribution by spring water to the ORBD during the period of interest, or of how the inputs may have varied across the area.
A significant or sole role for Lake Gunnison for our samples is also possible. The three data points of ours that outlie the composition of Lake Gunnison are at most only 0.00014 greater. By contrast, 87Sr/86Sr ratios of samples from Lake Gunnison (Hart et al., Reference Hart, Quade, Madsen, Kaufman and Oviatt2004) have a range of 0.0012, which is almost an order of magnitude larger variation. It is likely that Lake Gunnison (and later groundwaters from the Sevier basin) would have an even larger range of 87Sr/86Sr than the seven samples measured by Hart et al. (Reference Hart, Quade, Madsen, Kaufman and Oviatt2004). This is supported by a Sr study on carbonate dust (Carling et al., Reference Carling, Fernandez, Rey, Hale, Goodman and Nelson2020) that shows dust from the dry bed of Sevier Lake with 87Sr/86Sr values between ~0.7097–0.7108, but with a similar mean (0.71008), as in-place carbonates (0.70989; Hart et al., Reference Hart, Quade, Madsen, Kaufman and Oviatt2004).
There are a number of non-unique paths between Sr reservoirs at the time of the ORBD deposition that either solely or in conjunction with other reservoirs could have produced the results. However, because of geomorphologic, stratigraphic, hydrologic, and the other lines of evidence presented by the various, previously cited authors, our preferred interpretation also agrees with that of Bradbury et al. (Reference Bradbury, Jewell, Fernandez, Lerback, DeGraffenried and Petersen2020) and Rhode and Louderback (Reference Rhode, Louderback, Madsen, Schmitt and Page2015)—namely that the 87Sr/86Sr values of ORBD gastropods result from the influx of Lake Gunnison waters prior to the cessation of overflow. The samples of the other datasets that are younger than the cessation of overflow have similar results, and therefore Lake Gunnison-sourced ground water is plausible. However, data from all of the ORBD samples do not preclude contribution by or mixing between less- and more-radiogenic water sources. In other words, the whole of the ORBD Sr data is inconclusive on the source of Sr in these samples, but are not contradictory to models of early overflow from Lake Gunnison and later Sevier basin groundwater.
Three of the six biofacies identified in this investigation suggest intervals of shallow lake and wetland formation in the southern GSLD during the YDC that we refer to as the ORBDL. Strata I and III contain ostracodes and mollusks that indicate a lacustrine environment, with ostracode species typically found in cold freshwater habitats. A facies similar to that of Strata I and III has not been found previously in the Bonneville basin, and its presence indicates that, despite the likely dry climate, the basin floor was very wet at the time Strata I and III were being deposited. One possibility for the interpretation of Strata I and III is that an extensive water body or lake existed in the GSLD at those times. The maximum elevation of occurrences of Stratum III near the Wishbone site is close to 1295 m, and if that elevation were to be taken as the shoreline of the lake and traced around the GSLD, the line would connect with Great Salt Lake (Fig. 1). Such a lake would have been very large, but shoreline landforms or offshore sediments of this age have not been recognized. Another possible interpretation of Strata I and III is that the sediments were deposited in lakes that were confined by landforms, such as natural levees, associated with the ORBD wetland system. Qualifying landforms are not apparent now in the GSLD, but considering the extensive Holocene-aged deflation in this area (Oviatt et al., Reference Oviatt, Madsen and Schmitt2003; field observations as part of this study), it is possible that confining landforms could have been present while the ORBDL was present, but are now erased from the landscape. For this paper, we prefer the interpretation that Strata I and III were deposited in a large, but short-lived lake because that interpretation is consistent with the available observations. More information is needed to be certain.
By contrast with Pigati et al. (Reference Pigati, Rech, Quade and Bright2014), Springer et al. (Reference Springer, Manker and Pigati2015), and Forester et al. (Reference Forester, Carter, Quade and Smith2016), who associated hypogean ostracodes with groundwater discharge, we did not find them in sediments deposited in samples from Strata I and III, even though we sieved our sediments using a 63 μm (230 US Standard) sieve to separate the sand fraction from the finer sediments. Based on the faunal association identified in ORBDL, it is likely that seasonal flooding introduced detrital sediments to the area during the rainy season(s). The system apparently remained flooded during episodes of groundwater input, as suggested by the alternating grayish/whitish laminae characterizing Stratum III, but lake-level declined briefly during deposition of Stratum II.
The ORBD shows some similarity to the paleowetlands of the Okavango Delta in South Africa. For example, Stratum IV was deposited in paleowetlands, the deposits of which now are flanked by topographically inverted paleochannels; the wetlands were seasonally flooded, as were those described by McCarthy and Ellery (Reference McCarthy and Ellery1998). However, Strata I and III contain a lacustrine fauna that thrived in a permanent lake for, at least, 200 years. While the Okavango Delta created a mosaic of wetland habitats in different successional stages due to the constant change in primary channels, the paleowetlands of ORBD show no significant variation in faunal composition within each stratum (Figs. 3, 4).
The PHT across North America marks a change in human land-use, shifting from a wide-ranging, hunting-based subsistence economy to a more local and plant-oriented focus. There is no direct evidence of human occupation of the study area in Biofacies 1–3, but restricted anthropogenic use of peripheral areas towards the south, or proximal ORBD (where the elevation was higher), is possible. The human occupants of the Wishbone site in the GSLD arrived at ca. 12,300 cal yr BP between deposition of Biofacies 3 and the beginning of deposition of Biofacies 4. At the Wishbone site, a waterfowl-associated cooking feature and Haskett projectile points demonstrate that these hunting-and-gathering people moved and camped along the intervening dry landforms between wetland channels early in the Stratum IV depositional episode. Deposition continued as the ORBD endured through the early Holocene until the wetlands gradually desiccated and disappeared (Duke, Reference Duke2011; Madsen et al., Reference Madsen, Schmitt and Page2015b).
CONCLUSIONS
Sediments in the ORBD contain a rich and diverse fauna that shows the composition and changing environmental conditions in the southern GSLD following recession of Lake Bonneville. We found evidence of a large body of cold, freshwater in the GSLD beginning prior to the YDC and persisting into the YDC—a time during which the climate was relatively dry but also conducive to low evaporation rates. Our hypothesis is that Strata I–III represent facies of what we call the ORBDL, which fluctuated in depth for several hundred years and contained a lacustrine fauna. Humans took advantage of a vast and verdant freshwater marshland associated with river flow and groundwater discharge in the southern GSLD forming the ORBDL and expanded into the vast ORBD distributary network by the early Holocene. The mollusk data track well with the multiple lines of paleoenvironmental evidence showing a transition to warmer conditions by the early Holocene, leading to the ORBD's ultimate desiccation by, or intermittently within, the middle Holocene.
The archaeological record of the ORBD provides a trajectory of change through time that corresponds well with the paleoenvironmental data. The earliest observable occupations of the Bonneville basin begin ca. 13,000 years ago, and developing marshlands associated with the ORBDL and feeding distributaries were likely the key draw. In our study area, the ORBDL receded and was replaced by meandering channels of the ORBD by the mid-YCD. Warmer conditions in Biofacies 4 are apparent at this time, and people were able to access this area on dry streamside landforms, as evinced at the Wishbone site, ca. 12,300 cal yr BP. Haskett projectile points indicative of this time are common to the Wishbone site vicinity, but are uncommon across the greater ORBD (Duke Reference Duke2011, Reference Duke2015; Beck and Jones, Reference Beck, Jones, Madsen, Schmitt and Page2015), suggesting that the human population in the terminal Pleistocene was small. Later, Western Stemmed styles and a transition to Pinto attest to extensive human emphasis on the ORBD during the early Holocene. We know this from the chronology of ORBD channels provided by the various archaeological and paleoenvironmental work of others (Duke, Reference Duke2011; Madsen et al., Reference Madsen, Schmitt and Page2015b), but we do not have data representing this time in our study. Our Biofacies 5 demonstrates furthering warming conditions by ca. 10,200 cal yr BP during a period of the late early Holocene that largely post-dates the era of intensive use. We took a bookend approach to examining the paleoenvironment in the current study, and future work should sample black mats dating between ca. 12,000–10,200 to clarify the environment during this interval, especially to determine the transition between biofacies 4 and 5 (e.g., whether it was gradual or precipitous), or if there are other distinguishable biofacies; there are many channels of known age to choose from to address these issues. By Biofacies 6 times, representing the early part of the middle Holocene, the ORBD was largely desiccated with a minor area of persistent or intermittent groundwater in its eastern region near the base of the Cedar Mountains. Whatever water resources remained were apparently insufficient to draw frequent visits from the people of the Bonneville basin.
Supplementary Material
The supplementary material for this article can be found at https://doi.org/10.1017/qua.2021.49
Acknowledgments
The authors are grateful to Jaynie Hirschi and Anya Kitterman, archaeologists from the U.S. Air Force, for their support during various episodes of this investigation. This study is approved for distribution by the U.S. Air Force, Public Affairs Case No. 75ABW-2021-0021. We are grateful to our reviewers Christopher Bradbury and Jay Quade for their profound contributions to improve our manuscript.