INTRODUCTION
The climatic and biotic events of the 700-ka-long interval between the end of the Olduvai subchron (1.778 Ma) and the beginning of the Jaramillo subchron (1.072 Ma) are not known in detail, despite their considerable interest. From the climatic point of view, this time interval was characterized by increasingly cold glacial periods and by the beginning of the so-called early–middle Pleistocene transition around 1.4 Ma (Head and Gibbard, Reference Head and Gibbard2015), with a long-term average global ice-volume increase starting at 1.25 Ma (Clark et al., Reference Clark, Archer, Pollard, Blum, Rial, Brovkin, Mix, Pisias and Roy2006). This transition represents a major reorganisation in Earth’s climate system during which the frequency of glacial-interglacial cycles shifted from a dominant cyclicity of ~41 ka to ~100 ka. The increasing strength and duration of glacial stages affected atmospheric and oceanic circulation (Lawrence et al., Reference Lawrence, Sosdian, White and Rosenthal2010; Head and Gibbard, Reference Head and Gibbard2015), represented a series of ecological filters that exerted great influence on dispersal and dispersion of mammalian species across and between continents (Magri and Palombo, Reference Magri and Palombo2013; Markova and Vislobokova, Reference Markova and Vislobokova2016; Palombo, Reference Palombo2017), caused the diffusion of steppe vegetation over large areas of Europe (Bertini, Reference Bertini2010; Combourieu-Nebout et al., Reference Combourieu-Nebout, Bertini, Russo-Ermolli, Peyron, Klotz, Montade and Fauquette2015), and determined the regional extinctions of many plant taxa (Postigo-Mijarra et al., Reference Postigo-Mijarra, Morla, Barrón, Morales-Molino and García2010; Magri et al., Reference Magri, Di Rita, Aranbarri, Fletcher and González-Sampériz2017).
The late early Pleistocene also represents a critical phase in the evolution and dispersal of hominins (Head and Gibbard, Reference Head and Gibbard2015). By ~1.8 Ma, hominins were present in Georgia (Ferring et al., Reference Ferring, Oms, Agustí, Berna, Nioradze, Shelia, Tappen, Vekua, Zhvania and Lordkipanidze2011 and references therein). At ~1.5 Ma, hominin teeth and artefacts were found in Israel (Belmaker et al., Reference Belmaker, Tchernov, Condemi and Bar-Yosef2002). In Europe, the first hominin occupations are documented between 1.6 and 1.2 Ma (Carbonell et al., Reference Carbonell, Bermúdez de Castro, Parés, Pérez-González, Cuenca-Bescós, Ollé and Mosquera2008; Arzarello et al., Reference Arzarello, Marcolini, Pavia, Pavia, Petronio, Petrucci, Rook and Sardella2009; Toro-Moyano et al., Reference Toro-Moyano, Martínez-Navarro, Agustí, Souday, Bermúdez de Castro, Martinón-Torres and Fajardo2013). However, hominin sites are few, evidence is scarce, and the chronology is often controversial (Muttoni et al., Reference Muttoni, Scardia and Kent2013). Sedimentary deposits of late early Pleistocene age are generally dated by magnetostratigraphic studies, although in many cases there is ambiguity because the normal polarity can represent either the Brunhes or the Jaramillo normal polarity (sub)chron. In any case, considering that the Cobb Mountain normal polarity subchron is too short to be confidently documented in discontinuous continental sections, the chronology of the events between the end of the Olduvai (1.778 Ma) and the beginning of the Jaramillo subchron (1.072 Ma) remains rather vague. Additional chronological control may be provided by 40Ar/39Ar dating, when unaltered volcanic layers are embedded in the stratigraphic sequence, or by electron spin resonance (ESR) and cosmogenic burial or exposure dating techniques, which, however, are very dependent on pre- and postburial history of the sediments (Muttoni et al., Reference Muttoni, Scardia and Kent2018).
The available biostratigraphic information between the end of the Olduvai subchron and the beginning of the Jaramillo subchron is generally rather fragmentary and with only a regional significance. In central Italy, several faunal and/or floristic data are usually placed in the middle–late Matuyama interval, between about 1.7 and 1.2 Ma (Bertini, Reference Bertini2010; Magri et al., Reference Magri, Di Rita and Palombo2010, Reference Magri, Di Rita, Aranbarri, Fletcher and González-Sampériz2017; Corrado and Magri, Reference Corrado and Magri2011; Magri and Palombo, Reference Magri and Palombo2013; Martinetto et al., Reference Martinetto, Bertini, Basilici, Baldanza, Bizzarri, Cherin, Gentili and Pontini2014; Bertini et al., Reference Bertini, Toti, Marino and Ciaranfi2015; Martinetto, Reference Martinetto2015). This time interval was formerly associated with a succession of mammal records, referred to as Late Villafranchian Tasso, Farneta, and Pirro Faunal Units, respectively (Gliozzi et al., Reference Gliozzi, Abbazzi, Argenti, Azzaroli, Caloi, Capasso-Barbato and Di Stefano1997; Petronio et al., 2000–Reference Petronio, Argenti, Caloi, Esu, Girotti and Sardella2002; Argenti, Reference Argenti2004). These Faunal Units often lack a definitive time calibration but have regionally formed a reference scheme that is improving as new data are collected.
Considering the interest, the unknowns, and the difficulties of reconstructing the environmental changes of this time interval, the study of continental sedimentary deposits is challenging and always worth being pursued when thick sedimentary deposits are available and can be studied in a multidisciplinary way.
Through the Italian peninsula, at the western limb of the emerging Mesozoic folded nappes (Martini and Sagri, Reference Martini and Sagri1993), extensional basins formed from the Miocene onwards and gathered marine and/or continental deposits several hundreds of meters thick. This series of basins closely parallel to the Apennine Chain (north-northwest to south-southeast) records an almost continuous magnetostratigraphic signal, as well as a succession of palaeontological, biostratigraphic, and biochronological events. The oldest sediments (∼3.3 Ma) crop out in the Upper Valdarno Basin, while the oldest record inside the Tiberino Basin is placed between 3.3–3.1 (eastern branch) and 2.8 Ma (western branch). These deposits include the uppermost Gilbert and the Gauss magnetochrons (Abbazzi et al., Reference Abbazzi, Albianelli, Ambrosetti, Argenti, Basilici, Bertini, Gentili, Masini, Napoleone and Pontini1997; Albianelli et al., Reference Albianelli, Azzaroli, Bertini, Ficcarelli, Napoleone and Torre1997, Reference Albianelli, Magi, Mazzini and Napoleone2002; Napoleone et al., Reference Napoleone, Albianelli, Azzaroli, Bertini, Magi and Mazzini2003, Reference Napoleone, Albianelli and Fischer2004). The youngest sediments barely reached the magnetochron of Jaramillo, in a tiny interval of the reversal couplets at nearly 1.05 and 0.95 Ma (Ficcarelli et al., Reference Ficcarelli, Abbazzi, Albianelli, Bertini, Coltorti, Magnatti and Masini1997; Coltorti et al., Reference Coltorti, Albianelli, Bertini, Ficcarelli, Napoleone and Torre1998).
In this scenario, the case study from the eastern Tiberino Basin (Arquata quarry section, Fig. 1) provides new multidisciplinary palaeontological, palaeoecological, climatic, and palaeoenvironmental data, documenting the late early Pleistocene evolution.

Figure 1 (colour online) (A) Geographic localization and simplified geologic scheme for the study area. (B) Flight view and schematic geologic map for the Arquata quarry, as it appeared in 2003. FBM, Fornaci Briziarelli Marsciano brick factory.
This work proposes an integrated ecological and stratigraphic study through the middle and late Matuyama interval, enriching the palaeoenvironmental framework of the intermountain basins in central Italy and providing new insights on the climatic changes towards the early–middle Pleistocene boundary that not only are important at a regional scale, but may also be relevant when interpreting coeval southern European records.
GEOLOGIC SETTINGS
The eastern branch of the Tiberino Basin (named Valle Umbra) is a Plio-Pleistocene intermountain depression placed in the eastern part of the Umbria Region (central Italy) (Fig. 1). The structural assessment lies within a tectonic depression bordered by normal faults: the master-fault system, mainly dipping southwestwards and associated to the antithetic one, produced a half-graben structure with displacements of several hundreds of meters (Barchi et al., Reference Barchi, Brozzetti and Lavecchia1991). Such as for other northern Apennine extensional basins, the Valle Umbra underwent several deformation phases from the early Pleistocene onwards (Gregori and Cattuto, Reference Gregori and Cattuto1986; Barchi et al., Reference Barchi, Brozzetti and Lavecchia1991; Martini and Sagri, Reference Martini and Sagri1993; Bonini, Reference Bonini1998; Bucci et al., Reference Bucci, Mirabella, Santangelo, Cardinali and Guzzetti2016). From the Pliocene to present day, this part of the Tiberino basin followed a peculiar sedimentary evolution, which significantly differed from the evolution of the southwestern and northern areas (Ambrosetti et al., Reference Ambrosetti, Basilici, Capasso-Barbato, Carboni, Di Stefano, Esu, Gliozzi, Petronio, Sardella and Squazzini1995; Coltorti and Pieruccini, Reference Coltorti and Pieruccini1997; Melelli et al., Reference Melelli, Barchi, Brozzetti, Lupattelli, Mirabella, Pazzaglia, Pucci and Saccucci2010; Martinetto et al., Reference Martinetto, Bertini, Basilici, Baldanza, Bizzarri, Cherin, Gentili and Pontini2014). The area evolved through time, still maintaining its organization as an endorheic basin until historical time (Colacicchi and Bizzarri, Reference Colacicchi and Bizzarri2008; Bizzarri et al., Reference Bizzarri, Albianelli, Argenti, Baldanza, Colacicchi and Napoleone2011). Contrary to the Valtiberina (Tiber Valley), where several exposures are available, the Valle Umbra is fairly poor in outcrops, not allowing an appropriate recognition of its most recent geologic history. For the southern part (Spoleto area), Coltorti and Pieruccini (Reference Coltorti and Pieruccini1997) propose a local succession, characterized by two main depositional phases, referable to Pliocene and Pleistocene, respectively, and separated by a “tectonic-sedimentary discontinuity.” Both sedimentary cycles are characterized by fluvial-lacustrine and alluvial fan-delta facies, with dominance of lignite-bearing clay deposits, alternating with thin sandy levels (lower cycle) and with coarser sand and fine-grained conglomerates (upper cycle). Older deposits, outcropping near Morgnano (Spoleto area), document a rich mammal fauna dated to about 3.3–3.1 Ma (Triversa faunal unit: Petronio et al., 2000–Reference Petronio, Argenti, Caloi, Esu, Girotti and Sardella2002; Argenti, Reference Argenti2004). The younger sedimentary phase, on the contrary, is represented by thick alluvial-fan conglomerates deposited in the southern valley by streams draining the flanks of the mountains on both sides of the valley (Coltorti and Pieruccini, Reference Coltorti and Pieruccini1997). The main available succession of sediments in the northern part of the basin, representative of the latest sedimentary evolution of the basin, is exposed inside the Arquata quarry, near Bevagna (Fig. 1), where a nearly 70-m-thick stratigraphic profile was measured (Figs. 2 and 3). The deposits are mainly clays and sands with lignite horizons. The beds dip 10°–12° southwestwards and are cut in the upper profile by a well-recognizable southwards dipping extensional fault. Some minor surfaces may be locally recognized, either generated by tectonics or sin-depositional collapses, each less than 1 m in displacement. Throughout the stratigraphic succession, three main facies associations, attributed to shallow-water lake, alluvial plain with distal fluvial supplies, and marsh/pond, respectively, have been recognized. They correspond to lacustrine (unit 1) and fluvial/alluvial (unit 2) depositional environments (Figs. 2 and 3, Table 1; Bizzarri et al., Reference Bizzarri, Albianelli, Argenti, Baldanza, Colacicchi and Napoleone2011).
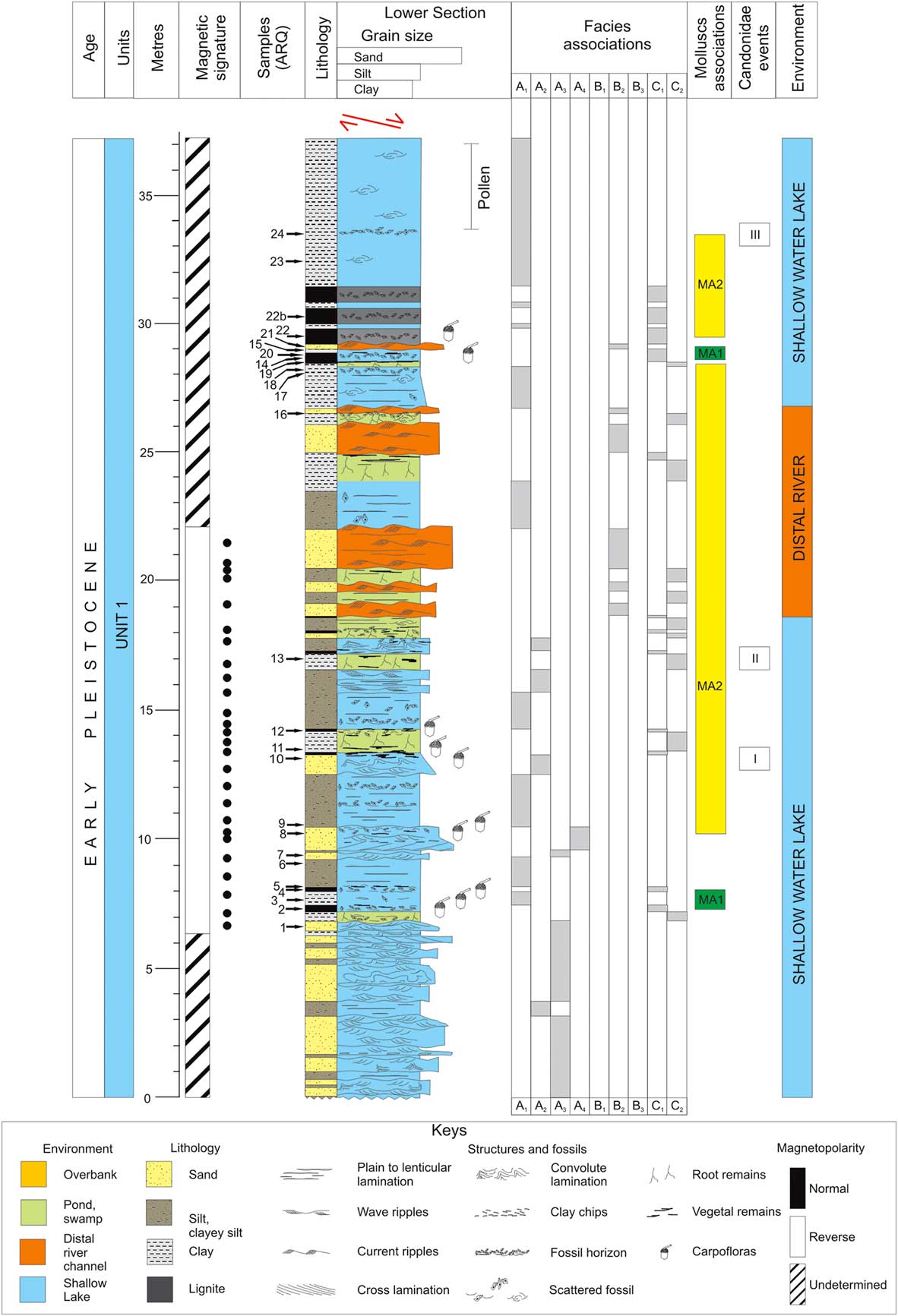
Figure 2 (colour online) Sedimentologic/stratigraphic log for the Arquata quarry (lower section).
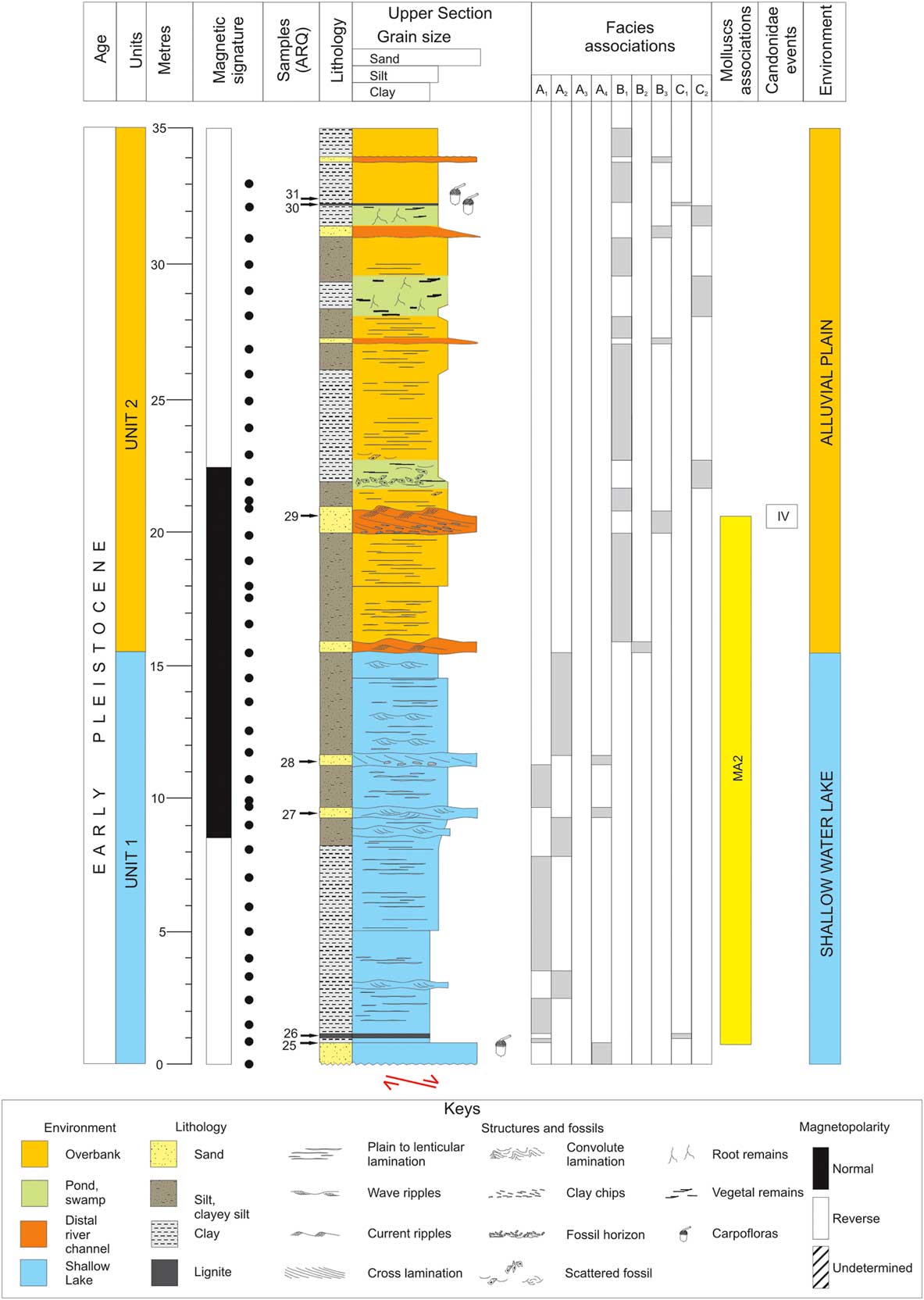
Figure 3 (colour online) Sedimentologic/stratigraphic log for the Arquata quarry (upper section).
Table 1 Synthesis of sedimentologic features, macroscopic fossil distribution, and palaeoenvironmental attribution of facies associations recognized in the Arquata quarry deposits (partly modified after Bizzarri et al., Reference Bizzarri, Albianelli, Argenti, Baldanza, Colacicchi and Napoleone2011). A, wave amplitude; Ac, clay activity; C, clay; Sa, sand; Si, silt; γs, weight density; λ, wave length.
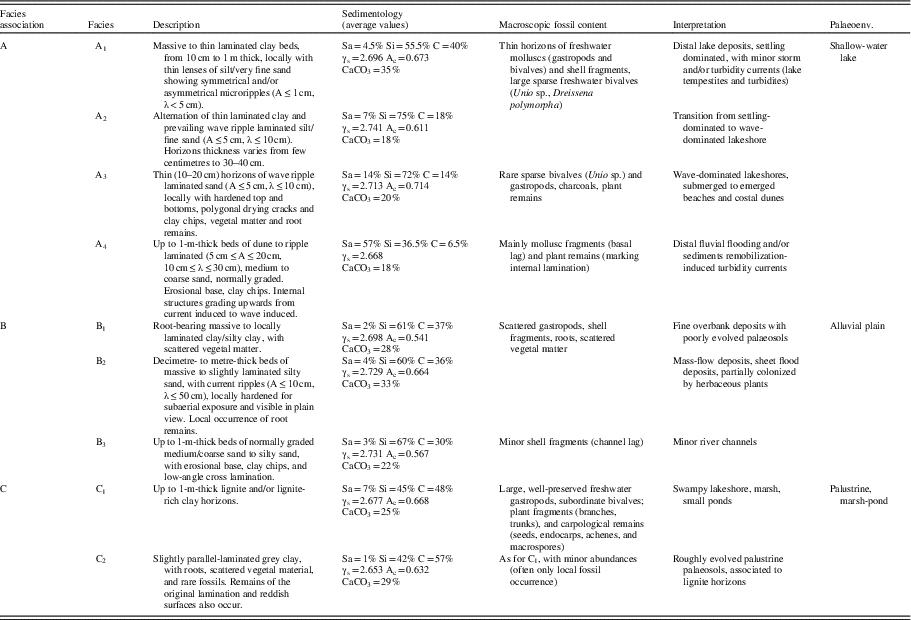
As reported in Bizzarri et al. (Reference Bizzarri, Albianelli, Argenti, Baldanza, Colacicchi and Napoleone2011), sampling for magnetic measurements was carried out preferably in clayey beds at an average spacing of 1 m (Figs. 2 and 3), similarly to previously investigated intermountain basins (Nakagawa et al., Reference Nakagawa, Niitsuma, Takayama, Matoba, Oda, Tokunaga, Kitazato, Sakai and Koizumi1997; Pontini et al., Reference Pontini, Albianelli, Basilici, Bertini and Napoleone2002; Albianelli et al., Reference Albianelli, Cantalamessa, Didaskalou, Micarelli, Napoleone and Potetti2003; Napoleone et al., Reference Napoleone, Albianelli, Azzaroli, Bertini, Magi and Mazzini2003, Reference Napoleone, Albianelli and Fischer2004). The original position of the 55 analysed samples is reported in Figures 2 and 3. All analyses were performed in the ETH Magnetic Laboratory at Zurich (where all the lacustrine sediments from central Italy studied in last two decades have been measured), following the standard laboratory procedures summarized by Napoleone et al. (Reference Napoleone, Albianelli, Azzaroli, Bertini, Magi and Mazzini2003) and Bizzarri et al. (Reference Bizzarri, Albianelli, Argenti, Baldanza, Colacicchi and Napoleone2011).
The analysed levels yielded three intervals of polarity directions, two of them being reversed (R1 and R2) and bracketing a normally magnetized zone (N1), within which the polarity change occurs in an evident continuity of the lithostratigraphic profile (Figs. 2 and 3). These intervals were interpreted as the late Matuyama and the Jaramillo subchrons (Bizzarri et al., Reference Bizzarri, Albianelli, Argenti, Baldanza, Colacicchi and Napoleone2011): such interpretation, as well alternative hypotheses, is here discussed in the context of integrated stratigraphy.
MATERIALS AND METHODS
The results presented herein are derived from data collected through time, often following advancing excavations, and several campaigns were lead on between 2003 and 2012. The former sedimentologic description, achieved directly in the field and reported in Bizzarri et al. (Reference Bizzarri, Albianelli, Argenti, Baldanza, Colacicchi and Napoleone2011), was here reconsidered and integrated with grain-size analysis, weight density, determination of Atterberg limits and clay activity, and CaCO3 content (Dietrich-Fruhling method), accomplished on 24 samples opportunely collected throughout the section. All analyses were performed at the Applied Geology Laboratory (Department of Physics and Geology, Perugia University). Accordingly, stratigraphic implications of palaeomagnetic data reported in Bizzarri et al. (Reference Bizzarri, Albianelli, Argenti, Baldanza, Colacicchi and Napoleone2011) are here reconsidered.
A total of 22 samples for pollen analysis were taken at 20 cm intervals from a 4.4 m-thick outcrop of massive lacustrine clay deposit in the intermediate section (Fig. 2). They contained enough pollen for counting, while sparse samples along the sedimentary succession in the Arquata quarry were sterile. For each sample, a known amount of dry sediment (1 g on average) was chemically processed as follows: removal of calcium carbonate (HCl 37%), silica (cold HF 40% for 12 h), and humic acids (boiling in NaOH 10%); mounting in glycerine. A known amount of Lycopodium spores was added to obtain estimates of pollen concentration per gram dry sediment. Pollen grains were identified according to Beug (Reference Beug2004), Reille (Reference Reille1992, Reference Reille1995, Reference Reille1998), and the reference collection at the Laboratory of Palaeobotany and Palynology of Sapienza University of Rome.
A total of 35 pollen types and one spore type were identified. Pollen of Taxodium type may include Taxodium and Glyptostrobus, similarly to other Italian records (Bertini, Reference Bertini2010). Deciduous Quercus includes also Quercus cerris type. For each sample, at least 200 terrestrial pollen grains were counted. The computer program Psimpoll 4.27 (Bennett, Reference Bennett2009) was used to plot the pollen diagram.
Palaeocarpological analyses concerned all the layers rich in compressed plant remains in the Arquata quarry section. A sum of 13 sediment samples (volume ∼0.5 dm3) has been analysed from bottom to top of the composite section. The palaeocarpological assemblages have been gathered by processing dry sediment samples with 3–5% hydrogen peroxide. After complete reaction, the floating fraction was sieved separately (final mesh size: 0.3 mm) from the sunken material (final mesh size: 1.5 mm). Finally, fruits, seeds, and related parts were picked up from the residue of both fractions and identified with the procedure described in Martinetto (Reference Martinetto2015).
For molluscs and ostracod analysis, 31 samples have been collected throughout the section, into clay, sand, lignite-bearing clay, and sandy clay deposits; about 1200 g per sample of sediments has been washed with hydrogen peroxide and water, and the residue was sieved with a 63 μm mesh net and dried in an oven at a constant temperature of 70°C. The entire residue was analysed under a stereomicroscope (model Nissho Optical TZ-240) for the picking of malacofauna, ostracods, and Charophyceae. A quantitative analysis of malacological assemblages was carried out, and distribution charts have been compiled for Prosobranchia and Bivalvia. A semiquantitative analysis on ostracods was also carried out, whereas the total number of specimens is reported for Charophyceae.
RESULTS
Sedimentology and depositional architecture
On the basis of the collected sedimentologic data, three main facies associations are described (Table 1), with their internal facies organization.
(A) Shallow-lake facies association: The association groups facies of shallow-water lacustrine environment and includes massive to thin laminated, fossil-bearing clay beds with thin fine-sand lenses showing wave and current ripples (facies A1); alternations of thin laminated clay and prevailing ripple laminated silt/fine sand (facies A2); wave ripple laminated sand, locally with hardened top and bottoms and polygonal drying cracks (lake beaches) and containing clay chips, vegetal matter, and root remains (facies A3); dune to ripple laminated sand resulting from minor turbidites (facies A4), attributable to distal fluvial flooding and/or to sediment remobilization. The fossil content is both represented by scattered specimens and/or thin shell beds. Banks of vegetal matter occur as thin lignite levels, although charcoals are widely found, either dispersed in the sediment or commonly marking parallel and/or cross lamination.
(B) Alluvial plain facies association: The main facies (B1) is represented by laminated silty clay, deposited in an alluvial plain environment, locally associated with root-bearing massive to laminated clay, interpreted as poorly drained palaeosols. Decimetre- to metre-thick levels of massive to slightly laminated silty sand (facies B2), locally associated with current ripple and/or cross laminated sands (facies B3), both resulting from distal fluvial influx (river flooding), cyclically recur.
(C) Palustrine/marsh-pond facies association: It is documented by less than 1-m-thick lignite horizons (facies C1) and massive, grey clayey palustrine palaeosols (facies C2) with roots, scattered vegetal material, and fossils. Remains of the original lamination and reddish surfaces also occur. Facies C1 and C2 are constantly associated, leading to poorly oxygenated and strongly reducing environments, such as swampy lake flanks and small ponds and marshes within the alluvial plain.
The lower two-thirds of the succession (unit 1: Fig. 2) are dominated by facies A1–A4, while alluvial plain deposits (facies B1–B3) prevail in the upper portion (unit 2: Fig. 2). Facies of association C occur in both units.
Postdepositional structures (convolute lamination, microfaulting, etc.) are recognized almost in all the facies.
The geotechnical analysis reveals no significant variations along the section, either for weight density or clay activity (Table 1), thus indicating a mineralogical/petrologic homogeneity. Finer deposits of associations A and B are prevalently silty, with variable percentages of sand and clay, whereas those of association C show a higher clay fraction (Table 1). The deposits are moderately enriched in calcium carbonate throughout the section, with average values of about 20–30% (Table 1).
Palaeofloristic record
Pollen
The pollen record obtained from the 4.4-m-thick section (Fig. 4) shows a clear dominance of arboreal taxa (80–95%) with respect to herbaceous taxa. In particular, there was a forest characterized by abundant Pinus, Cedrus, and Abies (>20%), accompanied by Tsuga and Picea (~5%), and discontinuous presence of the Cathaya type and Taxodium type. Among deciduous trees, only Carya displays high values (>20%), whereas Ulmus, Tilia, Zelkova, Liquidambar, and evergreen and deciduous Quercus are represented by percentage values not exceeding 10%. Considering that bisaccate pollen grains may have a distant origin, a mixed forest with conifers, deciduous elements, and a minor presence of broad-leaved evergreens might have been the local vegetation in the neighbourhood of the lake. Besides, two taxa especially useful for the chronological framework have been found: Sciadopitys and Liquidambar. Among herbs, Artemisia, Asteroideae, and Chenopodiaceae show values of 1–5%. Towards the top of the record, a significant presence of Olea and Ericaceae highlights increasingly Mediterranean conditions. Altogether, this pollen assemblage points to a temperate-wet interglacial period.

Figure 4 Pollen percentage diagram from Arquata quarry.
Carpological remains
The fruit and seed record originates from a few layers scattered from the bottom to the top of the sections, but only the basal samples, in the lower section, provided sufficiently diverse assemblages (Table 2). The taxonomic data preliminarily reported by Bizzarri et al. (Reference Bizzarri, Albianelli, Argenti, Baldanza, Colacicchi and Napoleone2011) are here completely revised. There is a clear dominance of herbaceous freshwater macrophytes (almost 100%), and the arboreal taxa are represented by a single fruit of Carpinus betulus in layer ARQ8. This situation is clearly the result of a very narrow range of dispersion of the fruits and seeds, which are a production of the local plant assemblages (see Teodoridis et al., Reference Teodoridis, Bruch, Vassio, Martinetto, Kvaček and Stuchlik2017), whereas the surrounding forest environments (documented by the pollen record) did not contribute to the carpoflora.
Table 2 Distribution of ostracods, mollusc assemblages, charophytes, and palaeocarpological remains through the facies described in the Arquata (ARQ) quarry section.
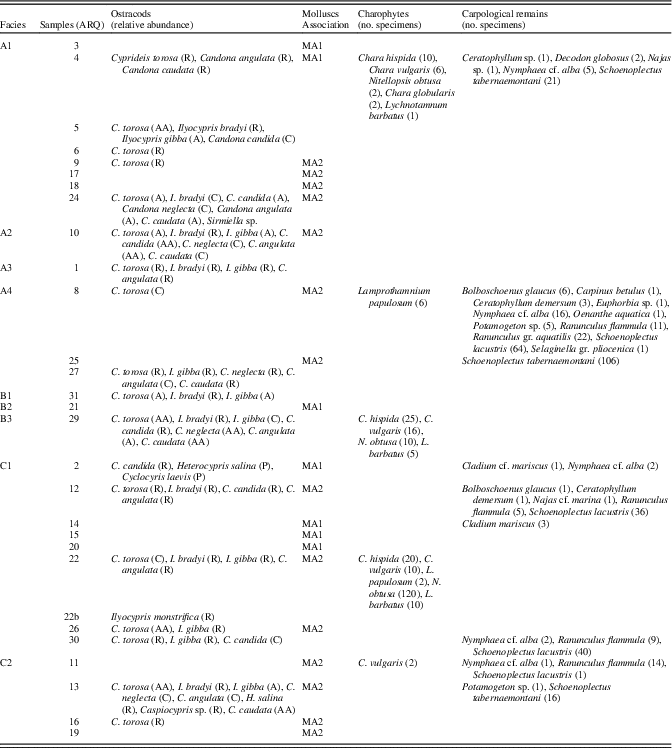
The carpological analysis is mainly relevant for the species-level identification of several plant remains (Fig. 5). This permitted detection of a prevalence of still-living taxa, as only Decodon globosus and Selaginella gr. pliocenica are extinct. The last record of D. globosus was in the mid-Calabrian PF2 layer of Pietrafitta (Martinetto et al., Reference Martinetto, Bertini, Basilici, Baldanza, Bizzarri, Cherin, Gentili and Pontini2014), and the new Arquata quarry record may be the youngest in Italy.

Figure 5 (colour online) (A–N) Carpological remains from Arquata quarry. (A) Bolboschoenus glaucus, achene assemblage. (B) Schoenoplectus lacustris, achene assemblage. (C) As in panel B, fruits assigned to Schoenoplectus lacustris, but with a morphotype considered typical of the fossil-species S. lacustroides. Velichkievich and Zastawniak (Reference Velichkievich and Zastawniak2003) already remarked the presence of a broad fruit variation in S. lacustris and a probably gradual chronological transition between S. lacustroides and this living species. (D) Schoenoplectus tabernaemontani, achene assemblage. (E) Fruit assemblage probably representing a bird coprolite with Schoenoplectus sp. and Oenathe aquatica. (F) Selaginella gr. pliocenica, megaspore. (G) Ceratophyllum demersum, fruit. (H, I) Nymphaea cf. N. alba, seeds. (J) Bolboschoenus glaucus, achene. (K) Schoenoplectus lacustris, achene. (L) Schoenoplectus tabernaemontani, achene. (M) Potamogeton sp., endocarp. (N–Q) Charophyte gyrogonites. (N) Lamprothamnium papulosum. (O) Chara hispida. (P, Q) Nitellopsis obtusa.
The abundant fruits of Schoenoplectus document the occurrence of two distinct, but strictly related taxa (Schoenoplectus lacustris and S. tabernaemontani) in different layers, starting from the bottom ones, thus indicating that their phylogenetic divergence preceded the time of sedimentation of the basal layers of the Arquata quarry section. Unfortunately, such divergence has not been dated with molecular clocks yet (Tena-Flores et al., Reference Tena-Flores, González-Elizondo, Herrera-Arrieta, Almaraz-Abarca, Mayek-Pérez and Vanzela2014). Furthermore, our sample (ARQ8, Table 2), assigned to Schoenoplectus lacustris, contains a few fruits with a morphotype considered typical of the fossil-species S. lacustroides, described by Velichkievich and Zastawniak (Reference Velichkievich and Zastawniak2003). These authors already remarked the presence of a broad fruit variation and a probably gradual chronological transition between this “species” and the modern Schoenoplectus lacustris. We can argue that the Schoenoplectus lacustris lineage acquired the modern fruit morphological variation during the early Pleistocene, starting from more elongated and strongly carinate fruit morphology (S. lacustroides by Velichkievich and Zastawniak, Reference Velichkievich and Zastawniak2003). In this sense, the occurrence of S. lacustroides–like fruits mixed up with modern-like S. lacustris fruits in the bottom layers of the Arquata section (ARQ8) seems to indicate that the morphological evolution in this lineage was still ongoing.
Other cases of morphological transitions, possibly attributable to microevolutionary processes, concern Cladium paleomariscus/C. mariscus and Ranunculus pseudoflammula/R. flammula. The available fossil record gathered into the CENOFITA database (Martinetto, Reference Martinetto2015) suggests that these occurred in the late Piacenzian–early Calabrian interval. Another interesting and newly reported occurrence concerns Bolboschoenus: six fruits found in the sample ARQ8 have clear diagnostic characters pointing to Bolboschoenus glaucus, one of the five European living species of Bolboschoenus (Wollstonecroft et al., Reference Wollstonecroft, Hroudová, Hillman and Fuller2011). Three fruits were measured (2.5 × 1.8, 2.4 × 1.8, and 2.2 × 1.8 mm), while two were too much deformed. Even if the measures stay within the upper part of the variation range of B. glaucus, the mummified specimens from Arquata certainly had larger dimensions because we measured shrinkage of 80–90% from the wet to dry sample in such conditions. Therefore, the Arquata section population represents an ancient population of B. glaucus with slightly larger fruits in comparison with the modern ones. It is interesting to consider that all the other European species of Bolboschoenus have larger, ~3- to 4-mm-long fruits (Wollstonecroft et al., Reference Wollstonecroft, Hroudová, Hillman and Fuller2011), so that the Arquata plant could assume an intermediate phylogenetic position between the modern B. glaucus and its unknown ancestor. Unfortunately, also in this case the divergence time of B. glaucus from other similar species has not been dated with molecular clocks yet (Wollstonecroft et al., Reference Wollstonecroft, Hroudová, Hillman and Fuller2011).
From the biochronological point of view, the occurrence in the bottom layers of the “young” species Cladium mariscus, Nymphaea cf. alba, Ranunculus flammula, and Schoenoplectus lacustris, combined with the “old” Decodon globosus and Selaginella gr. pliocenica, indicates a Gelasian-Calabrian age on the basis of the records contained in the CENOFITA database (Martinetto, Reference Martinetto2015). Furthermore, the exclusive occurrence at Torre Picchio (Girotti et al., Reference Girotti, Capasso Barbato, Esu, Gliozzi, Kotsakis, Martinetto, Petronio, Sardella and Squazzini2003) of a typical S. lacustroides morphotype suggests that this section, dated to the Gelasian/Calabrian transition, is older than the Arquata one.
From the climatic point of view, the taxa documented by fruits and seeds have broad tolerances, and the most relevant information comes from the occurrence of Nymphaea cf. alba from the bottom to the top of the section.
Charophyte occurrences
Throughout the section, at least five samples (Table 2) supported rich and diversified charophyte assemblages characterized by well-preserved gyrogonites, often with oospores kept inside, which testify of cyclically recurrent ideal ecological conditions for the development of submerged macroalgae (Soulié-Märsche and Garcia, Reference Soulié-Märsche and Garcia2015). The species Chara globularis, Chara hispida, Chara vulgaris, Lamprothamniun papulosum, Lychhnothamnus barbatus, and Nitellopsis obtusa were identified, although species diversity and relative abundance of specimens in assemblage vary from each sample to another (Table 2, Fig. 5).
Molluscs
From 31 samples, only 24 were productive for molluscs; the specimens are generally in medium to good preservation state. The recovered taxa (20 species of freshwater gastropods and 3 species of bivalves: Fig. 6) are listed in Table 3 (only complete specimens are considered). According to Ložek (Reference Ložek1964), the species documented throughout the section all belong to the Upper Ecological Group (D), grouping the species of subaerial swamp and freshwater, and in detail to the Ecological Group 10 (aquatic species that can live in different biotopes). The dominant ecological classes (Table 3, Fig. 7) are 10SF (7–100%), 10FQ (3.5–90%), and 10F (4–66%). All the other ecological classes are subordinate. The mollusc ecological constraints are deduced from the present-day habitat of still-living close relatives or from palaeoenvironmental reconstruction of similar fossil assemblages.
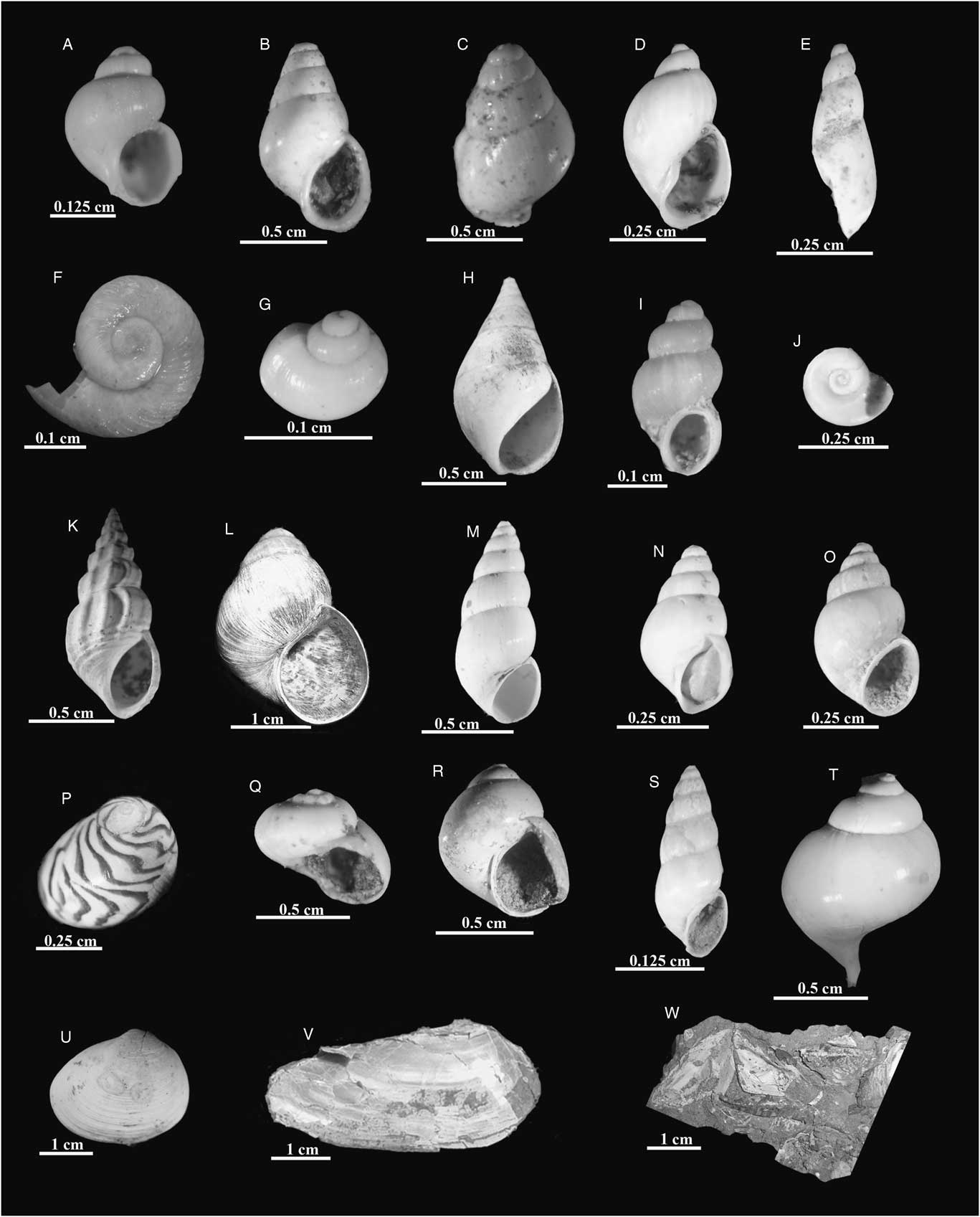
Figure 6 Selected molluscs from Arquata section. (A) Bithynia leachii. (B) Emmericia umbra. (C, D) Radix cf. labiata. (E) Lymnaea stagnalis. (F) Gyraulus crista. (G) Islamia minuta. (H) Melanopsis affinis. (I) Belgrandia sp. (J) Planorbis sp. (K) Melanoides curvicosta. (L) Viviparus belluccii. (M) Prososthenia oblonga. (N) Prososthenia cf. ovata. (O) Tournouerina belnensis. (P) Theodoxus groyanus. (Q) Valvata piscinalis. (R) Stefania bronni. (S) Semisalsa stagnorum. (T) Neumayria priscillae. (U) Pisidium sp. (V) Unio cf. pillai. (W) Dreissena polymorpha.

Figure 7 Percentages of molluscs grouped for ecological classes throughout the Arquata section.
Table 3 Collected mollusc species from the Arquata quarry section (numbers refer to specimens counted in each sample). Ecological classes (according to Ložek, Reference Ložek1964) and facies (see Table 1) are also indicated.

The analysed mollusc assemblages are nearly different for each sample, both for occurring species and the number of specimens. Two main assemblages are identified throughout the section (Tables 2 and 3; Figs. 2, 3, and 7).
The association MA1 is characterized by the dominance of Theodoxus groyanus and Melanopsis affinis (both pertaining to ecological class 10F) with rare Emmericia umbra (10FQ) and Dreissena polymorpha (10F[S]). This association occurred in the lower and middle portion of Arquata quarry composite section (Fig. 7, Tables 3 and 4). It reflects palaeoenvironmental conditions characterized by running clear waters.
Table 4 Ecology of selected species of ostracods, molluscs, and charophytes through the Arquata quarry section.
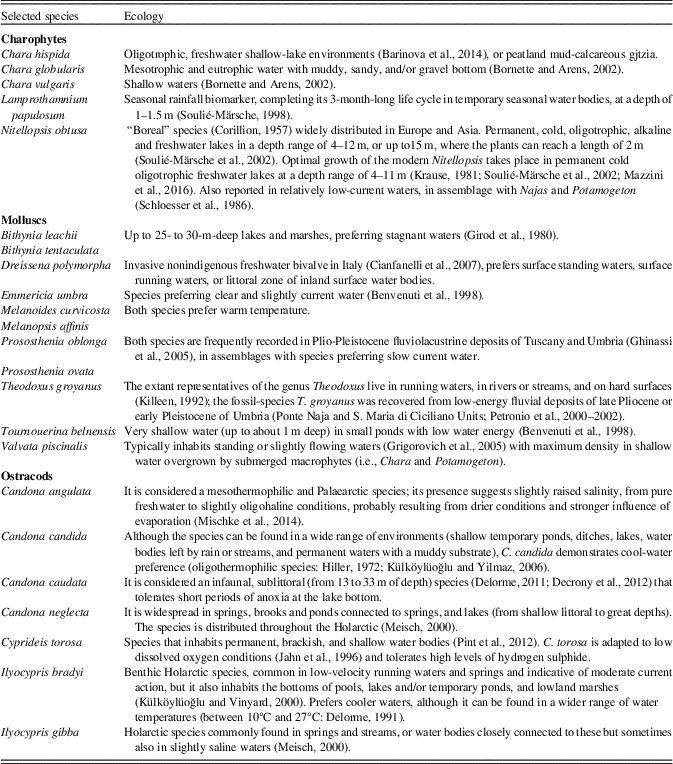
The association MA2 is characterized by the dominance of Valvata piscinalis (10SF), reaching percentages of 79% and 76% (in samples ARQ9 and 12, respectively: Table 3) but always present with different percentages in the recorded assemblages, locally accompanied by Prososthenia oblonga and Prososthenia ovata (both belonging to 10SF), E. umbra (10FQ), Bithynia leachii (10P), and Islamia minuta (10FQ). D. polymorpha too occurs in MA2, but sporadically and with very low percentage in the upper portion. This association (Tables 3 and 4) reveals palaeoenvironmental conditions dominated by standing or slowly running waters, with the sporadic occurrence of stagnant waters with submerged vegetation, where B. leachii developed. It was found in samples from the lower and middle portions of the section and from the upper portion (Fig. 7).
The two associations alternate along the section with prevalence of MA2 (Figs. 2 and 3).
I. minuta occurred in large quantity (1045 specimens) only in sample ARQ10, and it may be connected to a mechanical accumulation phenomenon. On the other hand, its large presence in sample ARQ29 (180 specimens) in assemblage only with rare Semisalsa stagnorum and opercula of Bithynia leachii could be indicative of a normal development of indigenous population.
In the upper portion (Fig. 3), a strong decrease of mollusc taxa is detected. The assemblages are definitely depleted of the markers of both described associations, except P. oblonga, a “survivor” of the MA2 in ARQ24; Tournouerina belnensis, in ARQ26; and V. piscinalis in various samples.
Ostracods
Among the 31 samples analysed for ostracod assemblages, only 19 were productive (Table 2), revealing a singular trend in both species diversity and relative abundances. The recovered specimens belong to the families Candonidae Kaufmann, 1900; Ilyocyprididae Kaufmann, 1900; and Cytherideidae Sars, 1925. The ostracod assemblages generally are poor, and the species diversity variable, with a minimum of one to a maximum of seven species (Table 2). The assemblages are interpreted on the basis of autecological data derived from literature (Table 4).
Minor species (Caspiocypris sp., Cyclocypris laevis, Heterocypris salina, Ilyocypris monstrifica, Sirmiella sp.) are also documented (Table 2).
DISCUSSION
Most of the early Pleistocene reference sections, not only in the Italian record but also at a European scale, lack age calibration (Magri et al., Reference Magri, Di Rita, Aranbarri, Fletcher and González-Sampériz2017; Muttoni et al., Reference Muttoni, Scardia and Kent2018). Besides, key-point data mostly span limited time periods (Fig. 8), and age constraints are often linked to biochronological data (cf. the common use of Faunal Units and Mammal Ages), with the related uncertainty (“local” vs. “global” signal). The only chance to establish reliable stratigraphic schemes and to investigate climatic changes through time is to study new reference sections and add calibrated points to the existing frameworks. Although such schemes initially have only a regional validity, they are crucial in discriminating the geographic component and the local signal from the general trend, and thus may be used to compose a general picture of the succession of biotic and climatic events during the Quaternary (Magri et al., Reference Magri, Di Rita, Aranbarri, Fletcher and González-Sampériz2017).

Figure 8 Stratigraphic setting and schematic pollen biostratigraphy of selected pollen taxa for the Arquata quarry, inside a scheme of selected early Pleistocene Italian pollen records (modified from Magri and Palombo, Reference Magri and Palombo2013; Magri et al., Reference Magri, Di Rita, Aranbarri, Fletcher and González-Sampériz2017).
Age calibration
Multidisciplinary data from the Arquata quarry section assign the deposits a late early Pleistocene age. The biochronological value of the malacofauna and ostracofauna allows referring the Arquata quarry deposits to the Late Villafranchian. Eight species in the assemblages (E. umbra, T. groyanus, M. affinis, D. polymorpha, T. belnensis, Viviparus belluccii, P. oblonga, and Melanoides curvicosta), mostly found in the lower section, were reported from other sites referred up to the Farneta faunal unit (1.5–1.4 Ma). The ostracod Cyprideis torosa, also present in assemblages just from the basal portion samples, has been signalled in the Pietrafitta Basin and referred to the Farneta faunal unit. Regarding the ostracods, the presence of C. torosa and Ilyocypris bradyi was never documented in Italy before the Olivola Faunal Unit (∼1.8 Ma; Gliozzi et al., Reference Gliozzi, Abbazzi, Argenti, Azzaroli, Caloi, Capasso-Barbato and Di Stefano1997). Consequently, the malacological assemblages and the occurrence of C. torosa suggest, for the lower section, an age not older than ∼1.7 Ma. Some taxa, such as V. belluccii, M. curvicosta, and V. interposita, have indeed been considered as typical of the Tasso-Farneta Faunal Unit interval and never documented afterwards (Gliozzi et al., Reference Gliozzi, Abbazzi, Argenti, Azzaroli, Caloi, Capasso-Barbato and Di Stefano1997). Thus, molluscs and ostracods indicate a 1.7–1.4 Ma time range.
A comparison of the studied section with other early Pleistocene pollen sites improves the biostratigraphic framework (Fig. 8). In particular, the presence and abundance of tree taxa, especially those currently absent from the Italian peninsula, have been considered (Magri and Palombo, Reference Magri and Palombo2013; Magri et al., Reference Magri, Di Rita, Aranbarri, Fletcher and González-Sampériz2017). The presence of Sciadopitys clearly points to pre-Jaramillo Calabrian biostratigraphical characters, as it is found with discontinuous appearances only in the Gelasian pollen record of Fosso Bianco (Pontini and Bertini, Reference Pontini and Bertini2000) and in the early Calabrian sites of Camerota (Brenac, Reference Brenac1984) and Pietrafitta (Lona and Bertoldi, Reference Lona and Bertoldi1972), younger than the Olduvai subchron and older than approximately 1.4 Ma. Sciadopitys was absent from the deposits of Madonna della Strada (Magri et al., Reference Magri, Di Rita and Palombo2010), Saticula (Russo Ermolli et al., Reference Russo Ermolli, Sardella, Di Maio, Petronio and Santangelo2010), Colle Curti (Bertini, Reference Bertini2000), and Fontana Ranuccio (Corrado and Magri, Reference Corrado and Magri2011), recording the end of the early Pleistocene. Liquidambar, which is relatively abundant (5–10%) at the Arquata quarry and Pietrafitta, is sporadically found at Colle Curti, during and soon after the Jaramillo subchron, where it has its last appearances in central Italy. Cathaya type is represented by low percentage values not exceeding 1% at the Arquata quarry, similarly to Pietrafitta (Lona and Bertoldi, Reference Lona and Bertoldi1972) and Gubbio (Lona and Ricciardi, Reference Lona and Ricciardi1961) (Fig. 8). It has its last appearances in the European pollen record in the late early Pleistocene site of Fontana Ranuccio (Corrado and Magri, Reference Corrado and Magri2011).
In the Arquata quarry, Tsuga displays values between 2% and 5%, similar to Pietrafitta (Lona and Bertoldi, Reference Lona and Bertoldi1972) and Gubbio (Lona and Ricciardi, Reference Lona and Ricciardi1961), whereas at Madonna della Strada (Magri at al., 2010) and Colle Curti (Bertini, Reference Bertini2000), two sites younger than 1.3 Ma, it shows much higher values (10–30%). At Arquata, Carya shows pollen percentages comparable to Pietrafitta, Madonna della Strada, and Saticula (Fig. 8). Cedrus also shows values comparable to the other early Pleistocene sites from central Italy, although it is not possible to assess if it was locally present because of the difficulty of distinguishing local from long-distance transported pollen grains (Magri, Reference Magri2012).
Considering altogether the abundance of these tree taxa in the Arquata quarry, which are extinct in the modern European flora but still present elsewhere, we can suggest an age for this deposit and contribute to the ongoing discussion about the development of the Tiberino Basin (Martinetto et al., Reference Martinetto, Bertini, Basilici, Baldanza, Bizzarri, Cherin, Gentili and Pontini2014, Reference Martinetto, Momohara, Bizzarri, Baldanza, Delfino, Esu and Sardella2017). The pollen record from Arquata quarry is clearly older than Saticula and Madonna della Strada, dated around 1.3–1.1 Ma, based on the abundance of Sciadopitys and Liquidambar, and on the relatively low frequencies of Tsuga (Fig. 8). On the other hand, we can consider the pollen record younger than the Gelasian for various reasons, including the absence of Engelhardia, found at Camerota until the early Calabrian (Bertini, Reference Bertini2010), the scarcity of Taxodium type and Cathaya type, and the abundance of Carya, a taxon widely diffused in the Calabrian.
The Arquata quarry pollen record is very similar to two other sites from the Umbria region, Gubbio Basin (Lona and Ricciardi, Reference Lona and Ricciardi1961) and Pietrafitta (interval “E” of Lona and Bertoldi, Reference Lona and Bertoldi1972), the latter being considered 1.5–1.3 Ma old on the basis of the vertebrate fauna (Martinetto et al., Reference Martinetto, Bertini, Basilici, Baldanza, Bizzarri, Cherin, Gentili and Pontini2014). In Figure 8, the relative position of the pollen records from Gubbio Basin, Arquata quarry, and Pietrafitta is arbitrary, as no other data, apart from pollen, support this interpretation. The three records might also be more or less coeval.
On the whole, the pollen record suggests an age between 1.6 and 1.3 Ma, and this is in agreement with the information provided by carpological data, based on the concomitant occurrences and microevolutionary processes.
From the biochronological point of view, the occurrence in the Arquata quarry bottom layers of the “young” species Cladium mariscus, Nymphaea cf. alba, Ranunculus flammula, and Schoenoplectus lacustris, combined with the “old” Decodon globosus and Selaginella gr. pliocenica, indicates a Gelasian-Calabrian age on the basis of the records contained in the CENOFITA database (Martinetto, Reference Martinetto2015). Furthermore, the occurrence at Torre Picchio (Girotti et al., Reference Girotti, Capasso Barbato, Esu, Gliozzi, Kotsakis, Martinetto, Petronio, Sardella and Squazzini2003) of a typical S. lacustroides morphotype (probable ancestor of Schoenoplectus lacustris) suggests that this section, dated to the Gelasian/Calabrian transition, is older than the Arquata one.
The detailed investigation of fossil seeds of Nymphaea would be very important because researchers in eastern Europe (Velichkevich and Zastawniak, 2003, Reference Velichkevich and Zastawniak2008) showed that the modern species occur only since the Pleistocene, and particularly N. alba only since the middle Pleistocene (despite several erroneous or doubtful reports from older sediments). The seeds from Arquata section agree with the description of N. alba provided by Velichkevich and Zastawniak (Reference Velichkevich and Zastawniak2008); therefore, they could represent the oldest record of this living species in Europe. Other fossil seeds of Nymphaea reported from Gelasian and Calabrian sediments were not assigned to living species, but rather to fossil species (Martinetto et al., Reference Martinetto, Monegato and Vassio2012, Reference Martinetto, Bertini, Basilici, Baldanza, Bizzarri, Cherin, Gentili and Pontini2014).
A feasible age calibration also derives from the magnetostratigraphic record and its implications (Bizzarri et al., Reference Bizzarri, Albianelli, Argenti, Baldanza, Colacicchi and Napoleone2011). The three detected magnetozones (Figs. 2 and 3) have been correlated to the chrons of the GPTS and calibrated to their absolute age. Nonetheless, because of outcrop conditions, the magnetostratigraphic datum assumes a different relevance in the lowermost and uppermost sections, respectively. According to Bizzarri et al. (Reference Bizzarri, Albianelli, Argenti, Baldanza, Colacicchi and Napoleone2011), no appreciable changes were noticed for the presence of sulphide minerals, which on the contrary did affect some significant intervals in the lignite-bearing levels of the Valdarno with unstable magnetizations (Napoleone et al., Reference Napoleone, Albianelli, Azzaroli, Bertini, Magi and Mazzini2003, Reference Napoleone, Albianelli and Fischer2004). Such behaviour was mainly enhanced by the smooth trend of the alternate-field curves (fig. 6 in Bizzarri et al., Reference Bizzarri, Albianelli, Argenti, Baldanza, Colacicchi and Napoleone2011). The straightforward direction shown by both demagnetization treatments of the palaeomagnetic vector to the characteristic primary magnetization is interpreted as the typical behaviour because of the presence of a single component in the magnetic signature; in fact, the characteristic remnant magnetization (ChRM) was well isolated already at temperatures above 100–200°C or at applied fields above 0.2–0.3 mT (fig. 6 in Bizzarri et al., Reference Bizzarri, Albianelli, Argenti, Baldanza, Colacicchi and Napoleone2011). Thus, we considered magnetostratigraphy a fixed point for this section, and we treated it as a reference datum.
As palaeontological and biochronological data agree with a late early Pleistocene age and restrain the time interval to 1.7–1.4 Ma (Tasso to Farneta Faunal Units, both calibrated to the reversal magnetopolarity interval successive to Olduvai: Gliozzi et al., Reference Gliozzi, Abbazzi, Argenti, Azzaroli, Caloi, Capasso-Barbato and Di Stefano1997), the long interval of reversal polarity (R1, R2) can only be related to the Matuyama Chron.
Thickness and characters of the N1 magnetozone, together with sedimentologic considerations, formerly lead to the attribution to the Jaramillo subchron (Bizzarri et al., Reference Bizzarri, Albianelli, Argenti, Baldanza, Colacicchi and Napoleone2011). In this hypothesis, it lasted from 1.07 to 0.99 Ma.
Alternative interpretations need to be considered, and particularly the correlation of N1 magnetozone with the Cobb Mountain subchron (1.22–1.24 Ma), although this short normal signal inside the Matuyama has yet been rarely recognized in Italy (Scardia et al., Reference Scardia, Muttoni and Sciunnach2006). Moreover, short events are usually clearly identified where a long and continuous magnetic signal is available, such as in marine deposits (Horng et al., Reference Horng, Meng-Yang Lee, Pälike, Wei, Liang, Iizuka and Torii2002), or in continental deposits, where the sedimentation rate is fairly low (Ao et al., Reference Ao, An, Dekkers, Wei, Pei, Zhao and Zhao2012).
The age-versus-thickness model leads to the assignment of 80 ka duration to the 14-m-thick N1 interval (if calibrated to Jaramillo) or 20 ka (in the Cobb Mountain hypothesis). Thus, the time span for the upper section deposits could be roughly 210 ka (between 1.126 and 0.916 Ma) and 50 ka (between 1.253 and 1.203 Ma) in the two cases, respectively. An accumulation rate varying from about 19 m/100 ka to 70 m/100 ka is so calculated, net of unknown compaction. Lower values are largely within the range (20–35 m/100 ka) calculated several times for the Tiberino and the Valdarno basins (Abbazzi et al., Reference Abbazzi, Albianelli, Ambrosetti, Argenti, Basilici, Bertini, Gentili, Masini, Napoleone and Pontini1997; Pontini et al., Reference Pontini, Albianelli, Basilici, Bertini and Napoleone2002; Napoleone et al., Reference Napoleone, Albianelli and Fischer2004; Bizzarri et al., Reference Bizzarri, Albianelli, Argenti, Baldanza, Colacicchi and Napoleone2011). On the other hand, values measured in Holocene palustrine/alluvial deposits inside the Valle Umbra are about 0.8–1.1 mm/yr (Colacicchi and Bizzarri, Reference Colacicchi and Bizzarri2008), strictly comparable with higher values. Moreover, such a high sedimentation rate probably explains the lack of palynological record throughout the section, except for deeper lake deposits in the intermediate part.
Finally, some considerations can be proposed: (1) According to the multidisciplinary data, the age for the lower section should be between 1.4 and 1.3 Ma. The magnetostratigraphic inferences from the upper section do not influence this age attribution. (2) Assuming the same values for the sedimentation rate, the duration of the lower section ranges from 30 ka to 180 ka. Such high (to very high) values of sedimentation rate probably prevent the possibility of recording and/or identifying short duration magnetochrons inside the deposits.
Palaeoenvironmental reconstruction and palaeoclimatic inferences
Malacofaunas and ostracods, as well as charophytes, mark repeated fluctuations in energy, temperature, and chemical composition of water throughout the section. Two main depositional palaeoenvironments are found based on sedimentologic analysis: shallow- to relatively deep-water lake and alluvial plain. This is not unexpected, as these two main conditions alternated throughout the entire evolution of the basin (Coltorti and Pieruccini, Reference Coltorti and Pieruccini1997; Colacicchi and Bizzarri, Reference Colacicchi and Bizzarri2008; Bizzarri et al., Reference Bizzarri, Albianelli, Argenti, Baldanza, Colacicchi and Napoleone2011).
Two main mollusc associations (MA1: running clear waters with decreasing temperature; MA2: standing or slow current waters, with sporadical stagnant waters) alternate along the section, and their ecological spectra, as well as the ostracod distribution, agree with the sedimentary facies analysis (Tables 1–4). Among molluscs, the presence of markedly warm species is very spotty, as most species seem to tolerate a wide range of temperatures. On the other hand, only in the running-water association (MA1) is a marked cooling trend apparent. As reported, association MA2 largely prevails (Figs. 2 and 3, Tables 2 and 3). Nonetheless, minor variations are found in terms of both species occurrence and relative abundance, and an exact correspondence between ecological characters and facies is not always straightforward. This indicates that (1) in samples attributed to the same facies by means of sedimentology, ecological conditions could be somewhat different; (2) minimal variations of physical parameters are only recorded by fossils; and (3) a time gap between changes in sedimentation parameters and the response of organisms may have occurred.
The palaeofloristic record shows a diversified distribution through the facies associations. Determinable carpological remains concentrate in selected horizons within deposits of associations A and C, whereas they are totally missing in association B. The dominance of herbaceous freshwater macrophytes, accompanied by very few dispersed fruits and seeds produced by local plant assemblages, documents the vegetation living along the banks of a stable lacustrine basin and in temporary water pools.
Through the entire time interval recorded, lacustrine and/or running waters were mainly temperate to cool and, as documented by ostracods (Table 4), with variable salinity.
The overall abundance of the genus Ilyocypris, particularly in lacustrine facies (A1), points to oligohaline waters and to a muddy and vegetated lake bottom, while the wide occurrence of C. torosa could be indicative of dissolved salts in water (Table 4). The first cooling signal of the water, marked by ostracods, occurs very soon inside the lower section (ARQ5: Fig. 2, Tables 2 and 4). This record indicates the persistence of cold water temperature conditions at the lake floor through at least the lower section. According to stratigraphic constrains, this signal corresponds to sediments of late Matuyama age (1.4–1.3 Ma).
Four assemblages dominated by Candonidae and associated with the MA2 mollusc association have been identified (Candonidae events: Figs. 2 and 3), suggesting different environmental conditions. These four events (I–IV: Figs. 2 and 3, Table 2) do not depend on the facies association, but according to Candonidae autoecology (Meisch, Reference Meisch2000: Table 4), they indicate pronounced cool conditions. This “cool” signal is supported by the distribution of charophytes (Tables 2 and 4), which in two cases anticipate (ARQ11) or match (ARQ29) the Candonidae events (Figs. 2 and 3, Table 2). The third Candonidae event (III, ARQ24) occurred where the pollen assemblage points to a temperate-wet interglacial period.
This “cool” signal, particularly for unit 1, needs to be discussed. A shallow lake is expected to hold relatively warm waters, unless it is fed by cool springs (karst, ice melting) and/or the average air temperature is also low. The Candonidae events underline episodes of incipient and more pronounced environmental cooling, well inside the middle–late Matuyama and before the early–middle Pleistocene transition.
Besides the overall mollusc and ostracod record, which indicates incipient and recurrent cooling episodes throughout the section, the sedimentologic signal also needs to be considered. Although long-lasting and stable lacustrine conditions characterize the lower section, with a predominance of A1 and A2 facies (Figs. 2 and 3), minor variations are recorded, corresponding to a general shift from lacustrine and to subaerial alluvial plain conditions across the uppermost section. Though these shifts could be explained in terms of subsidence and/or variations in the sediment input from the catchment area, these palaeoenvironmental changes alternated throughout the basin history, from at least 3.1 Ma to the recent (Colacicchi and Bizzarri, Reference Colacicchi and Bizzarri2008; Bizzarri et al., Reference Bizzarri, Albianelli, Argenti, Baldanza, Colacicchi and Napoleone2011). Thus, variations are probably driven by climatic fluctuations, which superimpose on the general subsidence.
The 200- to 300 ka-long record of the Arquata section not only fits in the general schemes of Italian basins, but also reveals how, during the middle–late Matuyama, a climatic change towards incipient cooling may have acted at the middle latitudes, matching the general increase in the duration of glacial periods that is found in marine records stating at ca. 1.4 Ma (Head and Gibbard, Reference Head and Gibbard2015).
CONCLUSIONS
This multidisciplinary study sheds new light on the middle–late Matuyama interval in central Italy and contributes to the knowledge of the climatic and biotic characters of a long time period that is still poorly known in continental environments. Thus, the Arquata quarry represents a key section for both improving the current stratigraphic schemes and defining the climatic trends of the early Pleistocene record in southern Europe.
Similarly to most palaeoenvironmental records framed within the end of the Olduvai subchron and the beginning of the Jaramillo subchron, the chronology of the sedimentary deposit is largely based on biostratigraphical data, supported by magnetostratigraphical evidence. In the case of Arquata, there is a considerable agreement in the biostratigraphical indications provided by pollen data, carpological remains, and mollusc and ostracod assemblages, all pointing to a time interval between about 1.7 and 1.4 Ma.
The Arquata deposit further increases the rich palaeobotanical record of central Italy and contributes to a better definition of modes and timing of the disappearance of tree taxa in southern Europe. The abundance of extinct tree taxa, indeed, suggests an age corresponding to pre-Jaramillo late early Pleistocene. If compared with other sites in southern Europe, and particularly with the rich record from the Italian peninsula, the floristic data align according to a progressive, although not marked, shift towards cooler and drier conditions. This result agrees with a weak and incipient cooling trend marked by ostracofauna and charophytes, which alternates with temperate-wet (interglacial) phases. These cooling episodes might correspond to the initial phases of increasing strength and duration of glacial stages that occurred during the early–middle Pleistocene transition.
On the whole, this new section from the eastern Tiberino Basin provides valuable interdisciplinary results that may prove useful not only to assess the evolution of the intramountain basins in central Italy, but also to add more elements to the general picture of the succession of biotic and climatic events of the late early Pleistocene, which saw the progressive transition from the Villafranchian to Galerian mammal faunas, increasingly open landscapes, and the expansion of hominins into western Europe.
ACKNOWLEDGMENTS
The authors wish to thank the administration and the workers of Fornaci Briziarelli Marsciano, and in particular Mr. P. Antano for the allowance to visit the quarry and to collect samples. We would also like to thank Dr. M. Vantaggi, Dr. F. Famiani, and Dr. O. Pauselli for support during the repeated sample recoveries. Finally, we are grateful to P. Langdon, D. Booth, T. Utescher, and an anonymous referee for critical comments and help in improving the manuscript.